Gadoxetic acid disodium (Gd-EOB-DTPA) contrast-enhanced abbreviated magnetic resonance imaging (MRI) for hepatocellular carcinoma surveillance in at-risk patients: a multi-center study in China
Introduction
Hepatocellular carcinoma (HCC) is the most common primary liver cancer and the third leading cause of cancer-related deaths worldwide (1,2). The incidence of HCC has continued to rise in recent decades (3). In contrast to Western nations, the etiology of HCC in the Asia-Pacific countries is primarily caused by chronic hepatitis B or C virus (HBV and HCV, respectively) infection and cirrhosis (4). The therapeutic methods and prognosis of patients are determined by the stage of HCC. However, more than 70% of patients have been found with late-stage HCC, suggesting that they are no longer eligible for curative surgical therapies, and the 5-year survival is less than 10% in the advanced stage patients (5). Meanwhile, the 5-year survival rate for patients with early-stage HCC may improve significantly if curative treatment is timely provided (6,7). Therefore, the most effective way to improve the long-term survival of HCC is active antiviral treatment and early detection of HCC through regular surveillance in at-risk patients (8).
To identify potential curable HCC at an early stage, imaging-based surveillance in at-risk patients (chronic HBV or HCV infection and cirrhosis patients) utilizing biannual non-contrast enhanced ultrasonography (US) combined with serum alpha-fetoprotein (AFP) every 6 months is recommended by current clinical guidelines (9). However, the sensitivity of US is inadequate, particularly for patients with obesity, moderate to severe fatty liver, ascites, and liver dysfunction with Child-Pugh class B or C. Previous studies have demonstrated that to poor visualization, US is insufficient in detecting early-stage HCC or small HCC, ranging from 47% to 63% (10,11). In addition, AFP has limited efficacy for early-stage HCC with low specificity (12).
Given the limited capacity and suboptimal sensitivity of US, multiphasic computed tomography (CT), and dynamic contrast-enhanced (DCE) magnetic resonance imaging (MRI) have been suggested for the surveillance of HCC in at-risk patients. Contrast-enhanced MRI using a liver-specific contrast agent (13), gadoxetic acid disodium (Gd-EOB-DTPA) has been shown to provide good diagnostic performance with a high detection rate for hepatic lesions in the workup of HCC screening. Gd-EOB-DTPA contrast-enhanced MRI is more sensitive than US to detect early-stage HCC in high-risk patients with cirrhosis (14). However, the shortcomings of using MRI as a clinical surveillance tool mainly include the long examination time, inconvenience, and higher costs compared with US. Some researchers have proposed abbreviated MRI (AMRI) protocols that incorporate a few selected sequences that could enable HCC screening/surveillance to reach an equivalent diagnostic performance in a shorter time (15-17). Several prospective studies have demonstrated that AMRI was significantly higher than US in diagnostic sensitivity and diagnostic yield for HCC surveillance in high-risk patients, with its diagnostic performance closely approximating that of Gd-EOB-DTPA-enhanced MRI. Moreover, AMRI offers superior visualization, making it a viable alternative screening tool for patients with nonalcoholic fatty liver disease and cirrhosis when US is suboptimal (18-20). Theoretically, there is a reduction in the number of sequences in AMRI protocols which enables a lower cost of MRI and decreases image assessment complexity, making it more applicable for routine HCC surveillance. Any AMRI protocol consisting of 2–3 sequences as a surveillance tool has high potential as a promising substitute for the complete contrast-enhanced MRI.
Therefore, this multi-center, retrospective, blinded reader study aimed to compare the diagnostic performance of 3 AMRI protocols versus complete Gd-EOB-DTPA contrast-enhanced MRI for HCC surveillance in at-risk patients with chronic hepatitis and cirrhosis. We present this article in accordance with the STARD reporting checklist (available at https://qims.amegroups.com/article/view/10.21037/qims-24-941/rc).
Methods
Patients
The study was conducted in accordance with the Declaration of Helsinki (as revised in 2013). The study was approved by Institutional Review Board of the First Affiliated Hospital of Henan University of Chinese Medicine (No. 2023HL-034-01). All participating hospitals were informed and agreed with the study, and the requirement to obtain written informed consent was waived due to the retrospective collection of information from our patients. Consecutive patients with hepatitis or cirrhosis underwent a complete Gd-EOB-DTPA-enhanced MRI between January 2020 and January 2023 for HCC screening/surveillance. Only the first available examination was included for each patient to avoid duplication. The inclusion criteria were as follows: (I) patients with chronic HBV or HCV infection and/or cirrhosis; (II) patients who had no history of prior treatment including hepatectomy, transcatheter arterial chemoembolization (TACE), hepatic artery infusion chemotherapy (HAIC), ablation, radiotherapy, and systemic therapy; (III) a single lesion ≤5 cm in maximum diameter or up to 3 solitary lesions smaller than 3 cm (21). The exclusion criteria were as follows: (I) insufficient image quality with severe artifacts; (II) known primary or secondary non-HCC hepatic malignancy or hepatic metastasis or a history of extrahepatic malignant tumor; (III) no follow-up examinations or procedures; (IV) patients were examined using extracellular contrast agent (ECCA). Among 625 initial patients, 443 patients were mainly from the First Affiliated Hospital of Henan University of Chinese Medicine, 120 patients were from Hunan Provincial People’s Hospital, and 62 patients from Henan Cancer Hospital. According to the inclusion and exclusion criteria, 286 patients were excluded. Finally, 221, 78, and 40 patients were included for statistical analysis from the 3 hospitals, respectively (flowchart is shown in Figure 1).
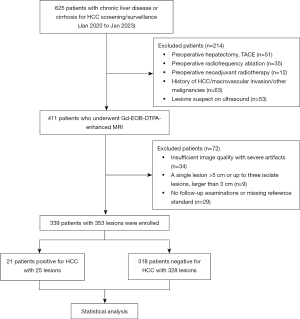
Imaging acquisition
All the MRI examinations were performed on 3.0T MRI imaging systems (Ingenia CX; Philips, Amsterdam, Netherlands) using a 16-channel body phased-array coil. The routine magnetic resonance (MR) sequences were as follows: (I) single shot turbo spin echo T2-weighted imaging (T2WI) coronal plane; (II) fast field echo T1-weighted imaging (T1WI) in/opposed phase; (III) DCE-T1WI: obtained using mDIXON-FFE (Philips) and performed after intravenous injection of 0.025 mmol/kg of Gd-EOB-DTPA (Xian Ai, CHIATAI TIANQING Pharmaceutical Co. Ltd., Hangzhou, China) at a rate of 1 mL/s, followed by a 20 mL saline flush using bolus-tracking methods. The early and late arterial phases (AP), portal venous phase (PVP), and transitional phase (TP) were acquired at 15–20 seconds, 60 seconds, and 120 seconds from the start of Gd-EOB-DTPA injection; (IV) turbo spin echo T2WI; (V) T2WI with fat suppression; (VI) diffusion-weighted imaging (DWI) and apparent diffusion coefficient (ADC): single shot echo planar imaging (SS-EPI) using b values of 50 and 800s/mm2; (VII) hepatobiliary phase (HBP): acquired at 15–20 minutes after the administration of Gd-EOB-DTPA (MRI parameters are shown in Table 1).
Table 1
Sequences | TR (ms) | TE (ms) | FOV (mm2) | Slice thickness (mm) | Slice gap (mm) | Scan matrix | Flip angle (°) |
---|---|---|---|---|---|---|---|
T1WI in-and out-of-phase | 10 | 2.3 | 400×384 | 6 | 1 | 268×176 | 9 |
Turbo field echo T1WI | 3.1 | 1.1 | 400×384 | 5 | 1 | 180×200 | 9 |
DCE-T1W | 3.1 | 1.1 | 400×384 | 5 | 2.5 | 180×200 | 9 |
Single shot fast echo T2WI | 1,250 | 80 | 400×384 | 6 | 1 | 320×282 | 180 |
T2WI sequence with fat suppression | 566 | 70 | 400×384 | 7 | 1 | 252×222 | 160 |
DWI | 2,400 | 65 | 400×384 | 7 | 1 | 132×114 | 90 |
HBP | 3.7 | 1.32 | 400×384 | 4 | 2 | 200×200 | 9 |
MRI, magnetic resonance imaging; TR, repetition time; TE, echo time; FOV, field of view; T1WI, T1-weighted imaging; DCE, dynamic contrast-enhanced; T2WI, T2-weighted imaging; DWI, diffusion-weighted imaging; HBP, hepatobiliary phase.
A total of 3 AMRI protocols were extracted from the complete Gd-EOB-DTPA contrast-enhanced MRI (see Figure 2): (I) noncontrast-AMRI (NC-AMRI), including T2WI + DWI; (II) dynamic-AMRI (Dyn-AMRI), including 3 phases (early and late APs, PVP) dynamic T1WI + DWI; (III) HBP-AMRI, including T2WI + DWI + HBP. According to the consensus report for Gd-EOB-DTPA contrast-enhanced MRI scanning scheme (22), T2WI and DWI could be performed after a contrast-enhanced dynamic study (before acquisition of HBP). The scanning times for NC-AMRI and HBP-AMRI were less than 5 minutes, and that for Dyn-AMRI was almost 6 minutes. The average scanning time for complete Gd-EOB-DTPA-enhanced MRI required approximately 25–30 minutes. The estimated scanning times of the 3 AMRI protocols (without calculating patient positioning on the table, sequence set-up, and exam termination) are shown in Figure 2.
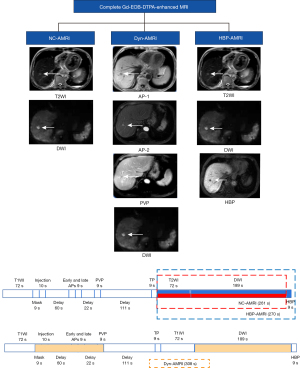
Imaging analysis
The 4 MRI protocols were independently reviewed in the PACS (picture archiving and communication system) by 2 abdominal radiologists, with 10 years and 15 years of experience in liver MR imaging. To simulate an MRI protocol for each patient included in the study, MR images were presented in a randomized distribution, and evaluation based on any AMRI protocol in the same patient was separated by at least 3 weeks to minimize recall bias. Each protocol was assessed by 2 radiologists, who were blinded to the imaging reports, clinical data, pathology, and laboratory results. The 4 MRI protocols were scored based on the Liver Imaging Reporting and Data System (LI-RADS) version 2018 (23). However, only the complete Gd-EOB-DTPA contrast-enhanced MRI and Dyn-AMRI allow non-rim AP hyperenhancement (APHE: lesion typically shows increased enhancement on AP compared to surrounding normal liver parenchyma) and non-peripheral washout on PVP (lesion shows a relative decrease in enhancement on PVP) as the main imaging features. Additionally, capsule appearance (peripheral rim of enhancement) was regarded as an important imaging feature of HCC. Since readers could not access previous imaging, threshold growth was not considered. NC-AMRI and HBP-AMRI depend on the presence of LI-RADS ancillary imaging features to detect and characterize lesions. The signal intensity of lesions was identified as hyperintensity, isointensity, and hypointensity using the surrounding normal liver parenchyma as a reference in each MRI sequence.
Diagnostic criteria for HCC on the complete Gd-EOB-DTPA contrast-enhanced MRI were as follows:
Lesions with restricted diffusion (mild to moderate hyperintensity on DWI and hypointensity on ADC map) (24), mild to moderate hyperintensity on T2WI (higher signal intensity than surrounding liver parenchyma), whereas hyperintensity similar to the bile ducts and other fluid-filled structures could not be regarded as positive, as these findings were suggestive of benign lesions, such as cysts and hemangiomas, lesions <20 mm with non-rim APHE and non-peripheral washout on PVP, regardless of capsule appearance, or lesions ≥20 mm with non-rim APHE with either washout and/or capsule on PVP, and hypointensity on HBP.
HCC was diagnosed as positive on AMRI as follows:
- NC-AMRI: lesions with restricted diffusion, mild to moderate hyperintensity on T2WI.
- Dyn-AMRI: lesions <20 mm with non-rim APHE and non-peripheral washout appearance, regardless of capsule appearance, or lesions ≥20 mm with non-rim APHE with either nonperipheral washout appearance and/or capsule appearance on PVP, and restricted diffusion on DWI.
- HBP-AMRI: lesions with restricted diffusion, hyperintensity on T2WI, and hypointensity on HBP.
When 1 or more lesions in a patient met the above imaging criteria, they were considered HCC positive (Figure 3A-3E); otherwise, they were considered HCC negative.
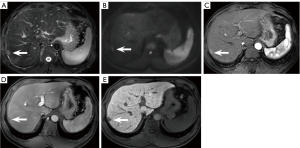
Reference standard
Each patient was classified as HCC positive/negative by the 4 MRI protocols. The reference standard for HCC diagnosis was based on the pathological results or typical imaging features with at least 6-month follow-up.
Patients were diagnosed as HCC positive when:
- The patient was pathologically confirmed as HCC within 12 months after Gd-EOB-DTPA contrast-enhanced MRI.
- They had LI-RADS 5 lesions, or LI-RADS 4 lesions that were observed to be ≥10 mm within 12 months of follow-up imaging (25,26).
- After locoregional therapy, HCC was confirmed based on the integrated imaging and clinical data within 12 months after MRI examination.
The clinical data mainly referred to the following 3 points:- Liver function tests: deterioration of liver function tests could suggest tumor progression, but may also result from cirrhosis or treatment effects.
- Symptoms: new or worsening symptoms, such as abdominal pain or weight loss, might raise suspicion.
- Laboratory findings:
- AFP: generally, AFP levels >20 ng/mL are considered indicative of HCC. However, the specific threshold may vary depending on the study and patient population, with some studies using 200 ng/mL for higher specificity.
Protein induced by vitamin K absence or antagonist-II (PIVKA-II or DCP): a PIVKA-II level >40 mAU/mL is widely accepted as a positive diagnostic criterion for HCC. - Circulating microRNAs: the threshold values for circulating microRNAs are complex and typically involve specific expression profiles and algorithms. Currently, there is no unified standard value, and the specific threshold needs to be determined based on the particular combination of microRNAs and detection methods used.
Lens culinaris agglutinin-reactive fraction of AFP (AFP-L3): the AFP-L3 percentage (the proportion of AFP-L3 in the total AFP) >10% is commonly used as a positive diagnostic criterion for HCC.
These biomarkers should be utilized in conjunction with imaging and clinical data to improve diagnostic accuracy, monitor disease progression, and evaluate treatment response.
Patients were diagnosed as HCC negative when:
- No lesion was found on the initial and follow-up MRI, or observations were scored as LI-RADS 1 and/or LI-RADS 2.
- They had pathologically proven benign lesion/s.
- No LI-RADS 4 and/or LI-RADS 5 lesions were identified within 6–12 months of follow-up imaging after the initial MRI (26).
Statistical analysis
Continuous variables with normal distribution were represented by mean ± standard deviation (x±s), and variables with non-normal distribution were represented by median (interquartile range). The statistical differences were analyzed using Student’s t-test or Mann-Whitney U test, and the acquisition time differences were subjected to was analysis of variance (ANOVA). Categorical variables were expressed as frequency and percentage, and compared using Chi-squared tests or Fisher’s exact tests. The sensitivity, specificity, positive predictive value (PPV), and negative predictive values (NPV) were obtained for each protocol with binomial 95% confidence intervals (CI). Moreover, the number needed to diagnose (NND) values for the 4 MRI protocols were calculated according to the formula: NND=1/[a/(a+c)−b/(b+d)]. The diagnostic performance was evaluated using Cochran’s Q test, and post hoc pairwise comparisons of sensitivity and specificity were performed using Dunn’s test (corrected by Bonferroni correction) among 4 MRI protocols. Receiver operating characteristic (ROC) curves for 3 AMRI protocols were evaluated, and the area under the ROC curve (AUROC) was calculated and compared by DeLong’s test. To evaluate the inter-reader agreement on imaging feature interpretation, the Kappa coefficient (k) was calculated, which was categorized into several grades: no agreement (k<0), slight (0 ≤k≤0.2), fair (0.2<k≤0.4), moderate (0.4<k≤0.6), good (0.6<k≤0.8), almost perfect (k>0.8), and perfect agreement (k=1). Statistical analysis was performed using the statistical software package SPSS 26.0 (IBM Corp., Armonk, NY, USA) and MedCalc version 19.0.7 (MedCalc Software Ltd., Ostend, Belgium). A 2-sided P value <0.05 was regarded as statistically significant.
Results
Patient characteristics
From the initial cohort of 625 patients, 286 were excluded after 2 screening rounds. Among these exclusions, 92 patients had HBV, 58 had HCV, 84 had cirrhosis, and 52 exhibited liver dysfunction (patients with Child-Pugh Grade A, B, and C were 17, 21, and 14, respectively). A total of 353 lesions were detected in the 339 included patients (median age 50.74±15.39 years; range, 20–88 years). Among them, 25 HCCs (median diameter 18.86±14.46 mm, range, 3.0–48.80 mm, 19 HCCs were ≤3 cm, 6 HCCs were ≤1 cm, and 16 were early-stage HCCs) were identified among 353 lesions. A total of 19 of 21 patients (90.5%) with HCC had chronic HBV infection, 2 of 21 (9.5%) had HCV infection, and 20 of 21 (95.2%) had liver cirrhosis. There were 10, 9, and 2 patients with Child-Pugh Grade A, B, and C, respectively. Furthermore, 288 of 318 patients (90.6%) with HCC negative had chronic HBV infection, 30 of 318 (9.4%) had HCV infection, and 249 of 318 (78.3%) had liver cirrhosis. There were 177, 107, and 24 patients with Child-Pugh Grade A, B, and C, respectively. There was no statistical significance between the patients with HCC positive and negative for the incidence of cirrhosis, however, patients with HCC positive were much older. The clinical characteristics of patients are summarized in Table 2.
Table 2
Clinical parameters | HCC positive (n=21) | HCC negative (n=318) | t/χ2 | P value |
---|---|---|---|---|
Gender | 0.802 | 0.371 | ||
Male | 14 (66.7) | 172 (54.1) | ||
Female | 7 (33.3) | 146 (45.9) | ||
Age (years) | 57.52±9.09 | 50.29±15.62 | 2.096 | 0.037 |
Virus | 0.005 | 0.945 | ||
HBV | 19 (90.5) | 288 (90.6) | ||
HCV | 2 (9.5) | 30 (9.4) | ||
Liver cirrhosis | 2.493 | 0.114 | ||
Presence | 20 (95.2) | 249 (78.3) | ||
Absence | 1 (4.8) | 69 (21.7) | ||
Child-Pugh grade | 0.109 | 0.574 | ||
Child-Pugh A | 10 (47.6) | 177 (55.7) | - | |
Child-Pugh B | 9 (42.9) | 107 (33.6) | - | |
Child-Pugh C | 2 (9.5) | 24 (7.5) | ||
Normal | 0 | 10 (3.1) |
Data are presented as mean ± standard deviation or number (%). The age comparison was performed using independent samples t-test. Except where noted, data were compared using the χ2 test. HCC, hepatocellular carcinoma; HBV, hepatitis B virus; HCV, hepatitis C virus.
Per-lesion diagnostic performance
A summary of the per-lesion diagnostic performance of the 4 MRI protocols is presented by Table 3. Statistical analysis showed significant differences in sensitivity (Cochran’s Q =14.667, P=0.002) and specificity (Cochran’s Q =59.682, P<0.001) among the 4 MRI protocols. HBP-AMRI had a significantly higher sensitivity than NC-AMRI (84.00% vs. 60.00%, respectively, P=0.001), Dyn-AMRI (84.00% vs. 64.00%, respectively, P=0.023), and the complete MRI (84.00% vs. 64.00%, respectively, P=0.023), but there was no significant difference between NC-AMRI and Dyn-AMRI (60.00% vs. 64.00%, respectively, P>0.99). Dyn-AMRI had a significantly higher specificity than both NC-AMRI and HBP-AMRI (99.39% vs. 93.59%, respectively, P<0.001; 99.39% vs. 92.98%, respectively, P<0.001), but no statistical significance compared with complete Gd-EOB-DTPA contrast-enhanced MRI (99.39% vs. 99.69%, respectively, P>0.99). The per-lesion PPV for the NC-AMRI, Dyn-AMRI, and HBP-AMRI protocols were 41.66%, 88.89%, and 47.72%, respectively, and the corresponding NPV were 96.84%, 97.31%, and 98.70%, respectively. The NND for the NC-AMRI, Dyn-AMRI, HBP-AMRI, and complete Gd-EOB-DTPA contrast-enhanced MRI were: 1.865, 1.577, 1.234, and 1.569, respectively. The AUROC value of NC-AMRI, Dyn-AMRI, and HBP-AMRI were 0.768 (95% CI: 0.720–0.811), 0.817 (95% CI: 0.773–0.856), and 0.885 (95% CI: 0.847–0.916) (the ROC curve is shown in Figure 4). DeLong’s test showed that the AUROC value of either Dyn-AMRI or HBP-AMRI was significantly higher than that of NC-AMRI (Z=2.330, P=0.019; Z=2.680, P=0.007, respectively), but there was no significant difference between HBP-AMRI and Dyn-AMRI (Z=1.643, P=0.100).
Table 3
Diagnostic performance | NC-AMRI | Dyn-AMRI | HBP-AMRI | Complete | P value | |||||
---|---|---|---|---|---|---|---|---|---|---|
NC-AMRI vs. Dyn-AMRI | NC-AMRI vs. HBP-AMRI | NC-AMRI vs. Complete | Dyn-AMRI vs. HBP-AMRI | Dyn-AMRI vs. Complete | HBP-AMRI vs. Complete | |||||
Sensitivity, % | 60.00 (38.66–78.87) |
64.00 (42.52–82.03) |
84.00 (63.91–95.46) |
64.00 (42.52–82.02) |
>0.99 | 0.001 | >0.99 | 0.023 | >0.99 | 0.023 |
Specificity, % | 93.59 (90.37–95.93) |
99.39 (97.81–99.92) |
92.98 (89.66–95.50) |
99.69 (98.31–99.99) |
<0.001 | >0.99 | <0.001 | <0.001 | >0.99 | <0.001 |
PPV, % | 41.66 (29.72–59.65) |
88.89 (66.07–97.04) |
47.72 (37.27–58.38) |
94.11 (68.86–99.14) |
– | – | – | – | – | – |
NPV, % | 96.84 (94.99–98.02) |
97.31 (95.51–98.39) |
98.70 (96.87–99.46) |
97.32 (95.56–98.39) |
– | – | – | – | – | – |
AUROC | 0.768 (0.72–0.81) |
0.817 (0.77–0.85) |
0.885 (0.84–0.91) |
– | 0.019 | 0.007 | – | 0.100 | – | – |
NND | 1.865 | 1.577 | 1.234 | 1.569 |
Data are performance measures in percentages with numerators and denominators in brackets and 95% confidence intervals in parentheses. HCC, hepatocellular carcinoma; MRI, magnetic resonance imaging; NC-AMRI, non-contrast AMRI; Dyn-AMRI, dynamic-AMRI; HBP-AMRI, hepatobiliary phase AMRI; Complete, complete Gd-EOB-DTPA contrast-enhanced MRI; PPV, positive predict value; NPV, negative predict value; AUROC, the area under the receiver operating characteristic curve; NND, number needed to diagnose; AMRI, abbreviated MRI; Gd-EOB-DTPA, gadoxetic acid disodium.
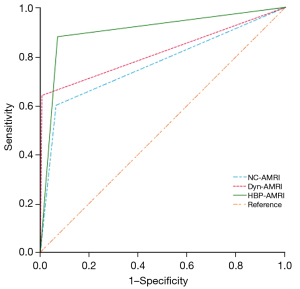
Interobserver agreement
The inter-observer agreement for classifying HCC-positive/HCC-negative in each of the 4 MRI protocols was as follows: NC-AMRI (k=0.786), Dyn-AMRI (k=0.810), HBP-AMRI (k=0.877), and complete Gd-EOB-DTPA contrast-enhanced MRI (k=0.876).
False positives
There were 6.4% (21/328) false positives on NC-AMRI. These were due to presumed hemangioma (n=4) (Figure 5A-5E), dysplastic nodule (n=5) (Figure 6A-6E), intrahepatic cholangiocarcinoma (n=2) (Figure 7A-7E), hepatic epithelioid angiomyolipoma (n=2), hepatic angiomyolipoma (n=1), primary hepatic lymphoma (n=1), hepatic epithelioid hemangioendothelioma (n=1), hepatocellular adenoma (n=2), focal eosinophilic necrosis (n=1), hepatic pseudo-lesion (n=1), and nodular fibrosis (n=1). There were 7.0% (23/328) false positives identified on HBP-AMRI, which mirrored the observations of NC-AMRI except for 2 additional dysplastic nodules that were considered positive on HBP-AMRI. The 0.6% (2/328) false positives on Dyn-AMRI were hepatic epithelioid angiomyolipomas.
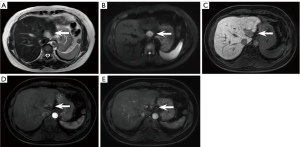
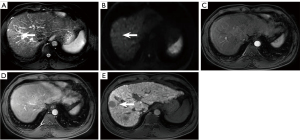
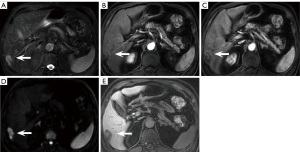
False negatives
There was 4% (10/25) false negativity on NC-AMRI, including 4 HCCs that were missed on both T2WI and DWI, 3 HCCs that were missed on T2WI only, and 3 HCCs that were missed on DWI only. There were 1.6% (4/25) false negatives on HBP-AMRI due to poor visibility on HBP. There were 3.6% (9/25) false negatives observed on Dyn-AMRI. Among them, 3 small HCCs and 4 micro-HCCs were missed, and 2 HCCs (Figure 8A-8E) were missed on the AP owing to motion artifacts.
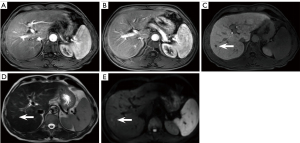
Acquisition time
The acquisition times (acquisition time for sequences only) were statistically different between the 4 MRI protocols (P<0.001). From the shortest to the longest, NC-AMRI (263.44±5.05 s) < HBP-AMRI (269.18±4.93 s) < Dyn-AMRI (307.71±4.93 s) < complete Gd-EOB-DTPA contrast-enhanced MRI (582.03±3.59 s). The acquisition time for the NC-AMRI protocol was the shortest.
Discussion
Although there have already been several studies on HCC surveillance by AMRI, most have involved a single center. This multi-center study in China enrolled patients from 3 different hospitals (a comprehensive Chinese medicine hospital, cancer hospital, and general western medicine hospital) addressing the limitation of a single sample study and spectrum bias due to the inclusion of massive tumors or late-stage HCC. In this study, we compared the diagnostic performance of 3 AMRI protocols with the complete Gd-EOB-DTPA contrast-enhanced MRI when histopathology and follow-up imaging were used as the reference standard. Our findings showed that HBP-AMRI yielded a high sensitivity of 84% in detecting HCC, supporting the feasibility and practicality of AMRI for HCC surveillance with satisfactory diagnostic performance in at-risk patients in China.
The NC-AMRI protocol is the simplest scanning scheme among the 3 AMRI protocols, comprising 2 fundamental sequences: T2WI and DWI. As HCC surveillance requires periodic repetitive examinations, many previous studies showed the potential value of NC-AMRI as a practical surveillance tool for HCC (19,27). It is well known that T2WI should be included for surveillance, as it is essential to exclude the benign lesions showing typical markedly high signal intensity on T2WI such as cysts or hemangiomas that can mimic HCC on DWI and help to localize abnormalities with better spatial resolution, thus improving the specificity and decreasing false-positive (28). Furthermore, DWI as a functional technique is routinely added to the standard liver MRI protocol based on higher cellularity and microstructural heterogeneity, reflecting that the presence of restricted diffusion shows higher signal intensity than background liver parenchyma on high b value DWI, which helps detect and characterize small tumors and improve the sensitive for HCC detection (29). Similar to the literature of a single retrospective surveillance cohort in which NC-AMRI was adopted with the lower per-patient sensitivity of 61.5% (27), the sensitivity of NC-AMRI was the lowest among the 3 AMRI protocols in our study. This can be mainly attributed to 2 factors: (I) NC-AMRI has a relatively low lesion-to-liver contrast, and some HCCs appear isointense to the liver on T2WI or are obscured by heterogeneous background hepatic parenchyma caused by cirrhosis (30); (II) DWI is vulnerable to artifacts, especially in the left lobe and liver dome (31), and well-differentiated HCC may not show obvious restriction on DWI (24). However, NC-AMRI does not warrant contrast agents, thereby decreasing the underlying contrast-related adverse reactions such as allergy, gadolinium tissue deposition, and nephrogenic sclerosing fibrosis (15). In addition, in a comparative study conducted Liu et al. (32), NC-AMRI demonstrated significant superiority over the standard US for HCC surveillance with a per-patient sensitivity of 93.2%. To harness the full potential of MRI for surveillance and screening, an abbreviated scanning protocol is needed to maximize efficacy while minimizing both surveillance time and time and associated costs. In this study, NC-AMRI achieved these goals with the shortest scanning time (263.44±5.05 s), low cost, and contrast agent exemption, suggesting that the NC-AMRI protocol could emerge as an alternative tool in the setting of HCC surveillance, particularly when patients cannot tolerate long exposure.
Dyn-AMRI is composed of early AP, late AP, PVP, and DWI sequences. During carcinogenesis, hepatocellular nodules may lose their normal portal triads and develop unpaired arteries, resulting in increased arterial flow and decreased portal flow, showing APHE and washout on Dyn-AMRI (33). Typical imaging manifestations entail high specificity for the diagnosis of HCC and have been recommended as the major imaging features in LI-RADS (34). According to our results, Dyn-AMRI achieved the highest specificity of 99.39% among the 3 AMRI protocols, which approached that of complete Gd-EOB-DTPA contrast-enhanced MRI. Furthermore, Dyn-AMRI demonstrated superior diagnostic performance compared to NC-AMRI. Dyn-AMRI could be suggested as a reliable and feasible surveillance strategy with the following advantages: (I) Dyn-AMRI protocol can be easily replicated at all MRI scanners and the workflow is consecutive; (II) if a lesion meets the LI-RADS criteria on Dyn-AMRI, which could serve as both a screening and diagnostic tool, the diagnosis of HCC can be validated without histopathological confirmation or additional recall tests, as the major imaging features from LI-RADS for HCC diagnosis (APHE, washout, and capsule) are grounded on DCE imaging (35-37); (III) portal vein tumor thrombus can be detected by Dyn-AMRI. However, the pitfall of Dyn-AMRI is occasional suboptimal quality of AP images associated with motion artifacts, and transient severe motion (TSM) artifacts frequently occur on Gd-EOB-DTPA contrast-enhanced MRI (Figure 8A) (38). When patient is elderly or obese, has ascites, chronic pulmonary disease, or other factors, breath-hold failure may be induced, significantly increasing the probability of TSM during acquisition of AP images (39,40). In our study, TSM artifacts were commonly seen in elderly patients with poor liver function, leading to the degradation of AP images, which may reduce the sensitivity on Dyn-AMRI. Dyn-AMRI using ECCA can offer a cost-effective alternative, while achieving superior diagnostic accuracy. The reasons include low occurrence of TSM on AP, the optional identification of “washout” on PVP or equilibrium phase of ECCA contrast-enhanced MRI compared with the constrain of “washout” merely on PVP of Gd-EOB-DTPA contrast-enhanced MRI. Furthermore, when only utilizing DCE images, there was a small discrepancy in detecting hepatic lesions between Dyn-AMRI and a complete contrast-enhanced MRI using ECCA (41). Only 5% of LI-RADS 3 lesions were affected and upgraded to LI-RADS 4 with the inclusion of T2WI and DWI (41).
Our investigation revealed that the sensitivity of HBP-AMRI was 84.00%, which was higher than that of NC-AMRI, Dyn-AMRI, and complete Gd-EOB-DTPA contrast-enhanced MRI. The highest sensitivity of HBP-AMRI is primarily attributed to the strong lesion-to-liver contrast on HBP images subsequent to Gd-EOB-DTPA uptake, which enhances the conspicuity of lesions, particularly those of small or micro-lesions, as well as early-stage HCCs, thereby facilitating their detection (42). We also found that the absence of difference in the diagnostic performance between HBP-AMRI and Dyn-AMRI may be related to the divergence in study populations. Our enrolled patients had a history of chronic HBV or HCV infection and/or cirrhosis, and the majority of identified lesions were smaller than 3 cm. It is quite difficult to differentiate some cirrhotic nodules from HCC lesions that are smaller than 2 cm, which creates a susceptibility to misdiagnosis due to decreased sensitivity (43). As classified by the Barcelona Clinic Liver Cancer (BCLC) staging system (44), early-stage HCCs usually present as a single nodule measuring less than 3 cm in diameter. Most small HCCs, especially early-stage HCCs, may demonstrate atypical enhancement patterns like “isointensity” on AP or PVP, particularly hypovascular enhancement on AP (45), indicating the absence of APHE or lack of washout during dynamic imaging owing to incomplete neovascularization (35), and they may first become visible on HBP as hypointense nodules (Figure 8C) before APHE is detectable. Hence, we should intensify the follow-up observation of lesions that do not display the typical imaging features of HCC, yet present as hypointense on HBP. In addition, compared with Dyn-AMRI, HBP-AMRI can be acquired sequentially after injection outside the scanning room (46). It could be administered via a one-time hand injection, which can eliminate TSM artifacts, save time, and facilitate scanning in patients with poor intravenous access (47).
Although HBP hypointensity exhibits high sensitivity in detecting incipient alterations indicative of HCC, its specificity remains suboptimal. According to our experience, it is noted that high-grade dysplastic nodules (HGDNs) can also appear hypointense on HBP without APHE and mild hyperintensity on T2WI (Figure 6A-6E). Renzulli et al. (48) proposed that HGDNs and early-stage HCCs in a cirrhotic liver background could be differentiated via high b-value DWI as both of them might demonstrate hypointensity on HBP. Additionally, “targetoid sign” on DWI (restricted diffusion in the periphery with less or no restricted diffusion in the center) can exclude some non-HCC malignant neoplasms to decrease false positive and further improve the diagnostic specificity of HCC on HBP-AMRI protocol (Figure 7D) (49). A previous study (35) established the new diagnostic criteria on 5 different sequences, including APHE, PVP washout, HBP hypointensity, DWI hyperintensity, and mild to moderate T2 signal intensity. Their results demonstrated that HBP hypointensity exhibited the highest sensitivity, reaching 97.14% among all the diagnostic criteria. Nevertheless, we should be fully aware that various hepatic neoplasms such as hemangioma, adenoma, intrahepatic cholangiocarcinoma, and combined HCC-cholangiocarcinoma could also appear as hypointense on HBP (Figure 5E). Consequently, sole reliance on HBP imaging is far from sufficient to make any diagnosis; the combination of DWI and/or T2WI in AMRI protocol can effectively help differentiation, reducing the false positive diagnosis for HCC screening.
AMRI protocols can streamline the workflow and substantially reduce the scanning time while maintaining an acceptable diagnostic performance compared to the complete Gd-EOB-DTPA-enhanced MRI. According to our protocols, NC-AMRI and HBP-AMRI protocols can be performed in less than 5 minutes, whereas Dyn-AMRI takes a little bit longer (6 minutes). In clinical practice, the workflow of HBP-AMRI protocol can be optimized by administering Gd-EOB-DTPA approximately 10 minutes before entering the scanning room. This time-saving process effectively shortens overall examination time and duration, minimizing patient discomfort associated with prolonged procedures while preserving clinical efficacy and improving tolerability for claustrophobic patients.
The biggest obstacle to using MRI for HCC surveillance might be the high cost in most countries, although it is frequently used in China with comparatively low expense. According to a study (50), a risk-stratified HCC surveillance strategy using MRI for higher risk groups is cost-effective and outperforms non-stratified biannual routine US. Focused MRI protocols, especially NC-AMRI, might be reasonable for high-risk patients if considering the cost-effectiveness of surveillance. Nevertheless, our preliminary experience showed there was a low loss-to-follow-up rate when the complete Gd-EOB-DTPA contrast-enhanced MRI had been employed as HCC surveillance programs. In the terms of the forthcoming Chinese standardization for diagnosis and treatment of HCC (2024 version) (51), Gd-EOB-DTPA contrast-enhanced MRI has been recommended to detect sub-centimeter HCCs (≤10 mm) for surveillance in at-risk patients. Under the condition of low cost of MRI examination and local Chinese ECCA or Gd-EOB-DTPA, we could recommend AMRI protocols surveillance in China.
There are several limitations in our study. First, the AMRI protocols were simulated from a complete Gd-EOB-DTPA contrast-enhanced MRI, which could not fully reflect the real clinical practice. Second, pathological confirmation of HCC was not possible in all patients, mostly because of the inability to obtain biopsy samples by US-guidance and low rate of surgical resection. Third, we did not compare AMRI with other screening methods, especially US, as US has a very low sensitivity and detection rate for small or micro-HCCs among most of lesions (≤3 cm) in our study. Fourth, we did not include a cost-effectiveness analysis, since there are no special charging standards for AMRI protocols in China. Fifth, the number of patients with Child-Pugh grade C included in our study was comparatively small due to inadequate image quality and impaired performance of HBP imaging associated with poor liver function. Last, the relatively higher prevalence of HCC (6.2%) in our study population may potentially lead to the overestimation of AMRI protocols. The reasons may include that the majority of patients came from central China with a high prevalence of chronic HBV infection, and approximately 90% of patients had undergone contrast-enhanced MRI as the first line for liver examination, as the expense of MRI examination and price of Chinese contrast agents (including Gd-EOB-DTPA) are dramatically lower in China than they are in other Asian countries. However, it is important to acknowledge that the results of this study would not be fully representative of the global population. To address this limitation, we aim to further refine our study design with a multi-regional investigation in the future.
Conclusions
This multi-center, prospective, blinded reader study demonstrated that AMRI protocols can be implemented in clinical practice as a patient-centered and tailored regimen for HCC surveillance/screening to support the increasing high-risk patients in China. NC-AMRI might become an optional tool for HCC surveillance due to its minimal acquisition time, lower cost, and exemption from contrast agents. Dyn-AMRI as a reliable surveillance strategy for HCC achieved the highest specificity. HBP-AMRI as a favorable alternative for HCC surveillance showed a high sensitivity and NPV while maintaining considerable specificity and NND. As the surveillance strategy based on the individual HCC risks may enable delivery of precision medicine to patients, whether surveillance with AMRI protocols would improve survival rate and clinical outcomes in high-risk patients requires further investigation. In the future, more multiregional and multi-center studies are needed to provide further evidence to allow the adoption of AMRI protocols in the updated guidelines.
Acknowledgments
Funding: This work was supported by
Footnote
Reporting Checklist: The authors have completed the STARD reporting checklist. Available at https://qims.amegroups.com/article/view/10.21037/qims-24-941/rc
Conflicts of Interest: All authors have completed the ICMJE uniform disclosure form (available at https://qims.amegroups.com/article/view/10.21037/qims-24-941/coif). The authors have no conflicts of interest to declare.
Ethical Statement: The authors are accountable for all aspects of the work in ensuring that questions related to the accuracy or integrity of any part of the work are appropriately investigated and resolved. The study was conducted in accordance with the Declaration of Helsinki (as revised in 2013). The study was approved by institutional review board of the First Affiliated Hospital of Henan University of Chinese Medicine (No. 2023HL-034-01). All participating hospitals were informed of and agreed to the study, and the requirement to obtain written informed consent was waived due to the retrospective collection of information from our patients.
Open Access Statement: This is an Open Access article distributed in accordance with the Creative Commons Attribution-NonCommercial-NoDerivs 4.0 International License (CC BY-NC-ND 4.0), which permits the non-commercial replication and distribution of the article with the strict proviso that no changes or edits are made and the original work is properly cited (including links to both the formal publication through the relevant DOI and the license). See: https://creativecommons.org/licenses/by-nc-nd/4.0/.
References
- Chacko S, Samanta S. Hepatocellular carcinoma: A life-threatening disease Biomed Pharmacother 2016;84:1679-88. [Crossref] [PubMed]
- Piñero F, Dirchwolf M, Pessôa MG. Biomarkers in Hepatocellular Carcinoma: Diagnosis, Prognosis and Treatment Response Assessment. Cells 2020;9:1370. [Crossref] [PubMed]
- Park HJ, Seo N, Kim SY. Current Landscape and Future Perspectives of Abbreviated MRI for Hepatocellular Carcinoma Surveillance. Korean J Radiol 2022;23:598-614. [Crossref] [PubMed]
- Choo SP, Tan WL, Goh BKP, Tai WM, Zhu AX. Comparison of hepatocellular carcinoma in Eastern versus Western populations. Cancer 2016;122:3430-46. [Crossref] [PubMed]
- Yokoo T, Masaki N, Parikh ND, Lane BF, Feng Z, Mendiratta-Lala M, Lee CH, Khatri G, Marsh TL, Shetty K, Dunn CT, Al-Jarrah T, Aslam A, Davenport MS, Gopal P, Rich NE, Lok AS, Singal AG. Multicenter Validation of Abbreviated MRI for Detecting Early-Stage Hepatocellular Carcinoma. Radiology 2023;307:e220917. [Crossref] [PubMed]
- Llovet JM, Fuster J, Bruix J. Intention-to-treat analysis of surgical treatment for early hepatocellular carcinoma: resection versus transplantation. Hepatology 1999;30:1434-40. [Crossref] [PubMed]
- Trevisani F, Cantarini MC, Wands JR, Bernardi M. Recent advances in the natural history of hepatocellular carcinoma. Carcinogenesis 2008;29:1299-305. [Crossref] [PubMed]
- Singal AG, Zhang E, Narasimman M, Rich NE, Waljee AK, Hoshida Y, Yang JD, Reig M, Cabibbo G, Nahon P, Parikh ND, Marrero JA. HCC surveillance improves early detection, curative treatment receipt, and survival in patients with cirrhosis: A meta-analysis. J Hepatol 2022;77:128-39. [Crossref] [PubMed]
- Xie D, Shi J, Zhou J, Fan J, Gao Q. Clinical practice guidelines and real-life practice in hepatocellular carcinoma: A Chinese perspective. Clin Mol Hepatol 2023;29:206-16. [Crossref] [PubMed]
- Son JH, Choi SH, Kim SY, Jang HY, Byun JH, Won HJ, Lee SJ, Lim YS. Validation of US Liver Imaging Reporting and Data System Version 2017 in Patients at High Risk for Hepatocellular Carcinoma. Radiology 2019;292:390-7. [Crossref] [PubMed]
- Tzartzeva K, Obi J, Rich NE, Parikh ND, Marrero JA, Yopp A, Waljee AK, Singal AG. Surveillance Imaging and Alpha Fetoprotein for Early Detection of Hepatocellular Carcinoma in Patients With Cirrhosis: A Meta-analysis. Gastroenterology 2018;154:1706-1718.e1. [Crossref] [PubMed]
- Marrero JA, Feng Z, Wang Y, Nguyen MH, Befeler AS, Roberts LR, Reddy KR, Harnois D, Llovet JM, Normolle D, Dalhgren J, Chia D, Lok AS, Wagner PD, Srivastava S, Schwartz M. Alpha-fetoprotein, des-gamma carboxyprothrombin, and lectin-bound alpha-fetoprotein in early hepatocellular carcinoma. Gastroenterology 2009;137:110-8. [Crossref] [PubMed]
- Wang C, Yuan XD, Wu N, Sun WR, Tian Y. Optimization of hepatobiliary phase imaging in gadoxetic acid-enhanced magnetic resonance imaging: a narrative review. Quant Imaging Med Surg 2023;13:1972-82. [Crossref] [PubMed]
- Kim SY, An J, Lim YS, Han S, Lee JY, Byun JH, Won HJ, Lee SJ, Lee HC, Lee YS. MRI With Liver-Specific Contrast for Surveillance of Patients With Cirrhosis at High Risk of Hepatocellular Carcinoma. JAMA Oncol 2017;3:456-63. [Crossref] [PubMed]
- Altinmakas E, Taouli B. Abbreviated Liver Magnetic Resonance Imaging Protocols and Applications. Radiol Clin North Am 2022;60:695-703. [Crossref] [PubMed]
- Vietti Violi N, Taouli B. Abbreviated MRI for HCC surveillance: is it ready for clinical use? Eur Radiol 2020;30:4147-9. [Crossref] [PubMed]
- Gupta P, Soundararajan R, Patel A, Kumar-M P, Sharma V, Kalra N. Abbreviated MRI for hepatocellular carcinoma screening: A systematic review and meta-analysis. J Hepatol 2021;75:108-19. [Crossref] [PubMed]
- Park HJ, Kim SY, Singal AG, Lee SJ, Won HJ, Byun JH, Choi SH, Yokoo T, Kim MJ, Lim YS. Abbreviated magnetic resonance imaging vs ultrasound for surveillance of hepatocellular carcinoma in high-risk patients. Liver Int 2022;42:2080-92. [Crossref] [PubMed]
- Kim DH, Yoon JH, Choi MH, Lee CH, Kang TW, Kim HA, Ku YM, Lee JM, Kim SH, Kim KA, Lee SL, Choi JI. Comparison of non-contrast abbreviated MRI and ultrasound as surveillance modalities for HCC. J Hepatol 2024;81:461-70. [Crossref] [PubMed]
- Huang DQ, Fowler KJ, Liau J, Cunha GM, Louie AL, An JY, Bettencourt R, Jung J, Gitto Z, Hernandez C, Lopez SJ, Gupta H, Sirlin CB, Marks RM, Loomba R. Comparative efficacy of an optimal exam between ultrasound versus abbreviated MRI for HCC screening in NAFLD cirrhosis: A prospective study. Aliment Pharmacol Ther 2022;55:820-7. [Crossref] [PubMed]
- Mazzaferro V, Regalia E, Doci R, Andreola S, Pulvirenti A, Bozzetti F, Montalto F, Ammatuna M, Morabito A, Gennari L. Liver transplantation for the treatment of small hepatocellular carcinomas in patients with cirrhosis. N Engl J Med 1996;334:693-9. [Crossref] [PubMed]
- International Communication Group. Imaging Tech nology Society of Chinese Medical Association. Expert con sensus on hepatobiliary specific contrast agent gadolinium-ethoxybenzyl-diethylenetriamine pentaacetic acid enhanced MRI scanning scheme. J Clin Hepatol 2020;36:519-21.
- Caraiani C, Boca B, Bura V, Sparchez Z, Dong Y, Dietrich C. CT/MRI LI-RADS v2018 vs. CEUS LI-RADS v2017-Can Things Be Put Together? Biology (Basel) 2021;10:412. [Crossref] [PubMed]
- Sutherland T, Watts J, Ryan M, Galvin A, Temple F, Vuong J, Little AF. Diffusion-weighted MRI for hepatocellular carcinoma screening in chronic liver disease: Direct comparison with ultrasound screening. J Med Imaging Radiat Oncol 2017;61:34-9. [Crossref] [PubMed]
- Vietti Violi N, Lewis S, Liao J, Hulkower M, Hernandez-Meza G, Smith K, Babb JS, Chin X, Song J, Said D, Kihira S, Sirlin CB, Reeder SB, Bashir MR, Fowler KJ, Ferket BS, Sigel K, Taouli B. Gadoxetate-enhanced abbreviated MRI is highly accurate for hepatocellular carcinoma screening. Eur Radiol 2020;30:6003-13. [Crossref] [PubMed]
- Tillman BG, Gorman JD, Hru JM, Lee MH, King MC, Sirlin CB, Marks RM. Diagnostic per-lesion performance of a simulated gadoxetate disodium-enhanced abbreviated MRI protocol for hepatocellular carcinoma screening. Clin Radiol 2018;73:485-93. [Crossref] [PubMed]
- Park HJ, Jang HY, Kim SY, Lee SJ, Won HJ, Byun JH, Choi SH, Lee SS, An J, Lim YS. Non-enhanced magnetic resonance imaging as a surveillance tool for hepatocellular carcinoma: Comparison with ultrasound. J Hepatol 2020;72:718-24. [Crossref] [PubMed]
- Whang S, Choi MH, Choi JI, Youn SY, Kim DH, Rha SE. Comparison of diagnostic performance of non-contrast MRI and abbreviated MRI using gadoxetic acid in initially diagnosed hepatocellular carcinoma patients: a simulation study of surveillance for hepatocellular carcinomas. Eur Radiol 2020;30:4150-63. [Crossref] [PubMed]
- Coenegrachts K, Delanote J, Ter Beek L, Haspeslagh M, Bipat S, Stoker J, Van Kerkhove F, Steyaert L, Rigauts H, Casselman JW. Improved focal liver lesion detection: comparison of single-shot diffusion-weighted echoplanar and single-shot T2 weighted turbo spin echo techniques. Br J Radiol 2007;80:524-31. [Crossref] [PubMed]
- Kim DH, Choi SH, Shim JH, Kim SY, Lee SS, Byun JH, Choi JI. Meta-Analysis of the Accuracy of Abbreviated Magnetic Resonance Imaging for Hepatocellular Carcinoma Surveillance: Non-Contrast versus Hepatobiliary Phase-Abbreviated Magnetic Resonance Imaging. Cancers (Basel) 2021;13:2975. [Crossref] [PubMed]
- Chandarana H, Taouli B. Diffusion and perfusion imaging of the liver. Eur J Radiol 2010;76:348-58. [Crossref] [PubMed]
- Liu JKJ, Lee CH, Tan CH. Evaluation of non-contrast magnetic resonance imaging as an imaging surveillance tool for hepatocellular carcinoma in at-risk patients. Singapore Med J 2022;63:203-8. [Crossref] [PubMed]
- Joo I, Lee JM, Lee DH, Jeon JH, Han JK. Retrospective validation of a new diagnostic criterion for hepatocellular carcinoma on gadoxetic acid-enhanced MRI: can hypointensity on the hepatobiliary phase be used as an alternative to washout with the aid of ancillary features? Eur Radiol 2019;29:1724-32. [Crossref] [PubMed]
- Cerny M, Chernyak V, Olivié D, Billiard JS, Murphy-Lavallée J, Kielar AZ, Elsayes KM, Bourque L, Hooker JC, Sirlin CB, Tang A. LI-RADS Version 2018 Ancillary Features at MRI. Radiographics 2018;38:1973-2001. [Crossref] [PubMed]
- Wei Y, Ye Z, Yuan Y, Huang Z, Wei X, Zhang T, Wan S, Tang H, He X, Song B. A New Diagnostic Criterion with Gadoxetic Acid-Enhanced MRI May Improve the Diagnostic Performance for Hepatocellular Carcinoma. Liver Cancer 2020;9:414-25. [Crossref] [PubMed]
- Khatri G, Pedrosa I, Ananthakrishnan L, de Leon AD, Fetzer DT, Leyendecker J, Singal AG, Xi Y, Yopp A, Yokoo T. Abbreviated-protocol screening MRI vs. complete-protocol diagnostic MRI for detection of hepatocellular carcinoma in patients with cirrhosis: An equivalence study using LI-RADS v2018. J Magn Reson Imaging 2020;51:415-25. [Crossref] [PubMed]
- Kim JH, Joo I, Lee JM. Atypical Appearance of Hepatocellular Carcinoma and Its Mimickers: How to Solve Challenging Cases Using Gadoxetic Acid-Enhanced Liver Magnetic Resonance Imaging. Korean J Radiol 2019;20:1019-41. [Crossref] [PubMed]
- Tanabe M, Higashi M, Iida E, Onoda H, Ihara K, Ariyoshi S, Kameda F, Miyoshi K, Furukawa M, Okada M, Ito K. Transient respiratory motion artifacts in multiple arterial phases on abdominal dynamic magnetic resonance imaging: a comparison using gadoxetate disodium and gadobutrol. Jpn J Radiol 2021;39:178-85. [Crossref] [PubMed]
- Well L, Weinrich JM, Adam G, Bannas P. Transient Severe Respiratory Motion Artifacts After Application of Gadoxetate Disodium: What We Currently Know. Rofo 2018;190:20-30. [Crossref] [PubMed]
- Pietryga JA, Burke LM, Marin D, Jaffe TA, Bashir MR. Respiratory motion artifact affecting hepatic arterial phase imaging with gadoxetate disodium: examination recovery with a multiple arterial phase acquisition. Radiology 2014;271:426-34. [Crossref] [PubMed]
- Lee JY, Huo EJ, Weinstein S, Santos C, Monto A, Corvera CU, Yee J, Hope TA. Evaluation of an abbreviated screening MRI protocol for patients at risk for hepatocellular carcinoma. Abdom Radiol (NY) 2018;43:1627-33. [Crossref] [PubMed]
- Li XQ, Wang X, Zhao DW, Sun J, Liu JJ, Lin DD, Yang G, Liu H, Xia ZY, Jia CY, Li HJ. Application of Gd-EOB-DTPA-enhanced magnetic resonance imaging (MRI) in hepatocellular carcinoma. World J Surg Oncol 2020;18:219. [Crossref] [PubMed]
- Park SH, Kim B, Kim SY, Choi SJ, Huh J, Kim HJ, Kim KW, Lee SS. Characterizing Computed Tomography-Detected Arterial Hyperenhancing-Only Lesions in Patients at Risk of Hepatocellular Carcinoma: Can Non-Contrast Magnetic Resonance Imaging Be Used for Sequential Imaging? Korean J Radiol 2020;21:280-9. [Crossref] [PubMed]
- Bruix J, Reig M, Sherman M. Evidence-Based Diagnosis, Staging, and Treatment of Patients With Hepatocellular Carcinoma. Gastroenterology 2016;150:835-53. [Crossref] [PubMed]
- Wang JH, Qiu QS, Dong SY, Chen XS, Wang WT, Yang YT, Sun W, Rao SX. Diagnostic performance of gadoxetic acid-enhanced abbreviated magnetic resonance imaging protocol in small hepatocellular carcinoma (≤2 cm) in high-risk patients. Acta Radiol 2023;64:2687-96. [Crossref] [PubMed]
- Zech CJ, Ba-Ssalamah A, Berg T, Chandarana H, Chau GY, Grazioli L, Kim MJ, Lee JM, Merkle EM, Murakami T, Ricke J. B Sirlin C, Song B, Taouli B, Yoshimitsu K, Koh DM. Consensus report from the 8th International Forum for Liver Magnetic Resonance Imaging. Eur Radiol 2020;30:370-82. [Crossref] [PubMed]
- Marks RM, Ryan A, Heba ER, Tang A, Wolfson TJ, Gamst AC, Sirlin CB, Bashir MR. Diagnostic per-patient accuracy of an abbreviated hepatobiliary phase gadoxetic acid-enhanced MRI for hepatocellular carcinoma surveillance. AJR Am J Roentgenol 2015;204:527-35. [Crossref] [PubMed]
- Renzulli M, Biselli M, Brocchi S, Granito A, Vasuri F, Tovoli F, Sessagesimi E, Piscaglia F, D'Errico A, Bolondi L, Golfieri R. New hallmark of hepatocellular carcinoma, early hepatocellular carcinoma and high-grade dysplastic nodules on Gd-EOB-DTPA MRI in patients with cirrhosis: a new diagnostic algorithm. Gut 2018;67:1674-82. [Crossref] [PubMed]
- Min JH, Kim YK, Choi SY, Jeong WK, Lee WJ, Ha SY, Ahn S, Ahn HS. Differentiation between cholangiocarcinoma and hepatocellular carcinoma with target sign on diffusion-weighted imaging and hepatobiliary phase gadoxetic acid-enhanced MR imaging: Classification tree analysis applying capsule and septum. Eur J Radiol 2017;92:1-10. [Crossref] [PubMed]
- Goossens N, Singal AG, King LY, Andersson KL, Fuchs BC, Besa C, Taouli B, Chung RT, Hoshida Y. Cost-Effectiveness of Risk Score-Stratified Hepatocellular Carcinoma Screening in Patients with Cirrhosis. Clin Transl Gastroenterol 2017;8:e101. [Crossref] [PubMed]
- Expert committee of guideline for the diagnosis and treatment of primary liver cancer (2024 edition). Guideline for the diagnosis and treatment of primary liver cancer (2024 edition). Chin J Clin Med 2024;31:277-334.