Characteristics of subjective olfactory dysfunction and imaging features in patients with traumatic olfactory disorders: a retrospective case-control study
Introduction
Olfactory function is crucial for human survival (1), and thus olfactory dysfunction directly leads to a decline in quality of life (2), emotional disorders, and even depression (3) and is associated with higher mortality rates in older adult patients (4). The main causes of olfactory dysfunction include nasal-sinus inflammatory diseases, head trauma, upper respiratory tract infections, and neurodegenerative diseases (5). Traumatic olfactory dysfunction, in particular, is the most challenging to diagnose and treat and significantly differs from other types of olfactory dysfunction in several aspects, including a complex pathology, lack of treatment methods, poor prognosis, and ramifications in legal proceedings (6). Reports indicate that the incidence of olfactory dysfunction in patients with head trauma is particularly high, with the incidence rates in patients with mild and severe traumatic brain injury being approximately 20–44% (7) and 4–61% (8), respectively. Interestingly, studies have shown that the incidence of olfactory dysfunction in patients with traumatic olfactory dysfunction also depends on the cause of head trauma, with higher rates being observed in patients injured by assault or car accidents compared to those injured by other accidents (9). From a psychological perspective, this phenomenon might be explained by the impact of mental trauma on subjective olfactory function, but more commonly, patients might feign olfactory dysfunction to obtain higher compensation in legal cases. Therefore, the diagnosis of traumatic olfactory dysfunction in clinical settings is particularly important. Current objective diagnoses of traumatic olfactory dysfunction mainly rely on olfactory evoked potentials (10), but there are no internationally standardized diagnostic criteria for olfactory evoked potentials, and the required equipment is expensive and not widely used in clinical practice. Given the limitations of current diagnostic methods for traumatic anosmia, alternative approaches are urgently required. Magnetic resonance imaging (MRI) is the most sensitive technique available for observing brain tissue injury and recovery in cases of head trauma. Previous studies have demonstrated that patients with traumatic anosmia exhibit a reduction in both the volume (11,12) and functionality of specific brain regions compared to healthy controls, as revealed through structural and functional MRI. However, other olfactory disorders, such as those caused by upper respiratory tract infections or idiopathic factors (13,14), can exhibit similar imaging characteristics. The evidence to fully explain why traumatic olfactory dysfunction typically results in more severe impairment and worse prognoses compared to other types of olfactory disorders is currently insufficient. To address this, our study innovatively employed a control group consisting of patients with other olfactory disorders rather than the healthy volunteers typically used in traditional olfactory research. We included the internationally used “Sniffin’ Sticks” olfactory psychophysical test to analyze the characteristics of subjective olfactory dysfunction in patients with traumatic olfactory dysfunction and employed voxel-based morphometry (VBM) to determine the neuroimaging structural characteristics of these patients. The overarching aim was to gather more evidence concerning the diagnosis of traumatic olfactory dysfunction. We present this article in accordance with the STROBE reporting checklist (available at https://qims.amegroups.com/article/view/10.21037/qims-24-1185/rc).
Methods
Study participants
A retrospective case-control study was conducted, with data being collected from all patients with olfactory dysfunction treated at the Olfactory Disorder Clinic of Beijing Anzhen Hospital affiliated with Capital Medical University between 2016 and 2020. After detailed olfactory function-related examinations, patients were diagnosed by a clinician with more than 20 years of experience in the diagnosis and treatment of olfactory dysfunction. The olfactory function-related examinations included medical history inquiry, nasal endoscopy, nasal acoustic reflex, nasal resistance measurement, the T&T subjective olfactory function test, the Sniffin’ Sticks subjective olfactory function test, olfactory evoked potential examination, and olfactory pathway MRI.
The inclusion criteria for participants were as follows: (I) adolescents and adults aged 12–65 years according to international age classification standards; (II) trauma, upper respiratory tract infection, nasal sinus inflammation, and idiopathic olfactory dysfunction as causes of olfactory dysfunction; and (III) head trauma within 1 year for those with traumatic olfactory dysfunction. Meanwhile, the exclusion criteria were as follows: (I) neurological diseases such as stroke or Alzheimer disease that could affect brain tissue volume; (II) metal implants in the body that prevented MRI; (III) inability to cooperate with the study due to severe mental illness unable; and (IV) left handedness (12,15). The study was conducted in accordance with the Declaration of Helsinki (as revised in 2013). The study was approved by the Ethics Committee of Beijing Anzhen Hospital, Capital Medical University (No. 2018087X), and individual consent for this retrospective analysis was waived.
Subjective olfactory function test
The subjective olfactory function test method used in this study was the Sniffin’ Sticks olfactory function test (Burghart Messtechnik GmbH, Holm, Germany) (16). The Sniffin’ Sticks olfactory function test consists of three subtests: odor threshold test (OT), odor discrimination test (OD), and odor identification test (OI). In this test, the odors are stored in the cores of the sniffing sticks, and during the test, the pen cap is opened and placed about 3 cm below the nostrils for the participant to sniff. In the OT, 16 different concentration gradients of n-butanol odor are set. The participant is initially given a set of sniffing sticks with the lowest concentration, one containing n-butanol and two blank controls, and asked to identify the one with the odor. If incorrect, the concentration is increased until correct and then tested in the reverse direction until incorrect. This process is repeated seven times, and the average of the last four correct responses is recorded. In the OD, the participant is given a set of sniffing sticks, one with the target odor and two with the same interfering odor, and asked to identify the target odor after sniffing all three sticks, for a total of 16 sets. In the OI, the participant is given a sniffing stick with the target odor and asked to identify the target odor from four pictorial options corresponding to the odor, for a total of 16 sets. The final score of the Sniffin’ Sticks olfactory function test is the sum of the three subtests, which ranges from 1 to 48 points (17). Based on the final score, participants can be classified as having normal olfaction (≥30.5 points), hyposmia (≥16.5 points), or functional anosmia (<16.5 points).
Olfactory pathway MRI scanning
Olfactory pathway MRI was performed using a Philips 3-T scanner with a 32-channel phased array head coil for whole-brain scanning (Philips Healthcare, Best, the Netherlands). The scanning pulse sequence to obtain sagittal images was a 3D fast spin-echo T1-weighted sequence. The scanning parameters were as follows: voxel size, 0.98 mm × 0.98 mm × 1 mm; repetition time (TR), 6.68 ms; echo time, (TE) 3.15 ms; flip angle, 8°; number of slices, 184; slice thickness, 1 mm; and field of view (FOV), 256 mm.
MRI data processing and analysis
In this study, a blind method was used, and all data collection during the MRI scan was performed by the same MRI operator (the operator was unaware of the patient grouping). MATLAB software version 2013b (MathWorks, Natick, MA, USA) was used to run the functional MRI analysis program, SPM8 (Wellcome Trust Centre for Neuroimaging, Institute of Neurology, University College London, UK; http://www.fil.ion.ucl.ac.uk/spm) The “VBM8” package was used to perform VBM analysis on T1-weighted images of 101 patients with olfactory dysfunction (18). After confirmation of good image quality and a lack of artifacts, the images were segmented into gray-matter (GM), white-matter (WM), and cerebrospinal fluid (CSF) probability maps. Spatial normalization was then performed using DARTEL (diffeomorphic anatomical registration using exponentiated lie algebra) registration, with each patient’s GM, WM, and CSF probability maps being aligned to a standard brain template developed by the Montreal Neurological Institute (MNI) (18). Modulation and smoothing (6 mm full width at half maximum) were then applied to obtain the final GM volume (GMV) and WM volume (WMV) maps.
A two-sample t-test was performed with SPM12 to compare the GMV and WMV between the trauma and control groups, with sex and age being the covariates and the significance level set at uncorrected voxel-level P<0.001 (19). Multiple-comparison correction was performed on the significant statistical maps via xjView software (http://alivelearn.net/xjview) with family-wise error (FWE) correction (20) and a cluster-level significance of PFWE<0.05.
Multiple regression analysis was conducted using SPM12 to assess the correlation between GMV or WMV and subjective olfactory function scores (total score and three subtest scores) for the 101 patients with olfactory dysfunction, with the significance level set at P<0.0001 (19). RESTplus software was used to implement two multiple-comparison correction methods, FWE correction and AlphaSim correction (21), with a cluster-level significance of PAlphaSim<0.05. Clusters significant for both corrections were considered strongly correlated, those significant only with the AlphaSim correction were considered weakly correlated, and those significant for neither were considered noncorrelated.
The results were finally reported using xjView software and included (I) cluster size (number of voxels); (II) peak coordinates; (III) peak statistical values (T values); and (IV) brain region names according to the Automated Anatomical Labeling (AAL) template (22).
Statistical analysis
Data entry, organization, and analysis were performed using SPSS 20 (IBM Corp., Armonk, NY, USA). This included analyses of clinical characteristics, subjective olfactory function test results, and differences in brain GMV and WMV between the trauma and control groups. Normally distributed quantitative data are presented as the mean ± standard deviation, and categorical data are expressed as frequency (percentage). Initially, all data were subjected to the Kolmogorov-Smirnov test to determine conformity to a Gaussian distribution. The Mann-Whitney test was used for nonnormally distributed data, while the test was used for normally distributed data, with a significance level set at two-tailed values of P<0.05. Pearson correlation coefficients were used for correlation analyses between groups, and GraphPad Prism 7.0 was utilized for plotting. According to the calculation method of mean comparison between two independent samples, the total volume of GMV and WMV in the trauma group was 1,103.67±105.17 cm3 in the pre-experiment while that of the control group was 1,169.33±108.67 cm3 (α=0.05; β=0.2), and the proportion of lost to follow-up was 0%. Thus, the required minimum sample size of each group was 42 cases.
Results
Clinical data and subjective olfactory function
A total of 101 patients with olfactory dysfunction were included in this part of the study and placed in the trauma group or control group. The trauma group included 56 patients with traumatic olfactory dysfunction (mean age, 41.55±11.77 years; 36 males and 20 females). The control group included 45 patients with other types of olfactory dysfunction (mean age, 38.87±14.37 years; 19 males and 26 females). There was no significant difference in age distribution between the trauma and control groups (P=0.285). The trauma group had a significantly higher proportion of male patients compared to the control group (P=0.030). The trauma group had significantly lower OT, OD, OI, and total Sniffin’ Sticks test scores compared to the control group (P<0.05; Table 1, Figure 1).
Table 1
Demographics | Total patients (n=101) | Trauma (n=56) | Control (n=45) | P value |
---|---|---|---|---|
Age (years)† | 40.36±12.99 | 41.55±11.77 | 38.87±14.37 | 0.285 |
Male‡ | 55 (54.46) | 36 (64.29) | 19 (42.22) | 0.030 |
OT score§ | 1.35±1.33 | 1.04±0.33 | 1.67±1.42 | <0.001 |
OD score§ | 4.09±3.37 | 2.93±2.25 | 5.42±3.66 | <0.001 |
OI score§ | 4.57±3.51 | 2.77±1.94 | 6.71±3.55 | <0.001 |
TDI score§ | 10.02±7.37 | 6.74±3.91 | 13.81±7.63 | <0.001 |
Data are presented as mean ± SD or n (%). †, analysis was performed with the independent samples t-test. ‡, analysis was performed with the χ2 test. §, analysis was performed with the Mann-Whitney test. Two-tailed values of P<0.05 were considered statistically significant. OT, odor threshold test; OD, odor discrimination test; OI, odor identification test; TDI, total score of the Sniffin’ Sticks olfactory function test; SD, standard deviation.
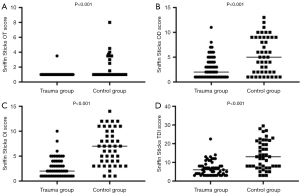
GMV
Compared to the control group, the trauma group had significantly GMV in the bilateral orbitofrontal cortex, bilateral anterior cingulate gyrus, bilateral cingulate gyrus, bilateral rectus gyrus, bilateral olfactory cortex, right superior frontal gyrus, bilateral insula, bilateral caudate nucleus, and bilateral thalamus (Table 2, Figure 2).
Table 2
Cluster size | Peak coordinates (MNI) | Peak statistical values (T values) | Brain region (AAL) | |||
---|---|---|---|---|---|---|
X | Y | Z | ||||
GM | 12,025 | 3 | 37.5 | −12 | 8.2388 | Orbital gyrus, middle orbital gyrus, anterior cingulate gyrus, paracingulate gyrus, rectus gyrus, olfactory cortex, superior orbital gyrus, insula, caudate nucleus, and thalamus |
685 | 4.5 | −16.5 | 1.5 | 4.4698 | ||
WM | 2,395 | −24 | 36 | −7.5 | 4.7875 | Left orbital gyrus, left rectus gyrus, left anterior cingulate gyrus, left paracingulate gyrus, left insula, right orbital gyrus, right rectus gyrus, right anterior cingulate gyrus, right paracingulate gyrus, right insula, and right caudate nucleus |
4,282 | 10.5 | 37.5 | −9 | 5.9876 |
P<0.001, cluster-level PFWE<0.05. The percentage represents the proportion of voxels within each cluster in a given brain region when the cluster spans multiple regions. GMV, gray-matter volume; WMV, white-matter volume; MNI, Montreal Neurological Institute; AAL, Automated Anatomical Labeling; GM, gray-matter; WM, white-matter; FWE, family-wise error.
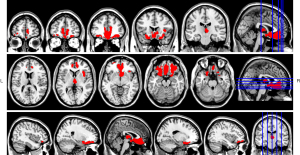
Correlation between GMV/WMV and subjective olfactory function
In the 101 patients with olfactory dysfunction, the OI scores were strongly positively correlated with GMB, with results passing both multiple comparison corrections. The OT and total Sniffin’ Sticks test scores were weakly positively correlated with GMV, passing only AlphaSim correction. The total score of the Sniffin’ Sticks olfactory function test was weakly positively correlated with WMV, passing only the AlphaSim correction. Other comparisons showed no correlation (Table 3). The brain regions that showed a strong correlation with OI values included the bilateral orbital middle frontal gyrus, bilateral orbital superior frontal gyrus, bilateral superior temporal gyrus, bilateral olfactory cortex, and bilateral caudate nucleus (Figure 3).
Table 3
FWE | AlphaSim | Correlation | |
---|---|---|---|
GM and OT | × | √ | Weak correlation |
WM and OT | × | × | No correlation |
GM and OD | × | × | No correlation |
WM and OD | × | × | No correlation |
GM and OI | √ | √ | Strong correlation |
WM and OI | × | × | No correlation |
GM and TDI | × | √ | Weak correlation |
WM and TDI | × | √ | Weak correlation |
√, cluster-level significance <0.05 (PFWE and PAlphaSim); ×, cluster-level significance >0.05 (PFWE and PAlphaSim). GMV, gray-matter volume; WMV, white-matter volume; FWE, family-wise error; GM, gray-matter; OT, odor threshold test; WM, white-matter; OD, odor discrimination test; OI, odor identification test; TDI, total Sniffin’ Sticks score.
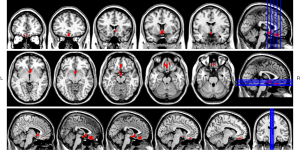
The brain regions showing a significant positive correlation between OI scores and GMV in the 101 patients with olfactory dysfunction overlapped with the regions in the trauma group that exhibited significantly reduced GMV compared to the control group. These overlapping regions include the bilateral middle orbital gyrus, right superior orbital gyrus, bilateral straight gyrus, bilateral olfactory cortex, and bilateral caudate nucleus (Figures 4,5).
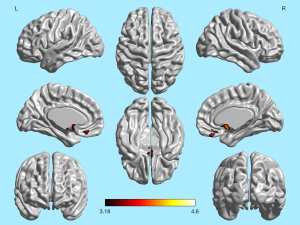
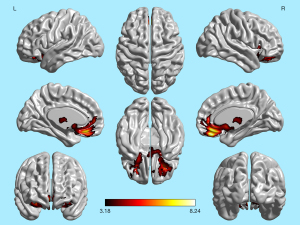
Discussion
Subjective olfactory function can qualitatively classify the type of olfactory dysfunction and quantify the degree of olfactory function loss (23). Previous studies have identified two main patterns of subjective olfactory function impairment in traumatic olfactory dysfunction: a pattern with impaired olfactory threshold function but preserved olfactory identification function, and another with significant declines in both olfactory threshold and olfactory identification test scores. The first pattern aligns with other types of olfactory dysfunction such as postinfection or inflammatory olfactory dysfunction, indicative of peripheral olfactory nerve damage, while the second pattern suggests central olfactory damage (24).
The Sniffin’ Sticks olfactory function test, used widely across the world, includes OT, OD, and OI, with strong qualitative and quantitative advantages (16). In our study, all of the Sniffin’ Sticks test scores were significantly lower in the trauma group compared to the control group, with a high proportion of functional anosmia in the trauma group (98.21%) compared to the control group (62.22%). Extensive research indicates that traumatic olfactory dysfunction typically manifests as anosmia (25) due to the complex nerve damage patterns in patients with this condition. Head trauma causes horizontal displacement of brain tissue within the anterior cranial fossa, leading to shearing forces that sever olfactory nerves at the cribriform plate, resulting in bilateral olfactory function loss (26). Trauma can also cause diffuse damage to the olfactory bulb, primary and higher olfactory centers, and WM connections between these regions. Acute posttraumatic damage to olfactory-related centers manifests as contusions, hemorrhage, and hematomas, with the nonacute phases potentially exhibiting localized liquefactive necrosis. Additionally, the olfactory bulb, located below the frontal lobe and fixed to the cribriform plate, is susceptible to secondary ischemic effects due to elevated intracranial pressure from brain edema (27).
In previous studies, the subjective olfactory function test scores (OT, OD, and OI) in patients with traumatic olfactory dysfunction have been reported to be significantly lower than those caused by other etiologies (28), but these differences are insufficient for etiological diagnosis (29). The development of neuroimaging techniques has provided more tools for studying traumatic olfactory dysfunction, enabling precise localization and quantification of brain damage in these patients (11).
Neuronal tissue undergoes degeneration and necrosis following head trauma (30), resulting in a significant volume reduction compared to that in healthy individuals (31). However, the olfactory nervous system possesses a strong regenerative capacity, which is particularly evident within 2–4 months after trauma (32) and stabilizes at 6–12 months (33). Consequently, for this study, we recruited patients with traumatic olfactory dysfunction within 1 year of injury to analyze olfactory function and imaging characteristics.
It is worth noting that other types of olfactory dysfunction (such as inflammatory, idiopathic, and postinfection) also exhibit reductions in GMV and WMV in olfactory-related brain regions (13,34). This volume reduction is attributed to the persistent deprivation of olfactory signal input (14,35). We hypothesize that this “bottom-up” neural reorganization process significantly differs from the direct neural damage observed in traumatic olfactory dysfunction. For the same reason, other pathologic mechanisms that may lead to brain volume decline, such as depression secondary to olfactory dysfunction, are also treated as confounders. These distinct neurostructural imaging characteristics can aid in the clinical diagnosis and assessment of traumatic olfactory dysfunction. Therefore, this study did not include individuals with normal olfactory function as controls but instead compared the subjective olfactory function and neurostructural imaging differences between patients with traumatic olfactory dysfunction and those with other types. This approach aimed to precisely define the unique subjective olfactory function and imaging characteristics resulting from direct damage to olfactory-related brain regions in traumatic olfactory dysfunction. The clinical implications of these findings are as follows: (I) otolaryngologists should monitor olfactory function closely and intervene if injury to these areas is observed in the MRI examinations of patients with head trauma, as olfactory symptoms are often overlooked by these patients. (II) For forensic purposes, brain injury in these regions may provide diagnostic evidence for traumatic olfactory dysfunction.
We further found that the trauma group had significantly reduced GMV and WMV in specific brain regions compared to the control group. The orbitofrontal cortex, part of the secondary olfactory center, receives olfactory signals from the piriform cortex, encoding and integrating olfactory signals with taste and visual signals (36,37). The anterior cingulate gyrus, which is part of the secondary olfactory center and located anatomically in the medial prefrontal cortex, receives input from the orbitofrontal cortex and is associated with olfactory function (38,39). Previous MRI studies have shown that damage to the olfactory bulb, olfactory tract, and olfactory cortex in patients with head trauma and hyposmia indicates severe olfactory dysfunction (40-42). The insular cortex is involved in olfactory signal processing, with its thickness being significantly correlated with composite olfactory scores (43). Our findings additionally included significantly reduced GMV and WMV in the bilateral rectus gyrus of the trauma group compared to those in the control group. Curiously, a previous study found that the volume of the bilateral rectus gyrus in perfumers was significantly increased compared to that in normal individuals (44), suggesting it plays a role in olfactory signal processing. Our study further confirms the prominent contribution of these brain regions in maintaining olfactory function.
Certain limitations of this study should be noted. Most importantly, we did not analyze the olfactory bulb, a critical olfactory-related brain region, due to the inability of VBM technology to register this area into a standard brain template. Future studies will address this by manually delineating and calculating the volume of the olfactory bulb as a region of interest.
Conclusions
Compared to other types of olfactory dysfunction, patients with traumatic olfactory dysfunction have significantly poorer subjective olfactory functions and more severe damage to olfactory-related brain regions, including the bilateral orbitofrontal cortex, anterior cingulate gyrus, cingulate gyrus, rectus gyrus, insula, and olfactory cortex. OI scores better reflect the function of these brain regions. The olfactory nerve damage in these brain regions can be used as a basis for diagnosing traumatic olfactory disorders.
Acknowledgments
Funding: This study was supported by
Footnote
Reporting Checklist: The authors have completed the STROBE reporting checklist. Available at https://qims.amegroups.com/article/view/10.21037/qims-24-1185/rc
Conflicts of Interest: All authors have completed the ICMJE uniform disclosure form (available at https://qims.amegroups.com/article/view/10.21037/qims-24-1185/coif). The authors have no conflicts of interest to declare.
Ethical Statement: The authors are accountable for all aspects of the work in ensuring that questions related to the accuracy or integrity of any part of the work are appropriately investigated and resolved. The study was conducted in accordance with the Declaration of Helsinki (as revised in 2013). The study was approved by the Ethics Committee of Beijing Anzhen Hospital, Capital Medical University (No. 2018087X), and individual consent for this retrospective analysis was waived.
Open Access Statement: This is an Open Access article distributed in accordance with the Creative Commons Attribution-NonCommercial-NoDerivs 4.0 International License (CC BY-NC-ND 4.0), which permits the non-commercial replication and distribution of the article with the strict proviso that no changes or edits are made and the original work is properly cited (including links to both the formal publication through the relevant DOI and the license). See: https://creativecommons.org/licenses/by-nc-nd/4.0/.
References
- Philpott CM, Bennett A, Murty GE. A brief history of olfaction and olfactometry. J Laryngol Otol 2008;122:657-62. [Crossref] [PubMed]
- Simopoulos E, Katotomichelakis M, Gouveris H, Tripsianis G, Livaditis M, Danielides V. Olfaction-associated quality of life in chronic rhinosinusitis: adaptation and validation of an olfaction-specific questionnaire. Laryngoscope 2012;122:1450-4. [Crossref] [PubMed]
- Jia Y, Bai S. A study on the quality of life and mental health of sinusitis patients with olfactory disorders. Lin Chuang Er Bi Yan Hou Tou Jing Wai Ke Za Zhi 2020;34:149-52. [Crossref] [PubMed]
- Chen H, Shrestha S, Huang X, Jain S, Guo X, Tranah GJ, Garcia ME, Satterfield S, Phillips C, Harris TB. Olfaction and incident Parkinson disease in US white and black older adults. Neurology 2017;89:1441-7. [Crossref] [PubMed]
- Boesveldt S, Postma EM, Boak D, Welge-Luessen A, Schöpf V, Mainland JD, Martens J, Ngai J, Duffy VB. Anosmia-A Clinical Review. Chem Senses 2017;42:513-23. [Crossref] [PubMed]
- Costanzo RM, Miwa T. Posttraumatic olfactory loss. Adv Otorhinolaryngol 2006;63:99-107. [Crossref] [PubMed]
- Sigurdardottir S, Jerstad T, Andelic N, Roe C, Schanke AK. Olfactory dysfunction, gambling task performance and intracranial lesions after traumatic brain injury. Neuropsychology 2010;24:504-13. [Crossref] [PubMed]
- Green P, Rohling ML, Iverson GL, Gervais RO. Relationships between olfactory discrimination and head injury severity. Brain Inj 2003;17:479-96. [Crossref] [PubMed]
- Frasnelli J, Laguë-Beauvais M, LeBlanc J, Alturki AY, Champoux MC, Couturier C, Anderson K, Lamoureux J, Marcoux J, Tinawi S, Dagher J, Maleki M, Feyz M, de Guise E. Olfactory function in acute traumatic brain injury. Clin Neurol Neurosurg 2016;140:68-72. [Crossref] [PubMed]
- Hummel T, Whitcroft KL, Andrews P, Altundag A, Cinghi C, Costanzo RM, et al. Position paper on olfactory dysfunction. Rhinol Suppl 2017;54:1-30. [Crossref] [PubMed]
- Han P, Winkler N, Hummel C, Hähner A, Gerber J, Hummel T. Alterations of Brain Gray Matter Density and Olfactory Bulb Volume in Patients with Olfactory Loss after Traumatic Brain Injury. J Neurotrauma 2018;35:2632-40. [Crossref] [PubMed]
- Gao X, Su B, Sun Z, Xu L, Wei Y, Wu D. Patterns of Gray and White Matter Volume Alterations in Patients With Post-Traumatic Anosmia: A Voxel-Based Morphometry Study. Front Neurol 2022;13:690760. [Crossref] [PubMed]
- Yao L, Pinto JM, Yi X, Li L, Peng P, Wei Y. Gray matter volume reduction of olfactory cortices in patients with idiopathic olfactory loss. Chem Senses 2014;39:755-60. [Crossref] [PubMed]
- Rombaux P, Duprez T, Hummel T. Olfactory bulb volume in the clinical assessment of olfactory dysfunction. Rhinology 2009;47:3-9.
- Arnold TC, You Y, Ding M, Zuo XN, de Araujo I, Li W. Functional Connectome Analyses Reveal the Human Olfactory Network Organization. eNeuro 2020;7. ENEURO. [Crossref] [PubMed]
- Kobal G, Hummel T, Sekinger B, Barz S, Roscher S, Wolf S. "Sniffin' sticks": screening of olfactory performance. Rhinology 1996;34:222-6.
- Rumeau C, Nguyen DT, Jankowski R. How to assess olfactory performance with the Sniffin' Sticks test Eur Ann Otorhinolaryngol Head Neck Dis 2016;133:203-6. [Crossref] [PubMed]
- Ashburner J, Friston KJ. Voxel-based morphometry--the methods. Neuroimage 2000;11:805-21. [Crossref] [PubMed]
- Woo CW, Krishnan A, Wager TD. Cluster-extent based thresholding in fMRI analyses: pitfalls and recommendations. Neuroimage 2014;91:412-9. [Crossref] [PubMed]
- Zhang H, Gomez LJ, Guilleminot J. Uncertainty quantification of TMS simulations considering MRI segmentation errors. J Neural Eng 2022; [Crossref]
- Li J, Gong H, Xu H, Ding Q, He N, Huang Y, Jin Y, Zhang C, Voon V, Sun B, Yan F, Zhan S. Abnormal Voxel-Wise Degree Centrality in Patients With Late-Life Depression: A Resting-State Functional Magnetic Resonance Imaging Study. Front Psychiatry 2019;10:1024. [Crossref] [PubMed]
- Tzourio-Mazoyer N, Landeau B, Papathanassiou D, Crivello F, Etard O, Delcroix N, Mazoyer B, Joliot M. Automated anatomical labeling of activations in SPM using a macroscopic anatomical parcellation of the MNI MRI single-subject brain. Neuroimage 2002;15:273-89. [Crossref] [PubMed]
- Fortin A, Lefebvre MB, Ptito M. Traumatic brain injury and olfactory deficits: the tale of two smell tests! Brain Inj 2010;24:27-33. [Crossref] [PubMed]
- Schofield PW, Moore TM, Gardner A. Traumatic brain injury and olfaction: a systematic review. Front Neurol 2014;5:5. [Crossref] [PubMed]
- Gudziol V, Hoenck I, Landis B, Podlesek D, Bayn M, Hummel T. The impact and prospect of traumatic brain injury on olfactory function: a cross-sectional and prospective study. Eur Arch Otorhinolaryngol 2014;271:1533-40. [Crossref] [PubMed]
- Mann NM. Head injury and anosmia. Conn Med 2003;67:545-7.
- Drareni K, Hummel T, Bensafi M, Serex CA, Hugentobler M, Rimmer J, Friedrich H, Voruz F, Terzic A, Landis BN. Olfactory and Gustatory Function in Patients With Different Types of Maxillofacial Trauma. Laryngoscope 2021;131:E331-7. [Crossref] [PubMed]
- Manan HA, Yahya N, Han P, Hummel T. A systematic review of olfactory-related brain structural changes in patients with congenital or acquired anosmia. Brain Struct Funct 2022;227:177-202. [Crossref] [PubMed]
- Temmel AF, Quint C, Schickinger-Fischer B, Klimek L, Stoller E, Hummel T. Characteristics of olfactory disorders in relation to major causes of olfactory loss. Arch Otolaryngol Head Neck Surg 2002;128:635-41. [Crossref] [PubMed]
- Rezaeyan A, Asadi S, Kamrava SK, Zare-Sadeghi A. Brain structural analysis in patients with post-traumatic anosmia: Voxel-based and surface-based morphometry. J Neuroradiol 2023;50:482-91. [Crossref] [PubMed]
- Trivedi MA, Ward MA, Hess TM, Gale SD, Dempsey RJ, Rowley HA, Johnson SC. Longitudinal changes in global brain volume between 79 and 409 days after traumatic brain injury: relationship with duration of coma. J Neurotrauma 2007;24:766-71. [Crossref] [PubMed]
- Wu AP, Davidson T. Posttraumatic anosmia secondary to central nervous system injury. Am J Rhinol 2008;22:606-7. [Crossref] [PubMed]
- Haxel BR, Grant L, Mackay-Sim A. Olfactory dysfunction after head injury. J Head Trauma Rehabil 2008;23:407-13. [Crossref] [PubMed]
- Han P, Whitcroft KL, Fischer J, Gerber J, Cuevas M, Andrews P, Hummel T. Olfactory brain gray matter volume reduction in patients with chronic rhinosinusitis. Int Forum Allergy Rhinol 2017;7:551-6. [Crossref] [PubMed]
- Mueller A, Rodewald A, Reden J, Gerber J, von Kummer R, Hummel T. Reduced olfactory bulb volume in post-traumatic and post-infectious olfactory dysfunction. Neuroreport 2005;16:475-8. [Crossref] [PubMed]
- Kjelvik G, Evensmoen HR, Brezova V, Håberg AK. The human brain representation of odor identification. J Neurophysiol 2012;108:645-57. [Crossref] [PubMed]
- Peter MG, Mårtensson G, Postma EM, Nordin LE, Westman E, Boesveldt S, Lundström JN. Morphological changes in secondary, but not primary, sensory cortex in individuals with life-long olfactory sensory deprivation. Neuroimage 2020;218:117005. [Crossref] [PubMed]
- Gottfried JA. Smell: central nervous processing. Adv Otorhinolaryngol 2006;63:44-69. [Crossref] [PubMed]
- Shepherd GM. Smell images and the flavour system in the human brain. Nature 2006;444:316-21. [Crossref] [PubMed]
- Bitter T, Brüderle J, Gudziol H, Burmeister HP, Gaser C, Guntinas-Lichius O. Gray and white matter reduction in hyposmic subjects--A voxel-based morphometry study. Brain Res 2010;1347:42-7. [Crossref] [PubMed]
- Weiss T, Soroka T, Gorodisky L, Shushan S, Snitz K, Weissgross R, Furman-Haran E, Dhollander T, Sobel N. Human Olfaction without Apparent Olfactory Bulbs. Neuron 2020;105:35-45.e5. [Crossref] [PubMed]
- Çullu N, Yeniçeri İÖ, Güney B, Özdemir MY, Koşar İ. Evaluation of olfactory bulbus volume and olfactory sulcus depth by 3 T MR. Surg Radiol Anat 2020;42:1113-8. [Crossref] [PubMed]
- Frasnelli J, Lundström JN, Boyle JA, Djordjevic J, Zatorre RJ, Jones-Gotman M. Neuroanatomical correlates of olfactory performance. Exp Brain Res 2010;201:1-11. [Crossref] [PubMed]
- Delon-Martin C, Plailly J, Fonlupt P, Veyrac A, Royet JP. Perfumers' expertise induces structural reorganization in olfactory brain regions. Neuroimage 2013;68:55-62. [Crossref] [PubMed]