Image fusion for intraoperative evaluation of microwave ablation in non-small cell lung cancer: a retrospective cohort study
Introduction
Surgical resection remains the main treatment method for early-stage non-small cell lung cancer (NSCLC) (1). Lobectomies are the gold standard for the surgical treatment of early NSCLC, with a reported 5-year overall survival (OS) rate ranging from 60% to 80% (2,3). However, about 20% of patients with NSCLC are intolerant or refuse surgical resection (mostly due to comorbidities), and the 5-year OS rate for these patients is poor (ranging from 6% to 14%) (4,5). In recent years, thermal ablation has made progress in improving the local control and the long-term survival of patients with inoperable early-stage NSCLC. Thermal ablation mainly consists of radiofrequency ablation (RFA) and microwave ablation (MWA). In comparison with RFA, MWA has been shown to achieve higher intratumoral temperatures, a larger ablation zone, and less energy dissipation to adjacent vasculature or bronchi (heat-sink effect) (6,7). In a retrospective study, MWA therapy was performed on 50 patients with malignant lung tumors and achieved a local control rate of 67% and a 3-year survival rate of 45%. Overall, research has shown that MWA is effective and safe (6). One study used computed tomography (CT)-guided MWA in 47 patients with stage I peripheral inoperable NSCLC, which yielded local control rates of 96%, 64%, and 48% at 1, 3, and 5 years, respectively. The median cancer-specific survival and OS were 47.4 and 33.8 months, respectively (8). In a retrospective study, the efficacy and complications of MWA and lobectomy were compared in patients with stage I NSCLC. There was no significant difference in OS rate and disease-free recurrence survival rate between the two groups, but the incidence of complications in the MWA group was significantly lower than that in the lobectomy group. Therefore, MWA has similar efficacy as that of lobectomy in the treatment of stage I NSCLC but fewer complications (9). As a nonionizing radiation, thermal ablation represents the expansion of the treatment options for patients with early NSCLC who face high risks in surgery.
Image fusion is the process of combining multiple images based on their prominent features or spatial attributes to produce a single fused image, with a single or multiple modes being used to achieve improved or optimal results. The common practice of image fusion involves an image registration to process data alignment from various multimodal or time frame images, as well as image segmentation to manage the description and determination of related items in two-dimensional (2D) and three-dimensional (3D) visual data images (10). CT is the primary method of screening and diagnosing NSCLC (11) and shows some consistency with pathological diagnosis (12-14). CT is also the most commonly used technique for imaging guidance and immediate evaluation in MWA ablation therapy (15,16). In CT imaging after ablation, the density of the ablation zone is similar to that of pulmonary nodules, leading to confusion in imaging between these two regions. Therefore, fusing the pulmonary nodule images before ablation with ablation zone images after ablation can help the operators determine whether ablation is complete. However, the operator can only roughly estimate the relative position between the lesion and ablation zone during the operation using CT images, as the densities of the ablation zone and the lesion are highly similar (17). Currently, there is a lack of effective strategies for evaluating the relative position between the ablation zone and the lesion (18). The aims of this study were thus to validate the feasibility of using a 3D quantitative method of CT image fusion to determine whether the target regions have been completely ablated during MWA and to postoperatively analyze the ablative margin. Intraoperative image fusion may be able to obtain critical information regarding the success of the technique at the first opportunity. We present this article in accordance with the STROBE reporting checklist (available at https://qims.amegroups.com/article/view/10.21037/qims-24-690/rc).
Methods
Patients
This single-center retrospective observational study was carried out in Shanghai Ninth People’s Hospital, Shanghai Jiao Tong University School of Medicine, from August 2019 to August 2021.Through the admission system of Shanghai Ninth People’s Hospital, we obtained the information of patients who underwent CT-guided percutaneous MWA of NSCLC, consecutively enrolled these patients, and recorded their information. This study was conducted in accordance with the Declaration of Helsinki (as revised in 2013) and was approved by the Institutional Review Board of Shanghai Ninth People’s Hospital, Shanghai Jiao Tong University School of Medicine (approval No. SH9H-2022-171-1). Informed consent was obtained from all the patients.
A total of 29 patients with histologically proven stage IA or IB NSCLC were treated with CT-guided MWA and retrospectively analyzed (Table 1). In some lesions, we performed biopsies using a coaxial guide ablation needle sheath before ablation.
Table 1
Characteristic | Value |
---|---|
Age (years) | 65 (54, 77) |
Sex | |
Male | 12 (41.38) |
Female | 17 (58.62) |
Location | |
Upper lobe of left lung | 7 (21.88) |
Lower lobe of left lung | 3 (9.38) |
Upper lobe of right lung | 11 (34.38) |
Middle lobe of right lung | 1 (3.13) |
Lower lobe of right lung | 10 (31.25) |
History of lung surgery | |
Yes | 21 (72.4) |
No | 8 (27.6) |
Reason for MWA treatment | |
Treatment of secondary lesions following resection of primary lesion | 24 (75) |
Inability to tolerate surgery | 8 (25) |
Data are presented as median (interquartile range) or number (%). MWA, microwave ablation.
The inclusion criteria for the study were as follows: (I) stage IA or IB (based on the ninth edition of the TNM staging) pathologically confirmed NSCLC, (II) age ≥18 years, (III) noncentral tumor (distance between the tumor and the hilum ≥1 cm), (IV) surgical inoperability or refusal of surgery, (V) a lack of chemotherapy or radiotherapy before ablation, and (VI) Eastern Cooperative Oncology Group (ECOG) performance status of 0 to 2. Meanwhile, the exclusion criteria were as follows: (I) a lack of systemic examination before enrollment in the study, (II) a history of other primary cancers, and (III) central tumor (distance between the tumor and the hilum <1 cm).
Microwave ablation
All cases underwent whole-chest CT scanning after ablation. In some lesions, we performed biopsies using a coaxial guide ablation needle sheath before ablation. We then immediately began ablation after biopsy (19). Percutaneous CT-guided MWA was performed at the lung nodule’s site, and intraoperative image scanning was performed using a SOMATOM Definition CT (Siemens Healthineers, Erlangen, Germany). An MTC-3C Microwave Ablation System (National Medical Products Administration registration certificate No. 201530919781; Vison-China Medical Devices R&D Center, China) was used for MWA, with a microwave emission frequency of 2,450±50 Hz, an average output power of 45.2±3.5 watts, and an output time of 6.2±1.0 min.
According to existing research, ground-glass nodules (GGNs) with a solid component less than 25% and less than 2 cm under the 0804 standard (20) have almost no lymph node metastasis, making them suitable for ablation treatment. If the fused image ablation area is not covering the lesion during ablation, the ablation is considered incomplete, and supplementary ablation is required. Only when the ablation zone completely covers the lesion can the ablation treatment be considered complete.
Image fusion
For the acquisition of CT images, SOMATOM Definition CT unit (Siemens Healthineers) was used for intraoperative image scanning with a slice thickness of 1.5 mm, and pre- and postablation images were acquired separately. The postablation images used for image fusion were obtained immediately after ablation and 5 minutes after catheter removal. Images were transferred to the workstation in Digital Imaging and Communications in Medicine (DICOM) format.
The image fusion method we adopted was manual image fusion. Manual image fusion was performed with Lifex v. 7.2.0 software by a radiologist with 10 years of clinical experience who was blinded to the purpose of the study.
For the definition of successful image fusion, we employed the rigid image alignment method. This method was based on highly reproducible and relevant structural points adjacent to the lesion. The main structures adjacent to the lesion were selected for registration to best reflect the relative positions of the lesion and the ablation zone and to ensure that the structures in other positions corresponded to each other as much as possible. Therefore, all of our fused CT images had more than five rigid registration points (blood vessels, the trachea, and the bony structures of the chest wall, among others), and the images before and after ablation were aligned as much as possible. Subsequently, the built-in color bands of the software were used to convert the color of CT images before and after ablation into high contrast colors, in order to obtain the image information that had had strongest contrast between the lesion and the ablation zone, with the need to minimize image noise also being taken into account (Figures 1,2).
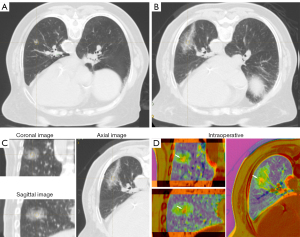
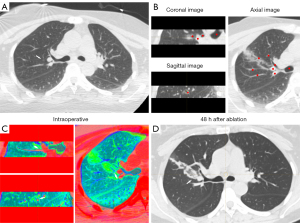
For the interpretation of fused image clarity, two clinicians (one thoracic surgeon and one radiologist) interpreted the multilayer CT images (coronal, sagittal, and axial) immediately after ablation and after software fusion to identify the relative positions of the lesion and the ablation zone at each level for measurement of the minimum ablative margin (MAM). Images were scored 3 points if the lesion and the ablation area could be clearly identified in all dimensions, 2 points if they were clear in two dimensions, 1 point if they were clear in one dimension, and 0 points if they were completely unclear.
In cases involving intraoperative pneumothorax complications, a tube was placed before image fusion to drain the gas sufficiently to allow maximum lung tissue re-expansion before image fusion, in order to avoid fusion failure caused by chest structural deformation due to lung tissue atrophy (Figure 3). In cases involving bleeding complications, which could partially obscure some major structures, the trachea and vascular alignment were carefully identified and image registration performed based on the images from three different dimensions (Figure 4).
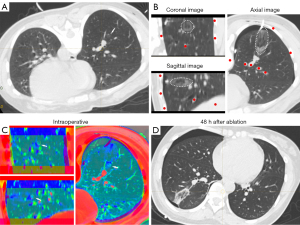
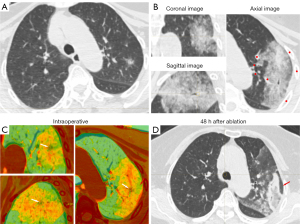
Follow-up
All patients underwent a chest CT scans immediately after ablation, 5 minutes after catheter removal, and the second day after ablation. Subsequently, chest CT examinations are conducted for follow-up at 1 month, 3 months, and 6 months after ablation. CT images from the 6-month follow-up examination were used to confirm technical success and complete ablation. Complete ablation was defined as the disappearance of the lesion, complete cavity formation, fibrosis of the lesion, pulmonary atelectasis, and absence of contrast-enhanced signs of the lesion on enhanced CT.
Data collection
Patient data and lesion characteristics (Table 1), including general patient history, pathology data of 32 lesions (Table 2), location, maximum diameter, and CT values of 32 lesions (Table 3), were collected. Data on the ablation procedure (Table 3), ablation time, ablation power, complications, numerical rating scale (NRS) indicating intraoperative discomfort score, success of the technique in the immediate intraoperative period, and complete ablation as evaluated by CT imaging at 6 months were recorded.
Table 2
History of lung surgery | Lesion number | Pathology | |
---|---|---|---|
Pulmonary nodule biopsy, biopsy of ablated lesion | Surgery, previous surgical pathology | ||
Yes | 24 | Adenocarcinoma, 2 (8.3) | AIS, 1 (4.2) |
Unclear, 2 (8.3) | MIA, 12 (50.0) | ||
None, 20 (83.4) | Invasive adenocarcinoma, 11 (45.8) | ||
No | 8 | AIS, 2 (25.0) | None |
Adenocarcinoma, 6 (75.0) |
Data are presented as number (%). AIS, adenocarcinoma in situ; MIA, minimally invasive adenocarcinoma.
Table 3
Ablation method | Microwave ablation |
---|---|
Lesion maximum diameter (mm) | 8.15 (6.6, 11.15) |
Lesion CT value (HU) | −560.5 (−629.5, −416.5) |
Ablation power (W) | 45 (45, 48.75) |
Ablation time (min) | 6 (5.25, 6.75) |
Intraoperative technical evaluation | |
Success | 29 (90.63) |
Failure | 2 (6.25) |
Undetermined | 1 (3.12) |
Number of lesions completely ablated (6 months) | |
Complete ablation | 30 (93.75) |
Incomplete ablation | 2 (6.25) |
Complications | |
Bleeding | 3 (9.38) |
Pneumothorax | 9 (28.13) |
Discomfort | 32 (100.00) |
NRS | |
1 | 9 (28.13) |
2 | 13 (40.62) |
3 | 10 (31.25) |
Data are presented as median (interquartile range) or number (%). CT, computed tomography; HU, Hounsfield unit; NRS, numerical rating scale.
Statistical analysis
Categorical variables are presented as frequencies and percentages (Tables 1-5), while continuous variables are presented as the mean ± standard deviation (SD) (Table 5) or as the median and interquartile range (IQR; 25th–75th percentile) (Tables 1,3). The chi-square test was used to calculate the kappa coefficients between the two investigators according to the following scheme: κ>0.75, good agreement, 0.75≥κ>0.5, fair agreement; and κ≤0.5 poor agreement. The correlation between each characteristic parameter of the lesion and image quality was analyzed with binary logistic regression. Statistical significance was defined as a two-sided P value <0.05. SPSS 25 software (IBM Corp., Armonk, NY, USA) was used for statistical analysis.
Table 4
Successful identification | Original CT images after ablation | Fused images after ablation |
---|---|---|
Thoracic surgeon | 7 (7.52) | 81 (87.1) |
Radiologist | 12 (12.9) | 80 (86.0) |
Data are presented as number (%). CT, computed tomography.
Table 5
Variable | Coronal | Sagittal | Axial | Total |
---|---|---|---|---|
MAM frequency | 14 (48.28) | 9 (31.03) | 6 (20.69) | 29 (100) |
Average MAM (mm) | 3.60±1.43 | 3.94±1.94 | 4.04±1.96 | 3.05±1.35 |
Data are presented as mean ± standard deviation or number (%). MAM, minimum ablative margin.
Results
Patients and pathology data
During the study period, 69 of 98 patients who underwent CT-guided percutaneous MWA of NSCLC were excluded (Figure 5), 31 due to their NSCLC stage exceeding stage IB, 26 due to a history of other primary cancers, and 12 for other reasons such as central tumor (distance between the tumor and the hilum <1 cm) or for receiving chemotherapy or radiotherapy before ablation.
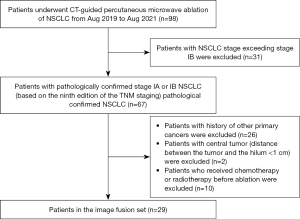
The median age of the remaining 29 patients, comprising 32 lesions was 65 years (IQR 54–77 years). Most lesions were located in the upper and lower lobes of the right lung, and 75% of the patients had previously undergone minimally invasive video-assisted thoracoscopic surgery (Table 1).
Pathology after lung surgery showed adenocarcinoma in situ in 1 case (4.2%), minimally invasive adenocarcinoma in 12 cases (50.0%), and invasive adenocarcinoma in 11 cases (45.8%). Among these 24 cases, a biopsy during MWA was performed in 4 cases, leading to 2 diagnoses of adenocarcinoma, with the other 2 biopsies being uninformative. Biopsies were obtained during ablation in 8 patients who did not undergo lung surgery. Among them, 2 cases were pathologically diagnosed as adenocarcinoma in situ and 6 cases as adenocarcinoma (Table 2).
MWA was used for all 32 lesions examined in the study. In 3 patients, 2 lesions were ablated. Image fusion was successful in 31 of 32 cases immediately after completion of ablation, while one case showed image fusion failure (fusion success rate 96.88%). The fusion time was 8–15 minutes (median time 10 minutes). The clinical data of patients are shown in Table 3.
The success rates of identification with fused image
One thoracic surgeon and one radiologist interpreted the 31 cases of successful intraoperative image fusion and their corresponding immediate postablation CT images in three dimensions: coronal, sagittal, and transverse. Images were scored 3 points if completely clear, 2 points if clear in two dimensions, 1 point if clear in one dimension, and 0 points if completely unclear. The scoring table is shown in Tables S1,S2. The success rates of identification through use of the original postoperative CT images by the two clinicians were only 7.52% and 12.90%, respectively. However, the image interpretation success rates after image fusion were 87.1% and 86.0%, respectively (Table 4, Figure 6). The kappa coefficient of the consistency test in the latter case was 0.859 (P<0.001) (Tables S3,S4).
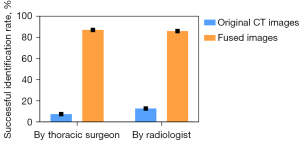
The relationship between image fusion and lesions
The quality of the fused image was positively correlated with the CT value but not correlated with the size or position of the target regions (Table S5). Intraoperative image fusion indicated technical success in 29 cases, and the MAM was 3.05±1.35 mm (range 1.1–6.7 mm). The MAM appeared in the coronal plane, sagittal plane, and axial plane accounting for 48.28%, 31.03%, and 20.69% respectively (Table 5, Figure S1). Two cases showed possible residual lesions, and one case could not be assessed due to image fusion failure, yielding a technical success rate of 90.6%. Six months after ablation, 29 patients underwent follow-up chest CT scans. One doctor reinterpreted the CT images of the 32 lesions and found that all lesions reached the standard of complete ablation. The assessments of complete ablation immediately after ablation based on image fusions were identical to the those on chest CT at 6 months postablation, with 100% sensitivity and specificity.
Discussion
Ablation of early-stage NSCLC differs from that of solid lung nodules. The background of early-stage NSCLC is lung tissue with visible blood vessels and airways (21,22). The edema reaction of lung tissue subjected to MWA is slow, resulting in an indistinct ablation zone during ablation, thus rendering it difficult to determine the actual range of the ablation. Typically 24–48 hours are needed after ablation to obtain a distinct image of the ablation zone (23-25). During operation, the target after ablation can be confused with the ablation zone, and it this thus challenging to identify the position of the target on the CT image and consequently difficult to immediately determine whether there are residual lesions or not (24). In addition, intraoperative complications such as pneumothorax and hemothorax can complicate the assessment of the relative positions of the lesion and the ablation zone (23). Currently, we use manual rigid alignment for image fusion, which takes about 8–15 minutes (with a median time of 10 minutes) We select images with minimal deformation for manual fusion registration and planes with minimal interference and ensure the collection of as many registration points as possible and as close to the target as possible, along with different dimensions of multiangle registration observation, to avoid the error caused by context change. Moreover, the fused image can help the operator distinguish similar colors of gray, black, and white in the original CT images through use of richer and more contrasting colors, which can facilitate the discernment of the relative positions of the target and the ablation more clearly. The feedback data from this process are crucial. Clear intraoperative identification of the relative position of the lesion and the ablation zone is essential for immediate the assessment of technical success.
The statistical analysis in our study revealed a positive correlation between the quality of the fused image and the CT value of the lesion. This is due to principle of the software discrimination of the lung nodules and the ablation boundaries after fusion of the same layers of images being based on the comparison of the CT values of the preoperative lesion and the postoperative ablation zone. Superimposition of the ablation zone with heterogeneous CT values made it difficult to clearly distinguish between these two areas through the contrast of colors in some fused images. It is hoped that image fusion software can further refine the contrast color corresponding to the CT value, expand the color bar, and leverage artificial intelligence to assist in capturing lesions on fused CT images so as to improve the quality of image fusion.
In previous studies, the MAM after ablation was considered to be >5 mm (18), which was based primarily on evaluation of CT images obtained 24 hours postoperatively. However, our observations indicated that the MAM in most cases is actually less than 5 mm. After 6 months of follow-up, we found that as long as the ablation zone covered the targeted area, complete ablation was achieved. The possible reasons for this finding are as follows: (I) early-stage NSCLC was the object of ablation, and (II) the MAM was measured immediately after ablation. First, according to the recent research (20), early-stage NSCLC typically has a consolidation-to-tumor ratio of 25%. The density of lung nodules is similar to that of normal lung tissue. After ablation, both the lesion and its surrounding lung tissue will shrink to some extent, and the shrinkage occurs mainly in the transverse plane perpendicular to the microwave needle. This can result in a more elongated appearance of the ablation zone, with tissue shrinkage of up to 20–65% after ablation, so the original ablation zone may be underestimated by 30–60% (26). Meanwhile, in this study, the images of the GGN used for image fusion were obtained from preablation CT images. We fused the original sized lung nodules before ablation with the shrunken ablation zone after ablation, resulting in underestimation of the MAM. Second, the intraoperative ablation zone appeared as an exudative shadow with unclear borders on CT, providing only a rough scope. Some studies have shown that the ablation zone margin starts to gradually expand within 12 hours postoperatively, and the actual ablation zone is gradually clearer at 24–48 hours postoperatively (25); thus, the actual MAM may be larger than the MAM on fused images. Finally, we measured the MAM of coronal plane, sagittal plane, and axial plane of each fused image. Interestingly, we found that only 29% of the MAM was shown in the axial plane. This highlights the importance of multidimensional image observation in accurately determining the MAM rather than solely relying on observations in the axial plane (27).
This study involved certain limitations which should be addressed. One limitation was that the image fusion software for automatic image registration lacked context-awareness depth estimation, so we adopted manual rigid image registration to avoid the error caused by context change as much as possible. However, there was still 1 case of image fusion failure. This was likely due to displacement of chest structure, such as the base of the lung near the diaphragm, greater respiratory movement, and complications such as pneumothorax (Figure 7). These can all cause severe deformation and displacement of structures, leading to image fusion failure. In the future, we wish to use an artificial intelligence software with context-awareness capability to resolve the above issues. Another limitation was that we only reported a limited number of cases in which pathological assessments were not performed before ablation of secondary lesions after surgical resection of a portion of the primary lesion; this is because the secondary lesions were not of higher pathological grade than the primary lesions in terms of imaging diagnosis, and multiple biopsies might have increased the intraoperative risk. Furthermore, early-stage lung nodules are highly indolent, and this part of the lesion may require a longer follow-up period for observation.
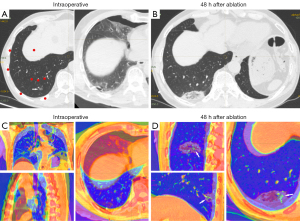
Conclusions
Image fusion techniques may be highly valuable to ensuring immediate technical success of ablation procedures. Assessment of the relative position of the lesion and ablation zone in the fused image can facilitate real-time decision-making for intraoperative treatment and provide guidance for postoperative follow-up, facilitating timely remedial ablation if necessary.
Acknowledgments
We would like to thank the doctors at the Department of Thoracic Surgery, Shanghai Ninth People’s Hospital, Shanghai Jiao Tong University School of Medicine for their assistance.
Funding: This study received funding from
Footnote
Reporting Checklist: The authors have completed the STROBE reporting checklist. Available at https://qims.amegroups.com/article/view/10.21037/qims-24-690/rc
Conflicts of Interest: All authors have completed the ICMJE uniform disclosure form (available at https://qims.amegroups.com/article/view/10.21037/qims-24-690/coif). The authors have no conflicts of interest to declare.
Ethical Statement: The authors are accountable for all aspects of the work in ensuring that questions related to the accuracy or integrity of any part of the work are appropriately investigated and resolved. This study was conducted in accordance with the Declaration of Helsinki (as revised in 2013) and was approved by the Institutional Review Board of Shanghai Ninth People’s Hospital, Shanghai Jiao Tong University School of Medicine (approval No. SH9H-2022-171-1). Informed consent was obtained from all patients.
Open Access Statement: This is an Open Access article distributed in accordance with the Creative Commons Attribution-NonCommercial-NoDerivs 4.0 International License (CC BY-NC-ND 4.0), which permits the non-commercial replication and distribution of the article with the strict proviso that no changes or edits are made and the original work is properly cited (including links to both the formal publication through the relevant DOI and the license). See: https://creativecommons.org/licenses/by-nc-nd/4.0/.
References
- Ettinger DS, Wood DE, Aisner DL, Akerley W, Bauman JR, Bharat A, et al. NCCN Guidelines® Insights: Non-Small Cell Lung Cancer, Version 2.2023. J Natl Compr Canc Netw 2023;21:340-50. [Crossref] [PubMed]
- Ginsberg RJ, Rubinstein LV. Randomized trial of lobectomy versus limited resection for T1 N0 non-small cell lung cancer. Lung Cancer Study Group. Ann Thorac Surg 1995;60:615-22; discussion 622-3. [Crossref] [PubMed]
- El-Sherif A, Gooding WE, Santos R, Pettiford B, Ferson PF, Fernando HC, Urda SJ, Luketich JD, Landreneau RJ. Outcomes of sublobar resection versus lobectomy for stage I non-small cell lung cancer: a 13-year analysis. Ann Thorac Surg 2006;82:408-15; discussion 415-6. [Crossref] [PubMed]
- Raz DJ, Zell JA, Ou SH, Gandara DR, Anton-Culver H, Jablons DM. Natural history of stage I non-small cell lung cancer: implications for early detection. Chest 2007;132:193-9. [Crossref] [PubMed]
- McGarry RC, Song G, des Rosiers P, Timmerman R. Observation-only management of early stage, medically inoperable lung cancer: poor outcome. Chest 2002;121:1155-8. [Crossref] [PubMed]
- Wolf FJ, Grand DJ, Machan JT, Dipetrillo TA, Mayo-Smith WW, Dupuy DE. Microwave ablation of lung malignancies: effectiveness, CT findings, and safety in 50 patients. Radiology 2008;247:871-9. [Crossref] [PubMed]
- Quirk MT, Lee S, Murali N, Genshaft S, Abtin F, Suh R. Alternatives to Surgery for Early-Stage Non-Small Cell Lung Cancer: Thermal Ablation. Clin Chest Med 2020;41:197-210. [Crossref] [PubMed]
- Yang X, Ye X, Zheng A, Huang G, Ni X, Wang J, Han X, Li W, Wei Z. Percutaneous microwave ablation of stage I medically inoperable non-small cell lung cancer: clinical evaluation of 47 cases. J Surg Oncol 2014;110:758-63. [Crossref] [PubMed]
- Yao W, Lu M, Fan W, Huang J, Gu Y, Gao F, Wang Y, Li J, Zhu Z. Comparison between microwave ablation and lobectomy for stage I non-small cell lung cancer: a propensity score analysis. Int J Hyperthermia 2018;34:1329-36. [Crossref] [PubMed]
- Yadav SP, Yadav S. Image fusion using hybrid methods in multimodality medical images. Med Biol Eng Comput 2020;58:669-87. [Crossref] [PubMed]
- Henschke CI, Yankelevitz DF, Libby DM, Pasmantier MW, Smith JP, Miettinen OS. Survival of patients with stage I lung cancer detected on CT screening. N Engl J Med 2006;355:1763-71. [Crossref] [PubMed]
- Hu B, Ren W, Feng Z, Li M, Li X, Han R, Peng Z. Correlation between CT imaging characteristics and pathological diagnosis for subcentimeter pulmonary nodules. Thorac Cancer 2022;13:1067-75. [Crossref] [PubMed]
- Park S, Lee SM, Choe J, Choi S, Kim S, Do KH, Seo JB. Differences in the prognostic implication of ground-glass opacity on CT according to pathological nodal status in lung cancers treated with lobectomy or pneumonectomy. Eur Radiol 2022;32:4405-13. [Crossref] [PubMed]
- Saeki Y, Kitazawa S, Yanagihara T, Kobayashi N, Kikuchi S, Goto Y, Ichimura H, Sato Y. Consolidation volume and integration of computed tomography values on three-dimensional computed tomography may predict pathological invasiveness in early lung adenocarcinoma. Surg Today 2021;51:1320-7. [Crossref] [PubMed]
- Cazzato RL, Battistuzzi JB, Catena V, Grasso RF, Zobel BB, Schena E, Buy X, Palussiere J. Cone-Beam Computed Tomography (CBCT) Versus CT in Lung Ablation Procedure: Which is Faster? Cardiovasc Intervent Radiol 2015;38:1231-6. [Crossref] [PubMed]
- Li XQ, Zhang Y, Huang DB, Zhang J, Zhang GS, Wen ZX, Li JH, Liu HL. Value of C-arm computed tomography in radiofrequency ablation of small lung lesions. Genet Mol Res 2014;13:6027-36. [Crossref] [PubMed]
- Dolmatch BL, Gurley JC, Baskin KM, Nikolic B, Lawson JH, Shenoy S, Saad TF, Davidson I, Baerlocher MO, Cohen EI, Dariushnia SR, Faintuch S, d'Othee BJ, Kinney TB, Midia M, Clifton J; Central Vein Work Group and the Technology Assessment Committee. Society of Interventional Radiology Reporting Standards for Thoracic Central Vein Obstruction: Endorsed by the American Society of Diagnostic and Interventional Nephrology (ASDIN), British Society of Interventional Radiology (BSIR), Canadian Interventional Radiology Association (CIRA), Heart Rhythm Society (HRS), Indian Society of Vascular and Interventional Radiology (ISVIR), Vascular Access Society of the Americas (VASA), and Vascular Access Society of Britain and Ireland (VASBI). J Vasc Access 2019;20:114-22.
- Vogl TJ, Nour-Eldin NA, Hammerstingl RM, Panahi B, Naguib NNN. Microwave Ablation (MWA): Basics, Technique and Results in Primary and Metastatic Liver Neoplasms - Review Article. Rofo 2017;189:1055-66. [Crossref] [PubMed]
- Zhang J, Xu K, Du K, Han X, Jiao D. Simultaneous percutaneous microwave ablation and biopsy for highly suspected malignant pulmonary nodules: a retrospective cohort study. Quant Imaging Med Surg 2023;13:7214-24. [Crossref] [PubMed]
- Suzuki K, Watanabe SI, Wakabayashi M, Saji H, Aokage K, Moriya Y, Yoshino I, Tsuboi M, Nakamura S, Nakamura K, Mitsudomi T, Asamura HWest Japan Oncology Group and Japan Clinical Oncology Group. A single-arm study of sublobar resection for ground-glass opacity dominant peripheral lung cancer. J Thorac Cardiovasc Surg 2022;163:289-301.e2. [Crossref] [PubMed]
- Austin JH, Müller NL, Friedman PJ, Hansell DM, Naidich DP, Remy-Jardin M, Webb WR, Zerhouni EA. Glossary of terms for CT of the lungs: recommendations of the Nomenclature Committee of the Fleischner Society. Radiology 1996;200:327-31. [Crossref] [PubMed]
- Bankier AA, MacMahon H, Colby T, Gevenois PA, Goo JM, Leung ANC, Lynch DA, Schaefer-Prokop CM, Tomiyama N, Travis WD, Verschakelen JA, White CS, Naidich DP. Fleischner Society: Glossary of Terms for Thoracic Imaging. Radiology 2024;310:e232558. [Crossref] [PubMed]
- Kim C. Understanding the nuances of microwave ablation for more accurate post-treatment assessment. Future Oncol 2018;14:1755-64. [Crossref] [PubMed]
- Ahmed M, Solbiati L, Brace CL, Breen DJ, Callstrom MR, Charboneau JW, et al. Image-guided tumor ablation: standardization of terminology and reporting criteria--a 10-year update. Radiology 2014;273:241-60. [Crossref] [PubMed]
- Kodama H, Ueshima E, Howk K, Lee SW, Erinjeri JP, Solomon SB, Srimathveeravalli G. Temporal evaluation of the microwave ablation zone and comparison of CT and gross sizes during the first month post-ablation in swine lung. Diagn Interv Imaging 2019;100:279-85. [Crossref] [PubMed]
- Kurilova I, Gonzalez-Aguirre A, Beets-Tan RG, Erinjeri J, Petre EN, Gonen M, Bains M, Kemeny NE, Solomon SB, Sofocleous CT. Microwave Ablation in the Management of Colorectal Cancer Pulmonary Metastases. Cardiovasc Intervent Radiol 2018;41:1530-44. [Crossref] [PubMed]
- Du K, Liu Y, Wu K, Sun Z, Han X, Jiao D. Percutaneous microwave ablation for lung tumors: a retrospective case-control study of conventional CT and C-arm CT guidance. Quant Imaging Med Surg 2023;13:5737-47. [Crossref] [PubMed]