Assessing regional homogeneity and cognitive function alterations in pediatric brain tumor patients: a resting-state functional magnetic resonance imaging study
Introduction
Central nervous system (CNS) tumors have recently overtaken leukemia as the most common tumor in children aged 0–14 years, with a reported incidence of 5.96 per 100,000 children annually, presenting a significant challenge to pediatric health (1). Despite the high malignancy rate in pediatric CNS tumors, advancements in medical science and technology have significantly improved the 5-year survival rates, which currently range from 18% to as high as 93% (2). This improvement in survival rates underscores the growing need to assess and understand cognitive abilities in these children, an area in which research is still limited (3).
Resting-state functional magnetic resonance imaging (rs-fMRI), a noninvasive and nonionizing technique offering high spatial resolution, is instrumental in detecting activated brain regions in vivo through blood oxygenation level-dependent (BOLD) signals and has become integral in brain disease research (4,5). Among the analytical methods derived from rs-fMRI, regional homogeneity (ReHo) is notable (6). ReHo reflects spontaneous brain functional activity as a measurement of the synchrony of time series between a target voxel and its neighboring voxels via the Kendall coefficient concordance (KCC). It is particularly valuable for characterizing the local attributes of spontaneous brain activity. Variations in ReHo, either as an increase or decrease, can indicate alterations in the synchronization or coordination of local brain activity, which may potentially underpin the cognitive dysfunction in patients (7).
The fourth edition of Wechsler Intelligence Scale for Children (WISC-IV), a cornerstone in the assessment of children’s cognitive function, has been extensively used in clinical practice (8). The development of the fourth edition of this scale in 2003 (9) marked a significant update in its approach to cognitive assessment. The Chinese version of the WISC-IV was introduced to China in 2008, broadening its application in diverse cultural contexts. The WISC-IV is designed for children aged 6–16 years and comprises several indices: the Verbal Comprehension Index (VCI), Perceptual Reasoning Index (PRI), Working Memory Index (WMI), Processing Speed Index (PSI), and the Full-Scale Intelligence Quotient (FSIQ). These indices collectively offer a multidimensional evaluation of a child’s cognitive abilities, covering aspects including language, visual-spatial processing, logic, and speed of information processing.
Given the prevalence of cognitive impairments in children with brain tumors, it is plausible that alterations in ReHo are present in this population and may correlate with cognitive function. Our study leveraged the rs-fMRI and ReHo methodologies to clarify the potential pathophysiological mechanisms behind local brain functional activity changes and cognitive dysfunction in patients with pediatric brain tumor. Our goal is to provide a neuroimaging foundation for the clinical diagnosis and prognostic evaluation of these patients. We present this article in accordance with the STROBE reporting checklist (available at https://qims.amegroups.com/article/view/10.21037/qims-24-529/rc).
Methods
Participants
A total of 21 patients were recruited from September 2021 to June 2023 at the Tsinghua University Yuquan Hospital (Tsinghua University Integrated Traditional Chinese and Western Medicine Hospital). Age- and sex-matched healthy controls (HCs) were also enrolled.
MRI scans were conducted on all participants using a 3-T MRI scanner (Philips, Amsterdam, the Netherlands) equipped with a 32-channel head coil. Two models, the 3-T Ingenia and the 3-T Achieva (Philips), were used at the respective facilities of Tsinghua University and Tsinghua University Yuquan Hospital in Beijing, China.
This study adhered to the Declaration of Helsinki (as revised in 2013) and received approval from the Ethics Committee of Yuquan Hospital, Tsinghua University (No. 20220711). Informed consent was obtained from all participants’ guardians prior to participation.
Children diagnosed with brain tumors were recruited based on the following inclusion criteria: (I) age range between 6 to 16 years, (II) right-handedness, and (III) confirmed brain tumor diagnosis. The exclusion criteria for these patients were prior chemotherapy and/or radiotherapy, extensive metastasis, MRI contraindications, and inability to cooperate during MRI procedures.
Age- and sex-matched HCs were enrolled according to the following inclusion criteria: (I) age between 6 to 16 years, (II) right-handedness, and (III) absence of CNS-related diseases. Meanwhile, the exclusion criteria for HCs included psychiatric or neurological disorders, MRI contraindications, and recent long-term medication use.
Assessment of cognitive function
Cognitive functioning was evaluated using the WISC-IV. This scale is suitable for children aged 6 to 16 years and encompasses 10 core subtests, such as block building and vocabulary, along with 4 supplementary tests. It measures various cognitive abilities, yielding scores for the VCI, PRI, WMI, PSI, and FSIQ. The WISC-IV assessments were conducted in a controlled environment within the psychiatric ward of Tsinghua Yuquan hospital and were administered by two certified psychologists.
Image acquisition
Brain MRI scans were performed for all participants using 3-T scanners, which included T1-weighted image three-dimensional (T1WI 3D) imaging and rs-fMRI. The T1WI 3D imaging parameters were set as follows: 160 transverse slices, a voxel size of 1×1×1 mm3, a repetition time/echo time (TR/TE) of 8.1/3.7 ms, a matrix size of 256×256, a flip angle of 6°, and a field of view (FOV) of 256×256 mm.
For rs-fMRI, the parameters included a voxel size of 3.0×3.0×3.5 mm3, a flip angle of 90°, 41 transverse slices without interslice gaps, a slice thickness of 3 mm, 240 time points, a TR/TE of 2,620/30 ms, an FOV of 192×192 mm², and a matrix of 64×55. During scanning, participants were instructed to remain still with their eyes closed but to remain awake. Foam padding and soft earplugs were provided to minimize head motion and reduce noise exposure. For younger participants aged below 10 years, a guardian was present to ensure compliance and comfort during scanning.
rs-fMRI data processing
The rs-fMRI image data were preprocessed using the Data Processing and Analysis for Brain Imaging (DPABI; V8.1_240101; http://rfmri.org/dpabi) toolkit (10) which is based on Statistical Parametric Mapping 12 (SPM12; http://www.fil.ion.ucl.ac.uk/spm/). The preprocessing steps included (I) conversion of image data from Digital Imaging and Communications in Medicine (DICOM) format, (II) removal of the initial 10 time points to achieve steady-state magnetization, (III) slice timing correction, (IV) realignment to exclude participants with excessive head motion (more than 2.5 mm translation or 2.5° rotation), (V) spatial normalization of the motion-corrected data to the Montreal Neurological Institute space with resampling of voxels to 3 mm3, and (VI) regression of nuisance covariates (including Friston-24 head movement parameters, linear trends, and signals from cerebral white matter and cerebrospinal fluid), followed by band-pass filtering within the range of 0.01 to 0.08 Hz.
Tumor lesions were delineated using ITK-Snap software, with individual lesion masks subsequently merged into a composite mask, as illustrated in Figure 1. The mask was then applied to exclude tumor-affected areas from the ReHo analysis. The lesion outlining was uniformly performed by a single neurosurgeon (Z.Z.).
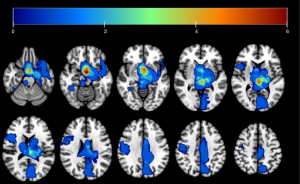
ReHo analysis
ReHo analysis was performed using the DPABI toolkit (6,10). The steps included were as follows: (I) calculation of the KCC for each voxel, with its time series and that of its 26 nearest neighbors being used (6); (II) normalization of ReHo maps, with the KCC value for each voxel being divided by the mean KCC value across the entire brain to minimize individual variations; and (III) spatial smoothing, with a Gaussian kernel of 4-mm full-width at half maximum (FWHM) used to reduce spatial noise and enhance the signal-to-noise ratio. The KCC formula was as follows.
Statistical analysis
Clinical data from both patients and HCs were analyzed using SPSS 26 (IBM Corp., Armonk, NY, USA). Age differences were evaluated using a nonparametric test, while chi-square tests were employed for gender distribution. All tests were two-tailed with a significance threshold set at P<0.05.
For ReHo analysis, a two-sample t-test was carried out on a voxel-by-voxel basis within a predefined gray-matter mask via DPABI. Covariates including age, gender, education level, and MRI scanner type were controlled in the analysis. DPABI software was used for thresholding statistical maps. We applied threshold-free cluster enhancement (TFCE) and familywise error correction (FWE) for multiple comparison corrections, with a significance level set at P<0.05. A total of 5,000 permutations were performed for each test.
To assess the clinical significance of our findings, Pearson correlation coefficients were calculated between significant ReHo values and clinical variables from the WISC-IV, including VCI, PRI, WMI, PSI, and FSIQ.
Results
Demographic information
This study included 21 pediatric patients diagnosed with brain tumors (11 females; mean age 9.47±2.82 years; age range, 6–15 years;) and 19 age- and sex-matched HCs (9 females; mean age 10.53±2.82; age range, 6–16 years). Statistical analysis revealed no significant differences in age, gender, or education levels between the two groups (P>0.05). Detailed demographic and clinical characteristics of both the patient and control groups are presented in Table 1.
Table 1
Item | HCs (n=19) | Tumor patients (n=21) | P value |
---|---|---|---|
Age (years) | 10.53±2.82 | 9.47±2.82 | 0.247 |
Gender (female/male) | 9/10 | 11/10 | 0.752 |
Education (years) | 4.37±2.64 | 3.21±2.78 | 0.187 |
Location of lesion | |||
Supratentorial | – | 21 | |
Infratentorial | – | 0 | |
Tumor diameter (cm) [mean (Q1, Q3)] | – | 3.2 (2.2, 3.8) | |
Pathology | |||
Low-grade glioma | – | 7 | |
High-grade glioma | – | 4 | |
Germ cell tumor | – | 5 | |
AT/RT | – | 1 | |
LCH | – | 1 | |
Schwannoma | – | 1 | |
Craniopharyngioma | – | 1 | |
No surgery | – | 1 | |
FSIQ | 108.32±8.63 | 98.05±11.41 | 0.003* |
VCI | 108.37±12.35 | 102.95±16.74 | 0.249 |
PRI | 108.74±10.86 | 104.19±13.33 | 0.243 |
WMI | 99.95±8.86 | 100.29±14.36 | 0.928 |
PSI | 103.11±12.98 | 91.90±18.08 | 0.030* |
Data are presented as number or mean ± standard deviation. *, P<0.05. HC, healthy control; AT/RT, atypical teratoid rhabdoid tumor; LCH, Langerhans cell histiocytosis; FSIQ, Full-Scale Intelligence Quotient; PRI, Perceptual Reasoning Index; PSI, Processing Speed Index; VCI, Verbal Comprehension Index; WMI, Working Memory Index.
ReHo analysis
Analysis revealed a significant reduction in ReHo in the left anterior cingulate and right middle frontal gyrus (MFG) in children with brain tumors as compared to HCs (P<0.05, TFCE and FWE corrected and 5,000 permutations). These findings are presented in Table 2 and illustrated in Figure 2.
Table 2
Brain area | Brodmann partition | MNI coordinates | T value | Cluster size | ||
---|---|---|---|---|---|---|
X | Y | Z | ||||
Anterior cingulate, L | – | −12 | 24 | 27 | −4.391 | 192 |
Middle frontal gyrus, R | 8 | 24 | 18 | 51 | −5.130 | 122 |
P<0.05, TFCE and FWE corrected and 5,000 permutations. ReHo, Regional Homogeneity; L, left; R, right; MNI, Montreal Neurological Institute; TFCE, Threshold-free Cluster Enhancement; FWE, familywise error.
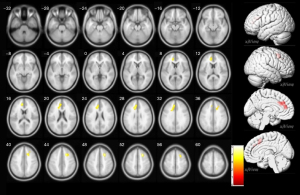
Correlation between ReHo and cognitive behavior
A significant correlation was observed between ReHo values in the right MFG of brain tumor patients and PRI scores (R=0.471; P=0.031) and WMI (R=0.531; P=0.013) of the WISC-IV. However, no significant correlation was found between ReHo in the anterior cingulate and any parameters of the WISC-IV, as shown in Figure 3 and Table 3.
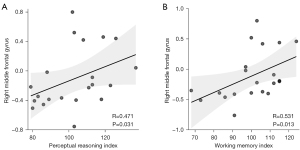
Table 3
WISC-IV | ReHo | ||||
---|---|---|---|---|---|
Anterior cingulate, L | MFG, R | ||||
R | P | R | P | ||
VCI | 0.073 | 0.755 | 0.096 | 0.679 | |
PRI | −0.060 | 0.798 | 0.471 | 0.031* | |
WMI | 0.182 | 0.430 | 0.531 | 0.013* | |
PSI | −0.096 | 0.680 | 0.051 | 0.827 | |
FSIQ | 0.052 | 0.822 | 0.363 | 0.106 |
*, P<0.05. ReHo, regional homogeneity; WISC-IV, fourth edition of the Wechsler Intelligence Scale for Children; L, left; MFG, middle frontal gyrus; R, right; VCI, Verbal Comprehension Index; PRI, Perceptual Reasoning Index; WMI, Working Memory Index; PSI, Processing Speed Index; FSIQ, Full-Scale Intelligence Quotient.
Discussion
Our study represents a pioneering effort in preoperative neuroimaging research, focusing on brain function differences in patients with pediatric brain tumor during rest. The application of rs-fMRI in our study revealed distinct ReHo value differences between patients with pediatric brain tumor and HCs, particularly in the MFG and left anterior cingulate. Correlation analysis between these ReHo values and WISC-IV scores indicated a positive relationship of ReHo values in the right MFG with the PRI and the WMI. The right MFG was the focus of this study because this brain area’s ReHo values were significantly different between patients with tumor and HCs and correlated with the behavioral results.
Tumor-induced alterations, such as increased intracranial pressure, reduced cerebral blood flow, and neurotransmitter availability limitations, may result in axonal and myelin damage or neuronal apoptosis causing cognitive impairment (11-13). The right MFG emerged as a focal point in our study due to significant ReHo differences and its correlation with cognitive performance outcomes. This observation aligns with existing research suggesting the involvement of the ventral frontoparietal network in the right hemisphere, particularly in attention regulation driven by sensory-driven, exogenous stimuli, which consists of the temporoparietal junction (TPJ) and ventral frontal cortex, including the MFG and inferior frontal gyrus (IFG). This regional network is known as the ventral attention network (VAN) (14,15). Each node of this network is thought to be involved in the attentional regulation of the formation of exogenous unexpected stimuli driven by bottom-up, nonautonomous stimuli (16). For example, the TPJ is active in any situation that requires orientation adjustment unrelated to expectation, whereas the right IFG and the right MFG are active only when orientation is adjusted to an unexpected stimulus (17). Meanwhile, the right IFG and the right MFG are active only when orientation is adjusted to an unexpected stimulus (17,18). In sum, this network is involved in the reorientation of relevant targets that appear in unexpected locations or important but less obvious targets (19,20).
One study suggests that the right MFG may play an important role in shifting attention from exogenous to endogenous control (21). In addition, another study demonstrated that the MFG has similar ability as does the Broca’s area in task-fMRI studies; it may thus be used as a predictor of language fluency to indicate hemisphental language dominance and as a supplementary measure for determining language laterality in preoperative planning (22). Subsequently, some researchers have studied the ability of the MFG to determine the dominant language hemisphere in patients with brain tumors based on rs-fMRI (23), indicating that the MFG has a similar function to the Broca’s area. In an rs-fMRI study of frontal lobe gliomas, voxel-mirrored homotopic connectivity (VMHC) in the right MFG was found to be decreased in patients with frontal lobe gliomas and was significantly and positively correlated with cognitive function (24). Our results align with previous rs-fMRI studies indicating the role of the right MFG in cognitive functions, including attentional shifts and language-processing abilities. The positive correlation of ReHo values in the right MFG with PRI and WMI in our study reinforces its potential role in cognitive changes, particularly in attention and working memory.
In Wechsler’s original model, verbal intelligence (VIQ) and performance intelligence (PIQ) combined to form Full-Scale Intelligence Quotient (FSIQ) (25). While VIQ includes VCI and WMI, PIQ includes PRI and PSI, with Block Design and Matrix Reasoning and Visual Puzzles being the core of PRI. These integrate multiple elements related to cognition, including visual perception, simultaneous processing, working memory, and spatial manipulation. Meanwhile, the WMI composite consists of two primary subtests, Digit Span and Arithmetic. It measures attention, concentration, and working memory. Working memory, based on attention and concentration, refers to the ability to temporarily retain information in the mind while the information is processed or engaged in distracting tasks and the subsequent ability to accurately reproduce the updated information or take action correctly. In previous studies, the Wechsler Intelligence Scale was widely used to measure cognitive function (26,27). In our study, ReHo values in the right MFG were found to be positively correlated with PRI and WMI, confirming the correlation between ReHo values in the right MFG and increased attention. The right MFG may be a potential target brain region for cognitive function changes in pediatric brain tumor patients, and the specific physiological mechanism needs to be further explored.
While some research has examined the functional aspects associated with brain tumors (28), our study focused on nontumor brain function changes. Due to the uneven distribution of tumor locations, we employed masking techniques to mitigate tumor influences in our statistical analysis (29,30). Several studies have shown that tumors can disrupt the structural brain networks in a variety of ways, such as physical displacement, invasion and destruction, altered connectivity, and pressure and inflammation (31-34). Our study preoperatively examined patients with brain tumor and cognitive impairment, which may be attributed to numerous factors, likely tumor size and extent, but subsequent studies should clarify the key factors (35).
This study has the following limitations: First, the sample size was relatively small, and we believe that a study with a larger sample size may benefit the generalizability of the findings. Our team will also continue to enroll research participants and conduct larger studies to determine the generalizability of the findings. Second, we did not perform age stratification to ensure adequate sample size because of the wide age range in our study, although we did include age as a covariate in the statistical analysis to eliminate its influence on the results. Third, due to the wide age distribution of participants, we employed an adult brain template, which may introduce some inaccuracies, particularly in younger children. Fourth, we did not group tumors according to specific location and pathology in our study. Considering that pediatric brain tumors often grow along the midline and progress rapidly in pathological studies (36), we will further verify these conclusions in a separate data set when sufficient samples have been collected. Moreover, validating our methodology through simulations or using existing larger datasets would enhance the robustness of our study. Although certain constraints prevented us from including such validations, we have outlined plans to incorporate them in future research. Finally, we used two functional MRI scans in our study because of force majeure, but both were operated by the same company and had the same parameters, and we included this factor as a covariate in our analysis.
Conclusions
We identified significant ReHo alterations in patients with pediatric brain tumor, primarily in brain regions associated with cognitive processing and found there to be a positive correlation between these alterations and specific cognitive functions. These findings contribute to understanding cognitive impairments in the patients with pediatric brain tumor and suggest potential areas for targeted intervention.
Acknowledgments
We would like to thank Rui Hao, Yan Lu, and Ting Gao for their technical support, as well as all participating children for their cooperation.
Funding: This work was supported by
Footnote
Reporting Checklist: The authors have completed the STROBE reporting checklist. Available at https://qims.amegroups.com/article/view/10.21037/qims-24-529/rc
Conflicts of Interest: All authors have completed the ICMJE uniform disclosure form (available at https://qims.amegroups.com/article/view/10.21037/qims-24-529/coif). The authors have no conflicts of interest to declare.
Ethical Statement: The authors are accountable for all aspects of the work in ensuring that questions related to the accuracy or integrity of any part of the work are appropriately investigated and resolved. This study adhered to the Declaration of Helsinki (as revised in 2013) and received approval from the Ethics Committee of Yuquan Hospital, Tsinghua University (No. 20220711). Informed consent was obtained from all participants’ guardians prior to participation.
Open Access Statement: This is an Open Access article distributed in accordance with the Creative Commons Attribution-NonCommercial-NoDerivs 4.0 International License (CC BY-NC-ND 4.0), which permits the non-commercial replication and distribution of the article with the strict proviso that no changes or edits are made and the original work is properly cited (including links to both the formal publication through the relevant DOI and the license). See: https://creativecommons.org/licenses/by-nc-nd/4.0/.
References
- Ostrom QT, Price M, Neff C, Cioffi G, Waite KA, Kruchko C, Barnholtz-Sloan JS. CBTRUS Statistical Report: Primary Brain and Other Central Nervous System Tumors Diagnosed in the United States in 2015-2019. Neuro Oncol 2022;24:v1-v95. [Crossref] [PubMed]
- Miller KD, Ostrom QT, Kruchko C, Patil N, Tihan T, Cioffi G, Fuchs HE, Waite KA, Jemal A, Siegel RL, Barnholtz-Sloan JS. Brain and other central nervous system tumor statistics, 2021. CA Cancer J Clin 2021;71:381-406. [Crossref] [PubMed]
- Peterson RK, Jacobson LA. Changes in executive function in pediatric brain tumor survivors. Pediatr Blood Cancer 2022;69:e29483. [Crossref] [PubMed]
- Bennett CM, Miller MB. How reliable are the results from functional magnetic resonance imaging? Ann N Y Acad Sci 2010;1191:133-55. [Crossref] [PubMed]
- Kong C, Xu D, Wang Y, Wang B, Wen J, Wang X, Zhan L, Sun Z, Jia X, Li M, Tang S, Hou D. Amplitude of low-frequency fluctuations in multiple-frequency bands in patients with intracranial tuberculosis: a prospective cross-sectional study. Quant Imaging Med Surg 2022;12:4120-34. [Crossref] [PubMed]
- Zang Y, Jiang T, Lu Y, He Y, Tian L. Regional homogeneity approach to fMRI data analysis. Neuroimage 2004;22:394-400. [Crossref] [PubMed]
- Yao X, Yin Z, Liu F, Wei S, Zhou Y, Jiang X, Wei Y, Xu K, Wang F, Tang Y. Shared and distinct regional homogeneity changes in bipolar and unipolar depression. Neurosci Lett 2018;673:28-32. [Crossref] [PubMed]
- Wilson CJ, Bowden SC, Vannier LC, Byrne LK, Weiss LG. Measurement invariance of the Wechsler Intelligence Scale for children, fifth edition, in Australian and New Zealand and U.S. standardization samples. Psychol Assess 2023;35:510-21.
- Kaufman AS, Flanagan DP, Alfonso VC, Mascolo JT. Test Review: Wechsler Intelligence Scale for Children, Fourth Edition (WISC-IV). J Psychoeduc Assess 2006;24:278-95.
- Yan CG, Wang XD, Zuo XN, Zang YF. DPABI: Data Processing & Analysis for (Resting-State) Brain Imaging. Neuroinformatics 2016;14:339-51. [Crossref] [PubMed]
- Del Bigio MR. Neuropathological changes caused by hydrocephalus. Acta Neuropathol 1993;85:573-85. [Crossref] [PubMed]
- Del Bigio MR. Neuropathology and structural changes in hydrocephalus. Dev Disabil Res Rev 2010;16:16-22. [Crossref] [PubMed]
- Air EL, Yuan W, Holland SK, Jones BV, Bierbrauer K, Altaye M, Mangano FT. Longitudinal comparison of pre- and postoperative diffusion tensor imaging parameters in young children with hydrocephalus. J Neurosurg Pediatr 2010;5:385-91. [Crossref] [PubMed]
- Corbetta M, Shulman GL. Human cortical mechanisms of visual attention during orienting and search. Philos Trans R Soc Lond B Biol Sci 1998;353:1353-62. [Crossref] [PubMed]
- Corbetta M, Shulman GL. Control of goal-directed and stimulus-driven attention in the brain. Nat Rev Neurosci 2002;3:201-15. [Crossref] [PubMed]
- Corbetta M, Kincade JM, Ollinger JM, McAvoy MP, Shulman GL. Voluntary orienting is dissociated from target detection in human posterior parietal cortex. Nat Neurosci 2000;3:292-7. [Crossref] [PubMed]
- Kincade JM, Abrams RA, Astafiev SV, Shulman GL, Corbetta M. An event-related functional magnetic resonance imaging study of voluntary and stimulus-driven orienting of attention. J Neurosci 2005;25:4593-604. [Crossref] [PubMed]
- Doricchi F, Macci E, Silvetti M, Macaluso E. Neural correlates of the spatial and expectancy components of endogenous and stimulus-driven orienting of attention in the Posner task. Cereb Cortex 2010;20:1574-85. [Crossref] [PubMed]
- Arrington CM, Carr TH, Mayer AR, Rao SM. Neural mechanisms of visual attention: object-based selection of a region in space. J Cogn Neurosci 2000;12:106-17. [Crossref] [PubMed]
- Indovina I, Macaluso E. Dissociation of stimulus relevance and saliency factors during shifts of visuospatial attention. Cereb Cortex 2007;17:1701-11. [Crossref] [PubMed]
- Japee S, Holiday K, Satyshur MD, Mukai I, Ungerleider LG. A role of right middle frontal gyrus in reorienting of attention: a case study. Front Syst Neurosci 2015;9:23. [Crossref] [PubMed]
- Dong JW, Brennan NM, Izzo G, Peck KK, Holodny AI. fMRI activation in the middle frontal gyrus as an indicator of hemispheric dominance for language in brain tumor patients: a comparison with Broca's area. Neuroradiology 2016;58:513-20. [Crossref] [PubMed]
- Gohel S, Laino ME, Rajeev-Kumar G, Jenabi M, Peck K, Hatzoglou V, Tabar V, Holodny AI, Vachha B. Resting-State Functional Connectivity of the Middle Frontal Gyrus Can Predict Language Lateralization in Patients with Brain Tumors. AJNR Am J Neuroradiol 2019;40:319-25. [Crossref] [PubMed]
- Hu G, Ge H, Yang K, Liu D, Liu Y, Jiang Z, Hu X, Xiao C, Zou Y, Liu H, Hu X, Chen J. Altered Static and Dynamic Voxel-mirrored Homotopic Connectivity in Patients with Frontal Glioma. Neuroscience 2022;490:79-88. [Crossref] [PubMed]
- Weiss LG, Saklofske DH, Coalson DL, Raiford SE. CHAPTER 3 - Theoretical, Empirical and Clinical Foundations of the WAIS-IV Index Scores. In: Weiss LG, Saklofske DH, Coalson DL, Raiford SE, editors. WAIS-IV Clinical Use and Interpretation. San Diego: Academic Press, 2010:61-94.
- Hu Z, Zou D, Mai H, Yuan X, Wang L, Li Y, Liao J, Liu L, Liu G, Zeng H, Wen F. Altered brain function in new onset childhood acute lymphoblastic leukemia before chemotherapy: A resting-state fMRI study. Brain Dev 2017;39:743-50. [Crossref] [PubMed]
- Zheng WJ, Guan XY, Fan KY, Gong J. Application of the CNS vital signs test and WISC-IV in the cognitive assessment of Chinese pediatric patients with intra-cranial space occupying lesion. Appl Neuropsychol Child 2024;13:229-38. [Crossref] [PubMed]
- Young JS, Morshed RA, Gogos AJ, Amara D, Villanueva-Meyer JE, Berger MS, Hervey-Jumper SL. The Glioma-Network Interface: A Review of the Relationship Between Glioma Molecular Subtype and Intratumoral Function. Neurosurgery 2020;87:1078-84. [Crossref] [PubMed]
- Aerts H, Colenbier N, Almgren H, Dhollander T, Daparte JR, Clauw K, Johri A, Meier J, Palmer J, Schirner M, Ritter P, Marinazzo D. Pre- and post-surgery brain tumor multimodal magnetic resonance imaging data optimized for large scale computational modelling. Sci Data 2022;9:676. [Crossref] [PubMed]
- Moretto M, Silvestri E, Facchini S, Anglani M, Cecchin D, Corbetta M, Bertoldo A. The dynamic functional connectivity fingerprint of high-grade gliomas. Sci Rep 2023;13:10389. [Crossref] [PubMed]
- Al Dahhan NZ, Cox E, Nieman BJ, Mabbott DJ. Cross-translational models of late-onset cognitive sequelae and their treatment in pediatric brain tumor survivors. Neuron 2022;110:2215-41. [Crossref] [PubMed]
- Shen C, Bao WM, Yang BJ, Xie R, Cao XY, Luan SH, Mao Y. Cognitive deficits in patients with brain tumor. Chin Med J (Engl) 2012;125:2610-7. [PubMed]
- Yang Y, Schubert MC, Kuner T, Wick W, Winkler F, Venkataramani V. Brain Tumor Networks in Diffuse Glioma. Neurotherapeutics 2022;19:1832-43. [Crossref] [PubMed]
- Semmel ES, Quadri TR, King TZ. Graph Theoretical Analysis of Brain Network Characteristics in Brain Tumor Patients: A Systematic Review. Neuropsychol Rev 2022;32:651-75. [Crossref] [PubMed]
- Zou QH, Zhu CZ, Yang Y, Zuo XN, Long XY, Cao QJ, Wang YF, Zang YF. An improved approach to detection of amplitude of low-frequency fluctuation (ALFF) for resting-state fMRI: fractional ALFF. J Neurosci Methods 2008;172:137-41. [Crossref] [PubMed]
- Louis DN, Perry A, Wesseling P, Brat DJ, Cree IA, Figarella-Branger D, Hawkins C, Ng HK, Pfister SM, Reifenberger G, Soffietti R, von Deimling A, Ellison DW. The 2021 WHO Classification of Tumors of the Central Nervous System: a summary. Neuro Oncol 2021;23:1231-51. [Crossref] [PubMed]