Assessment of left ventricular systolic function using a non-invasive index of myocardial work in amateur marathon runners
Introduction
As an affordable and low-risk activity, marathons are gaining popularity and have emerged as a national sport, and the number of non-elite recreational runners continues to increase (1). Long-lasting endurance exercise places a hemodynamic load on the left ventricle that is morphologically characterized by an increase in left ventricular (LV) wall thickness, diameter, and mass; these changes are commonly referred to as “athlete’s heart” (2). The development of the so-called “athlete’s heart” requires high-intensity endurance training. However, amateur marathon runners lack the corresponding training experience, raising the question as to whether they will develop the characteristics of the athlete’s heart.
Some studies have indicated that marathon exercise can induce a hyperphysiological stress response, which may result in heart fatigue and pathological changes such as the abnormal thickening of the interventricular septum (3). This effect is particularly pronounced among amateur marathon runners who lack professional training (4,5). Other studies have found that abnormal ventricular remodeling caused by excessive endurance exercise can induce arrhythmias, which is the most common cardiovascular problem in experienced endurance athletes (6,7). Thus, research needs to be conducted to investigate whether the subclinical alterations in cardiac structure and function in amateur marathon runners should be assessed using the conventional criteria employed to evaluate professional endurance athletes or ordinary adults. Alternatively, it may be necessary to develop a novel standard specifically tailored for amateur marathon runners, as there may be some overlap between the physiological adaptation of the athlete’s heart and some pathological conditions (8), and amateur marathon runners may also possess certain heart characteristics of professional endurance athletes.
Due to its comprehensive, rapid, non-invasive, safe, reproducible, and cost-effective advantages, echocardiography has gained widespread popularity as an examination technology. The conventional echocardiographic parameters for assessing left ventricular systolic function (LVSF) primarily include left ventricular ejection fraction (LVEF), stroke volume (SV), mitral annular systolic peak velocity (S’), and left ventricular fractional shortening (LVFS). Among these indicators, LVEF is the most commonly used parameter and is typically obtained using the Simpson method (9). The assessment of LVEF is based on a theoretical model of LV geometry and calculated using a formula; however, LVEF can be influenced by cardiac geometry, heart rate, preload, and afterload, and is susceptible to imprecise values and inter-/intra-observer variability (10).
Two-dimensional speckle-tracking echocardiography (2D-STE) is based on gray-scale imaging and tracks the spatial motion and geometric displacement of acoustic speckles in the myocardium frame by frame (11). It provides quantitative measurements of tissue velocity, displacement, strain, the strain rate, and the rotation angle, allowing for the detection of early subtle abnormalities in myocardial function. This technique enables the quantification of both segmental and global myocardial deformation (12). However, this technique has load-dependent limitations, and may lead to the inaccurate assessment of myocardial function when ventricular afterload is elevated (13).
Russell et al. (14) found that there was a strong correlation between the LV pressure curve obtained by non-invasive methods (e.g., brachial artery cuff pressure) and the LV pressure curve obtained by invasive methods (r=0.99, P<0.001). By combining the non-invasive blood pressure curve with 2D-STE, the authors introduced the concept of the non-invasive pressure-strain loop (PSL). The PSL integrates myocardial strain and ventricular pressure, effectively eliminating load dependence when assessing myocardial function. This approach allows for the more objective and accurate analysis of global and segmental myocardial function. Further, it has a high correlation (r=0.99) with the pressure-volume curve measured by cardiac catheterization while offering the advantage of being non-invasive, making it safer and more widely applicable.
Hubert et al. (13) confirmed that the estimate of LV myocardial work (MW) derived from the PSL area by Russell’s method is accurate. The PSL technique has been proven to be useful for the quantitative assessment of various diseases, such as hypertension, acute coronary syndrome, and heart failure with preserved ejection fraction, and has shown good diagnostic performance (15-17). If certain MW parameters undergo changes in amateur marathon runners it may be possible for sports medicine physicians to use PSL technology on amateur marathon athletes to perform focused echocardiography specifically rather than only performing general physical examinations such as electrocardiograms (18).
The objective of this study was to identify a more sensitive indicator of early changes in LVSF among amateur marathon runners using LV PSL technology. This study also aimed to evaluate the diagnostic efficacy of this indicator compared with conventional echocardiographic parameters, providing a basis for early detection of cardiac function changes related to marathon exercise. We present this article in accordance with the STROBE reporting checklist (available at https://qims.amegroups.com/article/view/10.21037/qims-24-657/rc).
Methods
Study population
A total of 35 amateur marathon runners were recruited for this study from October 2021 to August 2023. The participants had an average age of 35.2±6.7 years, and were all members of a local running club. Clinical data, such as age, body mass index (BMI), body surface area (BSA), heart rate and blood pressure, were recorded. All participants underwent echocardiography at rest at least a week before the marathon. To be eligible for inclusion in this study, the participants had to meet the following inclusion criteria: (I) have participated in marathon running for more than 1 year, and less than 3 years; (II) have completed at least one formal full marathon (42.195 km), (III) have a running frequency of ≥3 times per week, with a distance ≥10 km for each run; and (IV) have undergone no strenuous exercise in the week before the examination. Marathon runners with a history of congenital heart disease, hypertension, valvular heart disease, diabetes, kidney disease, or other systemic diseases were not included in the study. Additionally, marathon runners who had engaged in strength sports for an extended period or were professional athletes were also excluded.
In addition, 33 (24 male and 9 female) individuals with no history of long-term intensive training were selected from a physical examination center as healthy controls. The participants had an average age of 36.5±6.4 years. The participants were required to have high quality images for the myocardial speckle-tracking analysis, and no cardiovascular diseases, such as arrhythmia, valvular stenosis or regurgitation.
The study was conducted in accordance with the Declaration of Helsinki (as revised in 2013). The study was approved by the Scientific Research Ethics Committee of the Affiliated Hospital of Hangzhou Normal University (No. 2020HS-001), and informed consent was obtained from all the participants.
Echocardiographic analysis
Transthoracic echocardiography was performed to obtain images for analysis using the GE Vivid E9 ultrasound system equipped with a M5S heart probe (frequency 1.0–3.3 MHz), and the Echo PAC v. 204 workstation (GE Healthcare, Vingmed Ultrasound) was employed to conduct the echocardiographic analysis. For the scanning, the participants were placed in a left lateral decubitus position, and the electrocardiogram was connected synchronously under steadily breathe, and the heart rate of each participant was recorded. The height and weight of each participant were also recorded. Standard two-dimensional (2D) gray-scale dynamic images consisting of three consecutive cardiac cycles in the long-axis, apical 3-, 2-, and 4-chamber views were collected, and then transferred to the workstation for offline analysis.
Left ventricular end-diastolic diameter (LVEDd), diastolic interventricular septal thickness (IVSTd), and left ventricular diastolic posterior wall thickness (LVPWTd) were obtained in the parasternal long-axis section, and relative wall thickness (RWT) was calculated using the following formula: (IVSTd + PWTd)/LVDd. Left ventricular end-diastolic volume (LVEDV) and SV were measured using Simpson’s biplane method, and LVEF was calculated by the software and indexed to BSA. Left ventricular mass (LVM) was calculated using the standard cube formula: LVM(g) =0.8×1.04×[(IVSTd + PWTd + LVEDd)3 − LVEDd3] + 0.6. While the left ventricular mass index (LVMI) was calculated as follows: LVMI: LVM/BSA (g/m2) (19).
Strain analysis by 2D-STE
2D gray-scale dynamic images of apical 3-, 4-, and 2-chamber views (>50 frames/s) were obtained, and the data of three consecutive cardiac cycles with stable heart rates were gathered and saved. The images were imported into the Echo PAC workstation, and switched conventional mode to the automatic functional imaging (AFI) analysis mode. The apical 3-, 4-, and 2-chamber views of the left ventricle were selected. AFI automatically tracked the LV endocardial and epicardial boundaries in the three apical dynamic images; manual adjustments were performed as necessary. The global longitudinal strain (GLS) of the left ventricle was calculated systematically from the average value of the three views, including 17 segments of the myocardium. Longitudinal peak strain dispersion (PSD) was obtained to evaluate the synchronization of myocardial contraction in the left ventricle.
Quantification analysis of LV MW
The mitral valve closing time, aortic valve closing time, aortic valve opening time, and mitral valve opening time were determined in the LV 3-chamber view. Peak arterial pressure is assumed to be a substitute for peak systolic LV pressure, and was recorded as the brachial cuff systolic pressure measured immediately before the echocardiography. The LV MW parameters were automatically computed by the system, including the global work index (GWI), global constructive work (GCW), global wasted work (GWW), and global work efficiency (GWE) after inputting the participant’s blood pressure (Figure 1). The GWI (mmHg%) describes the total work done during LV systole from mitral valve closure to mitral valve opening. GCW (mmHg%) describes the work that contributes to ventricular ejection, including systolic myocardial shortening and isovolumic diastolic myocardial elongation. GWW (mmHg%) describes the work that is not conducive to ejection, including systolic myocardial elongation and isovolumic diastolic period shortening. GWE is the ratio of GCW to the sum of the GCW and GWW. All the parameters were measured three times, and the average value of each parameter was taken as the final result.
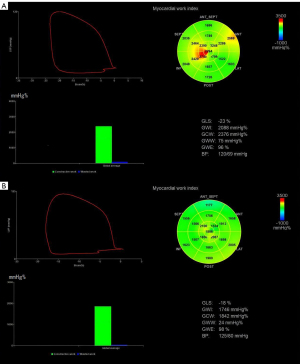
Statistical analysis
The statistical analysis was conducted using SPSS software version 26.0 (IBM, Chicago, IL, USA). The normality of the measured data was examined using the Shapiro-Wilk test. Normally distributed continuous variables are presented as the mean ± standard deviation, while non-normally distributed continuous variables are presented as the median with interquartile range (IQR). The categorical variables are presented as the frequency and percentage, and were compared using Fisher’s exact test. For comparisons between two groups, the LSD T-test was used for the normally distributed variables and the Mann-Whitney U test was used for the non-normally distributed variables. Differences between groups were compared using a one-way analysis of variance for the continuous variables and the Kruskal-Wallis test for the skewed variables. A Pearson correlation analysis was performed to examine the conventional echocardiography and MW parameters. Receiver operating characteristic (ROC) curves were used to identify the best parameters in athletes with sensitivity, specificity, and the area under the curve (AUC) of the ROC curve for predicting LV systolic function. Intra-observer variability and inter-observer variability were assessed in 10 randomly selected subjects from the amateur marathon runner group and control group, respectively, by two cardiac sonographers with over 15 years of experience. Intraclass correlation coefficients (ICCs) and the Bland-Altman method were used to evaluate the consistency of the MW parameters between and within the observers, and an inter/intraclass correlation >0.70 was regarded as a good correlation. A P value <0.05 (two-sided) was considered statistically significant.
Results
Clinical and conventional echocardiographic characteristics
A total of 35 amateur marathon runners and 33 healthy controls were included in the statistical analysis (Figure 2). There were no missing data in this text. The demographic data and conventional echocardiographic parameters of the participants are summarized in Tables 1 and 2. There were no significant differences between the two groups in terms of age, BMI, BSA, systolic blood pressure, and diastolic blood pressure (P>0.05). The heart rate of the amateur marathon runner group was significantly lower than that of the control group (P<0.05). Compared with the control group, the IVSTd, LVPWTd, LVEDd, LVMI, and LVEDV of the amateur marathon runner group were significantly larger (P<0.05), but the RWT, SV, and EF were similar (P>0.05).
Table 1
Variable | Marathon group (n=35) | Control group (n=33) | P value |
---|---|---|---|
Age (years) | 35.2±6.7 | 36.5±6.4 | 0.427 |
Male sex | 26 (74.3) | 24 (72.7) | 0.884 |
Height (m) | 169.5±6.9 | 170.8±6.3 | 0.433 |
Weight (kg) | 62.2±7.9 | 64.9±7.7 | 0.151 |
Body mass index (kg/m2) | 21.6±1.5 | 22.2±1.6 | 0.097 |
Body surface area (m2) | 1.7±0.1 | 1.7±0.1 | 0.838 |
Heart rate (bpm) | 61.8±8.4 | 76.9±6.8 | <0.001 |
Systolic blood pressure (mmHg) | 116.7±5.6 | 114.8±4.4 | 0.127 |
Diastolic blood pressure (mmHg) | 73.9±5.8 | 74.8±6.0 | 0.531 |
Data are expressed as the mean ± standard deviation or number (percentage). P<0.05, indicating a significantly different result from the control group.
Table 2
Variable | Marathon group (n=35) | Control group (n=33) | P value |
---|---|---|---|
IVSTd (cm) | 0.88±0.12 | 0.81±0.99 | <0.05 |
LVPWTd (cm) | 0.80±0.09 | 0.74±0.07 | <0.05 |
LVEDd (cm) | 5.09±0.38 | 4.71±0.37 | <0.001 |
RWT | 0.33±0.04 | 0.33±0.04 | 0.945 |
LVMI (g/m2) | 88.06±14.45 | 68.63±11.97 | <0.001 |
LVEDV (mL) | 124.83±21.67 | 103.20±20.14 | <0.001 |
SV (mL) | 69.83±14.65 | 65.74±13.37 | 0.234 |
EF (%) | 64.00±4.22 | 63.36±3.64 | 0.507 |
Data are expressed as the mean ± standard deviation. P<0.05, indicating a significantly different result from the control group. IVSTd, diastolic interventricular septal thickness; LVPWTd, left ventricular diastolic posterior wall thickness; LVEDd, left ventricular end-diastolic diameter; RWT, relative wall thickness; LVMI, left ventricular mass index; LVEDV, left ventricular end-diastolic volume; SV, stroke volume; EF, ejection fraction.
MW and strain characteristics
The results of the comparisons of the LV strain and MW parameters between the two groups are summarized in Table 3. Notably, GWW was higher and GWE was lower in the amateur marathon runner group than the control group (P<0.05). However, there were no statistically significant differences between the amateur marathon runner group and control group in terms of the GLS, GWI, GCW, and PSD.
Table 3
Variable | Marathon group (n=35) | Control group (n=33) | P value |
---|---|---|---|
GWI (mmHg%) | 1,967.80±218.01 | 1,990.19±183.66 | 0.649 |
GCW (mmHg%) | 2,260.69±236.99 | 2,237.71±186.02 | 0.659 |
GWW (mmHg%) | 91.31±45.20 | 70.29±33.22 | <0.05 |
GWE (%) | 95.54±1.89 | 96.81±1.61 | <0.05 |
GLS (%) | 19.74±2.03 | 20.55±1.90 | 0.095 |
PSD (msec) | 38.63±7.53 | 38.00±6.83 | 0.799 |
Data are expressed as the mean ± standard deviation. P<0.05, indicating a significantly different result from the control group. GWI, global work index; GCW, global constructive work; GWW, global wasted work; GWE, global work efficiency; GLS, global longitudinal strain; PSD, peak strain dispersion.
Analysis of the ROC curves for each parameter
The ROC curves were used to assess the diagnostic performance of different parameters in identifying subclinical changes in LVSF. The ROC curve analysis showed that the maximum area under the GWE curve was 0.681 [95% confidence interval (CI): 0.551–0.812] (Table 4, Figure 3), the optimal cut-off value was 96.14%, and the sensitivity and specificity were 75.8% and 62.9%, respectively.
Table 4
Variable | AUC (SE) | 95% CI | Cut-off value | Sensitivity | Specificity |
---|---|---|---|---|---|
GWI (mmHg%) | 0.539 (0.073) | 0.396–0.681 | 1,986.63 | 54.5% | 71.4% |
GCW (mmHg%) | 0.504 (0.071) | 0.364–0.644 | 2,500.12 | 25.7% | 90.9% |
GWW (mmHg%) | 0.612 (0.069) | 0.477–0.748 | 109.90 | 42.9% | 90.9% |
GWE (%) | 0.681 (0.066)* | 0.551–0.812 | 96.14 | 75.8% | 62.9% |
GLS (%) | 0.610 (0.069) | 0.475–0.744 | 20.09 | 68.6% | 57.6% |
*, P<0.05, significantly different from the control group. ROC, receiver operating characteristic; AUC, area under the curve; SE, standard error; CI, confidence interval; GWI, global work index; GCW, global constructive work; GWW, global wasted work; GWE, global work efficiency; GLS, global longitudinal strain.
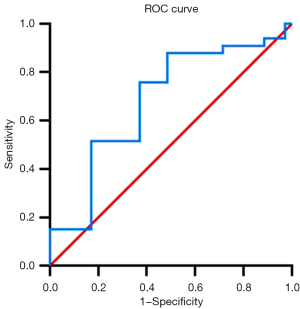
Correlation analysis of amateur marathon runners
The results of the correlation analysis are shown in Table 5 and Figure 4. GWE was correlated with all parameters. The IVSTd, LVPWTd and GLS were correlated with GWW (all P<0.05).
Table 5
Variable | GWI (mmHg%) | GCW (mmHg%) | GWW (mmHg%) | GWE (%) |
---|---|---|---|---|
IVSTd (cm) | 0.286 | 0.379* | 0.352* | −0.416* |
LVPWTd (cm) | −0.315 | −0.274 | 0.395* | −0.674* |
LVMI (g/m2) | 0.024 | 0.100 | 0.306 | −0.393* |
EF (%) | 0.124 | 0.069 | −0.245 | 0.406* |
GLS (%) | 0.156 | 0.107 | −0.344* | 0.502* |
PSD (msec) | −0.103 | −0.158 | 0.132 | −0.424* |
Values are the correlation coefficient (r) of amateur marathon runners. *, P<0.05, the correlation was statistically significant. GWI, global work index; GCW, global constructive work; GWW, global wasted work; GWE, global work efficiency; IVSTd, diastolic interventricular septal thickness; LVPWTd, left ventricular diastolic posterior wall thickness; LVMI, left ventricular mass index; EF, ejection fraction; GLS, global longitudinal strain; PSD, peak strain dispersion.
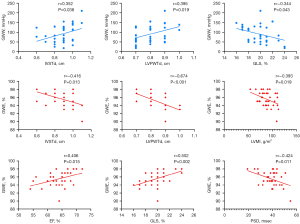
Reproducibility test
The intra-observer correlation coefficients of the GLS, GWI, GCW, GWW, and GWE were 0.777, 0.804, 0.912, 0.708, and 0.754, respectively. The inter-observer correlation coefficients of the GLS, GWI, GCW, GWW, and GWE were 0.778, 0.808, 0.821, 0.713, and 0.759, respectively. The MW parameters showed good repeatability and reproducibility (Figure 5).
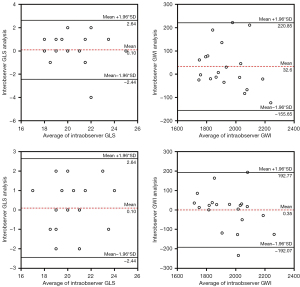
Discussion
This study used LV PSL technology combined with conventional echocardiographic indicators to evaluate the impact of marathon exercise on LVSF, and further analyzed the correlation between conventional echocardiographic indicators evaluating LV structure and function, and LV MW indicators. The main findings are as follows: (I) the parameters of LV structure and volume, including IVSTd, LVPWTd, LVEDd, LVEDV, and LVMI, were significantly higher in the amateur marathon runner group than the control group; (II) there were no significant differences in the GLS, EF, and SV, which were used to evaluate LVSF, between the amateur marathon runner group and control group; (III) the results of the PSL showed that GWW was significantly higher while GWE was statistically lower in the endurance group than the control group, and there were no significant differences in the other MW indicators. As an indicator of MW, GWE has high specificity and sensitivity, and has a strong correlation with the traditional indicators of LV structure and function.
In endurance athletes who have engaged in long-term high-intensity aerobic training, ventricular remodeling is mainly characterized by a LV diameter increase, accompanied by proportionally increased wall thickness and SV due to volume overload, and LV eccentric hypertrophy (20). A meta-analysis showed that the structural remodeling of the left ventricle induced by endurance training occurs relatively early, is rapid and is more pronounced in male adults than female adults (21).
The results of this study showed that for amateur marathon runners without professional training, the IVSTd, LVPWTd, LVEDd, LVEDV and LVMI are increased, indicating that the left ventricle still undergoes physiological remodeling even in amateur athletes with short exposure to marathon sports, which is consistent with previous findings on endurance athletes (22). The increase in capacity load during early marathon training leads to an increase in the LV diameter and RWT to maintain the LVSF required by exercise. However, this expansion is physiological, and is different from the abnormal structural expansion caused by some diseases, such as hypertrophic cardiomyopathy, as this exercise-induced structural remodeling is proportional to the increase in LVEDV (23).
In a complete cardiac cycle, the movement of the left ventricle is a relatively complex process. The LV myocardium is formed by the reverse spiral fibers of the endomyocardium and epicardium, and the annular fibers of the middle layer. According to the anatomical double-ring arrangement of the myocardial band, the change in LV volume is produced by the reciprocating torsion movement of the myocardial band. During contraction, the bottom of the heart moves spirally toward the apex, while the apex remains relatively stationary (24). The longitudinal movement of the left ventricle in the long-axis direction accounts for 60% of the LV SV, while 60% of the ejection capacity of the left ventricle is derived from the contraction of its inner longitudinal muscle fibers (25).
High-intensity endurance training can lead to LV wall thickening and significantly increase myocardial oxygen consumption. However, the number of coronary arteries supplying the hypertrophied myocardium does not change significantly, and blood supply cannot be increased accordingly, resulting in relative myocardial ischemia, particularly in the subendocardial myocardium, which is most susceptible to microcirculation dysfunction and myocardial fibrosis (26-28).
The assessment of MW using PSL has been shown to accurately reflect the metabolic demand and oxygen consumption of the myocardium (14,29). Our MW analysis results revealed significantly higher GWW in the amateur marathon runner group than the control group, and lower GWE. This increase of GWW may be related to arrhythmia, myocardial desynchrony or impaired relaxation (30). In general, high-intensity marathon exercise can significantly augment cardiac output to more than five-fold the normal level, reaching 25 L/min (31). Amateur marathon runners lack the cardiac adaptability to long-distance marathons developed by professional athletes through extensive endurance training, resulting in increased strain on the coronary vessels and electrical conduction system, leading to elevated myocardial metabolism and oxygen consumption. Additionally, the enlargement of the LV cavity contributes to a reduction in subendocardial microvessels that regulate each segment of the myocardium, resulting in a decrease in wall stress and a potential decrease in LVSF (32).
In this study, there was no statistically significant difference in PSD between the amateur marathon runner group and the control group, but a slight numerical increase was observed. This may be due to the different degrees of thickening from the apex to the base of the heart caused by endurance exercise. Due to the influence of morphology and the local curvature radius, the LV base may be preferentially affected by the increase in wall stress after volume overload, and the wall thickening may be greater. This incongruity may lead to an increase in GWW and a decrease in GWE.
GLS can provide quantitative monitoring of the degree of LV remodeling and systolic function changes. A study using speckle-tracking technology found that endurance exercise can cause a decrease in LV GLS, which is correlated with an increase in LVEDV and BSA (33). Our study results showed that there were no significant differences in the GLS, EF, and SV between the control group and the marathon runner group, indicating that compared with traditional indicators for evaluating LV systolic function, PSL can detect subclinical changes in LVSF at an earlier stage. The ROC analysis of the LV MW parameters showed that GWE had some value in identifying subclinical changes in LV systolic function (AUC =0.681), which was better than GLS and other MW indicators. The correlation analysis showed that GWE had a good correlation with LV structure and the function indicators, indicating the reliability and accuracy of PSL technology in the diagnosis of early LV function changes.
A previous study suggested that screening echocardiography had an additional value (about 10% more) in identifying participants with cardiovascular abnormalities (34). Similarly, the inclusion of MW parameters in preparticipation screening and the performance of echocardiogram screening would provide some guidance for the training of amateur marathon athletes. It could benefit both professional and amateur athletes with variable blood pressure and loading conditions and at different stages of their training program (35). Further research needs to be conducted on the frequency of PPS to validate the findings. In addition, there was a significant negative correlation between GLS and GWW, which indirectly indicates that GLS has certain diagnostic value for LV systolic function changes.
Limitations
This study only evaluated early amateur marathon runners; however, the sample size was limited and only a small number of the runners were female, which might interfere with the conclusions drawn. Errors may arise in measuring upper arm brachial artery blood pressure using cuffs and sphygmomanometers. This study only analyzed the MW of amateur marathon runners at rest. The changes in MW before and after marathons and its relationship with training years requires further study. PSL technology assumes that all segments of the ventricular wall are equal in thickness, so the thickening of the ventricular wall in a certain segment may lead to errors in MW parameters. Additional research is needed to elucidate the influence of ventricular wall thickness variations on MW.
Conclusions
The results of this study revealed that early amateur marathon runners with short training and competition time not only show LV eccentric remodeling, but also have increased GWW and decreased GWE, which are well correlated with GLS. GWE shows accuracy and certain diagnostic efficacy. As a novel indicator of LVSF, LV MW is more sensitive than GLS, is less load-dependent, and has emerged as a promising parameter for assessing potential changes in early LV systolic function in amateur athletes.
Acknowledgments
Funding: This project was funded by
Footnote
Reporting Checklist: The authors have completed the STROBE reporting checklist. Available at https://qims.amegroups.com/article/view/10.21037/qims-24-657/rc
Conflicts of Interest: All authors have completed the ICMJE uniform disclosure form (available at https://qims.amegroups.com/article/view/10.21037/qims-24-657/coif). The authors have no conflicts of interest to declare.
Ethical Statement: The authors are accountable for all aspects of the work in ensuring that questions related to the accuracy or integrity of any part of the work are appropriately investigated and resolved. The study was conducted in accordance with the Declaration of Helsinki (as revised in 2013), and it was approved by the Scientific Research Ethics Committee of the Affiliated Hospital of Hangzhou Normal University (No. 2020HS-001). Written informed consent was obtained from all participants.
Open Access Statement: This is an Open Access article distributed in accordance with the Creative Commons Attribution-NonCommercial-NoDerivs 4.0 International License (CC BY-NC-ND 4.0), which permits the non-commercial replication and distribution of the article with the strict proviso that no changes or edits are made and the original work is properly cited (including links to both the formal publication through the relevant DOI and the license). See: https://creativecommons.org/licenses/by-nc-nd/4.0/.
References
- Predel HG. Marathon run: cardiovascular adaptation and cardiovascular risk. Eur Heart J 2014;35:3091-8. [Crossref] [PubMed]
- Maron BJ. Structural features of the athlete heart as defined by echocardiography. J Am Coll Cardiol 1986;7:190-203. [Crossref] [PubMed]
- Kim YJ, Park KM. Effects of Super-ultramarathon Running on Cardiac Structure and Function in Middle-aged Men. J Cardiovasc Imaging 2020;28:202-10. [Crossref] [PubMed]
- Lewicka-Potocka Z, Dąbrowska-Kugacka A, Lewicka E, Kaleta AM, Dorniak K, Daniłowicz-Szymanowicz L, Fijałkowski M, Nabiałek-Trojanowska I, Ratkowski W, Potocki W, Raczak G. The "athlete's heart" features in amateur male marathon runners. Cardiol J 2021;28:707-15. [Crossref] [PubMed]
- Sengupta SP, Mahure C, Mungulmare K, Grewal HK, Bansal M. Myocardial fatigue in recreational marathon runners: A speckle-tracking echocardiography study. Indian Heart J 2018;70:S229-34. [Crossref] [PubMed]
- Goel R, Majeed F, Vogel R, Corretti MC, Weir M, Mangano C, White C, Plotnick GD, Miller M. Exercise-induced hypertension, endothelial dysfunction, and coronary artery disease in a marathon runner. Am J Cardiol 2007;99:743-4. [Crossref] [PubMed]
- O'Keefe JH, Patil HR, Lavie CJ, Magalski A, Vogel RA, McCullough PA. Potential adverse cardiovascular effects from excessive endurance exercise. Mayo Clin Proc 2012;87:587-95. [Crossref] [PubMed]
- Palermi S, Cavarretta E, D'Ascenzi F, Castelletti S, Ricci F, Vecchiato M, Serio A, Cavigli L, Bossone E, Limongelli G, Biffi A, Monda E, La Gerche A, Baggish A, D'Andrea A. Athlete's Heart: A Cardiovascular Step-By-Step Multimodality Approach. Rev Cardiovasc Med 2023;24:151. [Crossref] [PubMed]
- Kim WC, Beqiri A, Lewandowski AJ, Puyol-Antón E, Markham DC, King AP, Leeson P, Lamata P. Beyond Simpson's Rule: Accounting for Orientation and Ellipticity Assumptions. Ultrasound Med Biol 2022;48:2476-85. [Crossref] [PubMed]
- Monge García MI, Jian Z, Settels JJ, Hunley C, Cecconi M, Hatib F, Pinsky MR. Determinants of left ventricular ejection fraction and a novel method to improve its assessment of myocardial contractility. Ann Intensive Care 2019;9:48. [Crossref] [PubMed]
- Blessberger H, Binder T. NON-invasive imaging: Two dimensional speckle tracking echocardiography: basic principles. Heart 2010;96:716-22. [Crossref] [PubMed]
- Mizuguchi Y, Oishi Y, Miyoshi H, Iuchi A, Nagase N, Oki T. The functional role of longitudinal, circumferential, and radial myocardial deformation for regulating the early impairment of left ventricular contraction and relaxation in patients with cardiovascular risk factors: a study with two-dimensional strain imaging. J Am Soc Echocardiogr 2008;21:1138-44. [Crossref] [PubMed]
- Hubert A, Le Rolle V, Leclercq C, Galli E, Samset E, Casset C, Mabo P, Hernandez A, Donal E. Estimation of myocardial work from pressure-strain loops analysis: an experimental evaluation. Eur Heart J Cardiovasc Imaging 2018;19:1372-9. [Crossref] [PubMed]
- Russell K, Eriksen M, Aaberge L, Wilhelmsen N, Skulstad H, Remme EW, Haugaa KH, Opdahl A, Fjeld JG, Gjesdal O, Edvardsen T, Smiseth OA. A novel clinical method for quantification of regional left ventricular pressure-strain loop area: a non-invasive index of myocardial work. Eur Heart J 2012;33:724-33. [Crossref] [PubMed]
- Boe E, Russell K, Eek C, Eriksen M, Remme EW, Smiseth OA, Skulstad H. Non-invasive myocardial work index identifies acute coronary occlusion in patients with non-ST-segment elevation-acute coronary syndrome. Eur Heart J Cardiovasc Imaging 2015;16:1247-55. [Crossref] [PubMed]
- Tadic M, Cuspidi C, Pencic B, Vukomanovic V, Taddei S, Grassi G, Celic V. Association between myocardial work and functional capacity in patients with arterial hypertension: an echocardiographic study. Blood Press 2021;30:188-95. [Crossref] [PubMed]
- Tomoaia R, Beyer RS, Zdrenghea D, Dadarlat-Pop A, Cismaru G, Gusetu G, Rosu R, Serban A, Pop D. Global work index by non-invasive pressure-strain loops: a novel parameter to assess left ventricular performance in the early stages of heart failure with preserved or mid-range ejection fraction after acute myocardial infarction. Med Ultrason 2021;23:62-9. [Crossref] [PubMed]
- Palermi S, Serio A, Vecchiato M, Sirico F, Gambardella F, Ricci F, Iodice F, Radmilovic J, Russo V, D'Andrea A. Potential role of an athlete-focused echocardiogram in sports eligibility. World J Cardiol 2021;13:271-97. [Crossref] [PubMed]
- Jafary FH. Devereux formula for left ventricular mass--be careful to use the right units of measurement. J Am Soc Echocardiogr 2007;20:783. [Crossref] [PubMed]
- D'Andrea A, Caso P, Scarafile R, Salerno G, De Corato G, Mita C, Di Salvo G, Allocca F, Colonna D, Caprile M, Ascione L, Cuomo S, Calabrò R. Biventricular myocardial adaptation to different training protocols in competitive master athletes. Int J Cardiol 2007;115:342-9. [Crossref] [PubMed]
- Morrison BN, George K, Kreiter E, Dixon D, Rebello L, Massarotto RJ, Cote AT. Effects of endurance exercise training on left ventricular structure in healthy adults: a systematic review and meta-analysis. Eur J Prev Cardiol 2023;30:772-93. [Crossref] [PubMed]
- Vitarelli A, Capotosto L, Placanica G, Caranci F, Pergolini M, Zardo F, Martino F, De Chiara S, Vitarelli M. Comprehensive assessment of biventricular function and aortic stiffness in athletes with different forms of training by three-dimensional echocardiography and strain imaging. Eur Heart J Cardiovasc Imaging 2013;14:1010-20. [Crossref] [PubMed]
- Hiemstra YL, van der Bijl P, El Mahdiui M, Bax JJ, Delgado V, Marsan NA. Myocardial Work in Nonobstructive Hypertrophic Cardiomyopathy: Implications for Outcome. J Am Soc Echocardiogr 2020;33:1201-8. [Crossref] [PubMed]
- Carreras F, Ballester M, Pujadas S, Leta R, Pons-Llado G. Morphological and functional evidences of the helical heart from non-invasive cardiac imaging. Eur J Cardiothorac Surg 2006;29:S50-5. [Crossref] [PubMed]
- Carlsson M, Ugander M, Mosén H, Buhre T, Arheden H. Atrioventricular plane displacement is the major contributor to left ventricular pumping in healthy adults, athletes, and patients with dilated cardiomyopathy. Am J Physiol Heart Circ Physiol 2007;292:H1452-9. [Crossref] [PubMed]
- Suzuki R, Mochizuki Y, Yoshimatsu H, Niina A, Teshima T, Matsumoto H, Koyama H. Layer-specific myocardial function in asymptomatic cats with obstructive hypertrophic cardiomyopathy assessed using 2-dimensional speckle-tracking echocardiography. J Vet Intern Med 2019;33:37-45. [Crossref] [PubMed]
- Tsai WC, Liu YW, Huang YY, Lin CC, Lee CH, Tsai LM. Diagnostic value of segmental longitudinal strain by automated function imaging in coronary artery disease without left ventricular dysfunction. J Am Soc Echocardiogr 2010;23:1183-9. [Crossref] [PubMed]
- Ran H, Yao Y, Wan L, Ren J, Sheng Z, Zhang P, Schneider M. Characterizing stenosis severity of coronary heart disease by myocardial work measurement in patients with preserved ejection fraction. Quant Imaging Med Surg 2023;13:5022-33. [Crossref] [PubMed]
- Edwards NFA, Scalia GM, Shiino K, Sabapathy S, Anderson B, Chamberlain R, Khandheria BK, Chan J. Global Myocardial Work Is Superior to Global Longitudinal Strain to Predict Significant Coronary Artery Disease in Patients With Normal Left Ventricular Function and Wall Motion. J Am Soc Echocardiogr 2019;32:947-57. [Crossref] [PubMed]
- D'Andrea A, Radmilovic J, Carbone A, Mandoli GE, Santoro C, Evola V, Bandera F, D'Ascenzi F, Bossone E, Galderisi M, Cameli MEchocardiography Study Group of the Italian Society of Cardiology. Speckle tracking evaluation in endurance athletes: the "optimal" myocardial work. Int J Cardiovasc Imaging 2020;36:1679-88. [Crossref] [PubMed]
- Pelliccia A, Culasso F, Di Paolo FM, Maron BJ. Physiologic left ventricular cavity dilatation in elite athletes. Ann Intern Med 1999;130:23-31. [Crossref] [PubMed]
- Pelliccia A, Maron MS, Maron BJ. Assessment of left ventricular hypertrophy in a trained athlete: differential diagnosis of physiologic athlete's heart from pathologic hypertrophy. Prog Cardiovasc Dis 2012;54:387-96. [Crossref] [PubMed]
- Szauder I, Kovács A, Pavlik G. Comparison of left ventricular mechanics in runners versus bodybuilders using speckle tracking echocardiography. Cardiovasc Ultrasound 2015;13:7. [Crossref] [PubMed]
- Donati F, Guicciardi C, Lodi E, Fernando F, Palermi S, Modena MG, Biffi A. Echocardiography in the preparticipation screening: an old topic revisited. J Cardiovasc Med (Hagerstown) 2023;24:297-301. [Crossref] [PubMed]
- D'Andrea A, Sperlongano S, Russo V, D'Ascenzi F, Benfari G, Renon F, Palermi S, Ilardi F, Giallauria F, Limongelli G, Bossone E. The Role of Multimodality Imaging in Athlete's Heart Diagnosis: Current Status and Future Directions. J Clin Med 2021;10:5126. [Crossref] [PubMed]