Association of resting amygdalar activity with persistent atrial fibrillation mediated by right atrial inflammation activity on 18F-fluorodeoxyglucose positron emission tomography-computed tomography
Introduction
Atrial fibrillation (AF) is the most prevalent type of cardiac arrhythmia encountered in clinical practice and exhibits a progressive nature, characterized by the progression from paroxysmal AF (ParAF) to persistent AF (PerAF) (1). In contrast to patients with ParAF, those with PerAF have a heightened propensity for stroke, the most severe of the complications linked to AF (2). In one epidemiological study, individuals experiencing negative emotional states were found to have a fivefold increase in the risk of AF (3). Furthermore, stress has been shown to be a determining factor in the severity of AF. The interplay between the cardiac and cerebral domains assumes particular significance, as they are intricately interconnected through a myriad of feedback signals, potentially contributing to the mutual influence, exacerbation, and advancement of AF.
The amygdala’s efferent projections to the brainstem participate in the sympathetic responses to stress. The amygdala is recognized for its integral role in the pathophysiological processes that mediate the complex interactions between the central nervous system and the cardiovascular system (4,5). 18F-fluorodeoxyglucose (FDG) positron emission tomography-computed tomography (PET/CT), a sophisticated diagnostic imaging modality, offers a reliable and consistent metric for assessing the metabolic activity within the amygdala, which also enable a simultaneous estimation of bone-marrow activity (BMA) and right atrium (RA) inflammatory activity. Recently, increased amygdalar metabolic activity has been reported to be strongly associated with abnormal cardiac function and future major adverse cardiovascular events (MACEs), which is partly mediated by upregulated BMA (6-8). Our previous study indicated that RA inflammatory activity is a strong predictor of PerAF and poor prognosis (9). However, the correlations between resting amygdalar activity, BMA, RA inflammatory activity, and PerAF remain unclear.
We thus carefully conducted a study to clarify the correlation of 18F-FDG PET/CT-assessed resting amygdalar activity with BMA and RA inflammation activity, to assess their ability to predict PerAF, and to determine whether the association between resting amygdalar activity and PerAF is serially mediated by BMA and RA inflammation activity. We present this article in accordance with the STROBE reporting checklist (available at https://qims.amegroups.com/article/view/10.21037/qims-24-728/rc).
Methods
Study population
In this retrospective cohort study, 8,682 patients who had undergone a whole-body (skull to midthigh) 18F-FDG PET for the purpose of malignancy screening or staging at The Third Affiliated Hospital of Soochow University between January 1, 2018, and December 31, 2022, were enrolled. Of these patients, 186 patients had AF and underwent a series of diagnostic evaluations within a 1-week period, including electrocardiography, echocardiographic assessment, and pertinent hematological tests. In accordance with the established clinical guidelines (10), ParAF refers to episodes that persist for a duration of less than 7 days, with the potential for spontaneous reversion to sinus rhythm. Conversely, PerAF refers to persistence of the arrhythmia beyond the 7-day threshold, typically requiring pharmacological or electrical cardioversion for the restoration of normal sinus rhythm. The exclusion criteria for patients in this study were as follows: (I) presence of comorbidities with other cardiac conditions, including but not limited to coronary artery disease, congenital heart anomalies, valvular heart disorders, and pericardial pathologies; (II) presence of other acute or chronic inflammatory or autoimmune conditions at the start of the imaging procedure; (III) central nervous system tumor, lung, mediastinum, and bone tumor on 18F-FDG PET/CT imaging; and (IV) a history of prior AF ablation procedures and other types of cardiac surgical interventions. The cardiac diseases and inflammatory information were meticulously compiled through an exhaustive examination of the patients’ medical histories and extant health documentation, which was conducted prior to their enrollment in the study. Finally, 104 patients were included in the study population. The flowchart of participant screening is shown in Figure 1. No specific informed consent was needed for this study due to the retrospective nature of the analysis. This study was conducted in accordance with the Declaration of Helsinki (as revised in 2013) and approved by the Ethics Committee of The Third Affiliated Hospital of Soochow University ([2020] No. 34).
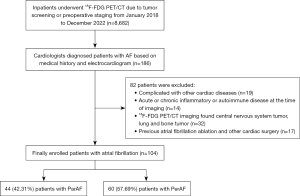
18F-FDG PET/CT imaging
The PET/CT equipment used in this study was a Biograph mCT 64, 52-ring LSO crystal/64-slice spiral CT device (Siemens Healthineers, Erlangen, Germany). Prior to initiation of the exam, patients underwent a fasting period of more than 8 hours, which was followed by the intravenous administration of 18F-FDG (Nanjing Jiangyuan Andy Cozhenge Research and Development Co., China) at a dosage of 3.7 MBq/kg, ensuring a precheck of blood glucose levels of less than 8 mmol/L. Thereafter, patients rested for a period of 60 minutes in a quiet and comfortable environment, maintaining a steady respiration while in a supine position. Initially, a 64-slice spiral CT scan was performed using a tube current of 35 mA and a tube voltage of 120 kV. Following the CT scan, three-dimensional PET images were acquired, with five to six bed positions being captured, each for a duration of 2.5 minutes. The range of the scan commenced at the apex of the cranium and extended distally to the middle and superior regions of the femur. Subsequently, the resulting images were subjected to an evaluation by a pair of experienced nuclear medicine physicians, each with more than 5 years of work experience.
18F-FDG PET/CT imaging analysis
This study incorporated both intra- and interobserver reproducibility assessments by executing the analysis twice under the observation of two independent evaluators (each with more than 5 years of experience). For the purpose of bias reduction, these evaluators were blinded to the patients’ medical history. Any discrepancies in visual analysis were adjudicated through consultation with an additional experienced physician for further insight. Quantitative analysis involved the averaging of the data measurement provided by the two initial evaluating physicians to determine the final outcome and included measurement of (I) resting amygdalar activity, (II) BMA, and (III) RA inflammation.
- For the measurement of resting amygdalar activity, the calculation of resting amygdalar activity involved dividing the maximum standardized uptake values (SUVs) in each amygdala by the mean SUVs in the ipsilateral temporal lobes, which served as background for resting amygdalar activity. The primary measure of amygdalar activity was determined by selecting the highest resting amygdalar activity value between the two amygdalae (7).
- For the measurement of the BMA, in order to measure bone marrow activity and spleen activity, regions of interest (ROIs) were positioned within each individual vertebra (L1 to L5). BMA was quantified as the average of SUVmax of the lumbar vertebrae divided by the activity in the background venous blood (11).
- For the measurement of RA inflammation activity, the SUVmax was used as a representative metric to quantify the metabolic activity within the atrial myocardium. In instances where the 18F-FDG uptake was absent, three circular ROIs, each with a diameter of 5 millimeters, were meticulously positioned on the atrial wall or other pertinent anatomical regions by leveraging the coregistered PET/CT imaging data. For ascertaining the background level of 18F-FDG uptake, a circular ROI with a diameter of 5 millimeters was meticulously delineated within the lumen of the ascending aorta according to the imaging data from the PET/CT scan, and then the mean SUV (SUVmean) was recorded. Subsequently, the target:background ratio (TBR) was calculated (12,13). Given the challenge of visually distinguishing the structural boundaries between the atrial appendage and the atrium, these two anatomical structures were considered a single entity in the analysis of their respective FDG activities.
Echocardiography
Echocardiographic images were acquired using a EPIQ 7C color Doppler ultrasound system equipped with an X5-1 probe (Philips, Amsterdam, the Netherlands), operating at a frequency range of 1–5 MHz. The acquisition of all echocardiographic images adhered to the protocols established by the American Society of Echocardiography (14). Patients were oriented in the left lateral decubitus posture while maintaining a calm rhythm of breathing. The electrocardiogram was concurrently recorded to determine the heart rate (HR) and to delineate the phasic sequence, with the mean value being calculated from three to five consecutive cardiac cycles. The left ventricular ejection fraction (LVEF) was assessed using the biplane Simpson method.
Hematological test
Upon admission, all patients were assessed for plasma markers in a fasting state, which included measurements of triglycerides (TG), total cholesterol (TC), low-density lipoprotein cholesterol (LDL-C), hemoglobin (Hb), neutrophils, and lymphocytes, along with white blood cell count (WBC).
Statistical analysis
SPSS 25 (IBM Corp., Armonk, NY, USA) and R v. 4.2.2 (The R Foundation for Statistical Computing) were employed to conduct the statistical analysis. The normality of the distribution for continuous variables was evaluated using the Kolmogorov-Smirnov test. These variables were subsequently reported in the format of mean with standard deviation (SD) or median with interquartile range (IQR; 25th to 75th percentile). The comparison between groups was conducted using the t test for normally distributed data and the Mann-Whitney test for data that deviated from normal distribution. Categorical variables are expressed in terms of percentages and were analyzed with the Chi-squared test or Fisher exact test. Univariable linear regression analyses were conducted for parametric data, and Spearman rank correlation coefficient was employed to analyze the relationships between the nonparametric data sets to evaluate the cardiometabolic disease parameters associated with resting amygdalar activity. To investigate the determinants influencing the occurrence of PerAF, we employed binary logistic regression model. Spearman correlations were used to quantify the correlation between resting amygdalar activity, BMA, and RATBR. Causal mediation analysis was employed as a statistical method to gauge the relative scale of various pathways and mechanisms through which an exposure could potentially influence an outcome. The total effect, indirect effect, and direct effect coefficients were estimated along with their 95% confidence intervals (CIs). The proportion mediated was also calculated along with the corresponding 95% CI (15). Subsequently, we conducted a comprehensive evaluation of the intra- and interobserver reliability in the measurement of FDG by employing the intraclass correlation coefficient (ICC) as a statistical metric for assessing reproducibility. An ICC cutoff value of at least 0.8 was selected to indicate that the metric had excellent repeatability. All P values were two-sided, and a threshold of P<0.05 denoted statistical significance.
Results
Patient characteristics
A total of 104 patients AF were selected based on predefined inclusion and exclusion criteria, 60 (57.7%) of whom had PerAF. Baseline characteristics are presented in Table 1. The population had an average age of 73.29±8.00 years and was 62.5% male. We calculated the CHA2DS2-VASc [congestive heart failure, hypertension, age ≥75 years (doubled), diabetes, stroke (doubled), vascular disease, age 65 to 74 years, and sex category (female)] score to assess stroke risk, which was slightly higher in the PerAF group than in the ParAF group but not significantly so (5.00±1.88 vs. 4.75±1.75; P=0.48). The TBR values for amygdalar, bone marrow, and RA uptake were 1.32±0.29, 1.93±0.62, and 1.99±0.71, respectively. Compared with patients ParAF, those with PerAF higher resting amygdalar activity (1.11±0.16 vs. 1.47±0.27), BMA (1.66±0.48 vs. 2.12±0.65), and RATBR (1.55±0.36 vs. 2.31±0.73) (all P values <0.001).
Table 1
Clinical characteristic | AF (N=104) | ParAF (n=44) | PerAF (n=60) | P value |
---|---|---|---|---|
Demographic parameters | ||||
Age (years), mean ± SD | 73.29±8.00 | 72.34±7.39 | 73.98±8.42 | 0.30 |
Male, n (%) | 65 (62.5) | 26 (59.1) | 39 (65.0) | 0.54 |
BMI (kg/m2), mean ± SD | 23.26±3.44 | 22.89±3.30 | 23.76±3.59 | 0.20 |
Previous cancer, n (%) | 78 (75.0) | 35 (79.5) | 43 (71.7) | 0.36 |
Smoking, n (%) | 32 (30.8) | 9 (20.5) | 23 (38.3) | 0.05* |
Drinking, n (%) | 23 (22.1) | 12 (27.3) | 11 (18.3) | 0.28 |
Hypertension, n (%) | 65 (62.5) | 23 (52.3) | 42 (70.0) | 0.07 |
Diabetes, n (%) | 27 (26.0) | 9 (20.5) | 18 (30.0) | 0.27 |
Hyperlipidemia, n (%) | 18 (17.3) | 8 (18.2) | 10 (16.7) | 0.84 |
CHA2DS2-VASc, mean ± SD | 4.89±1.82 | 4.75±1.75 | 5.00±1.88 | 0.48 |
Hematological parameters | ||||
Glu (mmol/L), mean ± SD | 6.45±2.30 | 6.23±1.77 | 6.62±2.63 | 0.40 |
TC (mmol/L), mean ± SD | 3.86±1.23 | 3.78±1.46 | 3.98±0.82 | 0.40 |
LDL (mmol/L), mean ± SD | 2.27±1.18 | 2.21±1.46 | 2.36±0.65 | 0.54 |
WBC (109/L), median (IQR) | 5.70 (4.48–7.60) | 5.83 (4.48–8.35) | 5.64 (4.21–7.44) | 0.67 |
Neutrophil (109/L), median (IQR) | 3.67 (2.88–5.69) | 3.63 (2.90–5.93) | 3.86 (2.80–5.60) | 0.99 |
Lymphocyte (109/L), median (IQR) | 1.07 (0.80–1.44) | 1.07 (0.88–1.44) | 1.06 (0.75–1.50) | 0.66 |
Increased CRP, n (%) | 44 (42.3) | 20 (45.5) | 24 (40.0) | 0.86 |
ECG parameters | ||||
HR, mean ± SD | 89.73±26.36 | 88.03±26.23 | 92.05±26.66 | 0.45 |
Echo parameters | ||||
LVEF, mean ± SD | 58.99±6.02 | 59.43±5.90 | 58.67±5.90 | 0.52 |
Drugs | ||||
Beta blockers, n (%) | 37 (35.6) | 14 (31.8) | 23 (38.3) | 0.52 |
Statin, n (%) | 25 (24.0) | 8 (18.2) | 17 (28.3) | 0.23 |
Anticoagulants, n (%) | 42 (40.4) | 16 (36.4) | 26 (43.3) | 0.48 |
18F-FDG PET/CT parameters | ||||
Resting amygdalar activity, mean ± SD | 1.32±0.29 | 1.11±0.16 | 1.47±0.27 | <0.001* |
BMA, mean ± SD | 1.93±0.62 | 1.66±0.48 | 2.12±0.65 | <0.001* |
RATBR, mean ± SD | 1.99±0.71 | 1.55±0.36 | 2.31±0.73 | <0.001* |
*, P<0.05. AF, atrial fibrillation; ParAF, paroxysmal atrial fibrillation; PerAF, persistent atrial fibrillation; SD, standard deviation; BMI, body mass index; CHA2DS2-VASc, congestive heart failure, hypertension, age ≥75 years (doubled), diabetes mellitus, prior stroke or transient ischemic attack or thromboembolism (doubled), vascular disease, age 65–74 years, sex category (female); Glu, blood glucose; TC, total cholesterol; LDL, low-density lipoprotein; WBC, white blood cell count; IQR, interquartile range; CRP, C-reactive protein; ECG, electrocardiogram; HR, heart rate; LVEF, left ventricular ejection fraction; 18F-FDG PET/CT, 18F-fluorodeoxyglucose positron emission tomography-computed tomography; BMA, bone-marrow activity; RATBR, right atrium target:background ratio.
Amygdalar activity was associated with hematopoietic system activity and RA inflammatory activity
On 18F-FDG PET/CT, a relationship was identified between resting amygdalar activity and BMA (β=0.28; P=0.004) and RA inflammatory activity (β=0.23; P=0.02) (Table 2). Resting amygdalar activity was significantly correlated with BMA (r=0.38; P<0.001; Figure 2A) and RATBR (r=0.44; P<0.001; Figure 2B).
Table 2
Risk factor | Beta (P value) (N=104) |
---|---|
Age | 0.04 (0.66) |
Male | 0.16 (0.11) |
BMI | 0.16 (0.11) |
Previous cancer | 0.07 (0.47) |
Smoking | 0.13 (0.19) |
Drinking | 0.09 (0.34) |
Hypertension | 0.003 (0.98) |
Diabetes | −0.03 (0.75) |
Hyperlipidemia | −0.12 (0.22) |
Glu | 0.07 (0.49) |
TC | −0.001 (0.99) |
LDL | −0.01 (0.92) |
WBC | −0.07 (0.48) |
Neutrophil | −0.06 (0.54) |
Lymphocyte | −0.07 (0.47) |
HR | 0.09 (0.36) |
LVEF | −0.16 (0.11) |
RATBR | 0.23 (0.02*) |
BMA | 0.28 (0.004*) |
*, P<0.05. BMI, body mass index; Glu, blood glucose; TC, total cholesterol; LDL, low-density lipoprotein; WBC, white blood cell count; HR, heart rate; LVEF, left ventricular ejection fraction; RATBR, right atrium target:background ratio; BMA, bone-marrow activity.
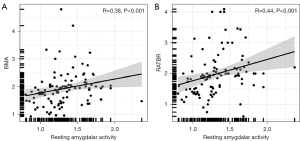
The relationship between resting amygdalar activity and RA inflammation activity appeared to be mediated by hematopoietic system activity.
A mediation analysis was conducted to estimate the effect of resting amygdalar activity on RA inflammation with BMA set as the mediator (Table 3). After adjustments were made for conventional cardiovascular risk factors (age, gender, hypertension, diabetes, and hyperlipidemia) and previous cancer, the total effect coefficient for the relationship between resting amygdalar activity and RA inflammation was 0.30 (95% CI: 0.15–0.42; P<0.001). The indirect effect coefficient mediated by BMA was 0.15 (95% CI: 0.07–0.27; P<0.001), and the direct effect coefficient was 0.15 (95% CI: 0.06–0.23; P<0.001). BMA significantly mediated the associations between resting amygdalar activity and RA inflammation, accounting for 50.2% (95% CI: 35.8–76.7%) of the association.
Table 3
Independent variable | Mediator | Total effect | Indirect effect | Direct effect | Proportion mediated (95% CI) | |||||
---|---|---|---|---|---|---|---|---|---|---|
Coefficient (95% CI) |
P value | Coefficient (95% CI) |
P value | Coefficient (95% CI) |
P value | |||||
Resting amygdalar activity | BMA | 0.30 (0.15–0.42) | <0.001 | 0.15 (0.07–0.27) | <0.001 | 0.15 (0.06–0.23) | <0.001 | 50.2% (35.8–76.7%) |
The mediation analyses were adjusted for age, gender, cancer, hypertension, DM, and hyperlipidemia. RATBR, right atrium target:background ratio; CI, confidence interval; BMA, bone-marrow activity; DM, diabetes mellitus.
Univariate and multivariate analyses for the factors influencing PerAF
In the univariate and multiple regression analyses of the 104 patients with AF, PerAF was the dependent variable. The univariate analysis indicated significant correlations of PerAF with several variables, including resting amygdalar activity, BMA, and RATBR (all P values <0.05). Variables that exhibited statistical significance during the univariate analysis phase were subsequently incorporated into the multivariate logistic regression model for further investigation. The only independent variables for PerAF were resting amygdalar activity [odds ratio (OR) =6.81; 95% CI: 2.34–19.75; P<0.001) and RATBR (OR =9.01; 95% CI: 3.04–26.70; P<0.001) (Table 4).
Table 4
Parameter | Univariate | Multivariate | |||
---|---|---|---|---|---|
OR (95% CI) | P value | OR (95% CI) | P value | ||
RATBR (per SD) | 9.79 (3.63–26.40) | <0.001* | 6.81 (2.34–19.75) | <0.001* | |
BMA (per SD) | 3.08 (1.64–5.82) | <0.001* | 1.34 (0.52–3.02) | 0.45 | |
Resting amygdalar activity (per SD) | 11.25 (4.33–29.25) | <0.001* | 9.01 (3.04–26.70) | <0.001* |
*, P<0.05. PerAF, persistent atrial fibrillation; OR, odds ratio; CI, confidence interval; RATBR, right atrium target:background ratio; SD, standard deviation; BMA, bone-marrow activity.
The relationship between resting amygdalar activity and PerAF through mediation of RA inflammation activity
We conducted a mediation analysis to quantify the indirect effect of resting amygdalar activity on PerAF by setting RATBR as the mediator (Table 5). After adjustments for conventional cardiovascular risk factors (age, gender, hypertension, diabetes, and hyperlipidemia) and previous cancer, the total effect coefficient for the relationship between resting amygdalar activity and AF was 0.25 (95% CI: 0.12–0.41; P<0.001). The indirect effect coefficient as mediated by RATBR was 0.08 (95% CI: 0.04–0.18; P<0.001), and the direct effect coefficient was 0.16 (95% CI: 0.06–0.29; P<0.001). RATBR significantly mediated the associations between resting amygdalar activity and PerAF, accounting for 33.2% (95% CI: 16.6–52.4%) of the association. Table 6 presents the findings pertaining to the reproducibility of parameters derived from PET/CT imaging (resting amygdalar activity, BMA, and RATBR). The analysis of intra- and interobserver variability revealed a consistently high level of measurement reproducibility across all parameters assessed (all ICCs >0.8). Figures 3,4 show an overall schematic and a case example, respectively.
Table 5
Independent variable | Mediator | Total effect | Indirect effect | Direct effect | Proportion mediated (95% CI) | |||||
---|---|---|---|---|---|---|---|---|---|---|
Coefficient (95% CI) |
P value | Coefficient (95% CI) |
P value | Coefficient (95% CI) |
P value | |||||
Resting amygdalar activity | RATBR | 0.25 (0.12–0.41) | <0.001 | 0.08 (0.04–0.18) | <0.001 | 0.16 (0.06–0.29) | <0.001 | 33.2% (16.6–52.4%) |
The mediation analyses were adjusted for age, gender, cancer, hypertension, DM, and hyperlipidemia. PerAF, persistent atrial fibrillation; CI, confidence interval; RATBR, right atrium target:background ratio; DM, diabetes mellitus.
Table 6
Parameters | Intraobserver | Interobserver | |||
---|---|---|---|---|---|
ICC (95% CI) | P value | ICC (95% CI) | P value | ||
Resting amygdalar activity | 0.86 (0.82–0.90) | <0.001 | 0.90 (0.84–0.96) | <0.001 | |
BMA | 0.88 (0.84–0.93) | <0.001 | 0.93 (0.87–0.99) | <0.001 | |
RATBR | 0.91 (0.85–0.98) | <0.001 | 0.96 (0.91–0.99) | <0.001 |
ICC, intraclass correlation coefficient; CI, confidence interval; BMA, bone-marrow activity; RATBR, right atrium target:background ratio.
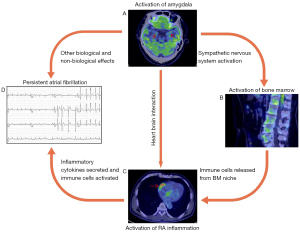
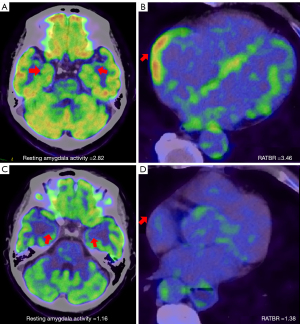
Discussion
The principal findings of the present study were as follows: (I) compared with patients with ParAF, those with PerAF had significantly higher resting amygdalar activity, BMA, and RATBR. (II) Resting amygdalar activity was found to be significantly associated with BMA and RATBR, and approximately 50.2% of the effect of resting amygdalar activity on RATBR was mediated by BMA. (III) Multivariate logistic regression analysis indicated that resting amygdalar activity and RATBR were independent risk factors of PerAF. (IV) Causal mediation analysis suggested that RATBR partially mediates the relationship between resting amygdalar activity and PerAF. The proportion mediated indicates that approximately 33.2% of the effect of resting amygdalar activity on PerAF is mediated by RATBR. To our knowledge, these are the first reported findings that substantiate this correlation.
The annual incidence of ParAF transitioning to PerAF is estimated to be approximately 5.5% and occurs concomitantly with an elevated risk of stroke. Moreover, this progression is often accompanied by atrial remodeling, a process frequently marked by irreversibility, and is associated with an unfavorable clinical prognosis (16). Lymphomononuclear infiltration accompanied by adjacent cardiomyocyte necrosis has been reported to occur in approximately 66.7% of patients with AF immediately after undergoing right atrial biopsies, implying the presence of a correlation between right atrial inflammation and the pathogenesis of AF (17). There is a growing abundance of evidence supporting a connection between psychological stressors, adverse emotional states, and both the onset and amplification of AF, although the pathophysiology remains unclear (18). One retrospective study reported elevated levels of C-reactive protein (CRP), an inflammation marker, in patients with PerAF as compared to those with ParAF, leading to speculation that the contribution of inflammation toward AF persistence may hold greater significance relative to its role in the emergence of AF (19). 18F-PET/CT has demonstrated efficacy in delineating inflammatory processes, including those of a low-grade nature, such as those occurring within the atrial chambers of patients with AF (20). Several PET/CT-based retrospective studies have found a correlation between augmented atrial 18F-FDG uptake and AF, especially in RA (21).
PET/CT imaging facilitates a comprehensive evaluation of metabolic activity across a variety of tissues including cerebral, heart, and bone marrow tissues, thus providing the capacity for extensive structural assessments spanning multiple organ systems. Consequently, PET/CT imaging is a valuable diagnostic tool for the assessment of pathophysiological pathways that encompass a spectrum of organ systems and can generate evidence for certain connections, such as the link between chronic stress and cardiovascular disease (22). The amygdala, an integral component of the limbic system, is located bilaterally within the temporal lobes and figures prominently in the mediation of both emotional and physiological responses to stress via its communication with key systems including the hypothalamic-pituitary-adrenal (HPA) axis, the sympathetic nervous system (SNS), and several other cerebral regions (23). In neuroimaging research, the uptake of 18F-FDG in the amygdala has been demonstrated to remain consistent over extended periods but is elevated in individuals experiencing stress-related conditions (24). Resting amygdalar activity is critically linked with inflammatory atherosclerosis and may be a valuable indicator of the stress response that initiates a neuroimmune response linking chronic stress to cardiovascular disease. Kang et al. hypothesize that the neural activity associated with emotional processing in the brain is mechanistically interconnected with the acute instability of atherosclerotic plaques through the process of macrophage hematopoiesis subsequent to an acute myocardial infarction (25). Tawakol et al. established an association between region-specific brain activity and the incremental risk of cardiovascular disease. Their findings suggest that heightened activity in the amygdala can independently and significantly predict the occurrence of cardiovascular events via a pathological pathway that is associated with heightened bone marrow activity and arterial inflammation (7). Osborne et al. proposed that there is a correlation between noise exposure and MACE, indicating the presence of a mechanism initiated by heightened stress-related limbic (amygdalar) activity with an accompanying increase in arterial inflammation (26). In their study, Mezue et al. found that alcohol consumption was associated with diminished activity within resting stress-associated neural networks, which occurred primarily due to a reduction in amygdalar activity. This neural effect mediated the advantageous impact of alcohol consumption on MACEs (27). Nevertheless, there is little empirical data regarding the interrelation between resting amygdalar activity and PerAF that has been generated with potential confounding factors being controlled for. Furthermore, the mechanisms that underpin this relationship have not yet been fully elucidated.
Our results demonstrated that the activity of the amygdala in a resting state us significantly correlated with BMA and RATBR. The bone marrow serves as the principal genesis point for circulating monocytes, which hold the potential to subsequently differentiate into macrophages within atherosclerotic lesions (28). A recent foundational study substantiated the existence of a neuro-hematopoietic-vascular axis in the pathogenesis of psoriasis and confirmed that bone marrow activity potentially mediated the pathway between resting amygdalar activity and aortic vascular inflammation (11). Our study yielded novel evidence elucidating the correlation between resting amygdalar activity and RA inflammation activity in the AF chronic inflammatory disease state, which is in part mediated by BMA. Our results suggest that elevated hematopoietic system activity could potentially contribute to the downstream development of AF within a stress-associated inflammatory milieu and further confirm that FDG uptake in the RA wall as assessed by 18F-FDG PET/CT may indicate pathological inflammation. However, the variation in FDG metabolic activity within hematopoietic tissue or bone marrow, the “incubator” for monocytes, did not achieve statistical significance between PerAF and ParAF.
The clinical relevance of our study lies in the linking of resting amygdalar activity with PerAF, and thus clinicians should be aware of the potential proinflammatory effects of resting amygdalar activity in individuals with PerAF, as this can facilitate a more nuanced understanding of the underlying pathophysiological mechanisms. Causal mediation analysis yielded insights into the mechanisms through which the elevated resting amygdalar activity contributed to the exacerbation of PerAF, particularly by delineating the extent to which this effect was mediated through heightened RA inflammation activity. Our analysis yielded pivotal insights into the cardio-cerebral nexus by revealing a neuroimmune pathway that originates within central nervous system nuclei and encompasses the upregulation of RA inflammatory processes. Novel therapeutic strategies for mitigating the burden of PerAF may involve interventions aimed at attenuating neural activity or the use of pharmacotherapies designed to specifically address atrial inflammation. For example, beta-adrenergic receptor blockers may be able to mitigate the proinflammatory sequelae of SNS activity, while anti-inflammatory medications or statins may exert a subsequent modulatory effect on RA inflammation (29,30). Although we focused specifically on sympathetic activity and inflammation in our study, involvement of the HPA axis and peripheral peptidergic signaling likely also play a role in the aforementioned AF-related pathway. Additional analyses or future studies evaluating markers of HPA axis activation (cortisol) and neuropeptides (substance P, calcitonin gene-related peptide) could provide evidence for the integration of these pathways with sympathetic activity and inflammation in relation to AF severity and outcomes.
The study still had several limitations that should be acknowledged. First, the applicability of our findings to broader populations may be limited, as we employed a single-center retrospective cohort analysis. Second, our study was exclusively observational, focusing on elucidating the correlation between amygdalar activity and PerAF. The medial prefrontal cortices, insulae, somatosensory cortices, and limbic system also play important roles in the autonomic regulation of the heart-brain axis, and thus the scope of our study was constrained. Moreover, patients were not subjected to subsequent PET/CT examinations posttreatment. A meticulously designed prospective and longitudinal study would be instrumental in elucidating the clinical implications of cerebral metabolic activity, atrial FDG uptake, and their prognostic significance in the context of treatment outcomes. Third, magnetic resonance imaging (MRI) of the brain, owing to its superior spatial resolution, can yield more comprehensive information as compared to CT. The use of PET-MRI could potentially enhance our comprehension of cerebral activity in patients with AF. Fourth, our study cohort consisted of a rather diverse patient group, encompassing individuals with various types of cancer. Patients undergoing PET/CT for oncological conditions may have heightened levels of stress and systemic inflammation, which could confound the association between resting amygdalar activity and AF. However, our regression models were adequately adjusted for the presence or absence of active malignancies. Furthermore, to mitigate this influence, we ensured that patients with acute or chronic inflammatory diseases unrelated to AF and those with central nervous system, lung, mediastinal, or bone tumors were excluded from the study. Despite these exclusions, we recognize that the oncological context remains a confounding factor. Future studies should aim to include a more diverse cohort, including patients without malignancies, to validate our findings and to better isolate the effects of amygdalar activity on AF. Finally, our analysis cannot serve as a replacement for empirical biological research; additional investigations are imperative to clarifying the underlying mechanisms and to ascertaining whether their manipulation can yield a significant favorable clinical impact.
Conclusions
Resting amygdalar activity and RATBR evaluated by PET/CT were significantly associated with PerAF. The association of resting amygdalar activity and PerAF was in part mediated by RA inflammatory activity, which could be a potential therapeutic target for PerAF. A prospective study is required to validate the generalizability of these findings.
Acknowledgments
Funding: This research was supported by
Footnote
Reporting Checklist: The authors have completed the STROBE reporting checklist. Available at https://qims.amegroups.com/article/view/10.21037/qims-24-728/rc
Conflicts of Interest: All authors have completed the ICMJE uniform disclosure form (available at https://qims.amegroups.com/article/view/10.21037/qims-24-728/coif). The authors have no conflicts of interest to declare.
Ethical Statement: The authors are accountable for all aspects of the work in ensuring that questions related to the accuracy or integrity of any part of the work are appropriately investigated and resolved. This study was conducted in accordance with the Declaration of Helsinki (as revised in 2013) and was approved by the Ethics Committee of The Third Affiliated Hospital of Soochow University ([2020] No. 34). The requirement for informed consent was waived due to the retrospective nature of the analysis.
Open Access Statement: This is an Open Access article distributed in accordance with the Creative Commons Attribution-NonCommercial-NoDerivs 4.0 International License (CC BY-NC-ND 4.0), which permits the non-commercial replication and distribution of the article with the strict proviso that no changes or edits are made and the original work is properly cited (including links to both the formal publication through the relevant DOI and the license). See: https://creativecommons.org/licenses/by-nc-nd/4.0/.
References
- Chugh SS, Havmoeller R, Narayanan K, Singh D, Rienstra M, Benjamin EJ, Gillum RF, Kim YH, McAnulty JH Jr, Zheng ZJ, Forouzanfar MH, Naghavi M, Mensah GA, Ezzati M, Murray CJ. Worldwide epidemiology of atrial fibrillation: a Global Burden of Disease 2010 Study. Circulation 2014;129:837-47. [Crossref] [PubMed]
- Hindricks G, Potpara T, Dagres N, Arbelo E, Bax JJ, Blomström-Lundqvist C, et al. 2020 ESC Guidelines for the diagnosis and management of atrial fibrillation developed in collaboration with the European Association for Cardio-Thoracic Surgery (EACTS): The Task Force for the diagnosis and management of atrial fibrillation of the European Society of Cardiology (ESC) Developed with the special contribution of the European Heart Rhythm Association (EHRA) of the ESC. Eur Heart J 2021;42:373-498. [Crossref] [PubMed]
- Segan L, Prabhu S, Kalman JM, Kistler PM. Atrial Fibrillation and Stress: A 2-Way Street? JACC Clin Electrophysiol 2022;8:1051-9. [Crossref] [PubMed]
- LeDoux JE, Iwata J, Cicchetti P, Reis DJ. Different projections of the central amygdaloid nucleus mediate autonomic and behavioral correlates of conditioned fear. J Neurosci 1988;8:2517-29. [Crossref] [PubMed]
- Templin C, Hänggi J, Klein C, Topka MS, Hiestand T, Levinson RA, Jurisic S, Lüscher TF, Ghadri JR, Jäncke L. Altered limbic and autonomic processing supports brain-heart axis in Takotsubo syndrome. Eur Heart J 2019;40:1183-7. [Crossref] [PubMed]
- Fiechter M, Roggo A, Burger IA, Bengs S, Treyer V, Becker A, Marȩdziak M, Haider A, Portmann A, Messerli M, Patriki D, Mühlematter UJ, von Felten E, Benz DC, Fuchs TA, Gräni C, Pazhenkottil AP, Buechel RR, Kaufmann PA, Gebhard C. Association between resting amygdalar activity and abnormal cardiac function in women and men: a retrospective cohort study. Eur Heart J Cardiovasc Imaging 2019;20:625-32. [Crossref] [PubMed]
- Tawakol A, Ishai A, Takx RA, Figueroa AL, Ali A, Kaiser Y, Truong QA, Solomon CJ, Calcagno C, Mani V, Tang CY, Mulder WJ, Murrough JW, Hoffmann U, Nahrendorf M, Shin LM, Fayad ZA, Pitman RK. Relation between resting amygdalar activity and cardiovascular events: a longitudinal and cohort study. Lancet 2017;389:834-45. [Crossref] [PubMed]
- Dai N, Tang X, Weng X, Cai H, Zhuang J, Yang G, Zhou F, Wu P, Liu B, Duan S, Yu Y, Guo W, Ju Z, Zhang L, Wang Z, Wang Y, Lu B, Shi H, Qian J, Ge J. Stress-Related Neural Activity Associates With Coronary Plaque Vulnerability and Subsequent Cardiovascular Events. JACC Cardiovasc Imaging 2023;16:1404-15. [Crossref] [PubMed]
- Wang B, Xu Y, Wan P, Shao S, Zhang F, Shao X, Wang J, Wang Y. Right Atrial Fluorodeoxyglucose Uptake Is a Risk Factor for Stroke and Improves Prediction of Stroke Above the CHA(2)DS(2)-VASc Score in Patients With Atrial Fibrillation. Front Cardiovasc Med 2022;9:862000. [Crossref] [PubMed]
- Kirchhof P, Benussi S, Kotecha D, Ahlsson A, Atar D, Casadei B, Castella M, Diener HC, Heidbuchel H, Hendriks J, Hindricks G, Manolis AS, Oldgren J, Popescu BA, Schotten U, Van Putte B, Vardas PESC Scientific Document Group. 2016 ESC Guidelines for the management of atrial fibrillation developed in collaboration with EACTS. Eur Heart J 2016;37:2893-962.
- Goyal A, Dey AK, Chaturvedi A, Elnabawi YA, Aberra TM, Chung JH, et al. Chronic Stress-Related Neural Activity Associates With Subclinical Cardiovascular Disease in Psoriasis: A Prospective Cohort Study. JACC Cardiovasc Imaging 2020;13:465-77. [Crossref] [PubMed]
- Mazurek T, Kiliszek M, Kobylecka M, Skubisz-Głuchowska J, Kochman J, Filipiak K, Królicki L, Opolski G. Relation of proinflammatory activity of epicardial adipose tissue to the occurrence of atrial fibrillation. Am J Cardiol 2014;113:1505-8. [Crossref] [PubMed]
- Watanabe E, Miyagawa M, Uetani T, Kinoshita M, Kitazawa R, Kurata M, Ishimura H, Matsuda T, Tanabe Y, Kido T, Kido T, Kurata A, Mochizuki T. Positron emission tomography/computed tomography detection of increased (18)F-fluorodeoxyglucose uptake in the cardiac atria of patients with atrial fibrillation. Int J Cardiol 2019;283:171-7. [Crossref] [PubMed]
- Lang RM, Bierig M, Devereux RB, Flachskampf FA, Foster E, Pellikka PA, Picard MH, Roman MJ, Seward J, Shanewise JS, Solomon SD, Spencer KT, Sutton MS, Stewart WJChamber Quantification Writing Group. American Society of Echocardiography's Guidelines and Standards Committee; European Association of Echocardiography. Recommendations for chamber quantification: a report from the American Society of Echocardiography's Guidelines and Standards Committee and the Chamber Quantification Writing Group, developed in conjunction with the European Association of Echocardiography, a branch of the European Society of Cardiology. J Am Soc Echocardiogr 2005;18:1440-63. [Crossref] [PubMed]
- Valeri L, Vanderweele TJ. Mediation analysis allowing for exposure-mediator interactions and causal interpretation: theoretical assumptions and implementation with SAS and SPSS macros. Psychol Methods 2013;18:137-50. [Crossref] [PubMed]
- Iwasaki YK, Nishida K, Kato T, Nattel S. Atrial fibrillation pathophysiology: implications for management. Circulation 2011;124:2264-74. [Crossref] [PubMed]
- Katritsis DG. Is atrial fibrillation an inflammatory disorder? Eur Heart J 2006;27:886-author reply 886. [Crossref] [PubMed]
- Lampert R, Jamner L, Burg M, Dziura J, Brandt C, Liu H, Li F, Donovan T, Soufer R. Triggering of symptomatic atrial fibrillation by negative emotion. J Am Coll Cardiol 2014;64:1533-4. [Crossref] [PubMed]
- Chung MK, Martin DO, Sprecher D, Wazni O, Kanderian A, Carnes CA, Bauer JA, Tchou PJ, Niebauer MJ, Natale A, Van Wagoner DR. C-reactive protein elevation in patients with atrial arrhythmias: inflammatory mechanisms and persistence of atrial fibrillation. Circulation 2001;104:2886-91. [Crossref] [PubMed]
- Vaidyanathan S, Patel CN, Scarsbrook AF, Chowdhury FU. FDG PET/CT in infection and inflammation--current and emerging clinical applications. Clin Radiol 2015;70:787-800. [Crossref] [PubMed]
- Fujii H, Ide M, Yasuda S, Takahashi W, Shohtsu A, Kubo A. Increased FDG uptake in the wall of the right atrium in people who participated in a cancer screening program with whole-body PET. Ann Nucl Med 1999;13:55-9. [Crossref] [PubMed]
- Taqueti VR, Di Carli MF, Jerosch-Herold M, Sukhova GK, Murthy VL, Folco EJ, Kwong RY, Ozaki CK, Belkin M, Nahrendorf M, Weissleder R, Libby P. Increased microvascularization and vessel permeability associate with active inflammation in human atheromata. Circ Cardiovasc Imaging 2014;7:920-9. [Crossref] [PubMed]
- Lagraauw HM, Kuiper J, Bot I. Acute and chronic psychological stress as risk factors for cardiovascular disease: Insights gained from epidemiological, clinical and experimental studies. Brain Behav Immun 2015;50:18-30. [Crossref] [PubMed]
- Zhu Y, Du R, Zhu Y, Shen Y, Zhang K, Chen Y, Song F, Wu S, Zhang H, Tian M. PET Mapping of Neurofunctional Changes in a Posttraumatic Stress Disorder Model. J Nucl Med 2016;57:1474-7. [Crossref] [PubMed]
- Kang DO, Eo JS, Park EJ, Nam HS, Song JW, Park YH, Park SY, Na JO, Choi CU, Kim EJ, Rha SW, Park CG, Seo HS, Kim CK, Yoo H, Kim JW. Stress-associated neurobiological activity is linked with acute plaque instability via enhanced macrophage activity: a prospective serial 18F-FDG-PET/CT imaging assessment. Eur Heart J 2021;42:1883-95. [Crossref] [PubMed]
- Osborne MT, Radfar A, Hassan MZO, Abohashem S, Oberfeld B, Patrich T, Tung B, Wang Y, Ishai A, Scott JA, Shin LM, Fayad ZA, Koenen KC, Rajagopalan S, Pitman RK, Tawakol A. A neurobiological mechanism linking transportation noise to cardiovascular disease in humans. Eur Heart J 2020;41:772-82. [Crossref] [PubMed]
- Mezue K, Osborne MT, Abohashem S, Zureigat H, Gharios C, Grewal SS, Radfar A, Cardeiro A, Abbasi T, Choi KW, Fayad ZA, Smoller JW, Rosovsky R, Shin L, Pitman R, Tawakol A. Reduced Stress-Related Neural Network Activity Mediates the Effect of Alcohol on Cardiovascular Risk. J Am Coll Cardiol 2023;81:2315-25. [Crossref] [PubMed]
- Fayad ZA, Swirski FK, Calcagno C, Robbins CS, Mulder W, Kovacic JC. Monocyte and Macrophage Dynamics in the Cardiovascular System: JACC Macrophage in CVD Series (Part 3). J Am Coll Cardiol 2018;72:2198-212. [Crossref] [PubMed]
- Tawakol A, Fayad ZA, Mogg R, Alon A, Klimas MT, Dansky H, Subramanian SS, Abdelbaky A, Rudd JH, Farkouh ME, Nunes IO, Beals CR, Shankar SS. Intensification of statin therapy results in a rapid reduction in atherosclerotic inflammation: results of a multicenter fluorodeoxyglucose-positron emission tomography/computed tomography feasibility study. J Am Coll Cardiol 2013;62:909-17. [Crossref] [PubMed]
- Heidt T, Sager HB, Courties G, Dutta P, Iwamoto Y, Zaltsman A, von Zur Muhlen C, Bode C, Fricchione GL, Denninger J, Lin CP, Vinegoni C, Libby P, Swirski FK, Weissleder R, Nahrendorf M. Chronic variable stress activates hematopoietic stem cells. Nat Med 2014;20:754-8. [Crossref] [PubMed]