Diagnosis of liver hemangioma using magnetic resonance diffusion-derived vessel density (DDVD) pixelwise map: a preliminary descriptive study
Introduction
Liver hemangioma (HG) has an incidence rate ranging between 0.4% and 20.0%, and is commonly discovered during any abdominal imaging work-up. According to the classification system of the International Society for the Study of Vascular Anomalies, liver HG is a vascular tumor (1-3). The pathogenesis of HG is ill-understood. Consistent with a female predilection (ratio of 2–5:1), HG is considered to be a congenital disorder with possible hormonal dependence (4,5). From a histopathological perspective, these neoplasms are characterized by cavernous venous spaces delineated by a lining of vascular endothelial cells and interspersed with connective tissue septa. With the hepatic artery serving as the principal source of vascular supply, the hemodynamics within HGs is notably impaired, exhibiting a markedly reduced flow rate. Morphologically, HGs are well-defined lesion with round or lobulated margins. The primary subtypes include cavernous HG, capillary HG, and sclerosing HG. The principal criterion for this classification is the extent of fibrous tissue present within the body of HG. Cavernous HG represents the most frequent subtype with the presence of larger vascular spaces coupled with a low quantity of connective tissue, and is closely aligned with the typical imaging profile of liver HG. Capillary HGs, also known as flash-filling or rapidly-filling HGs, account for approximately 16% of all liver HGs. This subtype is notably more prevalent in HG measuring less than 1 cm in diameter. Hyalinised or sclerosed HG is unusual and is believed to represent the end stage of a HG. Due to the replacement of the vascular spaces by fibrotic tissue, it is virtually impossible to propose a definitive diagnosis for sclerosed HG based on imaging, thus pathologic proof is necessary.
The vast majority of HG cases do not require treatment or monitoring. Notably, no cases of malignant transformation with liver HG have been documented. Large HG may give rise to complications in 4.5% to 19.7% of cases, consisting of bleeding, compressive effects on adjacent structures such as the bowel and torsion if pedunculated. A giant liver HG may cause serious coagulation disorders, such as Kasabach-Merritt syndrome, which presents as hemolytic anemia, thrombocytopenia, prolonged prothrombin time, and hypofibrinogenemia, as well as Budd–Chiari syndrome (6-10). Surgical resection is rarely indicated, except in the presence of Kasabach-Merritt syndrome (11,12). Transcatheter hepatic embolization can be applied to manage Kasabach-Merritt syndrome, as can a combination of systemic corticosteroids and vincristine (1,13).
Plain CT features of liver HG usually show a hypodense well-defined lesion, with an internal density similar to the vessels. On T1-weighted (T1-w) MR images, liver HGs display low signal intensity, and on T2-weighted (T2-w) images they show very high signal intensity due to the long T2 of its blood-filled vascular channels (14,15). In rare cases, mostly in advanced cirrhosis and hepatic steatosis, HGs can lose their typical imaging features. When MRI is the first line examination for the liver, for the majority of HG cases, the diagnosis is established with the application of a contrast enhanced imaging. Dynamic studies performed after the administration of extracellular compounds exhibit early nodular peripheral enhancement, since its feeding vessels originate from the hepatic artery. Subsequent phases of liver enhancement reveal a progressive slow centripetal fill-in, with hyper-intensity on delayed phase as compared to the normal liver parenchyma. Late intra-tumoral accumulation of contrast can be explained by the slow flowing blood within its vascular channels determining the absence of noticeable washout on the latter phases of the dynamic study (1-3,16,17). Nevertheless, this distinct enhancement pattern may not be discernible in lesions smaller than 5 mm. Less typical imaging findings of HGs can result from three main causes: altered morphology or structure, unusual blood flow patterns or associated liver abnormalities (1,3,18). When the diagnosis cannot be achieved with imaging, percutaneous biopsy may be required. Provided that a cuff of normal hepatic parenchyma is interposed between the capsule and the margin of HG, needle biopsy is not contraindicated. Caldironi et al. (19) reported an overall needle biopsy diagnostic accuracy of 96%.
The diffusion-weighted imaging (DWI)-derived surrogate biomarker diffusion-derived vessel density (DDVD) works on the principle that on spin-echo type echo-planar-imaging DWI, blood vessels (including micro-vessels) show high signal when there is no motion probing gradient [b=0 (s/mm2)], while they show low signal even when very low b-values (such as b=1 or 2) are applied. Thus, the signal difference between images when the motion probing gradient is off and on reflects the extent of tissue vessel density. DDVD is derived from the equation (20):
where ROIarea0 and ROIarea2 refer to the number of pixels in the selected region-of-interest (ROI) on b=0 and b=2 DWI, respectively. Sb0 refers to the measured total signal intensity within the ROI when b=0, and Sb2 refers to the measured total signal intensity within the ROI when b=2, thus Sb/ROIarea equates to the mean signal intensity within the ROI. Sb2 and ROIarea2 can also be approximated by other low b-values (such as b=10) DWI. If we consider a pixel is an individual ROI, DDVD pixelwise map (DDVDm) can be constructed pixel-by-pixel with this same principle (21).
In some cases, a HG can be diagnosed based on typical imaging features without the need for contrast enhanced scan. However, when MRI is the first line examination for the liver, contrast enhanced scan is commonly acquired to increase the diagnostic confidence for HG. In this study, we conducted a preliminary evaluation of magnetic resonance DDVDm for diagnosing HG.
Methods
There were three testing datasets of convenient samples initially not collected for the purpose of HG evaluation. All imaging data were acquired at 3.0T. For dataset-1, an intravoxel incoherent motion (IVIM) imaging DWI sequence was initially acquired with TR of 1,600 ms and TE of 59 ms and an acquisition spatial resolution of 3.02×3.11×7 mm3. DDVDm was reconstructed with b=0 and b=2 images. There were initially 47 patients, and three patients [1.0 cm HG; 1.24 cm focal nodular hyperplasia (FNH), 1.38 cm FNH] with a combination of too small lesion and/or position shift between b=0 and b=2 images were excluded. Finally, dataset-1 consisted of 16 HGs (lesion diameter median: 3.83 cm; range, 1.5–8.85 cm), 4 FNHs (lesion diameter median: 2.24 cm; range, 1.72–5.7 cm), and 24 hepatocellular carcinomas (HCCs, lesion diameter median: 5 cm; range, 1.83–12.77 cm). For dataset-2, an IVIM DWI sequence was initially acquired, with TR of 2,500 ms and TE of 84 ms and an acquisition spatial resolution of 2.73×2.73×5 mm3. DDVDm was reconstructed with b=0 and b=10 images. There were initially 7 patients, and one patient (HG lesion diameter: 1.3 cm) with a combination of small lesion and/or position shift between b=0 and b=10 images was excluded. Finally, dataset-2 consisted of 6 HGs (lesion diameter median: 2.76 cm; range, 1.14–6.2 cm). For dataset-3, DWI with two b-values of 0, 2 s/mm2 were acquired with TR of 313 ms and TE of 38 ms and an acquisition the spatial resolution of 3.04×3.04×7 mm3. DDVDm was reconstructed with b=0 and b=2 images. Dataset-3 consisted of 28 patients with 28 HCC (lesion diameter median: 5.78 cm; range, 1.91–13.52 cm) with DDVDm quality suitable for visual analysis. Detailed MR acquisition parameters for these three datasets are provided in Appendix 1. FNHs and HCCs all had pathological confirmation. HGs were diagnosed with typical contrast enhanced imaging appearances and/or with pathological confirmation. DDVDm in dataset-1, dataset-2, and dataset-3 were assigned with different pseudo-color scales, but within each dataset the same pseudo-color scale was applied to each patient’s images.
Three readers were involved in the image analysis. Reader-1 (C.Y.L.) was a senior trainee in radiology, and reader-2 (G.W.H.) and reader-3 (Y.X.J.W.) were specialist radiologists. Reader-1 and reader-2 had access to all the imaging data and clinical information of the patients of these three datasets, and some historical liver DDVDm data acquired in our unit. Reader-1 and reader-2 summarized the DDVDm features of HG and mass-forming lesion (MFL, i.e., FNH or HCC) and explained them to reader-3 (Y.X.J.W.) who had prior knowledge of liver DDVDm reading (21), and this was also aided with historical image data of liver DDVDm. Before the testing session, reader-3 had no knowledge of lesion specific information of dataset-1 and dataset-2. During the testing session for dataset-1 and dataset-2, which was completed in about 90 minutes, reader-3 was required to make a diagnosis solely based on DDVDm, and reader-1 was available to explain the location or extent of the lesions to reader-3 when asked. Reader-3 was required to make a decision for a lesion with four choices: (I) HG with confidence; (II) HG without confidence (lesion features suggesting HG but the diagnosis could not be made firmly); (III) solid MFL with confidence; (IV) solid MFL without confidence (lesion features suggesting MFL but the diagnosis could not be made firmly). Therefore, reader-3 was not asked to differentiate FNH from HCC.
After the testing session described above, three readers read dataset-3 together, to confirm whether the DDVDm features summarized from dataset-1 and dataset-2 would be generalizable to dataset-3. Reader-3 was also aware that dataset-3 only contained HCC lesions.
At the end of these readings, with reference to anatomical T1-w/T2-w images and DWI, the causes of misdiagnosis and other potential pitfalls were further analysed.
Results
The diagnostic performance of reader-3 for dataset-1 and dataset-2 during the testing session is shown in Table 1. Correct diagnosis was made in 90.9% of the HGs (72.7% with confidence) and 96.4% of the MFLs (85.7% with confidence). Figure 1 further shows that, for most of the larger lesions, the differentiation between HG and MFL was made with confidence.
Table 1
Category | Correct with confi | Correct without confi | Error without confi |
---|---|---|---|
HG (total n=22) | 72.7% (16/22) | 18.2% (4/22) | 9.1% (2/22) |
MFL (total n=28) | 85.7% (24/28) | 10.7% (3/28) | 3.6% (1#/28) |
#, if the T2-weighted images were available for analysis, then this case would not be mis-diagnosed. HG, hemangioma; MFL, mass-forming lesion; DDVDm, diffusion-derived vessel density pixelwise map; Confi, confidence.
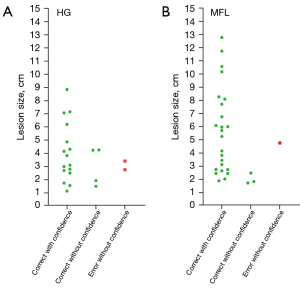
HGs generally showed substantially higher DDVD signal relative to background liver parenchyma (Figures 2-4). Though not necessarily, HG DDVD signals could be similar to those of blood vessels. Kidneys have higher perfusion than those of liver and spleen (22,23). Some HGs showed DDVD signals higher or similar to that of kidneys. Another sign noted in the current study is that a thick very low signal rim around, or partially around, the HG lesion (Figures 5-7, we term this as thick ‘black-out rim’). We did not see such a thick very low signal rim in MFLs in the current study. Two cases of mis-diagnosed HG lesions are shown in Figures 8,9. It was later considered that these two HGs might be associated with slow and stagnant blood flow.
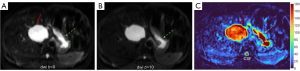
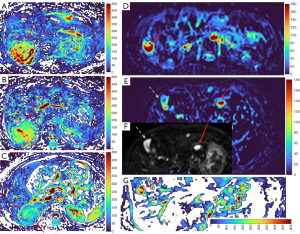
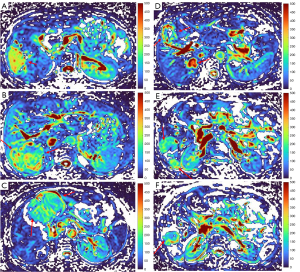
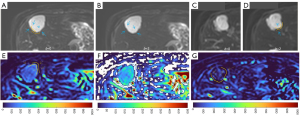
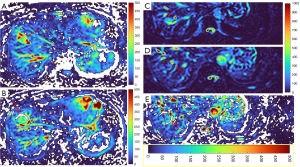
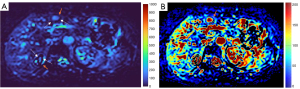
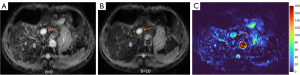
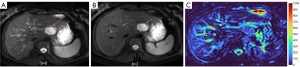
MFL generally showed DDVD signal only slightly higher, similar to, or even slightly lower, than that of background liver parenchyma (Figures 10,11). The case of mis-diagnosed MFL lesion is shown in Figure 12. It was later considered that a combination of peripheral necrotic foci and position shift between b=0 DWI and b=2 DWI caused artificial rim high signal on DDVDm.
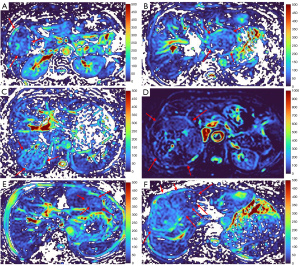
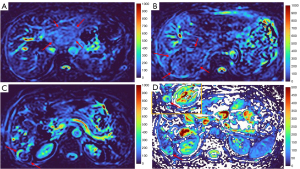
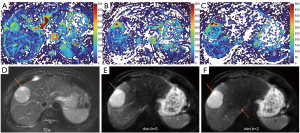
Dataset-3 were all HCC cases, and DDVDm of these cases were all consistent with MFL as agreed by the three readers. Figure 13 further shows two typical HCCs from dataset-3.
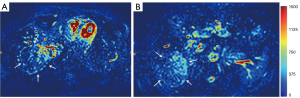
Discussion
It has been noted that the blood vessels show high signal when there is no motion probing gradient (b=0 s/mm2) and low signal when even very low b-values are applied (20). This phenomenon has not been fully validated with spin physics theory, but it is likely due to that, for spin-echo type echo-planar imaging (EPI) sequence, the second motion probing gradient after the 180-degree radiofrequency (RF) pulse could not fully re-focus the flowing spins in vessel and micro-vessels after being de-phased by the first motion probing gradient before the 180-degree RF pulse. DDVD measure based on this simple principle appears to be useful as a straightforward imaging biomarker. DDVD is a useful parameter for distinguishing of livers with and without fibrosis, and livers with severer fibrosis tend to have even lower DDVD measurements than those with milder liver fibrosis (20,24,25). Li et al. (26) applied DDVD to assess the perfusion of HCC. DDVD results (ratio of HCC DDVD to background liver DDVD equals around 3.0) approximately agree with other dynamic contrast enhanced CT/MRI literature data. Lu et al. (27) reported earlier clinical grades rectal carcinoma had a higher DDVD ratio (tumor to tumor-free rectal wall) than those of the advanced clinical grades (2.245 for grade 0&I, 1.460 for grade II, 1.430 for grade III, 1.130 for grade IV). These are all consistent with the biological behaviours of HCC and rectal carcinoma. Moreover, He et al. (28) reported that placenta DDVD as a perfusion biomarker of the placenta allows excellent separation of normal and early preeclampsia pregnancies. Lu et al. (29) reported that placenta regional DDVD is significantly higher in pregnant women with placenta accreta spectrum disorders than women with normal placenta, and especially higher in patients with placenta increta and percreta.
Liver HG has higher apparent diffusion coefficient (ADC) and T2 values than those of HCC and liver metastases, and lower ADC and T2 values than those of liver simple cysts. In some cases, a HG can be diagnosed based on typical imaging features without the need for contrast enhanced scan. However, as shown in Figure 14, a substantial portion of HG shows ADC and T2 overlapping with those of HCC and liver metastasis, and cyst (30-37). Therefore, contrast agent enhanced MRI is commonly used to confirm the diagnosis of liver HGs (38).
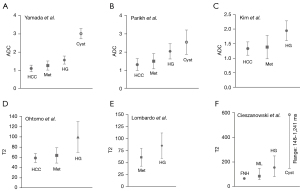
The analysis of DDVD requires only two b-values (with one being b=0 s/mm2), allowing a significantly shorter scanning time than contrast enhanced CT/MRI or IVIM imaging. Compared with contrast enhanced imaging, DDVD protocol does not involve contrast injection, data acquisition is faster. DDVD is conceptually as simple as ADC. Though ADC has been proposed to reflect tissue diffusion, recent analyses suggest that ADC value is heavily affected by T2 value of the tissue (39-41). In other words, T2 contribution to ADC quantification or ‘T2-shining through’ can never be eliminated. It has been recently also noted that non-invasive IVIM imaging cannot characterize HG (42). In fact, it has been shown that IVIM-perfusion fraction (PF) is also heavily affected by tissue’s T2, with longer T2 leading to a ‘depressed’ IVIM-PF measure (26,43,44). It is noted that liver simple cysts demonstrate very low signal on DDVDm (Figure 15). We anticipated that a substantial portion of ‘larger’ HGs could be diagnosed confidently with DDVDm alone, and this was confirmed in the current study. As shown in Table 1, based only on DDVDm, approximately 73% (16/22) of the HGs were diagnosed with confidence; for further 18% (4/22) of the HGs, a correct diagnosis was suggested. For the two cases (9% of the HGs) where an incorrect diagnosis was suggested, both cases might have had atypical blood flow pattern. For the MFLs, based only on DDVDm, approximately 86% (24/28) were diagnosed with confidence; for further 11% (3/28) of the MFLs, a correct diagnosis was suggested. Figure 1 suggests that larger lesions were more likely to be diagnosed with confidence. This is also the subjective experience of all three readers that large lesions could be differentiated with confidence. We noted that some cases of HG were associated with a ‘black-out rim’ (Figures 5-7). The HG’s ‘black-out rim’ differed from the rims of HCC in that HG’s ‘black-out rims’ were thicker, and HCC’s rims were more irregular. HG’s ‘black-out rim’ may be associated with the particular blood flow pattern of these HGs. More studies are needed to clarify this particular sign. It should be emphasized that the experience of reading liver DDVDm remained yet limited for the readers in this study. Diagnostic performance may improve with further accumulation of practical experience.
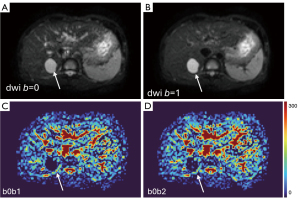
‘Position shift’ between b=0 image and b=2 (or 10) image is a major source of quantification error for DDVD calculation of the liver (21), as the liver is heavily subject to respiratory motion. Figure 16 Illustrates the principle that a ‘position shift’ between b=0 image and b=2 (or 10) image can cause artificially high, or low, or negative DDVD values. Figure 17 and Figure 18 further illustrate two cases with artificially high lesion DDVD signals. This strongly suggests that DDVDm should be viewed together with other structural images and DWI, and the extent of ‘position shift’ between b= 0 image and b=2 (or 10) image should be always noted. In fact, for the case illustrated in Figure 12, if the T2-weighted images were available for analysis, then this case would not be mis-diagnosed as HG by reader-3. For both dataset-1 and dataset-2, the images were initially acquired for IVIM analysis, without efforts being made to minimize the position shift between b=0 image and b=2 (or 10) image. One possible way to overcome this difficulty is to scan the DDVD protocol twice (or three) times, and manually select the pair of images with the most similar positions to reconstruct DDVDm. This approach may be attempted in the future particularly for smaller lesions. Breath-hold method with sufficiently long repetition time (TR), rather than free-breathing in dataset-1 and respiration-gating in dataset-2, is indeed practically feasible (20). In this study, DDVDm of dataset-3 were generally of lower image quality, possibly due to the shorter TR resulting in lower signal-to-noise ratio.
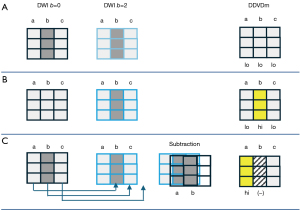
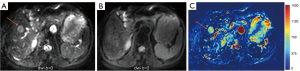
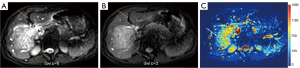
There are many limitations to this study. This is a preliminary descriptive study with small sample size, the full diversity of HG and MFL might not have been well presented in our samples. Notably, the lesions included in the analysis of the current study were mostly relatively large (Figure 1). Smaller lesions more likely cause diagnostic challenges. Future studies should include smaller lesions with well position-matched b=0 and b=2 images. Another limitation is that we did not include liver metastases. In this study, we did not conduct quantitative analysis, as in clinical practice the radiologists are more likely to make a diagnosis based on subjective assessment of the lesion’s signal intensity (as compared with the adjacent liver parenchyma and vessels such as the aorta and the portal/hepatic veins), the signal homogeneity of the lesion, and the morphology of the lesion, rather than conducting quantitative measurement. This study grouped together FNH and HCC as MFL, the potential separation of FNH and HCC based on DDVDm will be another topic of research for the future. For the HG lesions in this study, a thick ‘black-out rims’ was noted in some cases. The reliability of this particular sign for suggesting HG diagnosis shall be assessed with more studies. We did not compare the relative performance of DDVDm constructed from b=0 and b=2 images (as in dataset-1) and from b=0 and b=10 images (as in dataset-2), but our subjective impression was that these two types of DDVDm were comparable in HG evaluation. Note that, some of the clinical MRI scanners do not allow a non-zero b-value less than 10. In this study, we did not explore the optimal pseudo-color scale to demonstrate the lesions. Our future study will allow the pseudo-color scale to be adjusted individually during the image-reading, so that liver parenchyma show (dark) blue color, main vessels show red color, and then HGs show yellow, orange, or red color (Figures 19,20). Finally, this study only assessed the diagnostic performance of DDVDm, without considering the integration of other T2, ADC, and morphological features. We can anticipate that the integration of T2, ADC, and morphological features of the lesions can further improve the diagnostic performance of DWI. Note that, in this study, we did not compare relative diagnostic performance DDVDm with ADC map or T2 map. Our goal was to demonstrate that DDVDm can be another additional parameter based on a different contrast mechanism.
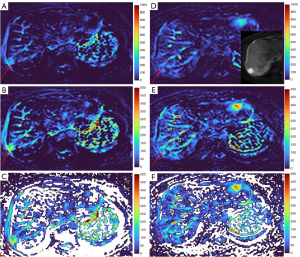
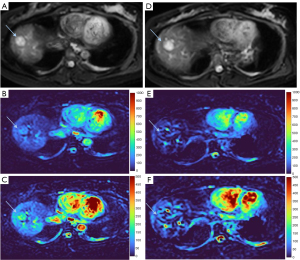
In conclusion, when DDVDm is used to evaluate the liver, HG can be diagnosed with confidence in a substantial portion of patients. A tentative pattern is noted that a small portion of HGs might be misdiagnosed as MFL, but a MFL is quite less likely to be misdiagnosed as HG. In practice, DDVDm should be considered together with lesion morphology and T2 signal and/or ADC features so to improve the diagnostic confidence. We anticipate that, with the integration of DDVDm to liver MRI, the number of gadolinium-contrast enhanced scans can be saved in a high proportion of patients, particularly for patients with large lesions.
Acknowledgments
We thank Mr. Dian-Qi Yao, a research student at the Chinese University of Hong Kong, for the help in image data post-processing.
Funding: This work was supported by
Footnote
Conflicts of Interest: All authors have completed the ICMJE uniform disclosure form (available at https://qims.amegroups.com/article/view/10.21037/qims-24-1837/coif). Y.X.J.W. serves as the Editor-in-Chief of Quantitative Imaging in Medicine and Surgery. Y.X.J.W. is the founder of Yingran Medicals Ltd., which develops medical image-based diagnostics software. W.C. is an employee of Philips Healthcare. M.S.Y.Z. contributed to the development of Yingran Medicals Ltd. The other authors have no conflicts of interest to declare.
Ethical Statement: The authors are accountable for all aspects of the work in ensuring that questions related to the accuracy or integrity of any part of the work are appropriately investigated and resolved. This study re-used historical image data for new analysis. All the diffusion weighed image data were acquired with the institutional ethical approval and with informed consent obtained from individual patients.
Open Access Statement: This is an Open Access article distributed in accordance with the Creative Commons Attribution-NonCommercial-NoDerivs 4.0 International License (CC BY-NC-ND 4.0), which permits the non-commercial replication and distribution of the article with the strict proviso that no changes or edits are made and the original work is properly cited (including links to both the formal publication through the relevant DOI and the license). See: https://creativecommons.org/licenses/by-nc-nd/4.0/.
References
- Kacała A, Dorochowicz M, Matus I, Puła M, Korbecki A, Sobański M, Jacków-Nowicka J, Patrzałek D, Janczak D, Guziński M. Hepatic Hemangioma: Review of Imaging and Therapeutic Strategies. Medicina (Kaunas) 2024;60:449. [Crossref] [PubMed]
- Sandulescu LD, Urhut CM, Sandulescu SM, Ciurea AM, Cazacu SM, Iordache S. One stop shop approach for the diagnosis of liver hemangioma. World J Hepatol 2021;13:1892-908. [Crossref] [PubMed]
- Caseiro-Alves F, Brito J, Araujo AE, Belo-Soares P, Rodrigues H, Cipriano A, Sousa D, Mathieu D. Liver haemangioma: common and uncommon findings and how to improve the differential diagnosis. Eur Radiol 2007;17:1544-54. [Crossref] [PubMed]
- Glinkova V, Shevah O, Boaz M, Levine A, Shirin H. Hepatic haemangiomas: possible association with female sex hormones. Gut 2004;53:1352-5. [Crossref] [PubMed]
- Giannitrapani L, Soresi M, La Spada E, Cervello M, D'Alessandro N, Montalto G. Sex hormones and risk of liver tumor. Ann N Y Acad Sci 2006;1089:228-36. [Crossref] [PubMed]
- Liu X, Yang Z, Tan H, Xu L, Sun Y, Si S, Liu L, Zhou W, Huang J. Giant liver hemangioma with adult Kasabach-Merritt syndrome: Case report and literature review. Medicine (Baltimore) 2017;96:e7688. [Crossref] [PubMed]
- Erdogan D, Busch OR, van Delden OM, Bennink RJ, ten Kate FJ, Gouma DJ, van Gulik TM. Management of liver hemangiomas according to size and symptoms. J Gastroenterol Hepatol 2007;22:1953-8.
- Gandolfi L, Leo P, Solmi L, Vitelli E, Verros G, Colecchia A. Natural history of hepatic haemangiomas: clinical and ultrasound study. Gut 1991;32:677-80. [Crossref] [PubMed]
- Sun JH, Nie CH, Zhang YL, Zhou GH, Ai J, Zhou TY, Zhu TY, Zhang AB, Wang WL, Zheng SS. Transcatheter Arterial Embolization Alone for Giant Hepatic Hemangioma. PLoS One 2015;10:e0135158. [Crossref] [PubMed]
- Sharma V, Aggarwal A, Singla R, Kalra N, Chawla YK. Giant hemangioma causing budd-Chiari syndrome. J Clin Exp Hepatol 2014;4:380-1. [Crossref] [PubMed]
- Hall GW. Kasabach-Merritt syndrome: pathogenesis and management. Br J Haematol 2001;112:851-62. [Crossref] [PubMed]
- O'Rafferty C, O'Regan GM, Irvine AD, Smith OP. Recent advances in the pathobiology and management of Kasabach-Merritt phenomenon. Br J Haematol 2015;171:38-51. [Crossref] [PubMed]
- Tlougan BE, Lee MT, Drolet BA, Frieden IJ, Adams DM, Garzon MC. Medical management of tumors associated with Kasabach-Merritt phenomenon: an expert survey. J Pediatr Hematol Oncol 2013;35:618-22. [Crossref] [PubMed]
- Mamone G, Miraglia R. The "light bulb sign" in liver hemangioma. Abdom Radiol (NY) 2019;44:2327-8. [Crossref] [PubMed]
- McFarland EG, Mayo-Smith WW, Saini S, Hahn PF, Goldberg MA, Lee MJ. Hepatic hemangiomas and malignant tumors: improved differentiation with heavily T2-weighted conventional spin-echo MR imaging. Radiology 1994;193:43-7. [Crossref] [PubMed]
- Brannigan M, Burns PN, Wilson SR. Blood flow patterns in focal liver lesions at microbubble-enhanced US. Radiographics 2004;24:921-35. [Crossref] [PubMed]
- Wilson SR, Burns PN. An algorithm for the diagnosis of focal liver masses using microbubble contrast-enhanced pulse-inversion sonography. AJR Am J Roentgenol 2006;186:1401-12. [Crossref] [PubMed]
- Ghai S, Dill-Macky M, Wilson S, Haider M. Fluid-fluid levels in cavernous hemangiomas of the liver: baffled? AJR Am J Roentgenol 2005;184:S82-5. [Crossref] [PubMed]
- Caldironi MW, Mazzucco M, Aldinio MT, Paccagnella D, Zani S, Pontini F, De Bellis ME, Rebuffi AG, Costantin G. Echo-guided fine-needle biopsy for the diagnosis of hepatic angioma. A report on 114 cases. Minerva Chir 1998;53:505-9.
- Wáng YXJ. Living tissue intravoxel incoherent motion (IVIM) diffusion MR analysis without b=0 image: an example for liver fibrosis evaluation. Quant Imaging Med Surg 2019;9:127-33. [Crossref] [PubMed]
- Yao DQ, Zheng CJ, Deng YY, Lu BL, Lu T, Hu GW, Li XM, Xiao BH, Ma FZ, Sabarudin A, King AD, Wáng YXJ. Potential diverse applications of diffusion-derived vessel density (DDVD) pixel-by-pixel mapping. Quant Imaging Med Surg 2024;14:2136-45. [Crossref] [PubMed]
- Vallée JP, Lazeyras F, Khan HG, Terrier F. Absolute renal blood flow quantification by dynamic MRI and Gd-DTPA. Eur Radiol 2000;10:1245-52. [Crossref] [PubMed]
- Winter JD, St Lawrence KS, Cheng HL. Quantification of renal perfusion: comparison of arterial spin labeling and dynamic contrast-enhanced MRI. J Magn Reson Imaging 2011;34:608-15. [Crossref] [PubMed]
- Xiao BH, Huang H, Wang LF, Qiu SW, Guo SW, Wáng YXJ. Diffusion MRI Derived per Area Vessel Density as a Surrogate Biomarker for Detecting Viral Hepatitis B-Induced Liver Fibrosis: A Proof-of-Concept Study. SLAS Technol 2020;25:474-83. [Crossref] [PubMed]
- Hu GW, Zheng CJ, Zhong WX, Zhuang DP, Xiao BH, Wáng YXJ. Usefulness of diffusion derived vessel density computed from a simplified IVIM imaging protocol: An experimental study with rat biliary duct blockage induced liver fibrosis. Magn Reson Imaging 2021;84:115-23. [Crossref] [PubMed]
- Li XM, Yao DQ, Quan XY, Li M, Chen W, Wáng YXJ. Perfusion of hepatocellular carcinomas measured by diffusion-derived vessel density biomarker: Higher hepatocellular carcinoma perfusion than earlier intravoxel incoherent motion reports. NMR Biomed 2024;37:e5125. [Crossref] [PubMed]
- Lu BL, Yao DQ, Wáng YXJ, Zhang ZW, Wen ZQ, Xiao BH, Yu SP. Higher perfusion of rectum carcinoma relative to tumor-free rectal wall: quantification by a new imaging biomarker diffusion-derived vessel density (DDVD). Quant Imaging Med Surg 2024;14:3264-74.
- He J, Chen C, Xu L, Xiao B, Chen Z, Wen T, Wáng YXJ, Liu P. Diffusion-Derived Vessel Density Computed From a Simplified Intravoxel Incoherent Motion Imaging Protocol in Pregnancies Complicated by Early Preeclampsia: A Novel Biomarker of Placental Dysfunction. Hypertension 2023;80:1658-67. [Crossref] [PubMed]
- Lu T, Wang L, Li M, Wang Y, Chen M, Xiao BH, Wáng YXJ. Diffusion-derived vessel density (DDVD) computed from a simple diffusion MRI protocol as a biomarker of placental blood circulation in patients with placenta accreta spectrum disorders: A proof-of-concept study. Magn Reson Imaging 2024;109:180-6. [Crossref] [PubMed]
- Yamada I, Aung W, Himeno Y, Nakagawa T, Shibuya H. Diffusion coefficients in abdominal organs and hepatic lesions: evaluation with intravoxel incoherent motion echo-planar MR imaging. Radiology 1999;210:617-23. [Crossref] [PubMed]
- Parikh T, Drew SJ, Lee VS, Wong S, Hecht EM, Babb JS, Taouli B. Focal liver lesion detection and characterization with diffusion-weighted MR imaging: comparison with standard breath-hold T2-weighted imaging. Radiology 2008;246:812-22. [Crossref] [PubMed]
- Kim HC, Seo N, Chung YE, Park MS, Choi JY, Kim MJ. Characterization of focal liver lesions using the stretched exponential model: comparison with monoexponential and biexponential diffusion-weighted magnetic resonance imaging. Eur Radiol 2019;29:5111-20. [Crossref] [PubMed]
- Ohtomo K, Itai Y, Furui S, Yashiro N, Yoshikawa K, Iio M. Hepatic tumors: differentiation by transverse relaxation time (T2) of magnetic resonance imaging. Radiology 1985;155:421-3. [Crossref] [PubMed]
- Lombardo DM, Baker ME, Spritzer CE, Blinder R, Meyers W, Herfkens RJ. Hepatic hemangiomas vs metastases: MR differentiation at 1.5 T. AJR Am J Roentgenol 1990;155:55-9. [Crossref] [PubMed]
- Cieszanowski A, Szeszkowski W, Golebiowski M, Bielecki DK, Grodzicki M, Pruszynski B. Discrimination of benign from malignant hepatic lesions based on their T2-relaxation times calculated from moderately T2-weighted turbo SE sequence. Eur Radiol 2002;12:2273-9. [Crossref] [PubMed]
- Duran R, Ronot M, Kerbaol A, Van Beers B, Vilgrain V. Hepatic hemangiomas: factors associated with T2 shine-through effect on diffusion-weighted MR sequences. Eur J Radiol 2014;83:468-78. [Crossref] [PubMed]
- Holzapfel K, Bruegel M, Eiber M, Ganter C, Schuster T, Heinrich P, Rummeny EJ, Gaa J. Characterization of small (≤10 mm) focal liver lesions: value of respiratory-triggered echo-planar diffusion-weighted MR imaging. Eur J Radiol 2010;76:89-95. [Crossref] [PubMed]
- EASL Clinical Practice Guidelines on the management of benign liver tumours. J Hepatol 2016;65:386-98. [Crossref] [PubMed]
- Wáng YXJ, Zhao KX, Ma FZ, Xiao BH. The contribution of T2 relaxation time to MRI-derived apparent diffusion coefficient (ADC) quantification and its potential clinical implications. Quant Imaging Med Surg 2023;13:7410-6. [Crossref] [PubMed]
- Wáng YXJ, Ma FZ. A tri-phasic relationship between T2 relaxation time and magnetic resonance imaging (MRI)-derived apparent diffusion coefficient (ADC). Quant Imaging Med Surg 2023;13:8873-80. [Crossref] [PubMed]
- Wáng YXJ. The very low magnetic resonance imaging apparent diffusion coefficient (ADC) measure of abscess is likely due to pus's specific T2 relaxation time. Quant Imaging Med Surg 2023;13:8881-5. [Crossref] [PubMed]
- Wáng YXJ, Sabarudin A. Underestimation of liver hemangioma perfusion fraction by standard intravoxel incoherent motion diffusion magnetic resonance imaging. Quant Imaging Med Surg 2024;14:2128-35. [Crossref] [PubMed]
- Ma FZ, Wáng YXJ. T2 relaxation time elongation of hepatocellular carcinoma relative to native liver tissue leads to an underestimation of perfusion fraction measured by standard intravoxel incoherent motion magnetic resonance imaging. Quant Imaging Med Surg 2024;14:1316-22. [Crossref] [PubMed]
- Yu WL, Ma FZ, Huang H, Xiao BH, Li XM, Wáng YXJ. Age and gender differences of normative values of spleen diffusion MRI parameters. Rofo 2024; Epub ahead of print. [Crossref]