Value of two-dimensional speckle-tracking echocardiography in evaluation of cardiac function in small fetuses
Introduction
Small fetuses, defined as an estimated fetal weight (EFW) or abdominal circumference (AC) under the 10th centile should be distinguished between constitutional small for gestational age (SGA) and fetal growth-restricted (FGR) fetuses. SGA mainly defines a majority of constitutionally small but healthy fetuses at lower risk of abnormal perinatal outcomes (1). FGR, also known as intrauterine growth restriction, is a common complication of pregnancy and affects approximately 10% of pregnant women worldwide (2,3). Currently, intrauterine diagnosis of FGR remains challenging and controversial, without a gold standard. It is widely believed that the Delphi consensus can better distinguish FGR from SGA, because of the improved diagnostic specificity of FGR (4).
Various adverse intrauterine environments can lead to FGR, including placental insufficiency, maternal disease, and fetal genetic abnormalities. FGR can increase the risk of an adverse pregnancy outcome, such as premature birth, stillbirth, or a low-birthweight infant. After birth, neonates with FGR are prone to hypoglycemia, hyperbilirubinemia, hypothermia, ventricular hemorrhage, and other complications.
Furthermore, in the long term, FGR increases the risk of diseases in adulthood, especially cardiovascular disorders (5-9). Most researchers now believe that cardiovascular disease in individuals with a history of FGR is programmed before birth. Epigenetics may play an important role in the fetal programming hypothesis. According to this theory, ischemia and hypoxia can induce changes in gene expression in the fetus that allow the heart to work in a compensatory manner to maintain bodily functions in the early stages, but decompensation occurs at a later stage, leading to cardiovascular disease (10). Furthermore, it is known that the fetal heart is very sensitive to the effects of a negative intrauterine environment.
According to Delphi consensus, prenatal ultrasonography is the best way to screen for FGR. Ultrasound can assess fetal growth by measuring biparietal diameter, head circumference, AC, and femur length, combined with functional parameters, such as cerebroplacental ratio (CPR), pulsatility index of umbilical artery and uterine artery (UA-PI and UtA-PI, respectively). Nevertheless, the resulting pregnancy outcomes are difficult to predict. Ultrasound was shown to perform poorly at predicting a composite of neonatal morbidity (4). Some SGA fetuses are not growth-restricted at birth, and some fetuses that are appropriate for gestational age (AGA) do not reach their growth potential (11). Therefore, we have been interested in methods that can be used to identify changes in cardiac function in FGR and SGA and then to further determine sensitive indices that could help to predict pregnancy outcomes and guide clinical diagnosis and treatment.
Two-dimensional speckle-tracking echocardiography (2D-STE) is a novel imaging technique based on two-dimensional, high frame frequency, gray-scale images. Stable speckles in different myocardial layers can be accurately tracked owing to the high image frame rate (10). 2D-STE is being used increasingly to study not only fetal congenital heart disease (12,13), but also the effects of maternal disease on the fetal heart (14-16). However, most studies have focused on global longitudinal strain (GLS) while overlooking changes in parameters such as global radial strain (GRS) and global circumferential strain (GCS) (14-17). Furthermore, the findings for GLS assessed by 2D-STE in fetuses with FGR continue to be controversial (18,19). The aim of this study was to determine the value of 2D-STE in the assessment of the fetal heart with FGR and SGA, and to identify more sensitive indicators of pregnancy outcomes of the two conditions by using the layer-specific strain technique. We present this article in accordance with the STROBE reporting checklist (available at https://qims.amegroups.com/article/view/10.21037/qims-24-794/rc).
Methods
Population
This prospective cohort study was performed at West China Second Hospital of Sichuan University between July 2023 and December 2023. Women with singleton pregnancies who were referred to us in their second or third trimester and all the small fetuses (EFW/AC <10th centile for gestational age) were enrolled. According to the Delphi consensus, small fetuses were divided into the following categories: (I) SGA defined by EFW/AC between the 3rd and 9th centile with normal CPR, UA, and UtA doppler. (II) FGR defined by EFW/AC <3rd centile and/or EFW/AC <10th centile with either solitary (absent end-diastolic flow in the UA) or contributory parameters (UA-PI or UtA-PI >95th centile or CPR <5th centile) (1,20). Women with no underlying disease who had healthy singleton pregnancies matched for gestational age at measurement were included as controls. Cases in which labor was induced and those with incomplete clinical information, poor-quality fetal imaging, structural fetal cardiac malformations, and persistent fetal arrhythmias were excluded. After birth, the neonates were divided further into favorable and adverse pregnancy outcome groups. Composite adverse pregnant outcomes including stillbirth, preterm birth, and low-birthweight (<2,500 g) neonates. Gestational age was estimated using the parietal-rump length at the time of nuchal translucency testing in early pregnancy (21). EFW and EFW z-score were calculated using the Hadlock formula (22).
The study was conducted in accordance with the Declaration of Helsinki (as revised in 2013) and was approved by the Institutional Review Board (IRB) of West China Second Hospital, Sichuan University (No. 2022-297). All pregnant women provided both verbal and written informed consent.
Study protocol
Baseline information, including maternal age, underlying diseases, gestational history, gestational age, mode of delivery, pregnancy outcome, and fetal birth weight, was recorded for all enrolled women.
A Philips EPIQ 7C ultrasound diagnostic instrument (Philips Healthcare, Amsterdam, Netherlands) with a C5-1 probe (frequency 1–5 MHz) was used for echocardiographic evaluation of the fetuses. All enrolled cases were examined by experienced fetal cardiac sonographers following the International Society of Ultrasound in Obstetrics and Gynecology (ISUOG) guidelines for examination of the fetal heart (23). Subsequently, a suitable four-chamber heart section was selected to clearly display the endocardium; the focus, depth, and sector range were adjusted to achieve an image frame rate higher than 90 frames/minute, and 3–5 cardiac cycle images were stored in the absence of significant fetal motion. After exporting the stored images in DICOM format, the images were analyzed using an offline fetal heart software program (2D Cardiac Performance Analysis Fetal; TomTec Imaging Systems GmbH, Munich, Germany) which uses M-mode ultrasound model features to identify the beginning and end of the cardiac cycle. Based on the M-model curve, our team identified two consecutive end-diastolic time periods as one cardiac cycle. Using this method, the examiner selects three specific anatomical landmarks in the diastolic four-chamber heart view to automatically identify the endocardium based on the software schematic, as well as both sides of the mitral valve and at the apex, and then adjusts the width of the region of interest based on the left ventricular wall thickness. After identifying the region of interest and starting the program, the software automatically tracks the endocardial and myocardial displacements and measures left ventricular systolic function and strain parameters, including ejection fraction (EF), myocardial global circumferential strain (myoGCS), myocardial global longitudinal strain (myoGLS), endocardial global circumferential strain (endoGCS), endocardial global longitudinal strain (endoGLS), and GRS. In this study, all cardiac parameters were averaged over two consecutive cardiac cycles. All cardiac parameters and baseline characteristics were compared between the FGR, SGA, and control group fetuses; multiple comparisons were made if statistically significant.
Doppler measurements of MCA and UA were performed according to the standard recommendations of ISUOG practice guidelines (11). As previously described, CPR was calculated as the ratio between MCA-PI and UA-PI. The cardiac and doppler parameters were compared between the favorable and adverse pregnancy outcome groups.
The images for 10 patients were randomly selected and analyzed independently by two observers to assess interobserver variability in measurements. The same images were re-analyzed by the same observers two weeks later to evaluate intraobserver variability.
Statistical analysis
All the measured data were tested for homogeneity of variance and normality. Quantitative data that were normally distributed are shown as the mean ± standard deviation. Analysis of variance was used to compare the means of multiple samples. Quantitative data that were not normally distributed are shown as the median and were compared between groups using the Kruskal-Wallis test. Counting data were examined using the Chi-squared test. The correlation analysis of bivariables was performed by Pearson test. Regression analysis was used in multi-index combined tests. After accounting for ultrasound indices, a receiver operating characteristic (ROC) curve analysis was carried out. Repeatability is expressed by the intraclass correlation coefficient (>0.8, excellent; >0.6 to 0.8, good). The statistical analysis was performed using the software SPSS 23.0 (IBM Corp., Armonk, NY, USA). All comparisons were performed at 5% significance level and presented with corresponding 95% confidence interval (CI).
Results
Initially, 71 small fetuses were included, 10 of whom were excluded because of induction of labor (n=3), incomplete information (n=2), poor image quality (n=2), intracardiac structural malformation (n=2), and persistent arrhythmia (n=1), leaving 61 eligible cases, including 32 FGR and 29 SGA. A total of 34 gestational age-matched healthy fetuses comprised the control group. After birth, 22 FGR and 5 SGA were assigned to the adverse pregnancy outcome group (stillbirth, n=2; premature, n=10; low-birthweight neonates, n=15), whereas 10 FGR and 24 SGA were allocated to the favorable group (Figure 1).
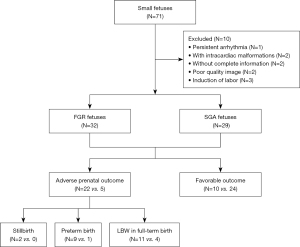
Baseline characteristics
The baseline characteristics of the study groups are shown in Table 1. There was no significant between-group difference in maternal age, gestational age, maternal body mass index, parity, or fetal sex at the time of collection of the fetal heart images (P>0.05). Compared with control group, vaginal birth was less common in the FGR and SGA group (P<0.05). Delivery was significantly earlier in the FGR group than in the SGA group and the control group (P<0.05). There were statistically significant differences in EFW, EFW z-score, and birth weight between the groups (P<0.05). Only two fetuses’ Apgar score<7; there was no significant difference in Apgar score (P>0.05). Maternal diabetes was more common in the FGR group than in the control group (P<0.05).
Table 1
Characteristic | Small fetuses (n=61) | Control group (n=34) | P value | |
---|---|---|---|---|
FGR (n=32) | SGA (n=29) | |||
Maternal characteristics | ||||
Maternal age (years) | 31.0±4.0 | 30.4±3.7 | 30.2±3.5 | 0.646 |
BMI at inclusion (kg/m2) | 23.9±1.2 | 24.2±1.0 | 24.7±1.5 | 0.175 |
GA at US (weeks) | 34±3 | 34±2 | 34±3 | 0.168 |
Primiparity | 21 (65.6) | 20 (68.9) | 23 (67.6) | 0.961 |
Underlying disease | ||||
Gestational diabetes | 8 (25.0) | 3 (10.3) | 0† | <0.05 |
Pre-eclampsia | 4 (12.5) | 3 (10.3) | 0 | 0.116 |
Hematologic diseases | 4 (12.5) | 2 (6.9) | 0 | 0.112 |
Fetal characteristics | ||||
EFW at US (g) | 1,730.7±490.0 | 2,080.3±410.0* | 2,377.1±548.7†‡ | <0.05 |
EFW z-score at US | −2.5±1.6 | −1.4±1.0* | 0.8±1.3†‡ | <0.05 |
UA-PI | 1.12±0.30 | 0.87±0.15* | 0.87±0.11† | <0.05 |
Mode of delivery | ||||
Vaginal birth | 14 (43.8) | 15 (51.7) | 26 (76.5)†‡ | <0.05 |
Neonatal characteristics | ||||
GA at delivery (weeks) | 36±3 | 39±1* | 39±1† | <0.05 |
Birth weight (g) | 2,046.1±7,107 | 2,869.6±393.4* | 3,388.0±449.8†‡ | <0.05 |
Female | 14 (43.8) | 16 (55.2) | 15 (44.1) | 0.6 |
Apgar <7 (%) | 2 (6.3) | 0 | 0 | 0.134 |
Data are presented as mean ± standard deviation or n (%). *, P<0.05, FGR group vs. SGA group; †, P<0.05, FGR group vs. control group; ‡, P<0.05, SGA group vs. control group. FGR, fetal growth-restricted; SGA, small for gestational age; BMI, body mass index; GA, gestation age; US, ultrasound; EFW, estimated fetal weight; UA-PI, pulsatility index of umbilical artery.
2D-STE findings in the FGR, SGA, and control groups
The findings for left ventricular systolic function and strain parameters in the three study groups are shown in Table 2 and Figure 2. There were statistically significant differences in myoGLS and endoGLS between the three groups (P<0.05), with gradual increases in their absolute values. EF, myoGCS, endoGCS, and GRS were significantly lower in the FGR group than in the SGA and control groups (P<0.05).
Table 2
Parameters | Small fetuses (n=61) | Control group (n=34) | P value | |
---|---|---|---|---|
FGR (n=32) | SGA (n=29) | |||
EF (%) | 44.85±10.04 | 53.28±6.70* | 55.46±6.94† | <0.05 |
myoGCS (%) | −11.89±4.24 | −14.42±4.31* | −14.93±4.77† | <0.05 |
myoGLS (%) | −9.26±4.24 | −12.63±4.11* | −16.15±3.84†‡ | <0.05 |
endoGCS (%) | −22.68±6.24 | −27.29±7.25* | −27.69±6.46† | <0.05 |
endoGLS (%) | −13.53±6.67 | −17.71±5.58* | −20.55±4.42†‡ | <0.05 |
GRS (%) | 24.98±12.44 | 33.37±9.33* | 36.87±12.69† | <0.05 |
Data are presented as mean ± standard deviation. *, P<0.05, FGR group vs. SGA group; †, P<0.05, FGR group vs. control group; ‡, P<0.05, SGA group vs. control group. FGR, fetal growth-restricted; SGA, small for gestational age; EF, ejection fraction; myoGCS, myocardial global circumferential strain; myoGLS, myocardial global longitudinal strain; endoGCS, endocardial global circumferential strain; endoGLS, endocardial global longitudinal strain; GRS, global radial strain.
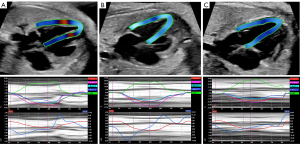
2D-STE findings and Doppler parameters between the adverse pregnancy outcome group and favorable group of small fetuses
The findings for left ventricular parameters and Doppler parameters between the adverse pregnancy outcome group and favorable group of small fetuses are shown in Table 3. Compared with the favorable group, the absolute values of myoGLS, endoGLS, and UA-PI were decreased in the adverse pregnancy outcome group (P<0.05). There was no significant difference in EF, myoGCS, endoGCS, GRS, MCA-PI, or CPR between the adverse pregnancy outcome group and favorable group (P>0.05).
Table 3
Parameters | Adverse outcome group (n=27) | Favorable group (n=34) | P value |
---|---|---|---|
EF (%) | 49.05±8.58 | 52.12±7.38 | 0.137 |
myoGCS (%) | −13.88±4.47 | −13.72±4.59 | 0.896 |
myoGLS (%) | −9.79±3.79 | −12.30±4.20* | 0.018 |
endoGCS (%) | −26.38±7.02 | −25.82±6.41 | 0.746 |
endoGLS (%) | −13.71±6.37 | −17.64±5.30* | 0.011 |
GRS (%) | 27.17±12.43 | 29.85±12.48* | 0.046 |
UA-PI | 1.10±0.33 | 0.93±0.18* | 0.016 |
MCA-PI | 1.55±0.53 | 1.52±0.58 | 0.795 |
CPR | 1.38±0.53 | 1.69±0.61 | 0.124 |
The data are presented as mean ± standard deviation. *, P<0.05, adverse outcome group vs. favorable group. EF, ejection fraction; myoGCS, myocardial global circumferential strain; myoGLS, myocardial global longitudinal strain; endoGCS, endocardial global circumferential strain; endoGLS, endocardial global longitudinal strain; GRS, global radial strain; UA-PI, pulsatility index of umbilical artery; MCA-PI, pulsatility index of mean cerebral artery; CPR, cerebroplacental ratio.
The predictive value of endoGLS and UA-PI for pregnancy outcome
The respective areas under the curve (AUC) were 0.66 for myoGLS (P<0.05, 95% CI: 0.52–0.80), 0.69 for endoGLS (P<0.05, 95% CI: 0.56–0.83), and 0.61for UA-PI (P>0.05, 95% CI: 0.56–0.76). At fixed specificity, the respective cutoff values of myoGLS and endoGLS for predicting an adverse pregnancy outcome were −7.69 (sensitivity 33.3%, specificity 91.2%) and −12.01 (sensitivity 44.4%, specificity 91.2%), respectively. The combination of endoGLS and UA-PI as a new predictor yielded a higher ROC curve than that of either one alone (P<0.05, AUC =0.75, 95% CI: 0.62–0.87) (Figure 3).
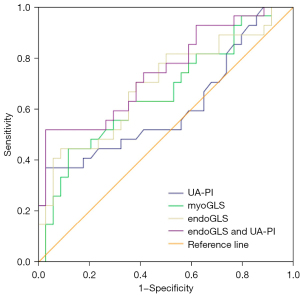
Correlation analysis of endoGLS with EFW, EFW z-score, and UA-PI in fetuses
The values of endoGLS for all enrolled fetuses had a low correlation with EFW (r=−0.231, P<0.05) and UA-PI (r=0.329, P<0.05), and had a moderate correlation with EFW z-score (r=−0.458, P<0.05), (as shown in Figure 4).
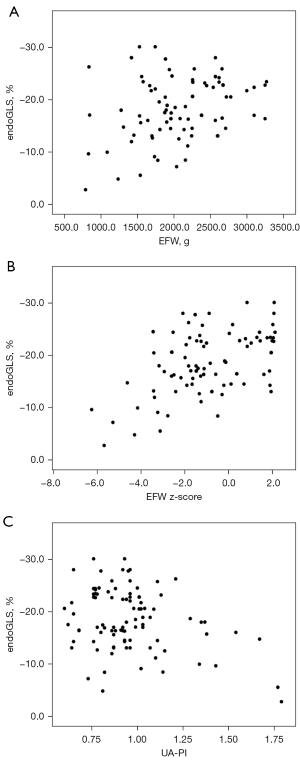
Repeatability and reliability
The results of the interobserver and intraobserver agreement analyses for EF, myoGCS, myoGLS, endoGLS, and GRS indicated good repeatability and reliability, yet those for endoGCS did not (Table 4).
Table 4
Parameters | Intra-observer | Inter-observer | |||
---|---|---|---|---|---|
ICC | 95% CI | ICC | 95% CI | ||
EF (%) | 0.88 | 0.60 to 0.97 | 0.72 | 022 to 0.92 | |
myoGCS (%) | 0.72 | 0.21 to 0.92 | 0.66 | 0.09 to 0.90 | |
myoGLS (%) | 0.72 | 0.20 to 0.92 | 0.66 | 0.10 to 0.90 | |
endoGCS (%) | 0.69 | 0.15 to 0.91 | 0.52 | −0.13 to 0.85 | |
endoGLS (%) | 0.70 | 0.16 to 0.92 | 0.66 | 0.10 to 0.90 | |
GRS (%) | 0.85 | 0.52 to 0.96 | 0.70 | 0.17 to 0.92 |
ICC, intraclass correlation coefficient; CI, confidence interval; EF, ejection fraction; myoGCS, myocardial global circumferential strain; myoGLS, myocardial global longitudinal strain; endoGCS, endocardial global circumferential strain; endoGLS, endocardial global longitudinal strain; GRS, global radial strain.
Discussion
FGR can be caused by a variety of negative events during pregnancy, the most important of which is placental insufficiency, which can lead to fetal malnutrition, hypoxia, and abnormalities in fetal cardiac preload and afterload (24). Current research suggests that disease in adult offspring is programmed early in life and is referred to as “developmental origin of health and disease” theory; that is, during embryonic development, negative environmental factors in the uterus create susceptibility factors for disease in the offspring and initiate programmed disease during adulthood (25). Therefore, it is important to identify changes in fetal cardiac function in cases of FGR and indicators that can predict adverse pregnancy outcomes of small fetuses at an early stage in order to better inform clinical diagnosis and treatment.
2D-STE is a novel, non-invasive, quantitative method for evaluation of cardiac function that can track the motion of the myocardium automatically and obtain deformation parameters. Compared with traditional echocardiography, 2D-STE has lower angle dependence and is less susceptible than Doppler ultrasound to fetal and maternal movement (26), allowing for more accurate detection of changes in fetal heart function. Several groups have used 2D-STE to investigate FGR-associated changes in the fetal heart, but their findings are controversial (18). Therefore, in this study, we used 2D-STE for further investigation of cardiac function in fetuses with FGR. At present, GLS is most commonly used in research on fetal cardiac function, and few in-depth studies have used parameters such as GCS and GRS. Moreover, there is limited research on layer-specific strain in the fetal heart with FGR. Therefore, with updating of the Tomtec software, our team paid attention to not only GLS but also GCS and GRS in fetal hearts with FGR. Furthermore, layer-specific strain technology was used to analyze layer-specific strain, including myocardial and endocardial GLS and GCS, in order to collect more information on myocardial strain in cases of FGR.
According to Delphi consensus, small fetuses with EFW or AC below the 10th percentile for gestational age were divided into FGR and healthy SGA groups in our study. We found that the absolute values of EF, myoGCS, myoGLS, endoGLS, endoGCS, and GRS were significantly lower in the FGR group than in the SGA group and in the control group, indicating that an adverse intrauterine environment can lead to change the pressure or volume load in the FGR heart, causing abnormalities in fetal cardiac function and structural remodeling. These changes in cardiac function might in turn contribute to distinguishing between FGR and SGA. Compared with the control group, we also found that the myoGLS and endoGLS had changed in SGA. Therefore, we should not only pay attention to the injured cardiac function of FGR function, but also monitor the cardiac change of SGA. It is necessary to establish a long-term follow-up method to observe the correlation between the quantitative indicators of the fetal heart and the occurrence of cardiovascular diseases in FGR and SGA fetuses, which require further research.
Studies have shown that FGR is the second leading cause of perinatal death and is responsible for 30% of stillbirths (27). Preterm and low-birthweight (<2,500 g after birth) neonates are at increased risk of disease in adulthood (28-30). Therefore, we roughly defined stillbirths, preterm, and low-birthweight after birth into adverse pregnancy outcomes, including 22 FGR and 5 SGA neonates. It was also reflected that Delphi consensus had some limitations in the prediction of adverse pregnancy outcomes (4). In addition to the commonly used UA-PI, CPR, and other Doppler parameters, we tried to find new indicators to predict adverse pregnancy outcomes. Compared with the favorable group, we found that the absolute value of myoGLS and endoGLS had decreased in the adverse pregnancy outcomes group. EF, as a conventional indicator of left ventricular systolic function, had no change between the adverse pregnancy outcomes group and the favorable group, as well as endoGCS, myoGCS, and GRS. We also found that the values of endoGLS were much more associated to fetal weight deficit than they were with fetal weight. These findings are consistent with a previous report indicating that GLS is more stable and sensitive than other strain parameters (31), allowing better risk stratification.
Layer-specific strain is a recent development in strain analysis which can quantify deformation in each layer of the heart. It is also a sensitive indicator of cardiac dysfunction (32-36). In view of the lack of layer-specific analyses of the fetal heart, we referenced previous analyses of myocardial layer-specific strain in children and adults. One study demonstrated that endoGLS was a particularly sensitive test in most types of ischemic heart disease (36), and another found that myoGLS was a better predictor of the cardiac prognosis (37,38). However, in our study, comparison of AUCs indicated that endoGLS and myoGLS were virtually similar (AUC of 0.69 vs. 0.66), yet both were rather poor predictors of pregnancy outcomes. We focused on endoGLS and found that endoGLS and UA-PI were lowly correlated, yet when endoGLS and UA-PI were combined as a new predictor, the ROC curve exhibited a considerably higher AUC (0.75) than myoGLS and endoGLS alone, which might contribute to predict the pregnancy outcome of small fetuses.
To our knowledge, this is the first study to prospectively analyze left heart function in fetuses with FGR using the layer-specific strain technique in 2D-STE. However, this study has some limitations. First, it was performed at a single center and had a small sample size. Therefore, the possibility of selection bias cannot be excluded, and a larger, multicenter prospective cohort study is needed to confirm our findings. Second, prenatal diagnosis of FGR is still challenging. For example, our diagnostic criteria for FGR may classify some FGR fetuses as SGA fetuses, which may have influenced our results.
Conclusions
This study showed that layer-specific strain technology in 2D-STE is a sensitive tool that can be used to evaluate left ventricular myocardial deformation in the fetal heart with small fetuses, and identify the changes of cardiac function between FGR and SGA. MyoGLS and endoGLS seems more fairly sensitive to evaluate cardiac function in fetuses. Moreover, the combination of endoGLS and UA-PI as a new parameter may have better performance in predicting pregnancy outcome of small fetuses.
Acknowledgments
Funding: This work was supported by
Footnote
Reporting Checklist: The authors have completed the STROBE reporting checklist. Available at https://qims.amegroups.com/article/view/10.21037/qims-24-794/rc
Conflicts of Interest: All authors have completed the ICMJE uniform disclosure form (available at https://qims.amegroups.com/article/view/10.21037/qims-24-794/coif). The authors have no conflicts of interest to declare.
Ethical Statement: The authors are accountable for all aspects of the work in ensuring that questions related to the accuracy or integrity of any part of the work are appropriately investigated and resolved. The study was conducted in accordance with the Declaration of Helsinki (as revised in 2013) and was approved by the IRB of West China Second Hospital, Sichuan University (No. 2022-297). All pregnant women provided both verbal and written informed consent.
Open Access Statement: This is an Open Access article distributed in accordance with the Creative Commons Attribution-NonCommercial-NoDerivs 4.0 International License (CC BY-NC-ND 4.0), which permits the non-commercial replication and distribution of the article with the strict proviso that no changes or edits are made and the original work is properly cited (including links to both the formal publication through the relevant DOI and the license). See: https://creativecommons.org/licenses/by-nc-nd/4.0/.
References
- Gordijn SJ, Beune IM, Thilaganathan B, Papageorghiou A, Baschat AA, Baker PN, Silver RM, Wynia K, Ganzevoort W. Consensus definition of fetal growth restriction: a Delphi procedure. Ultrasound Obstet Gynecol 2016;48:333-9. [Crossref] [PubMed]
- Lopez-Tello J, Arias-Alvarez M, Gonzalez-Bulnes A, Sferuzzi-Perri AN. Models of Intrauterine growth restriction and fetal programming in rabbits. Mol Reprod Dev 2019;86:1781-809. [Crossref] [PubMed]
- Black RE. Global Prevalence of Small for Gestational Age Births. Nestle Nutr Inst Workshop Ser 2015;81:1-7. [Crossref] [PubMed]
- Roeckner JT, Pressman K, Odibo L, Duncan JR, Odibo AO. Outcome-based comparison of SMFM and ISUOG definitions of fetal growth restriction. Ultrasound Obstet Gynecol 2021;57:925-30. [Crossref] [PubMed]
- Reynolds LP, Borowicz PP, Caton JS, Crouse MS, Dahlen CR, Ward AK. Developmental Programming of Fetal Growth and Development. Vet Clin North Am Food Anim Pract 2019;35:229-47. [Crossref] [PubMed]
- Seremak-Mrozikiewicz A, Barlik M, Drews K. Fetal programming as a cause of chronic diseases in adult life. Ginekol Pol 2014;85:43-8. [Crossref] [PubMed]
- Alexander BT, Dasinger JH, Intapad S. Fetal programming and cardiovascular pathology. Compr Physiol 2015;5:997-1025. [Crossref] [PubMed]
- Drever N, Saade GR, Bytautiene E. Fetal programming: Early-life modulations that affect adult outcomes. Curr Allergy Asthma Rep 2010;10:453-9. [Crossref] [PubMed]
- Rasmussen JM, Thompson PM, Entringer S, Buss C, Wadhwa PD. Fetal programming of human energy homeostasis brain networks: Issues and considerations. Obes Rev 2022;23:e13392. [Crossref] [PubMed]
- Marciniak A, Patro-Małysza J, Kimber-Trojnar Ż, Marciniak B, Oleszczuk J, Leszczyńska-Gorzelak B. Fetal programming of the metabolic syndrome. Taiwan J Obstet Gynecol 2017;56:133-8. [Crossref] [PubMed]
- Salomon LJ, Alfirevic Z, Da Silva Costa F, Deter RL, Figueras F, Ghi T, Glanc P, Khalil A, Lee W, Napolitano R, Papageorghiou A, Sotiriadis A, Stirnemann J, Toi A, Yeo G. ISUOG Practice Guidelines: ultrasound assessment of fetal biometry and growth. Ultrasound Obstet Gynecol 2019;53:715-23. [Crossref] [PubMed]
- Song X, Cao H, Hong L, Zhang L, Li M, Shi J, Liu J, Ma J, Cui L, Zhang Y, Li Y, Lv Q, Xie M. Ventricular Myocardial Deformation in Fetuses With Tetralogy of Fallot: A Necessary Field of Investigation. Front Cardiovasc Med 2021;8:764676. [Crossref] [PubMed]
- Luo L, Liu H, Zhou S, Zhao F, Zhu Q, Guo N, Chen J. Quantitative evaluation of fetal ventricular function by speckle tracking echocardiography. Echocardiography 2021;38:1924-31. [Crossref] [PubMed]
- Wang D, Liu C, Liu X, Zhang Y, Wang Y. Evaluation of prenatal changes in fetal cardiac morphology and function in maternal diabetes mellitus using a novel fetal speckle-tracking analysis: a prospective cohort study. Cardiovasc Ultrasound 2021;19:25. [Crossref] [PubMed]
- Orabona R, Mohseni Z, Sciatti E, Mulder EG, Prefumo F, Lorusso R, Frusca T, Ghossein-Doha C, Spaanderman MEA. Maternal myocardial dysfunction after normotensive fetal growth restriction compared with hypertensive pregnancies: a speckle-tracking study. J Hypertens 2020;38:1955-63. [Crossref] [PubMed]
- Duan S, Ha S, Li S, Wang Y, Shi Y, Zhao H, Zhang L, Zhang X, Wang Y. Evaluation of cardiac function and systolic dyssynchrony of fetuses exposed to maternal autoimmune diseases using speckle tracking echocardiography. Clin Rheumatol 2021;40:3807-15. [Crossref] [PubMed]
- Domínguez-Gallardo C, Ginjaume-García N, Ullmo J, Fernández-Oliva A, Parra J, Vázquez A, Cruz-Lemini M, Llurba E. Longitudinal Behavior of Left-Ventricular Strain in Fetal Growth Restriction. Diagnostics (Basel) 2023;13:1252. [Crossref] [PubMed]
- van Oostrum NHM, Derks K, van der Woude DAA, Clur SA, Oei SG, van Laar JOEH. Two-dimensional Speckle tracking echocardiography in Fetal Growth Restriction: a systematic review. Eur J Obstet Gynecol Reprod Biol 2020;254:87-94. [Crossref] [PubMed]
- DeVore GR, Gumina DL, Hobbins JC. Assessment of ventricular contractility in fetuses with an estimated fetal weight less than the tenth centile. Am J Obstet Gynecol 2019;221:498.e1-498.e22. [Crossref] [PubMed]
- Figueras F, Gratacós E. Update on the diagnosis and classification of fetal growth restriction and proposal of a stage-based management protocol. Fetal Diagn Ther 2014;36:86-98. [Crossref] [PubMed]
- Robinson HP, Sweet EM, Adam AH. The accuracy of radiological estimates of gestational age using early fetal crown-rump length measurements by ultrasound as a basis for comparison. Br J Obstet Gynaecol 1979;86:525-8. [Crossref] [PubMed]
- Hadlock FP, Harrist RB, Sharman RS, Deter RL, Park SK. Estimation of fetal weight with the use of head, body, and femur measurements--a prospective study. Am J Obstet Gynecol 1985;151:333-7. [Crossref] [PubMed]
- International Society of Ultrasound in Obstetrics and Gynecology. Carvalho JS, Allan LD, Chaoui R, Copel JA, DeVore GR, Hecher K, Lee W, Munoz H, Paladini D, Tutschek B, Yagel S. ISUOG Practice Guidelines (updated): sonographic screening examination of the fetal heart. Ultrasound Obstet Gynecol 2013;41:348-59. [Crossref] [PubMed]
- Crispi F, Crovetto F, Gratacos E. Intrauterine growth restriction and later cardiovascular function. Early Hum Dev 2018;126:23-7. [Crossref] [PubMed]
- Joss-Moore LA, Lane RH. The developmental origins of adult disease. Curr Opin Pediatr 2009;21:230-4. [Crossref] [PubMed]
- Day TG, Charakida M, Simpson JM. Using speckle-tracking echocardiography to assess fetal myocardial deformation: are we there yet? Ultrasound Obstet Gynecol 2019;54:575-81. [Crossref] [PubMed]
- Nardozza LM, Caetano AC, Zamarian AC, Mazzola JB, Silva CP, Marçal VM, Lobo TF, Peixoto AB, Araujo Júnior E. Fetal growth restriction: current knowledge. Arch Gynecol Obstet 2017;295:1061-77. [Crossref] [PubMed]
- Kuo AH, Li C, Huber HF, Clarke GD, Nathanielsz PW. Intrauterine growth restriction results in persistent vascular mismatch in adulthood. J Physiol 2018;596:5777-90. [Crossref] [PubMed]
- Ushida T, Cotechini T, Protopapas N, Atallah A, Collyer C, Toews AJ, Macdonald-Goodfellow SK, Tse MY, Winn LM, Pang SC, Adams MA, Othman M, Kotani T, Kajiyama H, Graham CH. Aberrant inflammation in rat pregnancy leads to cardiometabolic alterations in the offspring and intrauterine growth restriction in the F2 generation. J Dev Orig Health Dis 2022;13:706-18. [Crossref] [PubMed]
- Li P, He L, Lan Y, Fang J, Fan Z, Li Y. Intrauterine Growth Restriction Induces Adulthood Chronic Metabolic Disorder in Cardiac and Skeletal Muscles. Front Nutr 2022;9:929943. [Crossref] [PubMed]
- Negishi T, Negishi K. How to standardize measurement of global longitudinal strain. J Med Ultrason (2001) 2022;49:45-52. [Crossref] [PubMed]
- Opie LH, Commerford PJ, Gersh BJ, Pfeffer MA. Controversies in ventricular remodelling. Lancet 2006;367:356-67. [Crossref] [PubMed]
- Hamada S, Schroeder J, Hoffmann R, Altiok E, Keszei A, Almalla M, Napp A, Marx N, Becker M. Prediction of Outcomes in Patients with Chronic Ischemic Cardiomyopathy by Layer-Specific Strain Echocardiography: A Proof of Concept. J Am Soc Echocardiogr 2016;29:412-20. [Crossref] [PubMed]
- Yazaki K, Takahashi K, Shigemitsu S, Yamada M, Iso T, Kobayashi M, Akimoto K, Tamaichi H, Fujimura J, Saito M, Nii M, Shimizu T. In-Depth Insight Into the Mechanisms of Cardiac Dysfunction in Patients With Childhood Cancer After Anthracycline Treatment Using Layer-Specific Strain Analysis. Circ J 2018;82:715-23. [Crossref] [PubMed]
- Becker M, Ocklenburg C, Altiok E, Füting A, Balzer J, Krombach G, Lysyansky M, Kühl H, Krings R, Kelm M, Hoffmann R. Impact of infarct transmurality on layer-specific impairment of myocardial function: a myocardial deformation imaging study. Eur Heart J 2009;30:1467-76. [Crossref] [PubMed]
- Jiang Z, Zhang M, Qin Y, Li Y, Lu X. Usefulness of layer-specific strain for evaluating and predicting recovery of left ventricular myocardial function in patients undergoing hybrid coronary revascularization. Int J Cardiovasc Imaging 2023;39:491-9. [Crossref] [PubMed]
- Hagemann CA, Hoffmann S, Hagemann RA, Fritz-Hansen T, Olsen FJ, Jørgensen PG, Biering-Sørensen T. Usefulness of layer-specific strain in diagnosis of coronary artery disease in patients with stable angina pectoris. Int J Cardiovasc Imaging 2019;35:1989-99. [Crossref] [PubMed]
- Grove GL, Pedersen S, Olsen FJ, Skaarup KG, Jørgensen PG, Shah AM, Biering-Sørensen T. Layer-specific global longitudinal strain obtained by speckle tracking echocardiography for predicting heart failure and cardiovascular death following STEMI treated with primary PCI. Int J Cardiovasc Imaging 2021;37:2207-15. [Crossref] [PubMed]