Diffusion-derived vessel density (DDVD) for penumbra delineation in brain acute ischemic stroke: initial proof-of-concept results using single NEX DWI
Introduction
Stroke is a major cause of death and a leading cause of disability globally. Neuroimaging, including non-contrast computed tomography (CT), magnetic resonance imaging (MRI), vascular and perfusion imaging, is a cornerstone in the diagnosis and treatment decision-making. A rapid and accurate diagnosis is the key, specifically for time-critical reperfusion therapies. Identification of medium-large vessel occlusions by CT or MR angiography with additional perfusion imaging helps identify salvageable brain tissue (the ‘penumbra’) in patients who are likely to benefit from reperfusion therapies. Successful reperfusion of salvageable penumbra is expected to reduce the extent of ischemic stroke and thus improve clinical outcomes, whereas reperfusion of irreversibly affected brain tissue is thought to be futile and may result in harm due to the risk of hemorrhagic transformation (1,2). For neuroimaging work-up of acute stroke, CT is more commonly applied than MRI, due to the wider availability in emergency settings. However, CT is associated with administration of iodinated contrast agent and involves variable dosages of local ionizing radiation. Moreover, the CT perfusion readout threshold to define the infarct area remains controversial (3-5). On the other hand, MRI has the overall advantage of better tissue contrast, and the capability to acquire diffusion-weighted imaging (DWI) which remains the best imaging modality to define the infarct area (2,6,7). MRI diffusion imaging provides a reference standard for the presence of ischemia, core size, and penumbral tissue estimation. MRI also offers an added advantage of identification of smaller ischemic areas that may escape detection in early CT or CT perfusion, while ischemic core estimates using CT perfusion sometimes do not include the full extent of MR diffusion restriction (2).
Our exploration of DWI in liver fibrosis evaluation revealed that diffusion-derived vessel density (DDVD) could potentially reflect microvascular changes. This finding inspired us to investigate DDVD’s application in brain acute ischemic stroke, particularly in the delineation of the penumbra area, where timely intervention is critical. We noted that for spin-echo type echo-planar sequence (EPI) we can take the assumption that the second motion probing gradient after the 180-degree RF pulse cannot fully re-focus the flowing spins in vessel and micro-vessels after being de-phased by the first motion probing gradient before the 180-degree RF pulse (Figure S1). Therefore, liver blood vessels show high signal when there is no motion probing gradient (b=0 s/mm2) and low signal when even very low b-values (such as b=1, b=2) are applied (Figure 1) (9). Thus, the signal difference between images when the motion probing gradient is ‘off’ and ‘on’ reflects the extent of tissue vessel density, and we term this as DDVD (diffusion derived vessel density). DDVD is derived from the Eq. [1] (9):
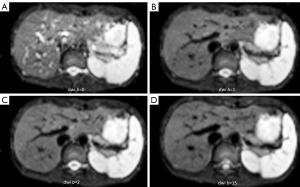
where ROIarea0 and ROIarea2 refer to the number of pixels in the selected region-of-interest (ROI) on b=0 and b=2 DWI, respectively. Sb0 refers to the measured total signal intensity within the ROI when b=0, and Sb2 refers to the measured total signal intensity within the ROI when b=2, thus Sb/ROIarea equates to the mean signal intensity within the ROI. Sb2 and ROIarea2 can also be approximated by other low b-values (such as b=10) DWI. If we consider a pixel is an individual ROI, DDVD pixelwise map (DDVDm) can be constructed pixel-by-pixel with this same principle (10). In our initial testing, with 20 healthy livers, 11 stage-1 fibrotic livers, and 5 stage-4 fibrotic livers, DDVD (arbitrary unit/pixel) was 26.5 for healthy livers, 21.8 for stage-1 fibrotic livers, and 12.1 for stage-4 fibrotic livers (9).
The analysis of DDVD requires only two b-values (with one being b=0 s/mm2 and the other being non-zero low b-value), with a significantly shorter scanning time than contrast enhanced CT/MRI while without the need of a contrast agent injection. DDVD is conceptually as simple as apparent diffusion coefficient (ADC). DDVD measure based on this simple principle appears to be useful as a straightforward imaging biomarker in diverse clinical scenarios. Huang et al. (11) showed that DDVD analysis demonstrates liver parenchyma has an age-dependent decrease of micro-perfusion in healthy women. This agrees with the known physiological age-dependent reduction in liver blood flow which has been well documented using a variety of technical methods including histology, dye dilution, indicator clearance. DDVD is a useful parameter for distinguishing of livers with and without fibrosis, and livers with severer fibrosis tend to have even lower DDVD measurements than those with milder liver fibrosis (9,12,13). With DDVD analysis, Zheng et al. (14) demonstrated that per unit micro-circulation of spleen is decreased in viral hepatitis-b liver fibrosis patients. This is consistent with, for example, the report of Gitlin et al. (15) with analysis of the washout curves of Xenon 133 injected in the splenic artery in patients with liver cirrhosis and portal hypertension. Among the patients, splenic blood flow, expressed as ml per 100 g of splenic tissue, was decreased. On the contrary, total splenic blood flow, calculated by multiplying specific splenic flow by spleen volume, was increased (15). He et al. (16) reported that placenta DDVD as a perfusion biomarker allows excellent separation of normal and early preeclampsia pregnancies. Lu et al. (17) reported that placenta regional DDVD is significantly higher in pregnant women with placenta accreta spectrum disorders than women with normal placenta, and especially higher in patients with placenta increta and percreta. Hu et al. (18) described that liver hemangiomas can be mostly differentiated from liver mass-forming lesions (hepatocellular carcinomas and focal nodular hyperplasia) solely based on DDVDm.
As absolute MR signal intensity is influenced by various factors, including B0/B1 spatial inhomogeneity, coil loading, receiver gain, etc., we use the ratio of a lesion to its adjacent native tissue [such as the ratio of hepatocellular carcinoma (HCC)’s DDVD to liver DDVD] to minimize these scaling factors. Li et al. (19) applied DDVD to assess the perfusion of HCC. DDVD results (ratio of HCC DDVD to background liver DDVD equals around 3.0) approximately agree with other dynamic contrast enhanced CT/MRI literature data. Lu et al. (20) reported earlier clinical grades rectal carcinoma had a higher DDVD ratio (DDVDr) (tumor to tumor-free rectal wall) than those of the advanced clinical grades (2.245 for grade 0&I, 1.460 for grade II, 1.430 for grade III, 1.130 for grade IV). These parameters are all consistent with the biological behaviors of HCC and rectal carcinoma. In an unpublished study, we used DDVDr to evaluate 24 pleomorphic adenomas (PA), 14 malignant tumors, and 16 Warthin’s tumors. DDVDr was DDVD of the tumor divided by DDVD of tumor free parotid gland tissue. A systematic literature search was conducted for parotid gland tumor perfusion imaging and histology microvessel density studies (21-36). Perfusion parameters of PAs, malignant tumors, and Warthin’s tumors were further normalized by PA’ measure (thus the normalized measure for PA is 1). The ratio results of malignant tumor DDVD and Warthin’s tumors DDVD were compared with literature results. It was noted that DDVDr ratios of both malignant tumor to PA (1.56) and Warthin’s tumor to PA (2.47) were very similar to the mean ratio of CT measured blood volume of these tumors (1.54 for malignant tumor to PA, and 2.51 for Warthin’s tumor to PA) (21-23). DDVDr ratio of malignant tumor to PA was also approximately close to the histology microvessel density ratio (1.29) reported by Zhao et al. (24), and DDVDr ratio of Warthin’s tumor to PA was close to the medium value of histology microvessel density ratio (2.41) of three reports (24-26). In these cases of this parotid gland tumors study, DDVD appears to offer more consistent and comparable results (comparable to CT and histology microvessel density results) than arterial spin labeling (25,27-31) and IVIM (30,32,33). IVIM-PF (intervoxel incoherent -perfusion fraction) is known to be affected by tissue’s T2 time, with longer T2 time leading to a ‘depressed’ perfusion fraction measure (19,37).
Case presentations
In this article, we suggest that DDVD can contribute to acute ischemic stroke assessment by providing insight into brain microvascular perfusion. DDVD, by requiring only two b-values, offers a faster alternative with no need for contrast agents. Hereby we describe our initial promising results of three brain acute ischemic stroke cases. Patient data were collected at Hainan Medical University Hospital, Haikou, China. The study was conducted in accordance with the Declaration of Helsinki (as revised in 2013). Institutional ethical approval was granted for this study, and verbal informed consent was obtained and recorded for this study. DWI data were acquired with a 3.0-T MRI scanner (Discovery MR750w, GE medical system, Milwaukee, USA) and a 16-channel head coil. DWI was performed using a single-shot spin-echo EPI, and the scan parameters included: repetition time (TR) =4,500 ms, echo time (TE) =78 ms, slice thickness =5 mm and inter-slice gap =1 mm, acquisition matrix = 120×120, field of view =240 mm × 240 mm, in plane resolution =2 mm. Number of excitation (NEX) =1. It is important to note that, the DDVDm in the current study appears low signal-to-noise ratio, as the DWI data acquisition used one NEX only. In further studies, we will improve the signal-to-noise ratio with higher NEX and explore de-noise techniques to improve the image quality. The figure contrast may be enhanced when cerebrospinal fluid high signal and noises are suppressed by applying masking and smoothing algorithms.
Case 1 was an 89-year-old woman admitted to the emergency department with a sudden onset of left limb weakness 12 hours earlier. Neurological examination showed a National Institutes of Health Stroke Scale (NIHSS) score of 10. CT angiogram showed occlusion at the right common carotid artery and extracranial internal carotid artery (Figure 2). The DDVDm, DWI, and CT perfusion source image of Case 1 are shown in Figure 3. The results show good agreement between DDVD perfusion deficit area and CT perfusion deficit.
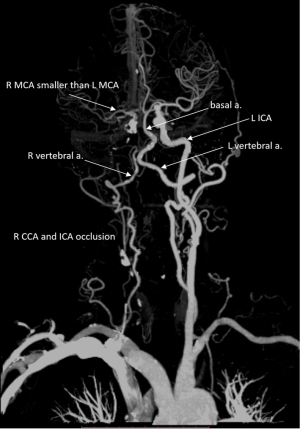
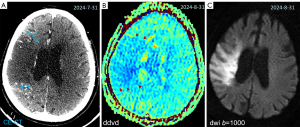
Case 2 was a 56-year-old man admitted to the emergency department, with a onset of left limb weakness 12 hours earlier. Neurological examination showed a NIHSS score of 5. Severe stenosis at the C7 segment of the right internal carotid artery was found on CT angiogram (Figure 4). The DDVDm, DWI, and CT perfusion cerebral blood volume (CBV) map, and mean transit time (MTT) map, time to maximum enhancement (Tmax) map of Case 2 are shown in Figure 5. The DDVD perfusion deficit area is slightly larger than the infarct area shown on DWI. Perfusion CT was performed 7 days after MRI. DDVD perfusion deficit area appears to agree with the Tmax perfusion deficit area.
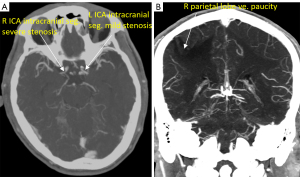
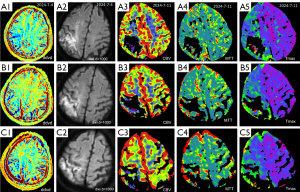
Case 3 was a 56-year-old man admitted to the hospital with a complaint of dysphasia for three days. Neurological examination showed a NIHSS score of 4. CT angiogram showed right internal carotid artery proximal segment stenosis and its intracranial segment severe stenosis (Figure 6). The DDVDm, DWI, and CT perfusion CBV map, and MTT map, Tmax map of Case 3 are shown in Figure 7. DDVD perfusion deficit area appears to agree with those of MTT and Tmax perfusion deficit area. CBV does not show an apparent infarct area, while DWI shows a high signal infarct region. The mismatch between DDVDm and DWI suggests a large at-risk penumbra area.
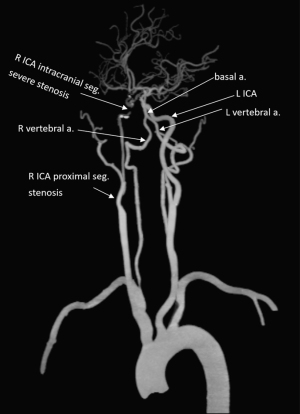
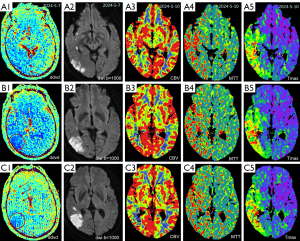
The principle of DDVD DWI protocol also allows for the generation of angiographic images, though currently more mature approaches for MRI-based cerebral artery angiography are already available. As a proof-of-principle example, a case of DDVD angiography of the pelvis region is shown in Figure 8 (10). Better data acquisition and image post-processing techniques are needed to make DDVD angiography clinically useable. Another interesting development is the availability of portable MRI scanners in recent years. Acquisition of MR images using lower field magnets has been made possible, with a significant decrease in the size and costs of such machines (38-41). These smaller machines are shown to provide portability with reasonable accuracy in acute stroke imaging in pilot studies (40,41). Portable MRI scanner and time-efficient assessment of brain perfusion with DDVD protocol may both make MRI more accessible in community emergency settings.
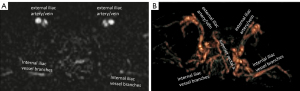
Conclusions
In conclusion, this study provides initial proof-of-concept results that DDVD may offer valuable information about the existence and size of penumbra area in acute ischemic stroke, potentially guiding clinical interventions. While promising, further research is needed to refine the technique, improve image quality, and validate its use in larger patient cohorts.
Acknowledgments
Funding: This work was supported by a
Footnote
Conflicts of Interest: All authors have completed the ICMJE uniform disclosure form (available at https://qims.amegroups.com/article/view/10.21037/qims-24-2139/coif). Y.X.J.W. serves as the Editor-in-Chief of Quantitative Imaging in Medicine and Surgery. Y.X.J.W. is the founder of Yingran Medicals Ltd., which develops medical image-based diagnostics software. The other authors have no conflicts of interest to declare.
Ethical Statement: The authors are accountable for all aspects of the work in ensuring that questions related to the accuracy or integrity of any part of the work are appropriately investigated and resolved. The study was conducted in accordance with the Declaration of Helsinki (as revised in 2013). Institutional ethical approval was granted for this study, and verbal informed consent was obtained and recorded for this study.
Open Access Statement: This is an Open Access article distributed in accordance with the Creative Commons Attribution-NonCommercial-NoDerivs 4.0 International License (CC BY-NC-ND 4.0), which permits the non-commercial replication and distribution of the article with the strict proviso that no changes or edits are made and the original work is properly cited (including links to both the formal publication through the relevant DOI and the license). See: https://creativecommons.org/licenses/by-nc-nd/4.0/.
References
- Mendelson SJ, Prabhakaran S. Diagnosis and Management of Transient Ischemic Attack and Acute Ischemic Stroke: A Review. JAMA 2021;325:1088-98. [Crossref] [PubMed]
- Sarraj A, Pujara DK, Campbell BC. Current State of Evidence for Neuroimaging Paradigms in Management of Acute Ischemic Stroke. Ann Neurol 2024;95:1017-34. [Crossref] [PubMed]
- Copen WA, Morais LT, Wu O, Schwamm LH, Schaefer PW, González RG, Yoo AJ. In Acute Stroke, Can CT Perfusion-Derived Cerebral Blood Volume Maps Substitute for Diffusion-Weighted Imaging in Identifying the Ischemic Core? PLoS One 2015;10:e0133566. [Crossref] [PubMed]
- Schaefer PW, Souza L, Kamalian S, Hirsch JA, Yoo AJ, Kamalian S, Gonzalez RG, Lev MH. Limited reliability of computed tomographic perfusion acute infarct volume measurements compared with diffusion-weighted imaging in anterior circulation stroke. Stroke 2015;46:419-24. [Crossref] [PubMed]
- Copen WA, Yoo AJ, Rost NS, Morais LT, Schaefer PW, González RG, Wu O. In patients with suspected acute stroke, CT perfusion-based cerebral blood flow maps cannot substitute for DWI in measuring the ischemic core. PLoS One 2017;12:e0188891. [Crossref] [PubMed]
- Ma X, Wang Y, Wang M, Zhang M, Meng N, Zhang L, Zhang J, Dou S, Wang M. Evaluation of infarct core and ischemic penumbra by absolute quantitative cerebral dynamic susceptibility contrast perfusion magnetic resonance imaging using self-calibrated echo planar imaging sequencing in patients with acute ischemic stroke. Quant Imaging Med Surg 2022;12:4286-95. [Crossref] [PubMed]
- Wang Q, Bie F, Lu T, Sun X, Sun Q, Wang G, Li P. The effect of cerebral blood perfusion on the correlation between cerebral stroke onset time and synthetic T2 mapping: a pilot study. Quant Imaging Med Surg 2023;13:3477-88. [Crossref] [PubMed]
- Huang H, Che-Nordin N, Wang LF, Xiao BH, Chevallier O, Yun YX, Guo SW, Wáng YXJ. High performance of intravoxel incoherent motion diffusion MRI in detecting viral hepatitis-b induced liver fibrosis. Ann Transl Med 2019;7:39. [Crossref] [PubMed]
- Wáng YXJ. Living tissue intravoxel incoherent motion (IVIM) diffusion MR analysis without b=0 image: an example for liver fibrosis evaluation. Quant Imaging Med Surg 2019;9:127-33. [Crossref] [PubMed]
- Yao DQ, Zheng CJ, Deng YY, Lu BL, Lu T, Hu GW, Li XM, Xiao BH, Ma FZ, Sabarudin A, King AD, Wáng YXJ. Potential diverse applications of diffusion-derived vessel density (DDVD) pixel-by-pixel mapping. Quant Imaging Med Surg 2024;14:2136-45. [Crossref] [PubMed]
- Huang H, Zheng CJ, Wang LF, Che-Nordin N, Wáng YXJ. Age and gender dependence of liver diffusion parameters and the possibility that intravoxel incoherent motion modeling of the perfusion component is constrained by the diffusion component. NMR Biomed 2021;34:e4449. [Crossref] [PubMed]
- Xiao BH, Huang H, Wang LF, Qiu SW, Guo SW, Wáng YXJ. Diffusion MRI Derived per Area Vessel Density as a Surrogate Biomarker for Detecting Viral Hepatitis B-Induced Liver Fibrosis: A Proof-of-Concept Study. SLAS Technol 2020;25:474-83. [Crossref] [PubMed]
- Hu GW, Zheng CJ, Zhong WX, Zhuang DP, Xiao BH, Wáng YXJ. Usefulness of diffusion derived vessel density computed from a simplified IVIM imaging protocol: An experimental study with rat biliary duct blockage induced liver fibrosis. Magn Reson Imaging 2021;84:115-23. [Crossref] [PubMed]
- Zheng CJ, Huang H, Xiao BH, Li T, Wang W, Wáng YXJ. Spleen in viral Hepatitis-B liver fibrosis patients may have a reduced level of per unit micro-circulation: non-invasive diffusion MRI evidence with a surrogate marker. SLAS Technol 2022;27:187-94. [Crossref] [PubMed]
- Gitlin N, Grahame GR, Kreel L, Williams HS, Sherlock S. Splenic blood flow and resistance in patients with cirrhosis before and after portacaval anastomoses. Gastroenterology 1970;59:208-13.
- He J, Chen C, Xu L, Xiao B, Chen Z, Wen T, Wáng YXJ, Liu P. Diffusion-Derived Vessel Density Computed From a Simplified Intravoxel Incoherent Motion Imaging Protocol in Pregnancies Complicated by Early Preeclampsia: A Novel Biomarker of Placental Dysfunction. Hypertension 2023;80:1658-67. [Crossref] [PubMed]
- Lu T, Wang L, Li M, Wang Y, Chen M, Xiao BH, Wáng YXJ. Diffusion-derived vessel density (DDVD) computed from a simple diffusion MRI protocol as a biomarker of placental blood circulation in patients with placenta accreta spectrum disorders: A proof-of-concept study. Magn Reson Imaging 2024;109:180-6. [Crossref] [PubMed]
- Hu GW, Li CY, Zhang G, Zheng CJ, Ma FZ, Quan XY, Chen W, Sabarudin A, Zhu MSY, Li XM, Wáng YXJ. Diagnosis of liver hemangioma using magnetic resonance diffusion-derived vessel density (DDVD) pixelwise map: a preliminary descriptive study. Quant Imaging Med Surg 2024; [Crossref]
- Li XM, Yao DQ, Quan XY, Li M, Chen W, Wáng YXJ. Perfusion of hepatocellular carcinomas measured by diffusion-derived vessel density biomarker: Higher hepatocellular carcinoma perfusion than earlier intravoxel incoherent motion reports. NMR Biomed 2024;37:e5125. [Crossref] [PubMed]
- Lu BL, Yao DQ, Wáng YXJ, Zhang ZW, Wen ZQ, Xiao BH, Yu SP. Higher perfusion of rectum carcinoma relative to tumor-free rectal wall: quantification by a new imaging biomarker diffusion-derived vessel density (DDVD). Quant Imaging Med Surg 2024;14:3264-74. [Crossref] [PubMed]
- Dong Y, Lei GW, Wang SW, Zheng SW, Ge Y, Wei FC. Diagnostic value of CT perfusion imaging for parotid neoplasms. Dentomaxillofac Radiol 2014;43:20130237. [Crossref] [PubMed]
- Niazi M, Mohammadzadeh M, Aghazadeh K, Sharifian H, Karimi E, Shakiba M, Baniasadi M, Rahmaty B, Adel S, Moharreri M. Perfusion Computed Tomography Scan Imaging in Differentiation of Benign from Malignant Parotid Lesions. Int Arch Otorhinolaryngol 2020;24:e160-9. [Crossref] [PubMed]
- Xu Z, Rong F, Yu T, Chen Y, Gao Q, Tian Zhou T, Pan A. Pleomorphic adenoma versus Warthin tumor of the parotid gland: Diagnostic value of CT perfusion imaging and its pathologic explanation. Journal of Tumor 2016;4:419-25.
- Zhao L, Mao Y, Mu J, Zhao J, Li F, Zhang S, Xin X. The diagnostic value of Superb Microvascular Imaging in identifying benign tumors of parotid gland. BMC Med Imaging 2020;20:107. [Crossref] [PubMed]
- Yamamoto T, Kimura H, Hayashi K, Imamura Y, Mori M. Pseudo-continuous arterial spin labeling MR images in Warthin tumors and pleomorphic adenomas of the parotid gland: qualitative and quantitative analyses and their correlation with histopathologic and DWI and dynamic contrast enhanced MRI findings. Neuroradiology 2018;60:803-12. [Crossref] [PubMed]
- Faur AC, Lazar E, Cornianu M. Vascular endothelial growth factor (VEGF) expression and microvascular density in salivary gland tumours. APMIS 2014;122:418-26. [Crossref] [PubMed]
- Hu H, Chen L, Zhu LN, Chen W, Su GY, Dou W, Bu SS, Wu FY, Xu XQ. Influence of post-label delay time on the performance of 3D pseudo-continuous arterial spin labeling magnetic resonance imaging in the characterization of parotid gland tumors. Eur Radiol 2022;32:1087-94. [Crossref] [PubMed]
- Kato H, Kanematsu M, Watanabe H, Kajita K, Mizuta K, Aoki M, Okuaki T. Perfusion imaging of parotid gland tumours: usefulness of arterial spin labeling for differentiating Warthin's tumours. Eur Radiol 2015;25:3247-54. [Crossref] [PubMed]
- Tanaka F, Umino M, Maeda M, Nakayama R, Inoue K, Kogue R, Obara M, Sakuma H. Tumor blood flow and apparent diffusion coefficient histogram analysis for differentiating malignant salivary tumors from pleomorphic adenomas and Warthin's tumors. Sci Rep 2022;12:5947. [Crossref] [PubMed]
- Ma G, Xu XQ, Zhu LN, Jiang JS, Su GY, Hu H, Bu SS, Wu FY. Intravoxel Incoherent Motion Magnetic Resonance Imaging for Assessing Parotid Gland Tumors: Correlation and Comparison with Arterial Spin Labeling Imaging. Korean J Radiol 2021;22:243-52. [Crossref] [PubMed]
- Razek AAKA. Multi-parametric MR imaging using pseudo-continuous arterial-spin labeling and diffusion-weighted MR imaging in differentiating subtypes of parotid tumors. Magn Reson Imaging 2019;63:55-9. [Crossref] [PubMed]
- Markiet K, Glinska A, Nowicki T, Szurowska E, Mikaszewski B. Feasibility of Intravoxel Incoherent Motion (IVIM) and Dynamic Contrast-Enhanced Magnetic Resonance Imaging (DCE-MRI) in Differentiation of Benign Parotid Gland Tumors. Biology (Basel) 2022;11:399. [Crossref] [PubMed]
- Zhang R, King AD, Wong LM, Bhatia KS, Qamar S, Mo FKF, Vlantis AC, Ai QYH. Discriminating between benign and malignant salivary gland tumors using diffusion-weighted imaging and intravoxel incoherent motion at 3 Tesla. Diagn Interv Imaging 2023;104:67-75. [Crossref] [PubMed]
- Zuo H. The Clinical Characteristics and CT Findings of Parotid and Submandibular Gland Tumours. J Oncol 2021;2021:8874100.
- Huang N, Chen Y, She D, Xing Z, Chen T, Cao D. Diffusion kurtosis imaging and dynamic contrast-enhanced MRI for the differentiation of parotid gland tumors. Eur Radiol 2022;32:2748-59. [Crossref] [PubMed]
- Huang N, Xiao Z, Chen Y, She D, Guo W, Yang X, Chen Q, Cao D, Chen T. Quantitative dynamic contrast-enhanced MRI and readout segmentation of long variable echo-trains diffusion-weighted imaging in differentiating parotid gland tumors. Neuroradiology 2021;63:1709-19. [Crossref] [PubMed]
- Ma FZ, Wáng YXJ. T2 relaxation time elongation of hepatocellular carcinoma relative to native liver tissue leads to an underestimation of perfusion fraction estimated by standard intravoxel incoherent motion MR imaging. Quant Imaging Med Surg 2024;14:1316-22. [Crossref] [PubMed]
- Su S, Hu J, Ding Y, Zhang J, Lau V, Zhao Y, Wu EX. Ultra-low-field magnetic resonance angiography at 0.05 T: A preliminary study. NMR Biomed 2024;37:e5213. [Crossref] [PubMed]
- Zhao Y, Ding Y, Lau V, Man C, Su S, Xiao L, Leong ATL, Wu EX. Whole-body magnetic resonance imaging at 0.05 Tesla. Science 2024;384:eadm7168. [Crossref] [PubMed]
- Sheth KN, Mazurek MH, Yuen MM, Cahn BA, Shah JT, Ward A, et al. Assessment of Brain Injury Using Portable, Low-Field Magnetic Resonance Imaging at the Bedside of Critically Ill Patients. JAMA Neurol 2020;78:41-7. [Crossref] [PubMed]
- Yuen MM, Prabhat AM, Mazurek MH, Chavva IR, Crawford A, Cahn BA, et al. Portable, low-field magnetic resonance imaging enables highly accessible and dynamic bedside evaluation of ischemic stroke. Sci Adv 2022;8:eabm3952. [Crossref] [PubMed]