Prenatal diagnosis of achondroplasia and hypochondroplasia using three-dimensional computed tomography: a case series at a single institution
Introduction
In most skeletal dysplasia cases, a single gene carries the mutation. Per the Nosology of Genetic Skeletal Disorders: 2023 edition, 771 genetic skeletal disorders are categorized into 41 groups (1,2). Fetal skeletal dysplasia (FSD) refers to skeletal dysplasia in which symptoms develop during the prenatal period. FSD diagnosed in the prenatal stage is detected using fetal ultrasonography (3). Despite the increased usage of fetal ultrasound imaging, accurate diagnosis and classification of FSD remain challenging due to its rarity. Furthermore, it is unsuitable for skeletal analysis because it is reflected by supersonic waves in the bone cortex. Additionally, when there is a bone in front of a target organ, it is difficult to image the organ for acoustic shadow. In contrast, three-dimensional computed tomography (3D-CT) is useful and has improved the differential diagnosis of FSD during pregnancy (1,4). In 2004, Ruano et al. (5) found that fetal 3D-CT is beneficial for skeletal disorder diagnosis, with added capabilities compared to ultrasonography. Additionally, in 2020, we reported similar results (6). In our precedent study, we reported that 3D-CT was used to diagnose 17 cases of FSD, including six patients with achondroplasia (ACH) and hypochondroplasia (HCH). In this study, we have included more patients with the same diagnosis.
ACH is the most frequent disease in the limb-shortening-type disease group, with an excellent prognosis, and occurs in 1 in 10,000–20,000 births. It is characterized by abnormal bone growth (short arms and legs and a large head) and facial features, including frontal prominence, nasal root depression, and midface hypoplasia (7-9). HCH is also characterized by skeletal features similar to those of ACH but is milder (10). Complications of mental disability may be common in HCH but not in ACH. Nonetheless, prenatal diagnosis of ACH/HCH is difficult before 26 weeks (11); therefore, detecting femoral bowing and shortening of femur length (FL) can be done only after 26 weeks based on sonographic features. However, it is possible to determine a prenatal diagnosis close to an accurate and differential diagnosis of ACH/HCH using 3D-CT after 26 weeks because of the timing of bone ossification.
The heterozygous activating mutation in the fibroblast growth factor receptor 3 (FGFR3) gene’s transmembrane domain causes ACH/HCH (8). Most ACH/HCH cases have new mutations. These mutations account for approximately 95% of ACH and 70% of HCH cases (9). Mutations that cause ACH arise in the same nucleotide, resulting in a glycine-to-arginine (Gly380Arg) or glycine-to-cysteine (Gly380Cys) substitution in the transmembrane domain of the FGFR3 protein (8). In contrast, mutations that cause HCH result in asparagine-to-threonine (Asn540Thr), asparagine-to-lysine (Asn540Lys), or isoleucine-to-valine (Ile538Val) substitutions (8).
Generally, we determine a prenatal diagnosis of FSD using sonography and 3D-CT and a definite diagnosis by gene analysis when we consider a genetic test necessary. Therefore, in this retrospective study, we aimed to determine the efficacy of 3D-CT in the prenatal diagnosis of ACH/HCH. We present this article in accordance with the AME Case Series reporting checklist (available at https://qims.amegroups.com/article/view/10.21037/qims-24-682/rc).
Case presentation
Study design
This was a retrospective study involving pregnant women whose pregnancies or childbirths were managed by our institution from February 2011 to August 2022. Pregnancies were included in the study if the fetus was suspected to have bone abnormalities based on routine ultrasonography. Additionally, we documented family histories of skeletal dysplasia and pregnancy-related complications.
Patients
Patients were selected for the study non-consecutively. Pregnant women who met the following selection criteria were included: (I) pregnancy at age ≥20 years at the time of consent; (II) fetal ultrasonography showing a short femur (below the 10th percentile of fetal measurements) or an image of a long bone curvature or deformity; and (III) written informed consent to undergo 3D-CT.
Prenatal examinations
The Voluson E8 and E10 ultrasound systems (General Electric Healthcare, Tokyo, Japan) were employed to identify additional abnormalities. The operators were all certified perinatal specialists with over 10 years of experience in fetal sonography. After sonography screening, 3D-CT scanning is recommended when fetal long bones, regardless of their shape, are found to be short, as it identifies potential FSD. Following a thorough explanation of potential radiation effects and obtaining informed consent from the mother, 3D-CT (Aquilion RXL; TOSHIBA, Tokyo, Japan) was performed. Predetermined settings (Table 1) were used for volume acquisition to minimize fetal exposure to radiation without compromising image quality. Settings for the 3D-CT consisted of a low-dose scan [a CT dose index volume (CTDIvol) of 3.5 mGy (range, 1.9–4.9 mGy)], which lowered radiation dose by nearly 30% compared with that of a standard abdominal CT.
Table 1
Radiation | Values |
---|---|
CTDIvol (mGy), median (range) | 3.5 (1.9–4.9) |
DLP (mGy·cm), median (range) | 105.8 (58.1–175.8) |
Parameter | |
Slice thickness (mm) | 1.0 |
Slice interval (mm) | 1.0 |
Rotation time (sec) | 0.5 |
Helical pitch | 1.4 |
Voltage (kV) | 120 |
Beam width (mm) | 16 |
3D-CT, three-dimensional computed tomography; CTDIvol, computed tomography dose index volume; DLP, dose length product.
Postnatal examinations
Postnatally, we weighed and measured the newborn, taking an X-ray to ensure proper bone development. After obtaining informed consent from the parents, genetic testing was carried out on patients to establish a diagnosis. The infant’s blood was used to isolate genomic DNA.
Patients’ characteristics
Patients’ characteristics are presented in Table 2. The mean maternal age was 32.4 years (range, 22–41 years), with no family history of skeletal dysplasia in the ACH/HCH group. The first findings showed a shortening of the FL in six patients and fetal growth restriction (FGR) in one. The mean gestational age at delivery was 38.3 weeks (range, 38–39 weeks), and the delivery modes were vaginal delivery for four of the infants and cesarean section (CS) for the other three.
Table 2
Case No. | Maternal age (years) | Family history of SD | First findings | GA at delivery (weeks) | Delivery mode |
---|---|---|---|---|---|
1 | 41 | No | Shortening of FL | 38 | CS |
2 | 33 | No | Shortening of FL | 38 | VD |
3 | 40 | No | FGR | 38 | VD |
4 | 29 | No | Shortening of FL | 38 | VD |
5 | 30 | No | Shortening of FL | 38 | CS |
6 | 32 | No | Shortening of FL | 39 | CS |
7 | 22 | No | Shortening of FL | 39 | VD |
ACH, achondroplasia; HCH, hypochondroplasia; SD, skeletal dysplasia; GA, gestational age; FL, femur length; CS, cesarean section; VD, vaginal delivery; FGR, fetal growth restriction.
Prenatal findings and diagnosis
The mean 3D-CT imaging period was 31.3 weeks (range, 24–35 weeks) of gestation. The prenatal ultrasonography and 3D-CT findings are presented in Table 3, and images of 3D-CT in Figure 1. All seven patients had shortened long bones; three had narrowed thoraxes, and two had trident hands. Macrocephaly was found in three cases; CS was performed in those three cases because of cephalopelvic disproportion. The prenatal diagnosis of ACH was confirmed in patients 1, 2, 3, 6, and 7; however, in patients 4 and 5, we were unable to establish a diagnosis of either ACH or HCH prenatally.
Table 3
Patient No. | GA at 3D-CT (weeks) | Prenatal findings from 3D-CT/sonography | Prenatal diagnosis |
---|---|---|---|
1 | 31 | Shortening of long bones, narrow thorax, trident hand, macrocephaly, hydramnios | ACH |
2 | 33 | Shortening of long bones, narrow thorax | ACH |
3 | 33 | Shortening of long bones, narrow thorax, trident hand | ACH |
4 | 35 | Shortening of long bones | ACH or HCH |
5 | 28 | Shortening of long bones, narrow thorax, macrocephaly | ACH or HCH |
6 | 35 | Shortening of long bones, macrocephaly | ACH |
7 | 24 | Shortening of long bones | ACH |
GA, gestational age; 3D-CT, three-dimensional computed tomography; ACH, achondroplasia; HCH, hypochondroplasia.
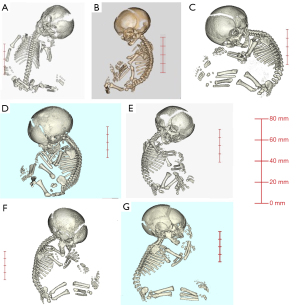
Postnatal diagnosis
The postnatal diagnoses are presented in Table 4. Genetic testing was performed in six infants (cases 1–3 and 5–7) by a direct mutation detection assay using polymerase chain reaction (PCR) to test for mutations in the FGFR3 gene, detected [c. 1138G>A (p. gly380Arg)] in all six infants. We made a definitive diagnosis of ACH based on the genetic testing results. However, in patient 4, genetic testing was not performed as we were unable to obtain informed consent for genetic testing from the infant’s parents. Instead, we diagnosed HCH using X-ray imaging of the total body bones.
Table 4
Patient No. | Birth weight (g) | Postnatal diagnosis | Genetic test [chromosome test] | Prognosis |
---|---|---|---|---|
1 | 2,080 | ACH, down syndrome | FGFR3 gene [c. 1138G>A (p. Gly380Arg), 47,XY,+21] | Alive |
2 | 3,400 | ACH | FGFR3 gene [c. 1138G>A (p. Gly380Arg)] | Alive |
3 | 2,621 | ACH | FGFR3 gene [c. 1138G>A (p. Gly380Arg)] | Alive |
4 | 2,496 | HCH | No genetic test | Alive |
5 | 2,924 | ACH | FGFR3 gene [c. 1138G>A (p. Gly380Arg)] | Alive |
6 | 2,892 | ACH | FGFR3 gene [c. 1138G>A (p. Gly380Arg)] | Alive |
7 | 3,210 | ACH | FGFR3 gene [c. 1138G>A (p. Gly380Arg)] | Alive |
ACH, achondroplasia; HCH, hypochondroplasia.
Based on ultrasound and 3D-CT scans, patient 1 was diagnosed prenatally with ACH due to shortened long bones, a narrowed thorax, and a trident hand. Following birth, Down syndrome’s unique features (narrow eyelid grooves, low nasal bridge, and large tongue) were observed alongside the following chromosomal findings: G-band; 47, XY, +21. Therefore, based on the results (genetic and chromosomal testing), patient 1 was diagnosed with ACH and Down syndrome.
Ethical statement
All procedures performed in this study were in accordance with the ethical standards of the institutional and/or national research committee(s) and with the Helsinki Declaration (as revised in 2013). The study was approved by the institutional review board of Kyoto Prefectural University of Medicine (No. ERB-C-991). Written informed consent was obtained from the pregnant women for publication of this case report and accompanying images. A copy of the written consent is available for review by the editorial office of this journal.
Discussion
Fetal ultrasonography is the primary diagnostic tool for identifying FSD. Ultrasonography is used for skeletal analysis due to its reflection of the bone cortex. Approximately 65% of prenatal diagnoses for skeletal dysplasias are accurate (12). Additionally, errors may occur among operators because advanced operation technology and abundant knowledge are essential to perform fetal sonography. Notably, 3D-CT complements ultrasonography in diagnosing and classifying FSD, but it is crucial to optimize ultrasound settings to reduce fetal radiation exposure without sacrificing image quality (11,13). Furthermore, 3D images enable more precise diagnoses from a 360-degree perspective by revealing the direction with the most visibility. In our institution, the dose of fetal 3D-CT was a mean CTDIvol of 3.5 mGy (range, 1.9–4.9 mGy), a “low dose” for fetal CT. The doses for fetal 3D-CT were reportedly a mean CTDIvol of 2.2 mGy in 2000 and 4.2 mGy in 2013 (12,14). In 2008, the International Commission on Radiological Protection determined that the intelligence quotient impact of in-utero exposure to less than 100 mGy is negligible, and the cancer risk following in-utero exposure would be associated with early childhood irradiation (15). Therefore, using low-dose 3D-CT imaging, we can create a maximum intensity projection (MIP) image of the point to be focused on. The MIP image of the fetus resembles all bone X-ray images of the infant and aids in making an accurate diagnosis of FSD.
ACH is the most common disease in limb-shortening skeletal dysplasia, with a benign prognosis. The estimated occurrence is 1 in 10,000 to 30,000 live births (16). ACH sonographic features are short limbs, femoral bowing, trident hand shape, narrow chest, square iliac bones, and polyhydramnios (7). Moreover, detecting limb shortening before 26 weeks was challenging because it appears during 22 to 26 weeks of pregnancy. The ossification is normal, and the limbs showed a slight curve without bone fractures. The calvarial expansion causes enlargement of the fetal biparietal diameter. The skeletal features of HCH are remarkably like those of ACH but usually tend to be milder. ACH/HCH can be difficult to differentiate from Down syndrome (17,18). During the second trimester, femoral shortening increases the risk of chromosomal abnormalities, including Down syndrome (19,20). Fetal femoral shortening may indicate chromosomal abnormalities, FGR, or other anomalies (20).
Non-skeletal symptoms are also crucial for proper differential diagnosis. We encountered a case of complicated ACH with Down syndrome; however, in the prenatal diagnosis of this case, we were unable to find the views to suspect Down syndrome because features of both ACH and Down syndrome are relatively common, i.e., shortening of long bones and macrocephaly. The three-dimensional sonography and 3D-CT images obtained during the prenatal period are shown in Figure 2A; X-rays during the postnatal period are shown in Figure 2B. Macrocephaly, shortening of the femur and humerus, trident hands, and hydramnios were observed on the sonogram. Additionally, we detected shortening of long bones, a narrow thorax, and trident hands by prenatal 3D-CT (Figures 1A,2A). These results led to the prenatal diagnosis of ACH. The results of the postnatal X-ray of the infant in patient 1 (Figure 2B) also support our prenatal diagnoses. However, this infant had characteristic facial features of Down syndrome; therefore, we suspected a complication of Down syndrome with ACH and performed genetic and chromosomal tests for an accurate diagnosis. There is rarely a co-occurrence of both conditions in the same patient (17). In cases with both ACH and Down syndrome, diagnosing the co-occurrence prenatally without typical Down syndrome markers may be challenging.
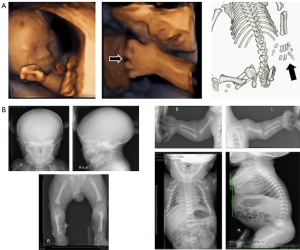
A strength of this study is the high quality of the fetal diagnosis using ultrasonography and 3D-CT. At our institution, we are experts at fetal sonography and perform high-quality examinations. Another strength was that genetic testing was completed by a direct mutation detection assay using PCR in the FGFR3 gene for infants in our institution.
ACH and HCH are autosomal-dominant disorders caused by mutations in the FGFR3 gene. Birth weight and length in HCH are often within the normal range, and the disproportion in limb-to-trunk length is often mild; however, the most common presenting feature is short stature with disproportionate limbs (21). The medical problems associated with skeletal features common in ACH occur less frequently in HCH; however, deficits in mental capacity may be more common in HCH than in ACH. In patient 4, we were unable to obtain informed consent from the parents; thus, we diagnosed HCH based on the images obtained by prenatal sonography, 3D-CT, and postnatal X-ray without genetic testing. The first finding of shortening of the femur was at 34 weeks of gestation, and fetal 3D-CT was performed at 35 weeks. Therefore, we were unable to discern whether ACH or HCH was present at the prenatal stage. On postnatal day 1, we performed an X-ray of the infant and noted a bell-shaped thorax, narrowed pelvic cavity, expanded metaphysis, lumbar kyphosis, and cupped distal ulna (Figure 3). We diagnosed the infant with HCH based on prenatal and postnatal images. A limitation of this study was the small database from a single institution. Therefore, our findings may be limited to race and residential area and not generalizable.
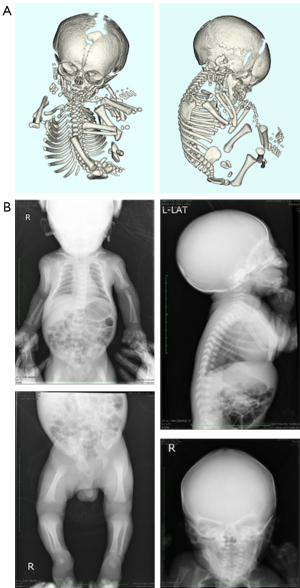
Conclusions
ACH and its mild form, HCH, are the most common forms of skeletal dysplasia. However, the prenatal diagnosis of ACH and HCH requires differentiation from FGR, chromosome aberrations and other limb-shortening diseases. Our results demonstrated that 3D-CT is a valuable tool to augment ultrasonography in diagnosing FSD. An accurate prenatal diagnosis is crucial for a definitive diagnosis using genetic screening for infants.
Acknowledgments
We would like to thank Editage (www.editage.com) for English language editing.
Funding: None.
Footnote
Reporting Checklist: The authors have completed the AME Case Series reporting checklist. Available at https://qims.amegroups.com/article/view/10.21037/qims-24-682/rc
Conflicts of Interest: All authors have completed the ICMJE uniform disclosure form (available at https://qims.amegroups.com/article/view/10.21037/qims-24-682/coif). The authors have no conflicts of interest to declare.
Ethical Statement: The authors are accountable for all aspects of the work in ensuring that questions related to the accuracy or integrity of any part of the work are appropriately investigated and resolved. All procedures performed in this study were in accordance with the ethical standards of the institutional and/or national research committee(s) and with the Helsinki Declaration (as revised in 2013). The study was approved by the institutional review board of Kyoto Prefectural University of Medicine (No. ERB-C-991). Written informed consent was obtained from the pregnant women for publication of this case report and accompanying images. A copy of the written consent is available for review by the editorial office of this journal.
Open Access Statement: This is an Open Access article distributed in accordance with the Creative Commons Attribution-NonCommercial-NoDerivs 4.0 International License (CC BY-NC-ND 4.0), which permits the non-commercial replication and distribution of the article with the strict proviso that no changes or edits are made and the original work is properly cited (including links to both the formal publication through the relevant DOI and the license). See: https://creativecommons.org/licenses/by-nc-nd/4.0/.
References
- Krakow D, Lachman RS, Rimoin DL. Guidelines for the prenatal diagnosis of fetal skeletal dysplasias. Genet Med 2009;11:127-33. [Crossref] [PubMed]
- Chen C, Jiang Y, Xu C, Liu X, Hu L, Xiang Y, Chen Q, Chen D, Li H, Xu X, Tang S. Skeleton Genetics: a comprehensive database for genes and mutations related to genetic skeletal disorders. Database (Oxford) 2016;2016:baw127. [Crossref] [PubMed]
- Unger S, Ferreira CR, Mortier GR, Ali H, Bertola DR, Calder A, et al. Nosology of genetic skeletal disorders: 2023 revision. Am J Med Genet A 2023;191:1164-209. [Crossref] [PubMed]
- Schumacher R, Seaver LH, Spranger J. Fetal Radiology A Diagnostic Atlas. Springer-verlag, Berlin, 2004.
- Ruano R, Molho M, Roume J, Ville Y. Prenatal diagnosis of fetal skeletal dysplasias by combining two-dimensional and three-dimensional ultrasound and intrauterine three-dimensional helical computer tomography. Ultrasound Obstet Gynecol 2004;24:134-40. [Crossref] [PubMed]
- Waratani M, Ito F, Tanaka Y, Mabuchi A, Mori T, Kitawaki J. Prenatal diagnosis of fetal skeletal dysplasia using 3-dimensional computed tomography: a prospective study. BMC Musculoskelet Disord 2020;21:662. [Crossref] [PubMed]
- Leiva-Gea A, Martos Lirio MF, Barreda Bonis AC, Marín Del Barrio S, Heath KE, Marín Reina P, Guillén-Navarro E, Santos Simarro F, Riaño Galán I, Yeste Fernández D, Leiva-Gea I. Achondroplasia: Update on diagnosis, follow-up and treatment. An Pediatr (Engl Ed) 2022;97:423.e1-423.e11.
- Legeai-Mallet L, Savarirayan R. Novel therapeutic approaches for the treatment of achondroplasia. Bone 2020;141:115579. [Crossref] [PubMed]
- Baujat G, Legeai-Mallet L, Finidori G, Cormier-Daire V, Le Merrer M. Achondroplasia. Best Pract Res Clin Rheumatol 2008;22:3-18. [Crossref] [PubMed]
- Borber MB, Bellus GA, Nikkel SM, Tiller GE. Hypochondroplasia. GeneReviews®. Internet. In: Adam MP, Mirzaa GM, Pagon RA, Wallace SE, Bean LJH, Gripp KW, Amemiya A. editors. University of Washington, Seattle, 1999:1993-2023.
- Macé G, Sonigo P, Cormier-Daire V, Aubry MC, Martinovic J, Elie C, Gonzales M, Carbonne B, Dumez Y, Le Merrer M, Brunelle F, Benachi A. Three-dimensional helical computed tomography in prenatal diagnosis of fetal skeletal dysplasia. Ultrasound Obstet Gynecol 2013;42:161-8. [Crossref] [PubMed]
- Doray B, Favre R, Viville B, Langer B, Dreyfus M, Stoll C. Prenatal sonographic diagnosis of skeletal dysplasias. A report of 47 cases. Ann Genet 2000;43:163-9. [Crossref] [PubMed]
- Adler-Levy Y, Yagel S, Nadjari M, Bar-ziv Y, Simanovsky N, Hiller N. Use of low dose computed tomography with 3D reconstructions for the prenatal evaluation of suspected skeletal dysplasia. Isr Med Assoc J 2015;17:42-6. [PubMed]
- Victoria T, Epelman M, Coleman BG, Horii S, Oliver ER, Mahboubi S, Khalek N, Kasperski S, Edgar JC, Jaramillo D. Low-dose fetal CT in the prenatal evaluation of skeletal dysplasias and other severe skeletal abnormalities. AJR Am J Roentgenol 2013;200:989-1000. [Crossref] [PubMed]
- Wrixon AD. New ICRP recommendations. J Radiol Prot 2008;28:161-8. [Crossref] [PubMed]
- Ornitz DM, Legeai-Mallet L. Achondroplasia: Development, pathogenesis, and therapy. Dev Dyn 2017;246:291-309. [Crossref] [PubMed]
- de Azevedo Moreira LM, Matos MA, Schiper PP, Carvalho AF, Gomes IC, Rolemberg JC, Ferreira de Lima RL, Toralles MB. Co-occurrence of achondroplasia and Down syndrome: Genotype/phenotype association. Birth Defects Res A Clin Mol Teratol 2010;88:228-31. [Crossref] [PubMed]
- Smith-Bindman R, Hosmer W, Feldstein VA, Deeks JJ, Goldberg JD. Second-trimester ultrasound to detect fetuses with Down syndrome: a meta-analysis. JAMA 2001;285:1044-55. [Crossref] [PubMed]
- Mathiesen JM, Aksglaede L, Skibsted L, Petersen OB, Tabor A. Outcome of fetuses with short femur length detected at second-trimester anomaly scan: a national survey. Ultrasound Obstet Gynecol 2014;44:160-5. [Crossref] [PubMed]
- Kaga A, Murotsuki J, Kamimura M, Kimura M, Saito-Hakoda A, Kanno J, Hoshi K, Kure S, Fujiwara I. Association of achondroplasia with Down syndrome: difficulty in prenatal diagnosis by sonographic and 3-D helical computed tomographic analyses. Congenit Anom (Kyoto) 2015;55:116-20. [Crossref] [PubMed]
- Cassart M, Massez A, Cos T, Tecco L, Thomas D, Van Regemorter N, Avni F. Contribution of three-dimensional computed tomography in the assessment of fetal skeletal dysplasia. Ultrasound Obstet Gynecol 2007;29:537-43. [Crossref] [PubMed]