Positron emission tomography-magnetic resonance imaging applications in pediatric musculoskeletal tumors
Introduction
There are numerous pediatric bone tumors, diagnosis requires a multimodality approach with radiography, computed tomography (CT), magnetic resonance imaging (MRI) and in recent times nuclear medicine has an important role in the diagnosis, surgical planning and follow-up monitoring of this pathology (1). Advances in genetic sequencing techniques have allowed for a more precise characterization of malignant musculoskeletal pathologies for therapeutic management and predicting the prognosis of these tumors.
The evaluation of pediatric bone tumors necessarily includes an MRI for local-regional staging of the tumor and whole-body (WB) bone scintigraphy and/or positron emission tomography (PET) to identify systemic disease.
The PET/MRI technique is a unique imaging diagnostic model because it combines, in a single scan, the information on biochemical and molecular processes provided by PET with the anatomical characterization of soft tissues, multiparametric, and functional information provided by MRI.
The development of PET/MRI hybrid systems began in the late 1990s. It is a technology that, after complex development and overcoming multiple technical limitations, managed to adapt PET detectors to the magnetic field action of 3T MRI (2).
Conventional PET detectors had to be physically separated from the MRI magnetic field using long cables. Recently, they have been replaced by semiconductor detectors, including avalanche photodiode detectors or silicon photomultipliers that are not sensitive to magnetic fields. Another challenge was to create suitable attenuation correction maps. In PET, positrons emitted by the radioisotopes injected into the patient interact with electrons in the body, producing gamma-ray photons. These photons travel in opposite directions and are detected by the PET scanner. However, as the photons traverse the body, they are absorbed or scattered by tissues, attenuating the signal, and potentially causing artifacts and errors in the final image. To correct this attenuation in PET/MRI hybrid systems, MRI is used to create attenuation maps. This process is more complex than in PET/CT because MR images do not directly provide information on tissue density in terms of gamma-ray attenuation. Instead, various techniques are used to infer tissue attenuation based on MR image characteristics. The technique most used is segmentation-based correction. This technique involves segmenting patient images into different tissue classes (e.g., background air, fat, muscle, lung tissue), based on their image based grey scales and assigning standard attenuation values to each region. Dedicated fast MRI sequences, mostly using the Dixon-technique providing fat and water images, are used to obtain images of tissue distribution and subsequent segmentation. Although less precise than CT or MR-based corrections, it is useful in situations where a detailed attenuation map is not available (Figure 1). Currently, the use of specialized MRI sequence techniques (i.e., ultra-short or zero-echo time sequences) has been implemented to generate even more precise attenuation correction maps, particularly in PET/MRI attenuation of the skull and brain. The MRI coils, both rigid and flexible, used in PET/MRI pose a challenge in creating attenuation correction maps because the former contains elements that alter these maps.
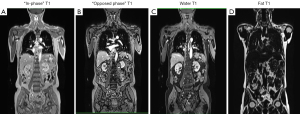
PET/MRI allows for the identification of the body’s and sectorial distribution of different metabolic tracers [18F-fluorodeoxyglucose (18F-FDG), 18F-fluorocholine, 18F-fluoride, 18F-fluorodopa, 68Ga-DOTA...]. Simultaneously, it correlates the distribution of these radiopharmaceuticals with the structural and functional characterization provided by MRI sequences, with 80% less exposure to ionizing radiation than conventional PET/CT scans, making it particularly suitable for pediatric patients who will require successive follow-ups due to their condition.
In PET/MRI, there are two acquisition modalities: sequential and simultaneous (3). Fully integrated simultaneous PET/MR imaging scanning is now possible in a simultaneous way with motion-free PET images and advance PET attenuation correction (Figure 2). The simultaneous acquisition of PET and MRI is especially useful in pediatric patients where cooperation may be limited, reducing acquisition time, and decreasing motion artifact, while obtaining advanced PET attenuation correction with magnetic resonance (MR) attenuation correction (AC) sequences.
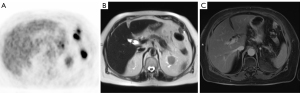
The aim of this study was to present the practical application of the PET/MRI technique and our clinical experience at our center in the diagnosis and follow-up of primary bone tumors and soft-tissue sarcomas in children. We present this article in accordance with the STROBE reporting checklist (available at https://qims.amegroups.com/article/view/10.21037/qims-24-621/rc).
Methods
Study design, participants and variables
We performed an observational retrospective study that included pediatric patients diagnosed with primary bone or soft-tissue sarcomas in the Pediatric Hematology and Oncology Unit at the HM Montepríncipe University Hospital, Boadilla del Monte, Madrid (Spain) from September 2017 to January 2023.
Patients underwent whole body 18F-FDG PET/MRI as a staging study and for follow-up evaluation for treatment response. At our institution, imaging data were acquired on a hybrid 3T Biograph mMR® MR-PET system (Siemens Healthcare, Erlangen, Germany) which allows for simultaneous acquisition. PET/MRI combining state-of-the-art 3T MRI with proven molecular imaging, fully integrated in one system.
It has a gantry of approximately 60 cm and an axial PET coverage of about 25 cm, providing sufficient space for pediatric patients and allowing WB PET studies using five to seven beds in most patients.
The PET/MRI application protocol in our center was applied following the main indications in pediatric musculoskeletal tumors.
The source of information was the electronic clinical history. The variables registered were age (in years), sex (male/female), and type of tumor (primary bone tumor/soft-tissue sarcoma). For those classified as primary bone tumor the followed variables were described: involvement of long bones of the extremities and affected bone, follow-up study (yes/no), metastases at the initial diagnosis (yes/no), metastases at follow up study (yes/no) and anatomical location of the metastases.
PET-MR imaging application protocol
Common indications for 18F-FDG PET/MR imaging in pediatric oncology include musculoskeletal tumors, providing prognostic information, and potentially for restaging and detection of relapse.
Primary malignant bone tumors
Malignant bone tumors account for 6% of all childhood neoplasms; typically, aggressive with a high tendency to metastasize, their treatment requires a multidisciplinary approach, combining surgery, chemotherapy (QT), and radiotherapy (RT) (1). Osteosarcoma (OS) is the most common primary malignant bone tumor in children, a rare malignancy accounting for only 2% of all cancers with a peak age between 10 and 14 years (4). It is an aggressive lesion with bone formation and typically presents with pulmonary metastases at diagnosis in 10–20% of cases (Figures 3-5).
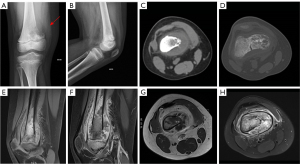
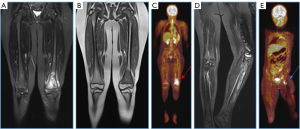
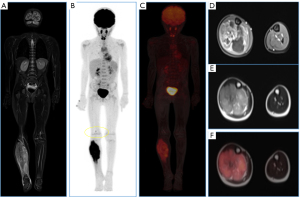
Ewing sarcoma, the second most common bone malignancy in the pediatric population, typically occurring around 10–15 years of age. It is centrally located, diaphyseal, lytic, with an onion-skin appearance, and highly aggressive. Most frequently locate in the femur, tibia, fibula, and pelvis. It mimics osteomyelitis and has a poor prognosis as metastasizes at diagnosis in 30% of cases, commonly involving the lungs, bones, adrenal glands, and brain. Aggressive periosteal reaction, Codman’s triangle, spiculated appearance, and often associated soft tissue mass are observed (5,6).
Malignant bone tumors often affect the metaphysis of long bones, especially around the knee and shoulder (1), and the distal femur, proximal tibia, and proximal humerus in the case of OS (5). However, they can also affect the pelvis, ribs, and vertebral bodies, especially in Ewing sarcoma. The presence of persistent localized pain and swelling warrants the performance of radiography (Figures 6-8).
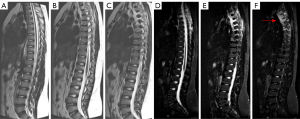
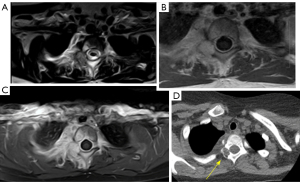
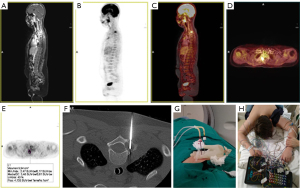
MRI is the preferred modality for the diagnosis and local staging. The utility of PET with 18F-FDG is not fully defined, although it presents greater diagnostic accuracy in detecting nodal and distant metastases and is more sensitive for bone metastases in Ewing sarcoma and OS, probably related to the predominant medullary infiltration in Ewing’s case and the osteoblastic metastases in OS. Combined PET/MRI images with 18F-FDG are likely to increase the overall accuracy of staging and response assessment. A significant disadvantage of PET/MR images is the limitation in detecting small pulmonary nodules (7).
PET allows differentiating perilesional edema and tumor infiltration and detects the presence of tumor thrombi because perilesional edema has a low or absent FDG uptake, while tumor thrombi have a high FDG uptake due to the presence of metabolically active tumor cells. Tumor thrombi are often associated with visible masses and may show invasion into adjacent structures.
The soft-tissue component of conventional OS is often hyperintense on T2 and has high uptake for 18F-FDG on PET, while the bone-forming component is usually hypointense on T2 and has low uptake for 18F-FDG. Descriptions of the extent of intra- and extra-osseous soft tissue, neurovascular bundle involvement, presence of intraarticular extension, and tumor thrombus are important as they may have implications for surgical treatment planning.
The main advantage of PET-MRI in Ewing sarcoma is that it is very useful as a guide for biopsy of hypermetabolic regions since it is a tumor with heterogeneous appearance on MRI. 18F-FDG PET/MRI can help evaluate tumor chemotherapy response based on a reduction of 18F-FDG uptake of more than 30%.
Soft-tissue sarcomas
Pediatric soft-tissue sarcomas constitute about 7% of all solid pediatric tumors. Rhabdomyosarcoma is the most common subtype (3), most frequently located in the head, neck, and genitourinary tract.
MRI is the preferred modality for diagnosis and local staging. Soft-tissue sarcomas have high uptake of 18F-FDG, and it is useful in detecting lymph node and bone metastases. Combined PET/MRI imaging with 18F-FDG, including diffusion weighted imaging (DWI), is likely to increase overall staging accuracy and response assessment (Figure 9), as it results in higher detection compared to MRI or PET alone (2).
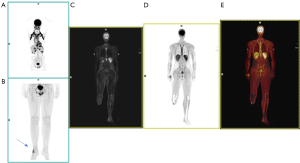
Langerhans cell histiocytosis (LCH)
LCH is a granulomatous disease in which dendritic cells proliferate abnormally, infiltrating various organs or tissues with significant variability in clinical presentation and biological behavior, thus mimicking different diseases. It can affect bones, skin, teeth, gingival tissue, ears, endocrine organs, lungs, liver, spleen, lymph nodes, and bone marrow. Bone involvement can be either unifocal or multifocal. The average age at diagnosis is 5–10 years. The most common site of involvement is flat bones (65–70%). Early lesions may appear highly aggressive (8).
Diagnostic imaging is of utmost importance. Lesions are visible on plain radiographs, CT scans, and MRI, thus initial evaluation with conventional radiography and bone scintigraphy (both with low specificity) has routinely been used as imaging modalities in the extension study of LCH, which is a crucial factor in defining treatment and prognosis. PET/MR imaging with 18F-FDG, including DWI, allows identification of active lesions and monitoring of therapeutic response in pediatric patients with LCH. Additionally, it has higher sensitivity for detecting marrow lesions compared to PET/CT and entails lower radiation exposure (Figure 10).
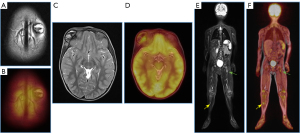
Patient preparation
The nuclear medicine physician explains in detail the study to the patient and parents/caregivers, who have been provided with information delivered verbally. Patients should be fasting for 4–6 h before 18F-FDG injection to decrease serum glucose level and to maintain a low insulin level. The serum glucose level is measured before the radiotracer administration. The glucose level should be below 200 mg/dL (11.1 mmol/L); the optimal level is below 140 mg/dL (7.8 mmol/L). The height and weight of the patient is measured on the day of the examination. The patient lying on a bed in a quiet, warm room for 18F-FDG injection and during the uptake phase. It is important that patients avoid talking, playing, exercising, or chewing before and after 18F-FDG is administered. Some small children required general anesthesia, they were previously evaluated by the anesthesiologist, and it was administered immediately before image acquisition.
Radiotracer
18F-FDG is the most common radiotracer used for PET imaging, with a half-life of approximately 110 min. FDG is an analog of glucose, which is taken up by cells via cell membrane glucose transporters and subsequently incorporated into the first step of the physiologic glycolytic pathway. The dose of 18F-FDG to be administered to patients can vary based on several factors, including patient weight, clinical indication, and institutional protocols. The administered activity should be the lowest possible dose that will produce diagnostic image quality. The administered activity follows the 2016 Update of the North American Consensus Guidelines for Pediatric Administered Radiopharmaceutical Activities and the 2016 EANM pediatric Dosage Card. The administered activity of 18F-FDG is 3.7–5.2 MBq/kg (0.10–0.14 mCi/kg) for a body PET/CT scan [with a minimum of 26 MBq (0.7 mCi)]. The administered dose is optimized according to the institutional policy and for PET/MRI systems. Our institutional guidelines protocol uses intravenous injection of 3 MBq/kg of 18F-FDG, which is lower than the standard dose, because the acquisition of MR sequences increases the PET image acquisition time, allowing for a significant reduction in the dose adjusted to the individual needs of the patient.
PET/MRI protocol
Considering the time to place the patient in the PET/MRI scanner and apply the surface coils, the PET scan starts 60 min after injection (9). The patient is lying decubitus supine on the table with arms close to the body. Specific mMR Tim radiofrequency coils (head and neck coil, spine coil and up to four body array coils) are placed under, on and around the patient’s body, respectively, covering the entire acquisition field. Imaging acquisition is performed caudally to cranially, typically starting from the feet up to the cranial vertex to avoid bladder image artifact (3) (Figures 11,12). The study usually starts with a basic module consisting of a WB PET acquisition accompanied by simultaneous WB MR imaging. In our protocol, PET acquisition time is usually 4 minutes per bed position and acquisition of MR sequence T2 Half Fourier Acquisition Single Shot Turbo Spin Echo (HASTE), and DWI axial MRI sequences with values b50, 500 y 1,000. Subsequently, intravenous gadolinium is administered and a volumetric gradient echo sequence with T1-weighted fat suppression (FS) is obtained (T1 VIBE). The different sequences are summarized in Table 1.
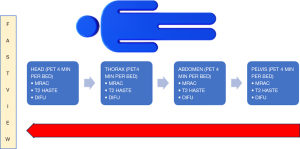
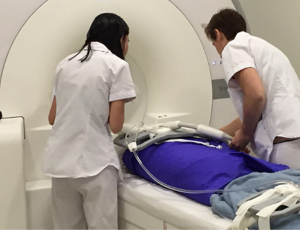
Table 1
Sequence (Siemens Biograph mMR) | Time | Plane | TR (ms) | TE (ms) | Matrix |
---|---|---|---|---|---|
WB PetAcquisition | 50:00 | – | – | – | – |
MRAC_CAIPI_HiRes (attenuation correction sequences) | 01:44 | – | – | – | – |
T2_haste_tra_p2_mbh_320 | 08:40 | Axial | 1,300 | 95 | 320×280 |
Ep2d_diff_stir_b50_800_tra (DWI) | 17:28 | Axial | 5,670 | 53 | 104×128 |
T2_tse_stir_cor (STIR) | 20:57 | Coronal | 7,070 | 53 | 154×320 |
T1_vibe_dixon_cor_p5_bh_320_iso | 03:12 | Coronal | 4.27 | 1.35 | 175×288 |
T1_vibe_dixon_tra_p3_bh_post | 02:31 | Axial | 4.57 | 1.34 | 176×288 |
PET/MR, positron emission tomography/magnetic resonance; MR, magnetic resonance; TR, repetition time; TE, echo time; WB, whole-body; HASTE, Half Fourier Acquisition Single Shot Turbo Spin Echo; tra, transverse/axial; DWI, diffusion weighted imaging; cor, coronal; STIR, short tau inversion recovery.
Interpretation of images
The interpretation of PET/MR studies is complex, as numerous MRI sequences and the PET study are available, thus a joint report is generated between a pediatric radiologist and a nuclear medicine physician, both specialists in pediatric pathology. Compared to adults, children exhibit different physiological and anatomical characteristics such as the presence of thymic tissue or physiological uptake of brown fat, as well as visualization of growth cartilage that must be considered to avoid incorrect interpretation (3,10,11) (Figure 13).
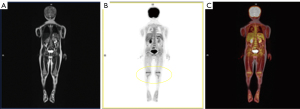
At our institution, radiological studies are reported by consensus among multidisciplinary teams consisting of a radiologist and a nuclear medicine physician. Complex cases are discussed in a multidisciplinary pediatric oncology committee that meets every Wednesday. In radiological reports of 18F-FDG PET/MRI, it is important to include the tumor size, presence or absence of metastatic disease, location of metastases, and changes in the size of index lesions for treatment monitoring (8).
Statistical analysis
Variables were described using percentages or medians and interquartile range (IQR). Since the inspection of the quantitative variables indicated a deviation from the normal distribution, the Kruskal-Wallis test for association was applied. To explore association between qualitative variables the Chi-squared test was performed. If the expected value in a cell was less than 5, Fisher’s exact test was performed.
Statistical significance was set at a two-tailed P value <0.05. The analysis was performed using the IBM SPSS Version 28.0 package.
Ethical considerations
The study was conducted in accordance with the Declaration of Helsinki (as revised in 2013). All the cases presented were part of routine clinical practice. Verbal informed consent was obtained from all patients or their legal guardians prior to the performance of the diagnostic tests.
Results
A total of 71 18F-FDG PET/MR studies were performed on 39 patients. Most of them (55%) were initial extension studies and (45%) were follow-up studies. Most of the patients studied were male (74.4%) with a median age of 15 years. The distribution of sex and age depending on the diagnosis and the initial finding of metastases can be seen in Table 2.
Table 2
Variables | Total | Primary bone tumors | Soft tissue tumors | LCH | Others | P value |
---|---|---|---|---|---|---|
No. of patients (%) | 39 (100.0) | 20 (51.3) | 8 (20.5) | 7 (17.9) | 4 (10.3) | |
Age (years), median [IQR] | 15 [8] | 16 [6] | 15 [9] | 11 [11] | 15.5 [10] | 0.205a |
Male, n (%) | 29 (74.4) | 15 (75.0) | 3 (37.5) | 7 (100.0) | 4 (100.0) | 0.183b |
Metastases at the initial diagnosis, n (%) | 5 (12.8) | 2 (10.0) | 3 (37.5) | 0 | 0 | 0.071b |
a, Kruskal-Wallis test; b, Chi-squared test. If the expected value in a cell was less than 5, Fisher’s exact test was performed. LCH, Langerhans cell histiocytosis; IQR, interquartile range.
Of the 39 cases, 51% were primary bone tumors (OS 28.2% and Ewing sarcoma 23.1%) while 18% were LCH and 21% soft tissue tumors and 10% other tumors (Table 2, Figure 14). There were no statistically significant differences in age or sex depending on the type of tumor diagnosed. Multifocal bone lesions were present in 7.6% of the cases. Among those with primary bone tumors, in 46.8% long bones of the extremities were involved, with the femur being the most affected one (25% of bone lesions and 20.5% of the total). Of the 71 studies, 5 (12.8%) showed metastases at the initial diagnosis, three presented lymph node involvement, one with local infiltration, and one with pulmonary metastasis. During follow-up, two cases with distant metastases were diagnosed. The different locations are summarized in Table 3.
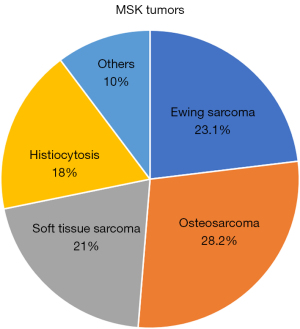
Table 3
Locations | Value (n=39) |
---|---|
Primary malignant bone tumors, n (%) | 32 (82.1) |
Spine | 4 (10.3) |
Skull | 5 (12.8) |
Rib | 3 (7.7) |
Femur | 8 (20.5) |
Humerus | 2 (5.1) |
Iliac | 2 (5.1) |
Hand | 1 (2.6) |
Fibula | 2 (5.1) |
Tibia | 2 (5.1) |
Multifocal | 3 (7.7) |
Soft tissue sarcomas, n (%) | 7 (17.9) |
Head and neck | 3 |
Arm | 1 |
Leg | 3 |
Discussion
The combined use of PET and MRI has represented a significant advancement in the evaluation of pediatric malignant neoplasms, with specific applications in musculoskeletal tumors showing clear benefits over PET/CT (12,13).
PET-MRI is superior to PET/CT in soft tissue assessment, combining tumor metabolism evaluation through PET and morphological, parametric, and functional assessment by MRI. The superior soft tissue contrast of MR is in general relevant for all types of soft tissue tumors regarding detection, delineation, characterization, and staging.
The reduction in ionizing radiation dose and the decrease in total examination time are notable advantages in pediatric patients. However, both MRI and PET have limited sensitivity for the detection of subcentimetric pulmonary nodules (14). Its sensitivity for detecting lung nodules is low due to the limited lung resolution of PET-MRI, thus requiring additional chest CT in the extension study to identify pulmonary metastases (7,12,13).
PET/MRI utility includes the characterization of the primary tumor, local-regional and distant staging, therapy planning, and post-treatment follow-up. It allows for WB evaluation in a single session, providing efficient staging and avoiding the need for the patient to undergo anesthesia twice. Additionally, estimation of tumor volume provides prognostic information.
Pediatric patients who underwent the described protocol at our center during the period of the study present an expected distribution of primary malignant bone tumors and soft tissue tumors. The characteristics of the described lesions are also in line with those described in the reviewed literature for their group of age.
The 18F-FDG PET/MRI has shown to increase diagnostic accuracy in primary bone and soft tissue tumors, as it allows for the comprehensive evaluation of their morpho-metabolic characteristics, locoregional, and distant involvement in a single study. This is particularly beneficial in the pediatric population as it avoids the need for multiple anesthesia procedures. It is also useful in surgical planning and post-treatment follow-up. The reduction in ionizing radiation dose and overall scanning time are notable advantages.
In heterogeneous tumors, PET is also useful for guiding biopsies towards areas of highest metabolic activity. Its main drawback is the low sensitivity for detecting pulmonary nodules; hence, chest CT remains essential in thoracic staging. Lymph node evaluation remains a limitation for WB MRI due to the lack of standardized apparent diffusion coefficient (ADC) values for distinguishing benign from malignant nodes, a limitation that PET-MR resolves.
Acknowledgments
We thank Lina Garcia Cañamaque, Chief of the PET/MRI Metabolic Service Center for their assistance with the acquisition of PET/MRI scans at the University Hospital HM Puerta del Sur at Móstoles (Madrid), and we thank Blanca Lopez Ibor, Chief of the Pediatric Hematology and Oncology Service at the HM Montepríncipe Hospital, who cares for children and adolescents.
Funding: None.
Footnote
Provenance and Peer Review: With the arrangement by the Guest Editors and the editorial office, this article has been reviewed by external peers.
Reporting Checklist: The authors have completed the STROBE reporting checklist. Available at: https://qims.amegroups.com/article/view/10.21037/qims-24-621/rc
Conflicts of Interest: All authors have completed the ICMJE uniform disclosure form (available at https://qims.amegroups.com/article/view/10.21037/qims-24-621/coif). The special issue “Advances in Diagnostic Musculoskeletal Imaging and Image-guided Therapy” was commissioned by the editorial office without any funding or sponsorship. J.M.V. served as the unpaid Guest Editor of the issue. The authors have no other conflicts of interest to declare.
Ethical Statement: The authors are accountable for all aspects of the work in ensuring that questions related to the accuracy or integrity of any part of the work are appropriately investigated and resolved. The study was conducted in accordance with the Declaration of Helsinki (as revised in 2013). All the cases presented were part of routine clinical practice. Verbal informed consent was obtained from all patients or their legal guardians prior to the performance of the diagnostic tests.
Open Access Statement: This is an Open Access article distributed in accordance with the Creative Commons Attribution-NonCommercial-NoDerivs 4.0 International License (CC BY-NC-ND 4.0), which permits the non-commercial replication and distribution of the article with the strict proviso that no changes or edits are made and the original work is properly cited (including links to both the formal publication through the relevant DOI and the license). See: https://creativecommons.org/licenses/by-nc-nd/4.0/.
References
- Nguyen JC, Baghdadi S, Pogoriler J, Guariento A, Rajapakse CS, Arkader A. Pediatric Osteosarcoma: Correlation of Imaging Findings with Histopathologic Features, Treatment, and Outcome. Radiographics 2022;42:1196-213. [Crossref] [PubMed]
- García Cañamaque L, Field CA, Furtado FS, Plaza DE. Las Heras I, Husseini JS, Balza R, Jarraya M, Catalano OA, Mitjavila Casanovas M. Contribution of positron emission tomography/magnetic resonance imaging in musculoskeletal malignancies. Q J Nucl Med Mol Imaging 2022;66:3-14. [Crossref] [PubMed]
- Kwatra NS, Lim R, Gee MS, States LJ, Vossough A, Lee EY. PET/MR Imaging: Current Updates on Pediatric Applications. Magn Reson Imaging Clin N Am 2019;27:387-407. [Crossref] [PubMed]
- Lee H, Wang A, Cheng R, Moran J, Al-Dasuqi K, Irshaid L, Maloney E, Porrino J. Update of pediatric bone tumors-notochordal tumors, chondrogenic tumors, and vascular tumors of the bone. Skeletal Radiol 2023;52:1101-17. [Crossref] [PubMed]
- Al-Dasuqi K, Cheng R, Moran J, Irshaid L, Maloney E, Porrino J. Update of pediatric bone tumors: osteogenic tumors and osteoclastic giant cell-rich tumors. Skeletal Radiol 2023;52:671-85. [Crossref] [PubMed]
- Murphey MD, Senchak LT, Mambalam PK, Logie CI, Klassen-Fischer MK, Kransdorf MJ. From the radiologic pathology archives: ewing sarcoma family of tumors: radiologic-pathologic correlation. Radiographics 2013;33:803-31. [Crossref] [PubMed]
- Manhas NS, Salehi S, Joyce P, Guermazi A, Ahmadzadehfar H, Gholamrezanezhad A. PET/Computed Tomography Scans and PET/MR Imaging in the Diagnosis and Management of Musculoskeletal Diseases. PET Clin 2020;15:535-45. [Crossref] [PubMed]
- Padwal J, Baratto L, Chakraborty A, Hawk K, Spunt S, Avedian R, Daldrup-Link HE. PET/MR of pediatric bone tumors: what the radiologist needs to know. Skeletal Radiol 2023;52:315-28. [Crossref] [PubMed]
- Antonio Catalano O. Clinical PET/MRI. 2023.
- Gatidis S, la Fougère C, Schaefer JF. Pediatric Oncologic Imaging: A Key Application of Combined PET/MRI. Rofo 2016;188:359-64. [Crossref] [PubMed]
- States LJ, Reid JR. Whole-Body PET/MRI Applications in Pediatric Oncology. AJR Am J Roentgenol 2020;215:713-25. [Crossref] [PubMed]
- Uslu-Beşli L, Atay Kapucu LÖ, Karadeniz C, Akdemir ÜÖ, Pinarli FG, Aydos U, Okur A, Kaya Z, Samanci C, Karabacak NI. Comparison of FDG PET/MRI and FDG PET/CT in Pediatric Oncology in Terms of Anatomic Correlation of FDG-positive Lesions. J Pediatr Hematol Oncol 2019;41:542-50. [Crossref] [PubMed]
- Schäfer JF, Gatidis S, Schmidt H, Gückel B, Bezrukov I, Pfannenberg CA, Reimold M, Ebinger M, Fuchs J, Claussen CD, Schwenzer NF. Simultaneous whole-body PET/MR imaging in comparison to PET/CT in pediatric oncology: initial results. Radiology 2014;273:220-31. [Crossref] [PubMed]
- Biondetti P, Vangel MG, Lahoud RM, Furtado FS, Rosen BR, Groshar D, Canamaque LG, Umutlu L, Zhang EW, Mahmood U, Digumarthy SR, Shepard JO, Catalano OA. PET/MRI assessment of lung nodules in primary abdominal malignancies: sensitivity and outcome analysis. Eur J Nucl Med Mol Imaging 2021;48:1976-86. [Crossref] [PubMed]