Evaluation of the presence and severity of spontaneous splenorenal or gastrorenal shunts via four-dimensional flow magnetic resonance imaging: a preliminary study
Introduction
In cirrhosis, there is a combination of increased passive resistance, with substantial structural alterations related to liver fibrosis, and active resistance related to the vasoconstriction of hepatic vascular smooth muscle cells, resulting in an increase in portal venous pressure. Portal hypertension often leads to many potentially life-threatening complications. In advanced stages, blood undergoes flow reversal in part of the portal vein (PV), and the formation of portosystemic collateral pathways can be observed. Portosystemic collateral circulation, also known as a spontaneous portosystemic shunt (SPSS), usually forms naturally to reduce portal pressure as a compensatory mechanism for increased portal venous pressure (1). SPSS is a highly common occurrence in patients with cirrhosis, and its prevalence is approximately 60% (2). Spontaneous splenorenal shunts (SRSs) and gastrorenal shunts (GRSs) are relatively common types of SPSS. However, excessive SRS or GRS can also cause deterioration of liver function and overt hepatic encephalopathy (HE) (3). Noninvasive prediction of the existence and severity of portosystemic shunts is highly important.
Imaging exams, such as multiple spiral computed tomography and ultrasound, can clearly reveal portosystemic shunts and are highly valuable for detecting shunts. Ultrasound can be used to evaluate blood flow parameters such as flow velocity and flow rate but has certain limitations, such as being highly dependent on the skill of the operating and suboptimal for evaluating deep lesions. Both methods are based on morphological assessments and simple blood flow parameters, which are lagging indicators (4). Quantitative approaches are much more precise, objective, and reproducible than are qualitative approaches. SRS/GRS can cause corresponding changes in the flow parameters, the measurement of which can enable the early diagnosis and monitoring of SRSs or GRSs. Indeed, the quantitative assessment of hemodynamics in the portal system plays an essential role in many hepatic pathologies.
Four-dimensional phase-contrast magnetic resonance imaging (4D flow MRI) is a relatively novel MRI acquisition technique that quantitatively assesses fluid velocities in all three-dimensional (3D) volumes in three directions throughout the cardiac cycle (5). The image acquisition of 4D flow MRI is synchronized with the cardiac cycle, and the data collection is distributed across multiple cardiac cycles through k-space segmentation techniques. 4D flow MRI provides a unique and comprehensive set of information within a single acquisition, including hemodynamic and anatomical information, making it a powerful tool for noninvasive comprehensive vascular imaging. Unlike ultrasound, it is generally not operator dependent and can easily obtain the 3D volume of interest to characterize the velocity vector in all three spatial directions. In addition, it is different from two-dimensional (2D) flow MRI in that it offers the ability to characterize blood vessels retrospectively, even those with complex anatomical structures. Thus far, this technique has been applied primarily for the heart and aortic regions (6-9). Most studies on 4D flow MRI of the liver have focused on the diagnosis and risk stratification of portal hypertension or liver cirrhosis and surgical planning (10,11). The technique has not yet been widely used in the portal venous system because of the complex hepatic double blood supply, low flow velocity, and respiratory movements, and very few studies related to 4D flow MRI and portal shunts have been conducted. Many advanced fluid dynamics metrics obtained from 4D flow MRI have been proven to be effective. For instance, it was found that the wall shear stress and flow values shown on 4D flow magnetic resonance (MR) images change after partial hepatectomy in living liver donors (12). Moreover, Hyodo et al. (13) reported that prolonged relative residence time values on 4D flow MRI were significantly associated with the presence of PV thrombosis in patients with cirrhosis. In another study, 4D flow MRI demonstrated increased blood flow in residual portal branches 3–4 days after PV embolization, offering insights into estimating presurgical future liver remnant volume (14). Iwata et al. (15) reported that total kinetic energy (TKE) measurements based on 4D flow MRI can detect flow alterations induced by a systolic flow jet and left ventricular (LV) outflow tract geometry. Dyverfeldt et al. (16) reported that increased vascular tortuosity is associated with decreased TKE. Blood flow mostly appears in the form of laminar flow although sometimes it can cause larger-scale turbulence with high-velocity fluctuations. It is important to quantify the turbulent velocity fluctuation because it increases the pressure drop along the vessel by increasing fluid energy dissipation, which consequently leads to a requirement for more energy to maintain blood flow perfusion. Hence, compared with the conventional evaluation of morphological changes, the evaluation of portal hemodynamic variations in blood flow via 4D flow MRI may be more objective, sensitive, and reliable (17).
Therefore, the purpose of our study was to determine the correlation between the presence and severity of SRS/GRS and 4D flow MRI-derived parameters and to provide a more comprehensive theoretical support for clinical therapeutic strategies and prognostic evaluation in patients with portal hypertension and cirrhosis. We present this article in accordance with the STROBE reporting checklist (available at https://qims.amegroups.com/article/view/10.21037/qims-24-826/rc).
Methods
Participants
The study was conducted in accordance with the Declaration of Helsinki (as revised in 2013) and approved by the institutional review board of the Second Affiliated Hospital of Chongqing Medical University (ethics reference No. 2021–240). Informed consent was obtained from each patient. In this study, data from patients with cirrhosis who were diagnosed with portal hypertension and who underwent 4D flow MRI and contrast-enhanced MR examination at the Second Affiliated Hospital of Chongqing Medical University between April 2022 and October 2023 were analyzed. The exclusion criteria were as follows: (I) history of splenectomy, liver resection or transplantation, transjugular intrahepatic portosystemic shunt (TIPS), or surgical treatment for esophageal or gastric varices; (II) other special types of SPSS, such as paraumbilical veins or abdominal wall varices; (III) PV system thrombosis; (IV) age <18 or >70 years; and (V) poor MR image quality. The appropriate sample size was estimated by using a sample size calculator. The alpha error probability was set to 0.05, and the power (1 − beta error probability) was set to 0.90; on this basis, the sample size was calculated to be 67.
Clinical data collection
The clinical characteristics included sex, age, cirrhosis etiology, presence of ascites, and presence of HE. Laboratory features, including the serum albumin (ALB) concentration, alkaline phosphatase (ALP) concentration, alanine aminotransferase (ALT) level, aspartate aminotransferase (AST) level, serum direct bilirubin concentration, total serum bilirubin concentration, creatinine level, prothrombin time (PT), and PT-international normalized ratio (PT-INR), were systematically collected from all the participants. The Child-Pugh score and model for end-stage liver disease (MELD) score were also calculated. The MELD score was calculated as follows: MELD score = (9.57 × log creatinine mg/dL + 3.78 × log bilirubin mg/dL + 11.20 × log PT-INR + 6.43).
MRI acquisition
MR studies were performed with clinical 3-T MR scanners (MAGNETOM Prisma, Siemens Healthineers, Erlangen, Germany; Ingenia CX, Philips Healthcare, Amsterdam, the Netherlands) with a dedicated 32-channel abdominal coil and an 18-channel phased-array coil for all patients. Patients fasted for a minimum of 12 hours to allow for voiding of the gut contents. Scopolamine (20 mg, 10 mg/mL) was injected intramuscularly 10 min before the examination. All patients routinely underwent conventional MRI and contrast-enhanced MRI of the upper abdomen. Gadolinium contrast agent (Omniscan, GE HealthCare, Chicago, IL, USA) was intravenously injected during imaging at a dose of 0.2 mmol/kg and at a rate of 2.5 mL/s. A 20-mL flush of saline was subsequently infused after contrast agent injection.
A 4D flow MRI research sequence was performed before the injection of gadolinium contrast agent. Phase-contrast imaging was performed in the same coronal orientation through use of a slab that covers the upper abdominal vessels. Coronal balanced steady-state free precession imaging was conducted for segmentation. 4D flow MR images were acquired under the following parameters: repetition time msec/echo time msec, 53.76/4.26; two-signal average; k-space segmentation, 2; number of sections, 26; field of view (FOV), 380 × 285; voxel size, 2.4 × 2.4 × 2.5 mm3; matrix, 160 × 112 × 107; 2.5-mm true acquired isotropic spatial resolution; flip angle, 15°; reconstructed time frames per RR interval, 15; generalized auto calibrating partial parallel acquisition, 2; and scan time, approximately 12 minutes depending on the heart rate. We determined the velocity encoding (VENC) parameter for 4D flow MR by adding 20% to the measured maximum velocity.
Enhanced MRI data analysis
Contrast-enhanced MR images were independently measured and evaluated by two radiologists with considerable clinical experience in liver imaging (7 and 3 years of experience, respectively) who were blinded to the clinical details and biochemical data. Any discrepancies or errors were resolved by discussion. Collateral circulation was evaluated in the portal venous phase. SRS was defined as any contiguous vascular communication between the left renal vein and the splenic vein (SV) at the splenic hilum region (18). Moreover, GRSs are known as indirect SRSs and are defined as connections between the left renal vein and the SV through the posterior or short gastric veins (19). All the SRSs and GRSs were evaluated via multiplanar reconstructions (coronal, axial, and sagittal planes) and were measured at the maximum diameter via automated vessel segmentation techniques (curved planar reconstruction). Patients with SRS or GRS were divided into three groups according to diameter: without SRS/GRS (W-SRS/GRS), with small (<10 mm) SRS/GRS (S-SRS/GRS), and with large SRS/GRS (≥10 mm) (L-SRS/GRS) (10).
4D flow MRI data analysis
All 4D flow MRI datasets were automatically reconstructed into images with 14 timeframes per cardiac cycle. Subsequent vessel segmentation, flow visualization, and measurements were performed via cvi42 version 5.6.6 software (Circle Cardiovascular Imaging, Inc., Calgary, AB, Canada) (Figure 1). Eddy currents, Maxwell terms, and phase aliasing of the 4D flow MRI data were corrected automatically during reconstruction. All corrected MR images were manually segmented by a radiologist with 7 years of experience in abdominal imaging diagnosis. The segmented vascular masks were applied postprocess to the flow speed data for flow analysis. Vascular regions of interest (ROIs) were manually selected and placed in the targeted segments for flow quantification and visualization via cvi42. The locations of the cut planes and the postprocessing workflow are presented in Figure 1. The main PV is proximal to the splenomesenteric confluence (PV1) and liver hilum (PV2); the left or right branch of the PV is proximal to the confluence of the left (LPV) or right branches (RPV) of the PV, respectively; the SV is at the mesosplenic confluence (SV1) and at the splenic hilum (SV2); and the superior mesenteric vein (SMV) is proximal to the splenomesenteric confluence (SMV1) and is 5 cm from the splenomesenteric confluence (SMV2). The following 4D flow MRI-derived parameters were measured in all the above planes: TKE, total volume (TV), maximum flow velocity (Vmax) and mean flow velocity (Vmean), blood flow volume (BFV), maximum flow (MF), maximum wall shear stress (WSSmax) and mean wall shear stress (WSSmean), and maximum pressure gradient (PGmax) and mean pressure gradient (PGmean). When the relative pressure and WSS were measured, the reference plane was placed at the PV, proximal to the splenomesenteric confluence. Relative pressure is a noninvasive estimation of pressure differences. Although invasive catheterization is the gold standard for pressure difference measurements, 4D flow MRI is a promising tool for enabling noninvasive quantification via the linking of highly spatially resolved velocity measurements with pressure differences via the incompressible Navier-Stokes equations. One study using 4D flow MRI revealed that it is sufficiently robust to obtain relative pressure (20).
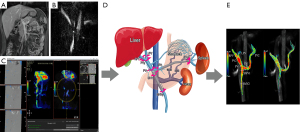
To assess consistency within observers, the above measurements were repeated by the same abdominal radiologist with a 1-week separation interval.
Statistical analysis
All the statistical analyses were performed in SPSS 27 software (IBM Corp., Armonk, NY, USA). The consistency of the judgment and grading of the SRS and GRS between the two radiologists was assessed with the Cohen κ coefficient. κ values were correlated with agreement as follows: less than chance agreement, <0; slight agreement, 0.01–0.20; fair agreement, 0.21–0.40; moderate agreement, 0.41–0.60; substantial agreement, 0.61–0.80; and almost perfect agreement, 0.81–0.99 (21). Intragroup consistency analysis of the MR data included in the study was conducted via the intraclass correlation coefficient (ICC) test. Reliability was considered poor for ICC values below 0.40, fair for values between 0.41 and 0.59, good for values between 0.60 and 0.74, and excellent for values between 0.75 and 1.00 (22). Categorical variables were tested with the Fisher exact test or the Chi-squared test. The test for normality was checked via the Kolmogorov-Smirnov test. The homogeneity of variance was verified with the Levene test. Comparisons among the three groups were performed via one-way analysis of variance (ANOVA). For the comparison of subgroups, the least significant difference (LSD) or Games-Howell (A) test was performed. The independent risk factors for S-SRS/GRS and L-SRS/GRS were identified via multivariate logistic regression analysis. To evaluate the predictive performance of the independent risk factors for the L-SRS/GRS, receiver operating characteristic (ROC) curves were plotted, and the corresponding area under the curve (AUC) values were calculated. The AUCs were subsequently compared via the DeLong test (MedCalc Software, Ostend, Belgium). The associations of the clinical data and 4D flow MRI-derived parameters with the GRS or SRS were evaluated via Spearman correlation coefficients. A two-sided P value <0.05 was considered statistically significant. Data are expressed as the mean ± standard deviation.
Results
Clinical characteristics
A total of 85 patients met the inclusion criteria. Of these, 2 were excluded because of TIPS or surgical treatment for esophageal or gastric varices, 7 were excluded because of complications associated with other special types of SPSS, 1 was excluded because of PV system thrombosis, and 5 were excluded because of poor MR image quality. Ultimately, 70 patients (26 women and 44 men) were included in our study, with an average age of 57.00±9.40 years (Figure 2). The numbers of patients in the W-SRS/GRS, S-SRS/GRS, and L-SRS/GRS groups were 27, 16, and 27, respectively. Among them, 33 had SRSs and 10 had GRSs, with a total average diameter of 12.21±6.06 mm. For the clinical data, no significant differences were observed between the three groups regarding HE, ascites, Child-Pugh score, or MELD score. There was a significant difference in the PT-INR between the W-SRS/GRS and S-SRS/GRS groups. No significant difference in any other clinical parameter was found between the two groups. The clinical characteristics of the patients are summarized in Table 1. The agreement between observers for the grading of SRS/GRS was excellent (κ=0.82).
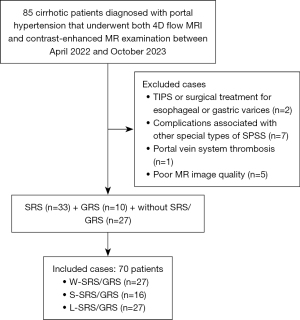
Table 1
Item | W-SRS/GRS | S-SRS/GRS | L-SRS/GRS | P |
---|---|---|---|---|
Age (years) | 55.89±10.84 | 58.94±8.61 | 56.96±8.41 | 0.596 |
Sex (male/female) | 18/9 | 6/10 | 20/7 | 0.056 |
Serum albumin, g/L | 35.31±6.20 | 37.71±6.97 | 36.60±4.84 | 0.433 |
ALP, U/L | 119.60±73.11 | 125.00±89.08 | 142.85±112.30 | 0.656 |
ALT, U/L | 60.85±77.16 | 39.73±18.23 | 34.85±43.15 | 0.215 |
AST, U/L | 70.44±54.97 | 54.93±32.70 | 60.85±60.49 | 0.638 |
Direct bilirubin, μmol/L | 26.15±42.91 | 10.07±9.60 | 55.81±126.26 | 0.201 |
Total bilirubin, μmol/L | 39.37±45.72 | 21.19±15.73 | 74.04±149.32 | 0.208 |
Serum creatinine, μmol/L | 64.45±20.35 | 65.39±14.40 | 68.84±17.86 | 0.669 |
PT-INR | 1.32±0.18 | 1.15±0.18 | 1.31±0.32 | 0.094 |
PT, s | 16.40±1.79 | 14.88±1.77 | 16.18±2.99 | 0.124 |
HE (yes/no) | 2/25 | 1/15 | 2/25 | >0.99 |
Ascites (no/mild/moderate/severe) | 16/8/2/1 | 10/4/1/1 | 21/6/0/0 | 0.542 |
Child-Pugh score (A/B/C) | 8/13/6 | 8/7/1 | 13/10/4 | 0.491 |
MELD score | 4.56±4.05 | 3.31±2.68 | 5.34±4.21 | 0.273 |
Data are conventionally expressed as the mean ± standard deviation. W-SRS/GRS, without SRS/GRS; S-SRS/GRS, small SRS/GRS; L-SRS/GRS, large SRS/GRS; SRS, splenorenal shunt; GRS, gastrorenal shunt; ALP, alkaline phosphatase; ALT, glutamic pyruvic transaminase; AST, glutamic oxaloacetic transaminase; PT, prothrombin time; INR, international normalized ratio; HE, hepatic encephalopathy; MELD, model for end-stage liver disease.
Univariate analysis of the 4D flow MRI-derived parameters among subgroups
The consistency between the measurements of the 4D flow MRI-derived parameters obtained twice by each individual operator was excellent (ICC =0.86). Among the W-SRS/GRS, S-SRS/GRS, and L-SRS/GRS groups, TVPV1 (P=0.003), BFVPV1 (P=0.011), MFPV1 (P=0.016), TVPV2 (P=0.036), BFVPV2 (P=0.025), and MFSMV2 (P=0.049) were significantly different (Table 2). Between the W-SRS/GRS and S-SRS/GRS groups, there were significant differences in the PGmaxPV1 (0.03±0.05 vs. 0.19±0.24; P=0.034) and PGmeanPV1 (−0.003±0.03 vs. 0.15±0.19; P=0.016). Meanwhile, between the W-SRS/GRS and L-SRS/GRS groups, there were significant differences in BFVLPV (0.34±0.25 vs. 0.18±0.14, P=0.31), TVPV1 (12.19±7.08 vs. 6.57±3.50; P<0.001), BFVPV1 (0.90±0.52 vs. 0.47±0.30; P=0.003), VmaxPV1 (20.47±5.73 vs. 17.25±5.76; P=0.048), MFPV1 (19.79±10.51 vs. 12.66±6.50; P=0.004), TVPV2 (11.06±8.50 vs. 6.45±4.09; P=0.015), BFVPV2 (0.89±0.60 vs. 0.48±0.29; P=0.009), MFPV2 (18.74±12.45 vs. 12.11±6.91; P=0.018), TVSV1 (6.42±5.86 vs. 3.52±4.16; P=0.043), BFVSV2 (0.18±0.09 vs. 0.30±0.17; P=0.032), and MFSMV2 (4.92±2.32 vs. 7.48±4.50; P=0.018). Between the S-SRS/GRS and L-SRS/GRS groups, there were significant differences in the TKE (52.39±21.79 vs. 77.73±54.9; P=0.49), PGmaxPV1 (0.19±0.24 vs. 0.02±0.13; P=0.023), and PGmeanPV1 (0.15±0.19 vs. 0.001±0.004; P=0.016). The representative maximum intensity projection (MIP) reconstruction and 4D flow MR images of the three groups are presented in Figures 3-5.
Table 2
4D flow MRI-derived parameters | W-SRS/GRS (n=27) | S-SRS/GRS (n=16) | L-SRS/GRS (n=27) | F | P |
---|---|---|---|---|---|
TVPV1 (mL) | 12.19±7.08 | 9.11±5.82 | 6.57±3.50 | 6.472 | 0.003 |
BFVPV1 (Vol/min) | 0.90±0.52 | 0.69±0.44 | 0.47±0.30 | 4.916 | 0.011 |
MFPV1 (mL/s) | 19.79±10.51 | 15.41±8.71 | 12.66±6.50 | 4.383 | 0.016 |
TVPV2 (mL) | 11.06±8.50 | 10.32±6.77 | 6.45±4.09 | 3.514 | 0.036 |
BFVPV2 (Vol/min) | 0.89±0.60 | 0.79±0.49 | 0.48±0.29 | 3.988 | 0.025 |
MFSMV2 (mL/s) | 4.92±2.32 | 6.96±4.50 | 7.48±4.50 | 3.167 | 0.049 |
Data are expressed as the mean ± standard deviation. 4D, four-dimensional; MRI, magnetic resonance imaging; W-SRS/GRS, without SRS/GRS; S-SRS/GRS, small SRS/GRS; L-SRS/GRS, large SRS/GRS; SRS, splenorenal shunt; GRS, gastrorenal shunt; TV, total volume, the volume of blood flow through the placed plane during one cardiac cycle; BFV, blood flow volume, the amount of blood that flows through a plane of a blood vessel per unit of time; MF, maximum flow, it is an instantaneous value; PV, portal vein; SMV, superior mesenteric vein.
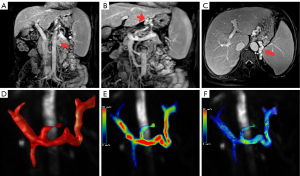
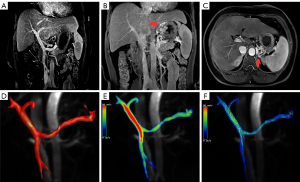
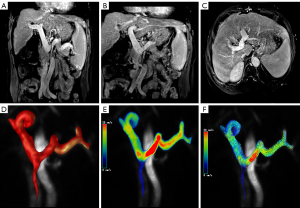
Differences in risk factors between the S-SRS/GRS and L-SRS/GRS groups
Six parameters, namely, TVPV1, BFVPV1, MFPV1, TVPV2, BFVPV2, and MFSMV2, were significantly different among the three groups according to multivariate logistic regression analysis. The above results revealed that none of these factors were independent risk factors for S-SRS/GRS. TVPV1, BFVPV1, TVPV2, BFVPV2, and MFSMV2 were found to be independent risk factors for L-SRS/GRS with intermediate diagnostic efficacy, with AUCTV PV1 =0.706 [95% confidence interval (CI): 0.519–0.853; sensitivity, 61.54%; specificity, 80.77%; P=0.018], AUCBFV PV1 =0.694 (95% CI: 0.507–0.844; sensitivity, 95.00%; specificity, 63.16%; P=0.035), AUCTV PV2 =0.729 (95% CI: 0.544–0.870; sensitivity, 77.78%; specificity, 66.67%; P=0.016), AUCBFV PV2 =0.718 (95% CI: 0.531–0.862; sensitivity, 60.00%; specificity, 82.35%; P=0.017), and AUCMF SMV2 =0.788 (95% CI: 0.608–0.912; sensitivity, 44.00%; specificity, 84.46%; P=0.005), respectively (Tables 3,4, Figure 6). When the TVPV1, BFVPV1, TVPV2, and BFVPV2 values were lower than the cutoff values of 6.645 mL, 0.779 Vol/min, 10.082 mL, and 0.419 Vol/min, respectively, the risk of L-SRS/GRS formation increased. When the MFSMV2 was higher than the predetermined cutoff value of 7.107 mL/s, the risk of L-SRS/GRS formation increased.
Table 3
Risk factor | B | Standard deviation | Wald | P | Exp (B) | 95% CI for Exp(B) | |
---|---|---|---|---|---|---|---|
Lower bound | Upper bound | ||||||
S-SRS/GRS | |||||||
TVPV1 | −3.537 | 3.057 | 1.339 | 0.247 | 0.029 | 7.279E−5 | 11.642 |
BFVPV1 | 40.402 | 39.234 | 1.060 | 0.303 | 3.517E+17 | 1.413E−16 | 8.756E+50 |
MFPV1 | 0.134 | 0.212 | 0.398 | 0.528 | 1.143 | 0.754 | 1.733 |
TVPV2 | 3.322 | 3.124 | 1.131 | 0.288 | 27.721 | 0.061 | 12634.645 |
BFVPV2 | −40.613 | 40.868 | 0.988 | 0.320 | 2.302E−18 | 3.759E−53 | 1.409E+17 |
MFSMV2 | 0.307 | 0.178 | 2.963 | 0.085 | 1.359 | 0.958 | 1.927 |
L-SRS/GRS | |||||||
TVPV1 | −10.370 | 4.388 | 5.586 | 0.018 | 3.135E−5 | 5.773E−9 | 0.170 |
BFVPV1 | 116.919 | 55.395 | 4.455 | 0.035 | 5.989E+50 | 4215.571 | 8.508E+97 |
MFPV1 | 0.432 | 0.378 | 1.312 | 0.252 | 1.541 | 0.735 | 3.229 |
TVPV2 | 10.366 | 4.287 | 5.848 | 0.016 | 3.176E+4 | 7.130 | 1.415E+8 |
BFVPV2 | −132.142 | 55.469 | 5.675 | 0.017 | 4.087E−58 | 2.492E−105 | 6.704E−11 |
MFSMV2 | 0.623 | 0.223 | 7.816 | 0.005 | 1.864 | 1.205 | 2.886 |
S-SRS/GRS, small SRS/GRS; L-SRS/GRS, large SRS/GRS; SRS, splenorenal shunt; GRS, gastrorenal shunt; CI, confidence interval; TV, total volume, the volume of blood flow through the placed plane during one cardiac cycle; BFV, blood flow volume, the amount of blood that flows through a plane of a blood vessel per unit of time; MF, maximum flow, it is an instantaneous value; PV, portal vein; SMV, superior mesenteric vein.
Table 4
Risk factor | AUC | Sensitivity (%) | Specificity (%) | Youden index | Cutoff value | 95% CI of AUC | DeLong P value on AUC |
---|---|---|---|---|---|---|---|
TVPV1 | 0.706 | 61.54 | 80.77 | 0.423 | 6.645 mL | 0.519–0.853 | AUCTV PV1 vs. BFV PV1, 0.649; AUCTV PV1 vs. TV PV2, 0.708; AUCTV PV1 vs. BFV PV2, 0.862; AUCTV PV1 vs. MF SMV2, 0.554; AUCBFV PV1 vs. TV PV2, 0.605; AUCBFV PV1 vs. BFV PV2, 0.734; AUCBFV PV1 vs. MF SMV2, 0.506; AUCTV PV2 vs. BFV PV2, 0.671; AUCTV PV2 vs. MF SMV2, 0.639; AUCBFV PV2 vs. MF SMV2, 0.579 |
BFVPV1 | 0.694 | 95.00 | 63.16 | 0.582 | 0.779 Vol/min | 0.507–0.844 | |
TVPV2 | 0.729 | 77.78 | 66.67 | 0.444 | 10.082 mL | 0.544–0.870 | |
BFVPV2 | 0.718 | 60.00 | 82.35 | 0.424 | 0.419 Vol/min | 0.531–0.862 | |
MFSMV2 | 0.788 | 44.00 | 88.46 | 0.325 | 7.107 mL/s | 0.608–0.912 |
4D, four-dimensional; MRI, magnetic resonance imaging; L-SRS/GRS, large SRS/GRS; SRS, splenorenal shunt; GRS, gastrorenal shunt; AUC, area under the curve; CI, confidence interval; TV, total volume, the volume of blood flow through the placed plane during one cardiac cycle; BFV, blood flow volume, the amount of blood that flows through a plane of a blood vessel per unit of time; PV, portal vein; SMV, superior mesenteric vein.
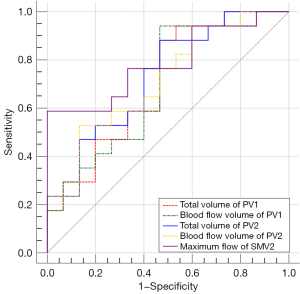
Associations of clinical data and 4D flow MRI-derived parameters with SRS/GRS outcomes
The presence of SRS/GRS was weakly correlated with TVLPV (r=−0.302; P=0.035), TVPV1 (r=−0.385; P=0.001), BFVPV1 (r=−0.360; P=0.010), MFPV1 (r=−0.341; P=0.004), TVPV2 (r=−0.301; P=0.013), MFPV2 (r=−0.291; P=0.017), and BFVSMV2 (r=0.371; P=0.008); meanwhile, the diameter of the SRS/GRS was moderately inversely correlated with ALT (r=−0.535; P<0.001) and BFVLPV (r=−0.560; P=0.008). AST levels were weakly inversely correlated with the diameter of the SRS/GRS (r=−0.321; P=0.038), and the MELD score was weakly positively correlated with the diameter of the SRS/GRS (r=0.323; P=0.039) (Table 5).
Table 5
Parameter | With/without SRS/GRS | Diameter of SRS/GRS | |||
---|---|---|---|---|---|
P | Correlation coefficient | P | Correlation coefficient | ||
TVLPV | 0.035 | −0.302* | 0.157 | −0.275 | |
TVPV1 | 0.001 | −0.385* | 0.376 | −0.140 | |
BFVPV1 | 0.010 | −0.360* | 0.335 | −0.176 | |
MFPV1 | 0.004 | −0.341* | 0.670 | −0.068 | |
TVPV2 | 0.013 | −0.301* | 0.126 | −0.237 | |
MFPV2 | 0.017 | −0.291* | 0.208 | −0.196 | |
BFVSMV2 | 0.008 | 0.371* | 0.360 | 0.170 | |
BFVLPV | 0.145 | −0.251 | 0.008 | −0.560** | |
ALT | 0.081 | −0.212 | <0.001 | −0.535** | |
AST | 0.118 | −0.190 | 0.038 | −0.321* | |
MELD | 0.368 | −0.115 | 0.039 | 0.323* |
*, mild correlation; **, moderate correlation. 4D, four-dimensional; MRI, magnetic resonance imaging; SRS, splenorenal shunt; GRS, gastrorenal shunt; TV, total volume, the volume of blood flow through the placed plane during one cardiac cycle; BFV, blood flow volume, the amount of blood that flows through a plane of a blood vessel per unit of time; MF, maximum flow, it is an instantaneous value; ALT, glutamic pyruvic transaminase; AST, glutamic oxaloacetic transaminase; MELD, model for end-stage liver disease; LPV, left portal vein; PV, portal vein; SMV, superior mesenteric vein.
Discussion
Noninvasive and quantitative assessments of the presence and severity of SRS/GRS are extremely important for early intervention and estimation of the prognosis of patients with cirrhosis. A previous case report confirmed that 4D flow MRI yields objective and visual assessment of the morphology and blood flow of the portal venous system, including the SPSS (23). In our study, we examined the relationships between SRS/GRS and 4D flow MRI-derived parameters in many cases and discovered that several 4D flow MRI-derived parameters correlate with the presence and severity of SRS/GRS. Additionally, TVPV1, BFVPV1, TVPV2, BFVPV2, and MFSMV2 were found to be independent risk factors for the development of L-SRS/GRS.
We further found that TVPV1, BFVPV1, MFPV1, TVPV2, BFVPV2, and MFSMV2 were significantly different between the W-SRS/GRS, S-SRS/GRS, and L-SRS/GRS groups. We speculate that this difference could be due mainly to microscopic changes in mechanical factors in the liver. The development of cirrhosis involves sustained hepatic inflammatory damage and leads to hepatocyte necrosis, fibrotic deposition, and nodular regenerative hyperplasia (24). In cirrhosis, hepatic resistance gradually increases, the flow resistance of the posterior sinus PV increases, the velocity in the PV decreases, and the shape and size of the PV system changes (25). A previous study demonstrated that 4D flow MR imaging-monitored flow volumes and peak velocities of the PV, SV, and SMV increase after TIPS placement (26). Indeed, the effects of SRS/GRS are analogous to those after TIPS, as this type of shunt diverts blood flow from the PV system directly into the systemic circulation, thereby reducing portal venous pressure. Subsequently, the vascular resistance decreases, resulting in faster blood flow and increased MF in the SMV. Moreover, due to SRS/GRS, there is only partial blood flow into the PV, leading to a decrease in the MF of PV1 proximal to the splenomesenteric confluence (27). For the same reason, decreased blood flow results in a decreased TV of PV1 and PV2.
Through subgroup analysis, we found that only PGmaxPV1 and PGmeanPV1 were significantly different between the W-SRS/GRS and S-SRS/GRS groups and between the S-SRS/GRS and L-SRS/GRS groups. This is likely because the blood flow changes were not as obvious between the two subgroups. Pressure was the most sensitive parameter with respect to blood flow changes, and there were only subtle differences in the PGmaxPV1 and PGmeanPV1 between the subgroups. However, relatively notable hemodynamic changes were detected between the W-SRS/GRS and L-SRS/GRS groups, resulting in significant differences between the two groups in the BFV, MF, TV, and Vmax of the LPV, PV, SV, and SMV. In contrast to MFSMV2, which increased with increasing GRS/SRS, the BFV, MF, TV, and Vmax of the PV and SV decreased with increasing SRS/GRS. The reason for this difference might attributable to greater SRS/GRS pushing blood flow from the PV and SV, causing decreases in the TV, flow velocity, and BFV, which predicates significant decreases in blood flow per unit time.
Previous studies have focused mainly on the outcome of SRS/GRS, such as the associations between SRS/GRS and liver function, cirrhosis, and HE (28-30). With respect to the causes of SRS/GRS, it is believed that liver fibrosis and alterations in cell signaling pathways and hepatic vascular cells cause increased intrahepatic vascular resistance, reversed hepatofugal flow in the PV, and consequently, the formation of collateral circulation (31). Indeed, portal flow resistance includes multiple factors, such as the BFV, TV, and flow velocity. However, it is still unclear which factor is decisive in this complex pathophysiological process. Multiple factor logistic regression analysis revealed that TVPV1, BFVPV1, TVPV2, BFVPV2, and MFSMV2 were independent risk factors for L-SRS/GRS. As TVPV1, BFVPV1, TVPV2, and BFVPV2 decrease, the risk of the formation of large shunts increases. As MFSMV2 increases, the risk of large shunt formation increases. TVPV1 and TVPV2 represent the flows that enter and leave PV1/PV2 during the cardiac cycle, respectively. BFVPV1 and BFVPV2 were defined as the blood flow passing through the PV1 and PV2, respectively, per unit of time. Decreased TV and BFV predict that portal blood flow might become partially retrograde and that blood flow through other pathways, such as the left gastric vein and SV, will increase and be followed by increased venous pressure, leading to the formation of anomalous passageways between the SV or left gastric vein and the renal vein (32). If the resistance of blood flow to the liver is high, the level of MFSMV2 and the blood flow in the left gastric vein and SV are increased, which can similarly increase the risk of L-SRS/GRS. Furthermore, we found that each risk factor has an equal weight on the L-SRS/GRS by comparing the diagnostic efficacy of each risk factor. Hence, close monitoring of portal pressure, portal TV, portal BFV, and blood flow velocity through the SMV is necessary for patients diagnosed with cirrhosis and portal hypertension. This constitutes a novel insight for the clinical treatment of patients with cirrhosis and GRS/SRS.
This study involved several limitations which should be mentioned. First, the sample size included in this study was limited, which might have led to bias. Second, the patients with cirrhosis included in the study had different etiologies, for which we lacked controls. Future studies will investigate patients with different cirrhosis etiologies. Third, due to technical limitations, the examination time was long, which might have contributed to patient motion and differences in image quality. Finally, the 4D flow MRI-derived parameters focused on large blood vessels, such as the PV, SMV, and SV. SRSs/GRSs are generally small and tortuous, so their 4D flow MRI-derived parameters cannot be easily obtained. In future research, we will seek to explore the 4D flow MRI-derived parameters of SRSs/GRSs directly. Additionally, we will continue to optimize the scanning protocol to obtain the 4D flow MRI-derived parameters of spontaneous SRSs.
Conclusions
Several 4D flow MRI-derived parameters were correlated with the presence and severity of SRS/GRS. Additionally, TVPV1, BFVPV1, TVPV2, BFVPV2, and MFSMV2 were found to be independent risk factors for L-SRS/GRS. Our results are highly relevant to providing important guidance for the formulation of specific therapeutic strategies for portal hypertension. Future prospective studies are warranted to explore the associations between 4D flow MRI-derived parameters of collateral vessels and PV in patients with liver cirrhosis.
Acknowledgments
Funding: This work was supported by
Footnote
Reporting Checklist: The authors have completed the STROBE reporting checklist. Available at https://qims.amegroups.com/article/view/10.21037/qims-24-826/rc
Conflicts of Interest: All authors have completed the ICMJE uniform disclosure form (available at https://qims.amegroups.com/article/view/10.21037/qims-24-826/coif). The authors have no conflicts of interest to declare.
Ethical Statement: The authors are accountable for all aspects of the work in ensuring that questions related to the accuracy or integrity of any part of the work are appropriately investigated and resolved. This study was conducted in accordance with the Declaration of Helsinki (as revised in 2013) and was approved by the institutional review board of the Second Affiliated Hospital of Chongqing Medical University (ethics reference No. 2021–240). Informed consent was obtained from each patient.
Open Access Statement: This is an Open Access article distributed in accordance with the Creative Commons Attribution-NonCommercial-NoDerivs 4.0 International License (CC BY-NC-ND 4.0), which permits the non-commercial replication and distribution of the article with the strict proviso that no changes or edits are made and the original work is properly cited (including links to both the formal publication through the relevant DOI and the license). See: https://creativecommons.org/licenses/by-nc-nd/4.0/.
References
- Tatsumi A, Maekawa S, Osawa L, Katoh R, Komiyama Y, Nakakuki N, et al. Spontaneous portosystemic shunt diameter predicts liver function after balloon-occluded retrograde transvenous obliteration. JGH Open 2022;6:139-47. [Crossref] [PubMed]
- Simón-Talero M, Roccarina D, Martínez J, Lampichler K, Baiges A, Low G, et al. Association Between Portosystemic Shunts and Increased Complications and Mortality in Patients With Cirrhosis. Gastroenterology 2018;154:1694-1705.e4. [Crossref] [PubMed]
- Vilstrup H, Amodio P, Bajaj J, Cordoba J, Ferenci P, Mullen KD, Weissenborn K, Wong P. Hepatic encephalopathy in chronic liver disease: 2014 Practice Guideline by the American Association for the Study of Liver Diseases and the European Association for the Study of the Liver. Hepatology 2014;60:715-35. [Crossref] [PubMed]
- Kageyama K, Yamamoto A, Jogo A, Izuta S, Himoto D, Kakimi A, Sohgawa E, Miki Y. Visualization of flow dynamics in the portal circulation using 320-detector-row computed tomography: a feasibility study. Eur Radiol Exp 2021;5:1. [Crossref] [PubMed]
- Soulat G, McCarthy P, Markl M. 4D Flow with MRI. Annu Rev Biomed Eng 2020;22:103-26. [Crossref] [PubMed]
- Reiter C, Reiter U, Kräuter C, Kolesnik E, Scherr D, Schmidt A, Fuchsjäger M, Reiter G. MR 4D flow-derived left atrial acceleration factor for differentiating advanced left ventricular diastolic dysfunction. Eur Radiol 2024;34:4065-76. [Crossref] [PubMed]
- Reiter U, Kovacs G, Reiter C, Kräuter C, Nizhnikava V, Fuchsjäger M, Olschewski H, Reiter G. MR 4D flow-based mean pulmonary arterial pressure tracking in pulmonary hypertension. Eur Radiol 2021;31:1883-93. [Crossref] [PubMed]
- Kollmeier JM, Kalentev O, van Zalk M, Voit D, Frahm J. Maximum velocity projections within 30 seconds: a combination of real-time three-directional flow MRI and cross-sectional volume coverage. Quant Imaging Med Surg 2023;13:3307-15. [Crossref] [PubMed]
- Bai X, Fu M, Li Z, Gao P, Zhao H, Li R, Sui B. Distribution and regional variation of wall shear stress in the curved middle cerebral artery using four-dimensional flow magnetic resonance imaging. Quant Imaging Med Surg 2022;12:5462-73. [Crossref] [PubMed]
- Motosugi U, Roldán-Alzate A, Bannas P, Said A, Kelly S, Zea R, Wieben O, Reeder SB. Four-dimensional Flow MRI as a Marker for Risk Stratification of Gastroesophageal Varices in Patients with Liver Cirrhosis. Radiology 2019;290:101-7. [Crossref] [PubMed]
- Stankovic Z, Rössle M, Euringer W, Schultheiss M, Salem R, Barker A, Carr J, Langer M, Markl M, Collins JD. Effect of TIPS placement on portal and splanchnic arterial blood flow in 4-dimensional flow MRI. Eur Radiol 2015;25:2634-40. [Crossref] [PubMed]
- Rutkowski DR, Reeder SB, Fernandez LA, Roldán-Alzate A. Surgical planning for living donor liver transplant using 4D flow MRI, computational fluid dynamics and in vitro experiments. Comput Methods Biomech Biomed Eng Imaging Vis 2018;6:545-55. [Crossref] [PubMed]
- Hyodo R, Takehara Y, Ishizu Y, Nishida K, Mizuno T, Ichikawa K, Horiguchi R, Kurata N, Ogura Y, Yokoyama S, Naganawa S, Jin N, Ichiba Y. Evaluation of 4D Flow MRI-Derived Relative Residence Time as a Marker for Cirrhosis Associated Portal Vein Thrombosis. J Magn Reson Imaging 2024; Epub ahead of print. [Crossref]
- Hyodo R, Takehara Y, Mizuno T, Ichikawa K, Horiguchi R, Kawakatsu S, Mizuno T, Ebata T, Naganawa S, Jin N, Ichiba Y. Four-dimensional Flow MRI Assessment of Portal Hemodynamics and Hepatic Regeneration after Portal Vein Embolization. Radiology 2023;308:e230709. [Crossref] [PubMed]
- Iwata K, Sekine T, Matsuda J, Tachi M, Imori Y, Amano Y, Ando T, Obara M, Crelier G, Ogawa M, Takano H, Kumita S. Measurement of Turbulent Kinetic Energy in Hypertrophic Cardiomyopathy Using Triple-velocity Encoding 4D Flow MR Imaging. Magn Reson Med Sci 2024;23:39-48. [Crossref] [PubMed]
- Dyverfeldt P, Trenti C, Ziegler M, Bjarnegård N, Lindenberger M. Helical flow in tortuous aortas and its relationship to turbulence: A whole-aorta 4D flow MRI study. Front Cardiovasc Med 2023;10:1124604. [Crossref] [PubMed]
- Bane O, Peti S, Wagner M, Hectors S, Dyvorne H, Markl M, Taouli B. Hemodynamic measurements with an abdominal 4D flow MRI sequence with spiral sampling and compressed sensing in patients with chronic liver disease. J Magn Reson Imaging 2019;49:994-1005. [Crossref] [PubMed]
- Saks K, Jensen KK, McLouth J, Hum J, Ahn J, Zaman A, Chang MF, Fung A, Schlansky B. Influence of spontaneous splenorenal shunts on clinical outcomes in decompensated cirrhosis and after liver transplantation. Hepatol Commun 2018;2:437-44. [Crossref] [PubMed]
- Moubarak E, Bouvier A, Boursier J, Lebigot J, Ridereau-Zins C, Thouveny F, Willoteaux S, Aubé C. Portosystemic collateral vessels in liver cirrhosis: a three-dimensional MDCT pictorial review. Abdom Imaging 2012;37:746-66. [Crossref] [PubMed]
- Krittian SB, Lamata P, Michler C, Nordsletten DA, Bock J, Bradley CP, Pitcher A, Kilner PJ, Markl M, Smith NP. A finite-element approach to the direct computation of relative cardiovascular pressure from time-resolved MR velocity data. Med Image Anal 2012;16:1029-37. [Crossref] [PubMed]
- Lai LM, Cheng JY, Alley MT, Zhang T, Lustig M, Vasanawala SS. Feasibility of ferumoxytol-enhanced neonatal and young infant cardiac MRI without general anesthesia. J Magn Reson Imaging 2017;45:1407-18. [Crossref] [PubMed]
- Feneis JF, Kyubwa E, Atianzar K, Cheng JY, Alley MT, Vasanawala SS, Demaria AN, Hsiao A. 4D flow MRI quantification of mitral and tricuspid regurgitation: Reproducibility and consistency relative to conventional MRI. J Magn Reson Imaging 2018;48:1147-58. [Crossref] [PubMed]
- Hyodo R, Takehara Y, Mizuno T, Ichikawa K, Ishizu Y, Sugiyama M, Naganawa S. Time-resolved 3D cine phase-contrast magnetic resonance imaging (4D-flow MRI) can quantitatively assess portosystemic shunt severity and confirm normalization of portal flow after embolization of large portosystemic shunts. Hepatol Res 2021;51:343-9. [Crossref] [PubMed]
- Rawla P, Sunkara T, Muralidharan P, Raj JP. Update in global trends and aetiology of hepatocellular carcinoma. Contemp Oncol (Pozn) 2018;22:141-50. [Crossref] [PubMed]
- Nardelli S, Riggio O, Gioia S, Puzzono M, Pelle G, Ridola L. Spontaneous porto-systemic shunts in liver cirrhosis: Clinical and therapeutical aspects. World J Gastroenterol 2020;26:1726-32. [Crossref] [PubMed]
- Bannas P, Roldán-Alzate A, Johnson KM, Woods MA, Ozkan O, Motosugi U, Wieben O, Reeder SB, Kramer H. Longitudinal Monitoring of Hepatic Blood Flow before and after TIPS by Using 4D-Flow MR Imaging. Radiology 2016;281:574-82. [Crossref] [PubMed]
- Oshita K, Ohira M, Honmyo N, Kobayashi T, Murakami E, Aikata H, Baba Y, Kawano R, Awai K, Chayama K, Ohdan H. Treatment outcomes after splenectomy with gastric devascularization or balloon-occluded retrograde transvenous obliteration for gastric varices: a propensity score-weighted analysis from a single institution. J Gastroenterol 2020;55:877-87. [Crossref] [PubMed]
- Yi F, Guo X, Wang L, Xu X, An Y, Tang Y, Zhang W, Tacke F, Arora A, Qi X. Impact of spontaneous splenorenal shunt on liver volume and long-term survival of liver cirrhosis. J Gastroenterol Hepatol 2021;36:1694-702. [Crossref] [PubMed]
- Liu G, Wang X, Luo X. Splenic Arteriovenous Fistula Coexisting With Spontaneous Splenorenal Shunt in a Cirrhotic Patient. Clin Gastroenterol Hepatol 2023;21:A33. [Crossref] [PubMed]
- Ke Q, Wang Z, Huang X, Li L, Wu W, Qiu L, Jiao Y, Xie Y, Peng X, Liu J, Guo W. Splenic vein embolization as a feasible treatment for patients with hepatic encephalopathy related to large spontaneous splenorenal shunts. Ann Hepatol 2022;27:100725. [Crossref] [PubMed]
- Iwakiri Y, Shah V, Rockey DC. Vascular pathobiology in chronic liver disease and cirrhosis - current status and future directions. J Hepatol 2014;61:912-24. [Crossref] [PubMed]
- Yokoyama K, Yamauchi R, Shibata K, Fukuda H, Kunimoto H, Takata K, Tanaka T, Inomata S, Morihara D, Takeyama Y, Shakado S, Sakisaka S. Endoscopic treatment or balloon-occluded retrograde transvenous obliteration is safe for patients with esophageal/gastric varices in Child-Pugh class C end-stage liver cirrhosis. Clin Mol Hepatol 2019;25:183-9. [Crossref] [PubMed]