Coronary computed tomography angiography for assessing the coronary artery and predicting adverse cardiovascular events in patients with thoracic malignancies
Introduction
Thoracic malignancies, which mainly include lung, esophageal, and breast cancers (1), are primarily treated with chemotherapy and chemoradiotherapy (2-4). However, these treatments exert toxic effects on coronary arteries, leading to hemodynamic alterations, lipid accumulation, and chronic inflammation, which can lead to major adverse cardiovascular events (MACEs), thereby significantly decreasing survival in patients with thoracic malignancies (5-7). Therefore, it is crucial to monitor the damage of coronary arteries and the associated MACEs in patients with thoracic malignancies before and after chemotherapy or chemoradiotherapy.
Coronary computed tomography angiography (CCTA) is a noninvasive imaging modality that is commonly used for diagnosing coronary artery disease (CAD) (8). Recent studies have observed that the coronary artery calcium (CAC) score derived from CCTA is associated with MACEs in patients with breast cancer or lung cancer who undergo radiotherapy (9,10). The Coronary Artery Disease-Reporting and Data System (CAD-RADS) has been demonstrated to predict MACEs in patients with chest pain (11). However, little is known about the value of the CAC score and the CAD-RADS classification in evaluating the risk of MACEs in patients with thoracic malignancies undergoing chemotherapy or chemoradiotherapy (5).
The fat attenuation index (FAI) derived from CCTA has been introduced as a novel imaging biomarker for evaluating coronary arterial inflammation (12). The FAI has been used to track the response to biologic therapy for CAD in psoriasis (13), and a higher FAI is associated with an increased risk of MACEs (14). Nevertheless, it is unclear whether the FAI can be used to identify coronary artery injury and whether the FAI is associated with MACEs in patients with thoracic malignancies treated by chemotherapy or chemoradiotherapy.
Data for the comprehensive evaluation of the longitudinal changes in coronary artery in patients with thoracic malignancies, as well as their association with MACEs, remain lacking. Therefore, we aimed to investigate the longitudinal changes in coronary artery using CCTA and to determine their association with MACEs in patients with thoracic malignancies receiving chemotherapy or chemoradiotherapy. We present this article in accordance with the STROBE reporting checklist (available at https://qims.amegroups.com/article/view/10.21037/qims-24-944/rc).
Methods
Study population
This retrospective, single-center study was conducted in accordance with the Declaration of Helsinki (as revised in 2013) and was approved by the institutional ethics committee of Chongqing University Cancer Hospital (No. CZLS2023017-A). The requirement for written informed consent was waived due to the retrospective nature of the analysis. The data that support the findings of this study are available from the corresponding author upon reasonable request.
From June 2013 to May 2019, consecutive patients with thoracic malignancies undergoing CCTA at Chongqing University Cancer Hospital were enrolled. The inclusion criteria were as follows: (I) lung cancer, esophageal cancer, or breast cancer with pathological confirmation and an estimated survival time of at least 1 year; and (II) stable typical/atypical chest pain or angina-like symptoms without confirmed CAD or with confirmed CAD. Meanwhile, the exclusion criteria were as follows: (I) no chemotherapy or chemoradiotherapy; (II) a history of allergy to computed tomography (CT) contrast agents; (III) incomplete baseline, treatment, and/or follow-up information; and (IV) presence of serious imaging artifacts. The flowchart of this study is shown in Figure 1, and detailed descriptions of the therapeutic regimens are provided in the Appendix 1. Patients were divided into chemotherapy group and chemoradiotherapy group to facilitate analysis of the differences in CCTA parameters between the two treatment modalities.
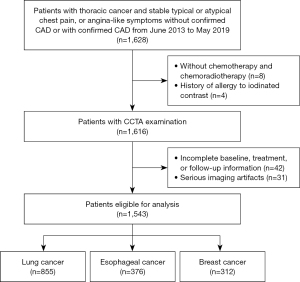
CCTA acquisition
All patients underwent CCTA examinations, which were performed on a Brilliance 64 CT scanner (Philips, Amsterdam, the Netherlands). The CCTA protocol schemes included initial nonenhanced calcium-scoring acquisition, which was followed by an arterially enhanced angiography. The scanning parameters were as follows: tube voltage, 120 kV; detector collimation, 40 mm; beam pitch, 1.5; slice thickness, 1.0 mm; ad slice gap, 2.0 mm. Electrocardiogram (ECG)-triggered acquisitions were continuously performed for the cardiac scans.
CCTA data analysis
Commercial artificial intelligence software, CoronaryDoc version 5.1.2 (ShuKun Technology, Beijing, China), run on a regular workstation, was used to segment and assess all CCTA images. The CCTA data were calculated with a deep learning algorithm. The CAC score and plaque calculation modules of the software used in this study have obtained the third-class medical device registration certification from the China National Medical Products Administration and the Conformité Européenne certification under the new Medical Device Regulation of the European Union. The CCTA data analyzed by the software were validated in 18 hospitals and comprise more than 10,000 cases and are thus reliable.
The CAC scores were automatically calculated by the software via nonenhanced calcium-scoring scanning (CACScoreDoc, ShuKun Technology), wherein each calcified area was multiplied by a local density factor as determined by the Hounsfield unit (HU) of the calcium (0, 0–129 HU; 1, 130–199 HU; 2, 200–299 HU, 3, 300–399 HU; 4, ≥400 HU) (Figure 2). The following plaque types were considered: no plaque, noncalcified plaque, calcified plaque, and mixed plaque. The CAD-RADS was used to classify the severity of stenosis from 0 (absence of CAD) to 5 (total coronary occlusion or subtotal occlusion) by two radiologists with 5 and 10 years of experience in cardiac CT imaging interpretation, respectively. The detailed information of this classification is presented in Table S1. Per-vessel and per-patient CAD-RADS categories were assigned based on the highest-grade stenosis. Analysis of left ventricle function was performed via CoronaryDoc Plus (ShuKun Technology), with ECG-triggered CCTA being used for automatically calculating the left ventricular ejection fraction (LVEF), left ventricular end-diastolic volume (LVEDV), left ventricular end-systolic volume (ESV), left ventricular stroke volume (LVSV), and left ventricular myocardial mass (LVMM).
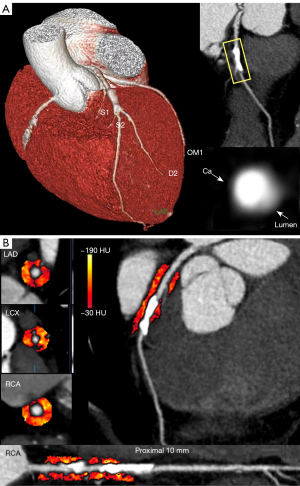
Dedicated research analysis software, Coronary FAI Analysis version 1.0.2, ShuKun Technology) was employed for FAI segmentation. For the right coronary artery (RCA), the perivascular FAI was measured according to the proximal 10- to 50-mm segments, while for the left anterior descending artery (LAD) and left circumflex artery (LCX), the proximal 40 mm of each vessel was analyzed (14). The detailed process was as follows. First, a well-trained deep learning model was used to segment the coronary arteries. Second, a skeleton erosion shrinkage algorithm was used to calculate the centerline of each branch. Third, the normal sections of the local blood vessels were cut at equal intervals and were stacked in sequence to reconstruct a three-dimensional straightened blood vessel image, with a sliding window being used to select the starting and ending positions of the FAI along the vessel. Briefly, pericoronary adipose tissue was sampled radially outward from the outer vessel wall of the plaques and measured as voxels with attenuation between −190 and −30 HU (12) (Figure 2).
Definition of MACEs
Twenty-four months of follow-up were conducted after completion of chemotherapy or chemoradiotherapy. Follow-up was performed through a review of medical records and telephone interviews by two radiologists at 3-month intervals until February 2022. MACEs were defined as cardiovascular death, myocardial infarction, stroke, complete heart block, and rehospitalization due to heart failure or aggravated angina symptoms (15).
Statistical analysis
Statistical analysis was performed using SPSS version 25 (IBM Corp., Armonk, NY, USA) and Stata Version 16 (StataCorp, College station, TX, USA). The one-sample Kolmogorov-Smirnov test was used to check the assumption of normal distribution. Normally distributed continuous variables were expressed as the mean ± standard deviation, while nonnormally distributed continuous variables were expressed as medians and quartiles. Categorical variables were reported as counts and percentages. The independent t-test and the Wilcoxon signed-rank test were used to compare normally and nonnormally distributed continuous variables, respectively. The Chi-squared test was used to compare categorical variables. The intraobserver and interobserver consistency of CCTA parameters were assessed by two certified radiologists (with 15 and 10 years of experience, respectively). The intraclass correlation coefficient (ICC) was used to assess continuous variables, while the kappa coefficient was used to evaluate categorical variables. Test-retest reliability was determined according to the following scheme: ICC 0.75 or greater, excellent; between 0.6 and 0.74, good; between 0.41 and 0.59, fair; and less than 0.4, poor (16).
Univariable and multivariable Cox proportional hazards models were used to determine the CCTA parameters associated with MACEs. Baseline variables that considered traditional cardiovascular risk factors, including age, sex, obesity (BMI ≥25 kg/m2), hypertension, diabetes, dyslipidemia, smoking, previous myocardial infarction, known CAD, previous heart failure, or those with a univariate relationship with MACEs (P value <0.1) were entered into multivariate Cox proportional-hazards regression analysis. Kaplan-Meier curves were drawn, and the log-rank test was used to compare the MACE-free survival between different subgroups. Statistical significance was defined as a two-tailed P value <0.05.
Results
Study population and clinical outcomes
Of the 1,628 patients with thoracic malignancy, 85 ineligible patients were excluded (8 patients without chemotherapy or chemoradiotherapy; 4 patients with a history of allergy to CT contrast agents; 42 patients without complete baseline, treatment, and/or follow-up information; and 31 patients with serious imaging artifacts). Finally, 1,543 patients with thoracic malignancy (855 patients with lung cancer, 376 patients with esophageal cancer, and 312 patients with breast cancer) were enrolled, including 808 patients receiving chemotherapy and 735 patients receiving chemoradiotherapy. All patients underwent two CCTA examinations within 1 year, with the median interval between baseline and follow-up CCTA in the chemotherapy group being 362 days [interquartile range (IQR) 352–374 days] and that in the chemoradiotherapy group being 361 days (IQR 350–375 days). Among the patients, 638 (41.3%) were male, and the median age was 67.00 years (IQR 56.00–72.00 years). The clinical characteristics of all patients are summarized by treatment type in Table 1 and Table S2.
Table 1
Parameter | Chemotherapy | Chemoradiotherapy | |||||
---|---|---|---|---|---|---|---|
Pretreatment (n=445) | Posttreatment (n=363) | P value | Pretreatment (n=428) | Posttreatment (n=307) | P value | ||
Age (years) | 67.00 (58.00, 74.00) | 67.00 (58.00, 70.00) | 0.124 | 66.00 (56.00, 72.00) | 65.50 (55.00, 72.00) | 0.882 | |
Sex | 0.136 | 0.227 | |||||
Female | 253 (56.9) | 233 (64.2) | 236 (55.1) | 183 (59.6) | |||
Male | 192 (43.1) | 130 (35.8) | 192 (44.9) | 124 (40.4) | |||
Body mass index (kg/m2) | 23.45±3.36 | 23.37±3.38 | 0.761 | 23.41±3.45 | 22.81±5.05 | 0.056 | |
Obesity | 31 (7.0) | 23 (6.3) | 0.721 | 31 (7.2) | 19 (6.2) | 0.259 | |
Hypertension | 138 (30.3) | 103 (27.6) | 0.403 | 145 (32.4) | 98 (30.4) | 0.555 | |
Diabetes mellitus | 48 (10.5) | 51 (13.7) | 0.165 | 63 (14.1) | 39 (12.1) | 0.424 | |
Smoking | 335 (73.5) | 253 (67.8) | 0.075 | 333 (74.5) | 220 (68.3) | 0.06 | |
Dyslipidemia | 274 (60.1) | 206 (55.2) | 0.159 | 259 (57.9) | 199 (61.8) | 0.282 | |
Previous MI | 12 (2.7) | 16 (4.4) | 0.186 | 14 (3.3) | 12 (3.9) | 0.644 | |
Known CAD | 188 (42.2) | 154 (42.4) | 0.960 | 177 (41.4) | 127 (41.4) | 0.997 | |
Previous HF | 14 (3.9) | 10 (2.2) | 0.180 | 15 (3.5) | 11 (3.6) | 0.955 | |
TNM | 0.990 | 0.077 | |||||
I | 47 (10.6) | 41 (11.3) | 36 (8.4) | 20 (6.5) | |||
II | 135 (30.3) | 109 (30.0) | 130 (30.4) | 72 (23.5) | |||
III | 213 (47.9) | 172 (47.4) | 221 (51.6) | 175 (57.0) | |||
IV | 50 (11.2) | 41 (11.3) | 41 (9.6) | 40 (13.0) | |||
Medication use | |||||||
Beta-blocker | 29 (6.5) | 29 (8.0) | 0.420 | 30 (7.0) | 22 (7.2) | 0.925 | |
Ca2+ channel blocker | 34 (7.6) | 28 (7.7) | 0.969 | 32 (7.5) | 19 (6.2) | 0.506 | |
ACEI/ARB | 31 (7.0) | 26 (7.2) | 0.914 | 34 (7.9) | 21 (6.9) | 0.583 | |
Statin | 84 (18.9) | 67 (18.5) | 0.879 | 85 (19.9) | 56 (18.3) | 0.597 | |
Aspirin | 33 (7.4) | 25 (6.9) | 0.772 | 34 (7.9) | 22 (7.2) | 0.704 | |
Mean heart dose (Gy) | – | – | – | 14.25±6.65 |
Values are expressed as the mean ± standard deviation, median (interquartile range), or n (%). MI, myocardial infarction; CAD, coronary artery disease; HF, heart failure; TNM, tumor-node-metastasis; ACEI/ARB, angiotensin-converting enzyme inhibitors/angiotensin receptor blocker.
The median follow-up duration was 26 months (IQR 24–33 months). MACEs occurred in 232 patients (15.0%), which included 87 cases of cardiovascular death, 40 cases of complete heart block, 33 strokes, 30 cases of rehospitalization due to heart failure or aggravated angina symptoms, 27 cases of acute myocardial infarction, 15 cardiogenic shocks, and 9 cardiac arrests. Higher FAI values for the LAD, LCX, and RCA were observed in patients with MACEs compared to those without MACEs. There were no significant differences in other baseline characteristics between the two groups. The details are presented in Table S3.
Intraobserver and interobserver consistency
The intra-observer and inter-observer consistency of FAI and left ventricle function data were excellent, with an ICC value of 0.967 [95% confidence interval (CI): 0.933–0.986] and 0.921 (95% CI: 0.880–0.962), respectively. Excellent intra-observer and inter-observer consistency was also observed for the total CAC score, coronary stenosis, and CAD-RADS classification, with the kappa coefficient ranging from 0.852 to 0.931.
CCTA-derived parameters in the chemotherapy group
There were no statistically significant differences in CAC score, plaque characteristics, diameter stenosis (DS), or CAD-RADS categories between the three coronary arteries before and after chemotherapy (all P values >0.05; Table 2, Table S4). A statistically significant increase in the FAI values of the LAD, LCX, and RCA was observed after treatment (−73.2±6.74, −73.2±8.10, and −73.4±7.40 HU, respectively) compared with those before treatment (−76.6±6.03, −76.3±6.47, and −76.5±5.98 HU, respectively) (all P values <0.001; Table 2). Additionally, in each enrolled thoracic malignancy, there was a statistically significant increase in FAI values of the LAD, LCX, and RCA after chemotherapy (all P values <0.05), as shown in Table S5.
Table 2
Parameters | Chemotherapy | Chemoradiotherapy | |||||
---|---|---|---|---|---|---|---|
Pretreatment (n=445) | Posttreatment (n=363) | P value | Pretreatment (n=428) | Posttreatment (n=307) | P value | ||
Total coronary artery calcium score | 0.171 | 0.324 | |||||
0 (0) | 124 (27.9) | 103 (28.4) | 158 (36.9) | 108 (35.2) | |||
1 (1 to 10) | 55 (12.4) | 42 (11.6) | 43 (10.0) | 20 (6.5) | |||
2 (11 to 100) | 64 (14.3) | 75 (20.7) | 74 (17.3) | 55 (17.9) | |||
3 (101 to 400) | 88 (19.8) | 63 (17.3) | 73 (17.1) | 66 (21.5) | |||
4 (>400) | 114 (25.6) | 80 (22.0) | 80 (18.7) | 58 (18.9) | |||
Total coronary diameter stenosis | 0.656 | 0.772 | |||||
None (0%) | 107 (24.0) | 75 (20.7) | 103 (24.1) | 82 (26.7) | |||
Slight (1% to 24%) | 43 (9.7) | 33 (9.0) | 33 (7.7) | 19 (6.2) | |||
Mild (25% to 49%) | 84 (18.9) | 82 (22.6) | 78 (18.2) | 52 (16.9) | |||
Moderate (50% to 69%) | 107 (24.0) | 87 (24.0) | 100 (23.4) | 78 (25.4) | |||
Severe (70% to 99%) | 104 (23.4) | 86 (23.7) | 114 (26.6) | 76 (24.8) | |||
CAD-RADS classification | 0.932 | 0.907 | |||||
0 | 106 (23.8) | 79 (21.8) | 106 (24.8) | 84 (27.4) | |||
1 | 43 (9.7) | 34 (9.4) | 30 (7.0) | 19 (6.2) | |||
2 | 86 (19.3) | 78 (21.5) | 78 (18.2) | 52 (16.8) | |||
3 | 104 (23.4) | 85 (23.3) | 102 (23.8) | 76 (24.8) | |||
4 | 106 (23.8) | 87 (24.0) | 112 (26.2) | 76 (24.8) | |||
5 | 0 (0.0) | 0 (0.0) | 0 (0.0) | 0 (0.0) | |||
LVEF (%) | 54.7±6.1 | 53.9±7.5 | 0.095 | 55.0±5.4 | 54.2±5.6 | 0.057 | |
LVEDV (mL) | 118.1±14.8 | 117.0±13.4 | 0.273 | 120.0±15.8 | 118.4±15.4 | 0.172 | |
LVESV (mL) | 52.7±9.8 | 53.3±8.3 | 0.354 | 52.5±8.4 | 53.0±9.3 | 0.447 | |
LVSV (mL) | 63.9±11.3 | 63.7±12.9 | 0.814 | 67.4±12.1 | 65.8±13.9 | 0.098 | |
LVMM (g) | 99.2±15.9 | 98.2±10.4 | 0.303 | 95.3±12.5 | 95.9±15.7 | 0.565 | |
FAI (HU) | |||||||
LAD | −76.6±6.03 | −73.2±6.74 | <0.001 | −76.6±6.31 | −70.3±6.48 | <0.001 | |
LCX | −76.3±6.47 | −73.2±8.10 | <0.001 | −76.7±7.25 | −70.4±4.66 | <0.001 | |
RCA | −76.5±5.98 | −73.4±7.40 | <0.001 | −76.3±6.52 | −70.6±6.19 | <0.001 |
Values are expressed as mean ± standard deviation or as n (%). CCTA, coronary computed tomography angiography; CAD-RADS, Coronary Artery Disease-Reporting and Data System; LVEF, left ventricular ejection fraction; LVEDV, left ventricular end-diastolic volume; LVESV, left ventricular end-systolic volume; LVSV, left ventricular stroke volume; LVMM, left ventricular myocardial mass; FAI, fat attenuation index; HU, Hounsfield unit; LAD, left anterior descending artery; LCX, left circumflex artery; RCA, right coronary artery.
CCTA-derived parameters in the chemoradiotherapy group
In the chemoradiotherapy group, no statistically significant differences were observed in CAC score, plaque characteristics, DS, or CAD-RADS categories of the three coronary arteries before and after chemoradiotherapy (all P values >0.05; Table 2, Table S4).
There were higher FAI values in the three coronary arteries observed after chemotherapy treatment than before treatment (all P values <0.001; Table 2). The FAI values of the LAD, LCX, and RCA after chemoradiotherapy were significantly increased in each enrolled thoracic malignancy (all P values <0.05), as presented in Table S6.
Comparison of CCTA-derived parameters between the chemotherapy and chemoradiotherapy groups
The CCTA parameters of the chemotherapy group and the chemoradiotherapy group before and after treatment are summarized in Table S7.
Before treatment, none of the parameters showed any differences between the two treatment groups. Moreover, after treatment, no statistically significant differences were observed in total CAC score, plaque characteristics, DS, CAD-RADS categories, or left ventricle function data (all P values >0.05), but the FAI values of the three coronary arteries were significantly higher in the chemoradiotherapy group than in the chemotherapy group (all P values <0.001), as shown in Figure 3. The pretreatment and posttreatment CCTA parameters for each enrolled thoracic malignancy in the two treatment groups are presented in Tables S8-S10.
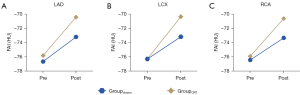
Association between FAI and MACEs
MACEs were associated with FAI values of the LAD, LCX, and RCA before chemotherapy [LAD: hazard ratio (HR) =3.745, 95% CI: 1.193–11.756, P=0.023; LCX: HR =3.460, 95% CI: 1.092–10.832, P=0.031; RCA: HR =4.175, 95% CI: 1.375–12.673, P=0.011] and chemoradiotherapy (LAD: HR =2.856, 95% CI: 1.210–6.742, P=0.016; LCX: HR =2.385, 95% CI: 1.037–5.487, P=0.040; RCA: HR =2.029, 95% CI: 1.074–3.834, P=0.029), as shown in Tables 3,4.
Table 3
Characteristic | Chemotherapy group | ||||
---|---|---|---|---|---|
Univariable analysis | Multivariable analysis | ||||
HR (95% CI) | P | HR (95% CI) | P | ||
Age | 0.980 (0.939–1.022) | 0.352 | 0.989 (0.937–1.045) | 0.716 | |
Male sex | 1.251 (0.541–2.891) | 0.599 | 1.742 (0.685–4.431) | 0.243 | |
Obesity | 3.659 (1.357–9.870) | 0.010 | 2.586 (0.708–9.445) | 0.150 | |
Hypertension | 0.897 (0.369–2.181) | 0.810 | 0.433 (0.139–1.343) | 0.147 | |
Diabetes | 0.391 (0.053–2.899) | 0.358 | 0.457 (0.059–3.558) | 0.454 | |
Dyslipidemia | 1.363 (0.960–1.822) | 0.115 | 0.643 (0.234–1.764) | 0.391 | |
Smoking | 0.489 (0.181–1.317) | 0.157 | 0.474 (0.138–1.629) | 0.236 | |
TNM | 1.076 (0.623–1.858) | 0.792 | – | – | |
Previous MI | 2.536 (0.341–18.887) | 0.364 | 1.347 (0.886–3.801) | 0.467 | |
Known CAD | 2.587 (0.097–6.104) | 0.129 | 0.844 (0.414–1.709) | 0.405 | |
Previous HF | 3.021 (0.406–22.452) | 0.280 | 1.014 (0.582–1.764) | 0.315 | |
Total stenosis | 0.903 (0.693–1.179) | 0.456 | – | – | |
Total calcium score | 1.115 (0.847–1.469) | 0.436 | – | – | |
CAD-RADS classification | 0.884 (0.677–1.153) | 0.362 | – | – | |
Medication use | |||||
Beta-blocker | 0.713 (0.096–5.292) | 0.741 | – | – | |
Ca2+ channel blocker | 0.942 (0.221–4.019) | 0.935 | – | – | |
ACEI/ARB | 0.871 (0.296–2.560) | 0.802 | – | – | |
Statin | 0.879 (0.299–2.584) | 0.815 | – | – | |
Aspirin | 0.742 (0.174–3.169) | 0.688 | – | – | |
LVEF (%) | 1.003 (0.968–1.040) | 0.844 | – | – | |
LVEDV (mL) | 1.011 (0.989–1.034) | 0.309 | – | – | |
LVESV (mL) | 1.012 (0.979–1.046) | 0.485 | – | – | |
LVSV (mL) | 1.004 (0.985–1.024) | 0.678 | – | – | |
LVMM (g) | 1.005 (0.992–1.019) | 0.462 | – | – | |
FAI of LAD (HU) | 10.324 (4.544–23.453) | <0.001 | 3.745 (1.193–11.756) | 0.023 | |
FAI of LCX (HU) | 10.177 (4.483–23.100) | <0.001 | 3.460 (1.092–10.832) | 0.031 | |
FAI of RCA (HU) | 11.099 (4.856–25.373) | <0.001 | 4.175 (1.375–12.673) | 0.011 |
MACE, major adverse cardiovascular event; HR, hazard ratio; CI, confidence interval; TNM, tumor-node-metastasis; MI, myocardial infarction; CAD, coronary artery disease; HF, heart failure; CAD-RADS, Coronary Artery Disease-Reporting and Data System; ACEI/ARB, angiotensin-converting enzyme inhibitors/angiotensin receptor blocker; LVEF, left ventricular ejection fraction; LVEDV, left ventricular end-diastolic volume; LVESV, left ventricular end-systolic volume; LVSV, left ventricular stroke volume; LVMM, left ventricular myocardial mass; FAI, fat attenuation index; LAD, left anterior descending artery; HU, Hounsfield unit; LCX, left circumflex artery; RCA, right coronary artery.
Table 4
Characteristic | Chemoradiotherapy group | ||||
---|---|---|---|---|---|
Univariable analysis | Multivariable analysis | ||||
HR (95% CI) | P | HR (95% CI) | P | ||
Age | 0.992 (0.971–1.014) | 0.471 | 0.990 (0.969–1.013) | 0.415 | |
Male sex | 0.816 (0.503–1.324) | 0.410 | 0.983 (0.570–1.698) | 0.953 | |
Obesity | 1.593 (0.760–3.336) | 0.217 | 1.752 (0.805–3.816) | 0.157 | |
Hypertension | 1.547 (0.947–2.528) | 0.081 | 1.276 (0.767–2.125) | 0.347 | |
Diabetes | 1.280 (0.669–2.447) | 0.454 | 1.059 (0.536–2.092) | 0.867 | |
Dyslipidemia | 1.727 (0.928–1.901) | 0.138 | 1.896 (0.979–3.674) | 0.257 | |
Smoking | 1.045 (0.607–1.800) | 0.873 | 0.818 (0.382–1.751) | 0.604 | |
TNM | 1.014 (0.786–1.308) | 0.911 | – | – | |
Previous MI | 0.932 (0.228–3.807) | 0.921 | 1.288 (0.304–5.447) | 0.730 | |
Known CAD | 0.706 (0.423–1.178) | 0.183 | 0.913 (0.698–1.542) | 0.541 | |
Previous HF | 0.413 (0.057–2.980) | 0.380 | 0.801 (0.670–1.741) | 0.499 | |
Total stenosis | 1.092 (0.928–1.286) | 0.285 | – | – | |
Total calcium score | 0.958 (0.818–1.123) | 0.600 | – | – | |
CAD-RADS classification | 1.132 (0.960–1.335) | 0.137 | – | – | |
Medication use | |||||
Beta-blocker | 1.074 (0.431–2.674) | 0.878 | – | – | |
Ca2+ channel blocker | 1.555 (0.770–3.142) | 0.218 | – | – | |
ACEI/ARB | 0.585 (0.298–1.146) | 0.118 | – | – | |
Statin | 0.694 (0.354–1.359) | 0.287 | – | – | |
Aspirin | 0.611 (0.245–1.521) | 0.289 | – | – | |
LVEF (%) | 1.005 (0.985–1.026) | 0.576 | – | – | |
LVEDV (mL) | 1.006 (0.993–1.018) | 0.327 | – | – | |
LVESV (mL) | 1.004 (0.981–1.027) | 0.707 | – | – | |
LVSV (mL) | 1.003 (0.992–1.014) | 0.514 | – | – | |
LVMM (g) | 1.010 (0.974–1.023) | 0.282 | – | – | |
FAI of LAD (HU) | 9.055 (5.548–14.780) | <0.001 | 2.856 (1.210–6.742) | 0.016 | |
FAI of LCX (HU) | 6.966 (4.276–11.350) | <0.001 | 2.385 (1.037–5.487) | 0.040 | |
FAI of RCA (HU) | 5.693 (3.467–9.348) | <0.001 | 2.029 (1.074–3.834) | 0.029 |
HR, hazard ratio; CI, confidence interval; MACE, major adverse cardiovascular event; TNM, tumor-node-metastasis; MI, myocardial infarction; CAD, coronary artery disease; HF, heart failure; CAD-RADS, Coronary Artery Disease-Reporting and Data System; ACEI/ARB, angiotensin-converting enzyme inhibitors/angiotensin receptor blocker; LVEF, left ventricular ejection fraction; LVEDV, left ventricular end-diastolic volume; LVESV, left ventricular end-systolic volume; LVSV, left ventricular stroke volume; LVMM, left ventricular myocardial mass; FAI, fat attenuation index; LAD, left anterior descending artery; HU, Hounsfield unit; LCX, left circumflex artery; RCA, right coronary artery.
According to a previous study (12), FAI values were divided into high- (≥−70.1 HU) or low-value (<−70.1 HU) groups. The MACE-free survival rates in patients with high FAI values were lower than those in patients with low FAI values both before chemotherapy and chemoradiotherapy, as presented in Figure 4. The Kaplan-Meier curves of each thoracic malignancy are shown in Figures S1-S9.
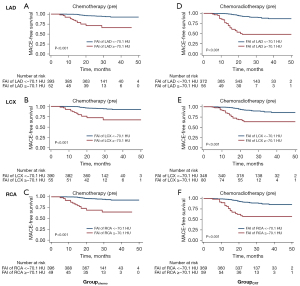
Discussion
This study focused on CCTA parameters in thoracic malignancies. The following observations were made. (I) The FAI increased after chemotherapy or chemoradiotherapy. (II) After treatment, the FAI was significantly higher in the chemoradiotherapy group than in the chemotherapy group. (III) The FAI before chemotherapy or chemoradiotherapy was associated with MACEs.
Chemotherapy and chemoradiotherapy, which are associated with damage to coronary arteries, have toxic effects on coronary endothelial cell function and viability, eventually resulting in plaque formation, coronary artery stenosis, hemodynamic changes, and coronary inflammation, thereby increasing the risk of CAD (17,18). In our study, FAI was increased after chemotherapy or chemoradiotherapy in patients with thoracic malignancies. A recent study found that FAI decrease is associated with moderate-to-severe psoriasis in patients receiving biologic therapy (13). In other research, it was found that FAI decreased after statin treatment in patients with noncalcified and mixed plaques (19). These findings suggest that the dynamic alterations of FAI can be observed not only in the treatment of patients with cancer but also in those without cancer. Therefore, CCTA allows for evaluating the longitudinal changes of coronary arteries in patients with thoracic malignancies and is beneficial to clinical treatment decision-making (20).
In this study, the FAI was higher in the chemoradiotherapy group than in the chemotherapy group after treatment. This result indicates that chemoradiotherapy may be considerably more deleterious to the coronary arteries compared to chemotherapy. Radiation can cause endothelial cellular stress, including generation of reactive oxygen species, DNA damage, and subcellular injury, as well as accelerated atherosclerosis, necrosis, and fibrosis of the media and adventitia. This eventually leads to irreversible structural and functional injury in the coronary artery (21,22). Chemotherapy, as a systemic treatment, transports drugs through veins into the coronary arteries, which may damage the endothelial cells in these arteries and cause cellular dysfunction. However, chemotherapeutics does not have a significant influence on the coronary arterial wall until the cumulative dose is reached (18,23). Moreover, chemoradiotherapy, the combination of chemotherapy and radiotherapy, may result in cumulative toxicity in the coronary arteries. A recent study reported that the risk of toxicity to the coronary arteries is higher in patients with breast cancer who receive both radiation therapy and chemotherapy (24).
Our results confirmed that the higher FAI values in the LAD, LCX, and RCA were associated with MACEs in patients with thoracic malignancies. Several studies indicate that increased FAI is a strong independent predictor of MACEs in patients without cancer (12,14,25). FAI is considered to be a novel method for assessing coronary inflammation (26). Pericoronary inflammation is regarded as an early indicator of CAD and may aid in predicting MACEs (12,27). Pericoronary inflammation may induce local fat breakdown and prevent lipid accumulation, leading to increased FAI values (28). Consequently, the FAI may be able to predict the occurrence of MACEs.
Certain limitations to this study should be considered. First, we employed a retrospective, single-center design, which might have introduced selection bias and constrained the generalizability of the findings. Second, the comparison of CCTA parameters before and after treatment was not based on the same group of patients, which could have introduced variability and confounding factors. Third, more relevant myocardial parameters were not taken into account for a more comprehensive assessment of cardiovascular health. In the future, a prospective, multicenter study will be conducted to further validate these findings.
Conclusions
We found that FAI derived from CCTA, as an imaging biomarker of coronary arterial inflammation, could identify coronary impairment and was associated with MACEs in patients with thoracic malignancies receiving chemotherapy or chemoradiotherapy.
Acknowledgments
Funding: This study received funding from
Footnote
Reporting Checklist: The authors have completed the STROBE reporting checklists. Available at https://qims.amegroups.com/article/view/10.21037/qims-24-944/rc
Conflicts of Interest: All authors have completed the ICMJE uniform disclosure form (available at https://qims.amegroups.com/article/view/10.21037/qims-24-944/coif). The authors have no conflicts of interest to declare.
Ethical Statement: The authors are accountable for all aspects of the work in ensuring that questions related to the accuracy or integrity of any part of the work are appropriately investigated and resolved. This study was conducted in accordance with the Declaration of Helsinki (as revised in 2013) and was approved by the institutional ethics committee of Chongqing University Cancer Hospital (No. CZLS2023017-A). Individual consent for this analysis was waived due to the retrospective nature of the analysis.
Open Access Statement: This is an Open Access article distributed in accordance with the Creative Commons Attribution-NonCommercial-NoDerivs 4.0 International License (CC BY-NC-ND 4.0), which permits the non-commercial replication and distribution of the article with the strict proviso that no changes or edits are made and the original work is properly cited (including links to both the formal publication through the relevant DOI and the license). See: https://creativecommons.org/licenses/by-nc-nd/4.0/.
References
- Miller KD, Nogueira L, Devasia T, Mariotto AB, Yabroff KR, Jemal A, Kramer J, Siegel RL. Cancer treatment and survivorship statistics, 2022. CA Cancer J Clin 2022;72:409-36. [Crossref] [PubMed]
- Thai AA, Solomon BJ, Sequist LV, Gainor JF, Heist RS. Lung cancer. Lancet 2021;398:535-54. [Crossref] [PubMed]
- Huang TX, Fu L. The immune landscape of esophageal cancer. Cancer Commun (Lond) 2019;39:79. [Crossref] [PubMed]
- Waks AG, Winer EP. Breast Cancer Treatment. JAMA 2019;321:316. [Crossref] [PubMed]
- Yakupovich A, Davison MA, Kharouta MZ, Turian J, Seder CW, Batus M, Fogg LF, Kalra D, Kosinski M, Taskesen T, Okwuosa TM. Heart dose and coronary artery calcification in patients receiving thoracic irradiation for lung cancer. J Thorac Dis 2020;12:223-31. [Crossref] [PubMed]
- Atkins KM, Rawal B, Chaunzwa TL, Lamba N, Bitterman DS, Williams CL, Kozono DE, Baldini EH, Chen AB, Nguyen PL, D'Amico AV, Nohria A, Hoffmann U, Aerts HJWL, Mak RH. Cardiac Radiation Dose, Cardiac Disease, and Mortality in Patients With Lung Cancer. J Am Coll Cardiol 2019;73:2976-87. [Crossref] [PubMed]
- Lyon AR, López-Fernández T, Couch LS, Asteggiano R, Aznar MC, Bergler-Klein J, et al. 2022 ESC Guidelines on cardio-oncology developed in collaboration with the European Hematology Association (EHA), the European Society for Therapeutic Radiology and Oncology (ESTRO) and the International Cardio-Oncology Society (IC-OS). Eur Heart J 2022;43:4229-361. [Crossref] [PubMed]
- Chen Q, Pan T, Wang YN, Schoepf UJ, Bidwell SL, Qiao H, Feng Y, Xu C, Xu H, Xie G, Gao X, Tao XW, Lu M, Xu PP, Zhong J, Wei Y, Yin X, Zhang J, Zhang LJ. A Coronary CT Angiography Radiomics Model to Identify Vulnerable Plaque and Predict Cardiovascular Events. Radiology 2023;307:e221693. [Crossref] [PubMed]
- Greco C, Kolesnick R, Fuks Z. Conformal Avoidance of Normal Organs at Risk by Perfusion-Modulated Dose Sculpting in Tumor Single-Dose Radiation Therapy. Int J Radiat Oncol Biol Phys 2021;109:288-97. [Crossref] [PubMed]
- Gal R, van Velzen SGM, Hooning MJ, Emaus MJ, van der Leij F, Gregorowitsch ML, et al. Identification of Risk of Cardiovascular Disease by Automatic Quantification of Coronary Artery Calcifications on Radiotherapy Planning CT Scans in Patients With Breast Cancer. JAMA Oncol 2021;7:1024-32. [Crossref] [PubMed]
- Lee JW, Kim JY, Han K, Im DJ, Lee KH, Kim TH, Park CH, Hur J, Coronary CT. Angiography CAD-RADS versus Coronary Artery Calcium Score in Patients with Acute Chest Pain. Radiology 2021;301:81-90. [Crossref] [PubMed]
- Oikonomou EK, Marwan M, Desai MY, Mancio J, Alashi A, Hutt Centeno E, et al. Non-invasive detection of coronary inflammation using computed tomography and prediction of residual cardiovascular risk (the CRISP CT study): a post-hoc analysis of prospective outcome data. Lancet 2018;392:929-39. [Crossref] [PubMed]
- Elnabawi YA, Oikonomou EK, Dey AK, Mancio J, Rodante JA, Aksentijevich M, Choi H, Keel A, Erb-Alvarez J, Teague HL, Joshi AA, Playford MP, Lockshin B, Choi AD, Gelfand JM, Chen MY, Bluemke DA, Shirodaria C, Antoniades C, Mehta NN. Association of Biologic Therapy With Coronary Inflammation in Patients With Psoriasis as Assessed by Perivascular Fat Attenuation Index. JAMA Cardiol 2019;4:885-91. [Crossref] [PubMed]
- Antonopoulos AS, Sanna F, Sabharwal N, Thomas S, Oikonomou EK, Herdman L, et al. Detecting human coronary inflammation by imaging perivascular fat. Sci Transl Med 2017;9:eaal2658. [Crossref] [PubMed]
- Lan Z, Ding X, Yu Y, Yu L, Yang W, Dai X, Ling R, Wang Y, Yang W, Zhang J. CT-derived fractional flow reserve for prediction of major adverse cardiovascular events in diabetic patients. Cardiovasc Diabetol 2023;22:65. [Crossref] [PubMed]
- Hessam S, Scholl L, Sand M, Schmitz L, Reitenbach S, Bechara FG. A Novel Severity Assessment Scoring System for Hidradenitis Suppurativa. JAMA Dermatol 2018;154:330-5. [Crossref] [PubMed]
- Xu S, Ilyas I, Little PJ, Li H, Kamato D, Zheng X, Luo S, Li Z, Liu P, Han J, Harding IC, Ebong EE, Cameron SJ, Stewart AG, Weng J. Endothelial Dysfunction in Atherosclerotic Cardiovascular Diseases and Beyond: From Mechanism to Pharmacotherapies. Pharmacol Rev 2021;73:924-67. [Crossref] [PubMed]
- Herrmann J. Vascular toxic effects of cancer therapies. Nat Rev Cardiol 2020;17:503-22. [Crossref] [PubMed]
- Dai X, Yu L, Lu Z, Shen C, Tao X, Zhang J. Serial change of perivascular fat attenuation index after statin treatment: Insights from a coronary CT angiography follow-up study. Int J Cardiol 2020;319:144-9. [Crossref] [PubMed]
- Peper J, Becker LM, van den Berg H, Bor WL, Brouwer J, Nijenhuis VJ, van Ginkel DJ, Rensing BJMW, Ten Berg JM, Timmers L, Leiner T, Swaans MJ. Diagnostic Performance of CCTA and CT-FFR for the Detection of CAD in TAVR Work-Up. JACC Cardiovasc Interv 2022;15:1140-9. [Crossref] [PubMed]
- Kim W, Lee S, Seo D, Kim D, Kim K, Kim E, Kang J, Seong KM, Youn H, Youn B. Cellular Stress Responses in Radiotherapy. Cells 2019;18:1105. [Crossref] [PubMed]
- van den Bogaard VA, Ta BD, van der Schaaf A, Bouma AB, Middag AM, Bantema-Joppe EJ, van Dijk LV, van Dijk-Peters FB, Marteijn LA, de Bock GH, Burgerhof JG, Gietema JA, Langendijk JA, Maduro JH, Crijns AP. Validation and Modification of a Prediction Model for Acute Cardiac Events in Patients With Breast Cancer Treated With Radiotherapy Based on Three-Dimensional Dose Distributions to Cardiac Substructures. J Clin Oncol 2017;35:1171-8. [Crossref] [PubMed]
- Galán-Arriola C, Vílchez-Tschischke JP, Lobo M, López GJ, de Molina-Iracheta A, Pérez-Martínez C, Villena-Gutiérrez R, Macías Á, Díaz-Rengifo IA, Oliver E, Fuster V, Sánchez-González J, Ibanez B. Coronary microcirculation damage in anthracycline cardiotoxicity. Cardiovasc Res 2022;118:531-41. [Crossref] [PubMed]
- Tu C, Shen H, Li X, Wang X, Miao Z, Deng W, Liu R, Lan X, Chen H, Zhang J. Longitudinal Evaluation of Coronary Arteries and Myocardium in Breast Cancer Using Coronary Computed Tomographic Angiography. JACC Cardiovasc Imaging 2024; Epub ahead of print. [Crossref]
- Sagris M, Antonopoulos AS, Simantiris S, Oikonomou E, Siasos G, Tsioufis K, Tousoulis D. Pericoronary fat attenuation index-a new imaging biomarker and its diagnostic and prognostic utility: a systematic review and meta-analysis. Eur Heart J Cardiovasc Imaging 2022;23:e526-36. [Crossref] [PubMed]
- Antoniades C, Antonopoulos AS, Deanfield J. Imaging residual inflammatory cardiovascular risk. Eur Heart J 2020;41:748-58. [Crossref] [PubMed]
- Hansson GK. Inflammation, atherosclerosis, and coronary artery disease. N Engl J Med 2005;352:1685-95. [Crossref] [PubMed]
- Grant RW, Stephens JM. Fat in flames: influence of cytokines and pattern recognition receptors on adipocyte lipolysis. Am J Physiol Endocrinol Metab 2015;309:E205-13. [Crossref] [PubMed]