Combination of single-source dual-energy computed tomography (CT) parameters and extracellular volume fraction for predicting lymphovascular and perineural invasion in colorectal cancer
Introduction
Colorectal cancer (CRC) is the third most prevalent cancer and the second leading cause of cancer-related deaths globally (1). Lymphovascular invasion (LVI) and perineural invasion (PNI) are important histopathological variables that are directly related to CRC survival and recurrence (2,3). The involvement of tumour cells in small endothelium-lined lymphatic or vascular channels, known as LVI, is considered a critical and early step in tumour metastasis (4,5). PNI, which is characterized by the growth of tumour cells within nerves and their sheaths, serves as a pathological indicator for a more aggressive tumour phenotype (6,7). Accumulating evidence has demonstrated that either one or both of them indicate an unfavorable prognosis for CRC (7,8). In addition, because LVI/PNI is also associated with lymph node involvement in T1 and T2 tumours (9), the National Comprehensive Cancer Network (NCCN) and American Society of Clinical Oncology (ASCO) clinical practice guidelines currently include LVI/PNI as a consideration for adjuvant chemotherapy in patients with stage II colon cancer (7). LVI/PNI can also serve as a factor in determining whether the duration of adjuvant chemotherapy is sufficient (10). However, LVI/PNI status is currently mainly identified using postoperative pathological analysis. Hence, a non-invasive preoperative assessment method for LVI and PNI should be explored for risk stratification and individualised treatment of patients with CRC.
Recently, there has been a great deal of interest in relating radiomics features extracted from radiographic images, such as computed tomography (CT), magnetic resonance imaging (MRI) and 18F-fluorodeoxyglucose positron emission tomography, to disease aggressiveness. The potential of radiomics in predicting the LVI/PNI status in patients with CRC was reported (11-13); however, their results are not currently available for clinical use because of the lack of standardised imaging protocols and radiomics workflows, which results in extensive heterogeneity among different models. Few studies have focused on predicting LVI/PNI status in CRC using conventional preoperative imaging. Wu et al. (14) examined the correlation between the diameter of the superior hemorrhoidal vein on preoperative CT and the presence of LVI. Chen et al. (15) demonstrated that tumour volumetry data, particularly gross tumour volume based on diffusion-weighted MRI, are associated with both LVI and lymph node metastases in resectable rectal cancer. However, due to insufficient validation in large-scale studies, these criteria for a preoperative image-based diagnosis of LVI in CRC are currently not widely recognized. With the introduction of material characterisation and decomposition functions, dual-energy CT is promising for improving the diagnostic accuracy of CRC grading, metastatic lymph node identification, neoadjuvant therapy response evaluation, and microsatellite instability status evaluation (16-19). Iodine concentration (IC) and effective atomic number in venous phase (VP) derived from spectral CT are useful for the preoperative evaluation of LVI and PNI in gastric cancer (20); however, no relevant research has been reported on CRC.
The extracellular volume (ECV) fraction, which comprises the intravascular space and extravascular ECV fractions, has been widely used in noninvasive assessment of pathologies, such as cardiac and hepatic fibrosis (21,22). The ECV fraction can be accurately quantified from dual-energy CT iodine density images without the need for registration between the pre- and post-contrast images (23). The ECV fraction is also a quantitative biomarker that can be used to assess the volume of the extracellular matrix (ECM) of solid tumours, an important component of the tumour microenvironment (TME). Song et al. (24) found in their study on the prognosis of patients with stage III CRC that the formation of cancer emboli in blood vessels and lymphatics was closely related to the immunosuppressive TME. To our knowledge, no previous study has explored the association between the ECV fraction and LVI/PNI status in patients with CRC. Therefore, this study aimed to investigate the value of combining the aforementioned dual-energy CT parameters and ECV fraction for predicting LVI/PNI in CRC. We present this article in accordance with the STARD reporting checklist (available at https://qims.amegroups.com/article/view/10.21037/qims-24-76/rc).
Methods
Patients
The study was conducted in accordance with the Declaration of Helsinki (as revised in 2013). The study was approved by the Ethics Committee of The Second Hospital of Lanzhou University (No. 2022A-007) and individual consent for this retrospective analysis was waived. Patients who underwent surgical resection and received a pathological diagnosis of CRC between June 2020 and March 2023 were included in this study. The inclusion criteria included: (I) patients diagnosed with CRC and definite LVI and PNI information; (II) who had undergone a routine abdominal contrast-enhanced dual-energy CT examination within 2 weeks prior to surgical resection; (III) without receiving any local or systemic therapy before the CT examination or surgery. The exclusion criteria were as follows: (I) patients with imperceptible target lesions on CT images; (II) poor image quality (including low signal-to-noise ratio or severe bowel motion artifacts); and (III) incomplete clinical information.
According to the postoperative pathological results, patients with LVI and/or PNI were categorized as positive for LVI/PNI (LVI/PNI-positive group), while patients without LVI and PNI were classified as negative for LVI/PNI (LVI/PNI-negative group).
Clinicopathological variables
Clinical data, including demographic information (sex and age), blood routine indexes [hematocrit, neutrophil-to-lymphocyte ratio (NLR), platelet-to-lymphocyte ratio (PLR), lymphocyte-to-monocyte ratio (LMR)], serum tumour markers [carcinoembryonic antigen (CEA), carbohydrate antigen 19-9 (CA19-9), carbohydrate antigen 125 (CA125), and alpha-fetoprotein (AFP)], and colonoscopic pathology [histological grade, Ki-67 index, DNA mismatch repair (MMR) status] were collected and calculated using a picture archiving and communication system.
Single-source dual-energy CT (ssDECT) imaging
All examinations were performed using an ssDECT scanner in gemstone spectral imaging (GSI) mode, specifically the 256-slice Revolution CT scanner manufactured by GE Healthcare. The scanning parameters for ssDECT were as follows: rapid switching between tube voltages of 80 and 140 kVp; automatic regulation of tube current; helical pitch of 0.992; rotation speed of 0.6 s/rot; field of view measuring 500 mm × 500 mm; image matrix size of 512×512; slice thickness/gap set at 5/5 mm; and reconstruction thickness at 1.25 mm. After a routine non-enhanced CT scan, the non-ionic contrast media (Iopamidol, 370 mg I/mL, Bracco) was injected at a dose of 1.2 mL/kg and an injection rate of 3.0–4.0 mL/s through the median cubital vein using a high-pressure syringe, followed by flushing with 30 mL saline at the same rate. Contrast-enhanced CT images were obtained at 30 s, 60 s, and 3 min after the injection of contrast agent for arterial phase (AP), VP, and delayed phase (DP) respectively. Furthermore, a 40% Asir-V iteration was employed to horizontally reconstruct the three-phase spectral-enhanced images at the conclusion of the scan.
Image analysis and data measurement
Two types of images were reconstructed from a single ssDECT scan for analysis: water and iodine-based material decomposition images, and virtual monochromatic images obtained within the photon energy range of 40 to 140 keV. All images were reconstructed with a slice thickness of 1.25 mm and then transferred to Advanced Workstation 4.7 (GE Healthcare, IL, USA) for measurement and analysis using the GSI Viewer software. Two radiologists, with 20 and 6 years of experience in abdominal imaging respectively, independently analyzed the images while being blinded to the histopathological findings. First, the two radiologists observed and recorded conventional imaging features (tumour location and maximal diameter) and evaluated the T and N stages by referring to the 8th edition of the American Joint Committee on Cancer (AJCC) staging system. Secondly, they chose AP monochrome images at 70 keV to delineate the regions of interest (ROIs) on sections with the largest axial diameter of the tumour (mean area 34.26 mm2; range, 10.58–153.85 mm2). The aim was to encompass as much of the solid tumour area as possible while avoiding significant necrotic regions. The measurements were conducted on the adjacent upper and lower slices, and then they were averaged to minimize measurement bias. Finally, they applied the corresponding ROIs to images of all photon levels, as well as iodine-based material decomposition images in VP and DP for consistency.
An ROI data file was saved for each measurement, which contains monochromatic CT values at different energy levels ranging from 40 to 140 keV (with a 5 keV increment) and the effective atomic number (Eff-Z) value of the tumour. The monochromatic CT values were utilized as a function of various energy levels to generate a spectral Hounsfield unit (HU) curve (where the x-axis represents photon energy ranging from 40 to 140 keV, and the y-axis represents the average CT value). The slope of the spectral HU curve was then calculated as follows: slope = (CT value at 40 keV − CT value at 100 keV)/60 (19). The IC of tumour was measured on the iodine-based material decomposition image. In order to minimize the variations caused by individual differences in cardiac function and hemodynamics among patients, the arterial IC of the same tumour slice was measured (external iliac artery for rectal cancer patients and abdominal aorta for colon cancer patients), and the normalized iodine concentration (NIC) of the tumour was calculated using the following formula: NIC = ICtumour/ICartery. The ECV fraction of the tumour was calculated as follows: ECV (%) = (1 − hematocrit) × (ICtumour/ICartery) × 100% (23), where hematocrit measurements were obtained within a 3-day period prior to the examination. To mitigate potential measurement bias, the final results were obtained by averaging measurements from two radiologists. Simultaneously, rigorous testing was carried out to ensure the consistency of the raw data.
Pathological examination
Each tumour resection specimen was independently reviewed by two pathologists (with 18 and 5 years of experience, respectively). Hematoxylin and eosin staining was used to evaluate the LVI/PNI status, while CD31 immunohistochemical staining was employed for further identification of blood vessels. The presence of tumour cells within the small endothelium-lined lymphatic or vascular channels was diagnosed as LVI. The diagnosis of PNI indicated the presence of tumour infiltration within nerves and their surrounding sheaths. Furthermore, the histological grade, MMR proteins, and lymph node metastasis were evaluated in accordance with current guidelines.
Statistical analysis
The statistical analyses were conducted using SPSS 25.0 software (IBM, Armonk, NY, USA), and statistical significance was determined with a P value threshold of less than 0.05 (two-tailed). The mean ± standard deviation () was used to represent normally distributed data, while the median (25% quartile, 75% quartile) [M (Q1, Q3)] was employed for non-normally distributed data. The two-sample t-test was employed to compare data that followed a normal distribution, whereas the Mann-Whitney U test was utilized for comparing non-normally distributed data. The comparison of categorical data was conducted using either the Chi-square or Fisher’s exact probability test. We employed binary logistic regression to construct models incorporating multiple parameters. The performance of various parameters or models in predicting LVI/PNI in CRC was analyzed using receiver operating characteristic (ROC) curves. The area under the curves (AUCs), sensitivities, specificities, accuracies, positive/negative predictive values (PPVs/NPVs), and positive/negative likelihood ratios (+LRs/−LRs) were calculated. The 95% confidence intervals (CIs) were also computed to evaluate the discriminative capacities of these parameters in distinguishing between the two groups. The Youden index was employed to ascertain the optimal cut-off values. Moreover, considering the imbalance between the two cohorts, we utilized the F1 score to compare and evaluate the performance of different parameters [F1 score = 2 × precision × recall/(precision + recall)].
The inter-reader reliability in measuring quantitative parameters was assessed based on the intraclass correlation coefficient (ICC) and its corresponding 95% CI. ICC values below 0.5 indicate inadequate reliability, while values between 0.5 and 0.75 suggest moderate reliability, those between 0.75 and 0.9 imply good reliability, and values above 0.90 demonstrate excellent reliability.
Results
Patients
The study comprised a cohort of 118 patients (82 men and 36 women; mean age: 61±11.04 years, range, 27–82 years). Among them, both LVI and PNI were detected in 68 patients, while LVI alone was observed in 15 patients and PNI alone was identified in 2 patients. Consequently, the LVI/PNI-positive group encompassed a total of 85 patients (85/118, 72.03%), whereas the LVI/PNI-negative group consisted of 33 patients (33/118, 27.97%). The flowchart of the patient selection process is illustrated in Figure 1.
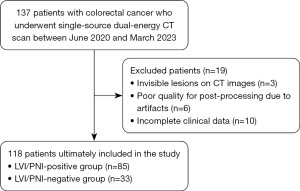
Clinicopathological variables
The LVI/PNI-positive and LVI/PNI-negative groups did not show any significant differences in terms of sex, age, tumour location or maximum diameter. The incidence of T3–4 and N1–2 CRCs was significantly higher in the LVI/PNI-positive group (72/85, 45/85) compared to the -negative group (20/33, 7/33) (P=0.010, P=0.007). The LVI/PNI-positive group had a higher proportion of patients (44/85) with serum CEA levels ≥5 ng/mL compared to the -negative group (10/33) (P=0.036). The levels of Serum AFP, CA125, and CA19-9 did not exhibit any significant differences between the two groups. Similarly, no significant variations were observed in the NLR, PLR, and LMR values between the two groups. The incidence of poorly differentiated CRCs was significantly higher in the LVI/PNI-positive group (21/85) compared to the -negative group (1/33) (P=0.007). The Ki-67 index and MMR status did not differ significantly between the two groups. The clinical data of the patients are presented in Table 1.
Table 1
Variables | LVI/PNI-positive group (n=85; 72.03%) | LVI/PNI-negative group (n=33; 27.97%) | P value |
---|---|---|---|
Age (years) | 61.38±10.99 | 60.30±10.74 | 0.633 |
Sex | 0.976 | ||
Female | 26 | 10 | |
Male | 59 | 23 | |
Tumour location | 0.912 | ||
Rectum | 42 | 15 | |
Left hemi-colon | 25 | 10 | |
Right hemi-colon | 18 | 8 | |
Maximum diameter (cm) | 5.00 (4.00, 5.90) | 5.00 (3.15, 6.50) | 0.648* |
CEA (ng/mL) | 0.036 | ||
<5 | 41 | 23 | |
≥5 | 44 | 10 | |
AFP (ng/mL) | 2.69 (2.01, 3.86) | 2.64 (2.14, 3.73) | 0.890* |
CA125 (U/mL) | 11.30 (8.17, 15.40) | 9.37 (7.05, 15.65) | 0.430* |
CA19-9 (U/mL) | 12.00 (6.67, 20.00) | 9.83 (6.22, 17.30) | 0.280* |
NLR | 2.63 (1.73, 2.63) | 2.39 (1.89, 4.10) | 0.841* |
PLR | 151.47 (105.50, 209.74) | 118.11 (95.73, 224.50) | 0.492* |
LMR | 3.52 (2.59, 4.69) | 2.94 (2.18, 4.42) | 0.234* |
Histological grade | 0.007 | ||
Well differentiated | 12 | 8 | |
Moderately differentiated | 52 | 24 | |
Poorly differentiated | 21 | 1 | |
Ki-67 index | 0.80 (0.70, 0.80) | 0.80 (0.70, 0.80) | 0.682* |
MMR | 0.765 | ||
pMMR | 78 | 29 | |
dMMR | 7 | 4 | |
T stage | 0.010 | ||
T1 | 2 | 6 | |
T2 | 11 | 7 | |
T3 | 59 | 18 | |
T4 | 13 | 2 | |
N stage | 0.007 | ||
N0 | 40 | 26 | |
N1 | 28 | 5 | |
N2 | 17 | 2 |
Quantitative data are reported as means ± standard deviations or medians with interquartile ranges; using the t-test (* using Mann-Whitney U test). Qualitative variables are reported as raw numbers; using the Chi-squared test. LVI/PNI, lymphovascular invasion/perineural invasion; CEA, carcinoembryonic antigen; AFP, alpha-fetoprotein; CA125, carbohydrate antigen 125; CA19-9, carbohydrate antigen 19-9; NLR, neutrophil-to-lymphocyte ratio; PLR, platelet-to-lymphocyte ratio; LMR, lymphocyte-to-monocyte ratio; MMR, DNA mismatch repair; dMMR, deficient mismatch repair; pMMR, proficient mismatch repair.
Comparative analysis of ssDECT-derived parameters
The consistent measurement results of the two radiologists (all ICCs >0.75) indicate good inter-observer agreement (Table 2). In the VP, the IC, NIC, slope and Eff-Z of the LVI/PNI-positive group [17.96 (14.96, 20.53), 0.52±0.14, 2.26 (1.75, 2.47), 8.66±0.23] were significantly higher than those of the -negative group [16.04 (13.46, 18.43), 0.45± 0.16, 1.91 (1.68, 2.21), 8.55±0.28] (P=0.038, P=0.019, P=0.031 and P=0.022). In the DP, the IC, NIC, slope, Eff-Z and ECV of the LVI/PNI-positive group [17.52±3.75, 0.75 (0.69, 0.87), 2.13±0.45, 8.63±0.21, 0.40 (0.32, 0.46)] were significantly higher than those of the -negative group [14.78±4.04, 0.62 (0.50, 0.74), 1.79±0.49, 8.48±0.23, 0.32 (0.28, 0.39)] (P=0.001, P<0.001, P<0.001, P=0.001 and P=0.001). There was no significant difference in the parameters mentioned above between the two groups during the AP (Table 3, Figure 2). The representative images obtained from ssDECT for the LVI/PNI-positive and -negative groups are illustrated in Figures 3 and 4, respectively.
Table 2
Variables | Reader 1 | Reader 2 | ICC (95% CI) |
---|---|---|---|
IC AP (100 μg/cm3) | 14.62±4.30 [1.14–25.78] | 14.67±4.54 [2.24–27.36] | 0.825 (0.756–0.874) |
NIC AP | 0.135±0.046 [0.032–0.374] | 0.139±0.049 [0.026–0.298] | 0.842 (0.781–0.886) |
Slope AP | 1.74±0.44 [0.14–3.85] | 1.82±0.56 [0.16–4.07] | 0.769 (0.683–0.832) |
Eff-Z AP | 8.44±0.29 [7.54–9.24] | 8.46±0.33 [7.58–9.26] | 0.896 (0.783–0.935) |
IC VP (100 μg/cm3) | 16.92±5.68 [2.22–29.51] | 17.08±5.30 [3.54–33.75] | 0.786 (0.692–0.847) |
NIC VP | 0.48±0.19 [0.10–0.92] | 0.51±0.15 [0.13–0.96] | 0.814 (0.706–0.882) |
Slope VP | 2.11±0.43 [0.24–4.13] | 2.05±0.56 [0.26–3.56] | 0.796 (0.703–0.876) |
Eff-Z VP | 8.59±0.27 [7.66–9.38] | 8.62±0.22 [7.64–9.42] | 0.885 (0.776–0.924) |
IC DP (100 μg/cm3) | 16.10±4.22 [3.47–27.29] | 16.19±3.54 [3.28–26.81] | 0.819 (0.750–0.869) |
NIC DP | 0.67±0.24 [0.22–1.24] | 0.71±0.19 [0.26–1.25] | 0.816 (0.711–0.872) |
Slope DP | 1.99±0.44 [0.38–3.23] | 1.95±0.50 [0.41–3.17] | 0.806 (0.719–0.894) |
Eff-Z DP | 8.55±0.20 [7.79–9.07] | 8.56±0.25 [8.04–9.14] | 0.945 (0.916–0.971) |
Quantitative data are reported as means ± standard deviations; numbers in square brackets are ranges. Reader 1 refers to senior radiologist with 20 years of experience (J.Z.). Reader 2 refers to junior radiologist with 6 years of experience (S.L.). ICC, intraclass correlation coefficient; CI, confidence interval; IC, iodine concentration; AP, arterial phase; NIC, normalized iodine concentration; Slope, slope of spectral Hounsfield unit curve; Eff-Z, effective atomic number; VP, venous phase; DP, delayed phase.
Table 3
Variables | LVI/PNI-positive group (n=85; 72.03%) |
LVI/PNI-negative group (n=33; 27.97%) |
P value |
---|---|---|---|
IC AP (100 μg/cm3) | 15.09±4.32 | 14.13±5.56 | 0.322 |
NIC AP | 0.14±0.05 | 0.13±0.05 | 0.297 |
Slope AP | 1.82±0.52 | 1.71±0.68 | 0.371 |
Eff-Z AP | 8.47±0.25 | 8.43±0.30 | 0.461 |
ECV AP | 0.065 (0.052, 0.083) | 0.056 (0.047, 0.090) | 0.513* |
IC VP (100 μg/cm3) | 17.96 (14.96, 20.53) | 16.04 (13.46, 18.43) | 0.038* |
NIC VP | 0.52±0.14 | 0.45±0.16 | 0.019 |
Slope VP | 2.26 (1.75, 2.47) | 1.91 (1.68, 2.21) | 0.031* |
Eff-Z VP | 8.66±0.23 | 8.55±0.28 | 0.022 |
ECV VP | 0.27 (0.21, 0.33) | 0.21 (0.17, 0.36) | 0.075* |
IC DP (100 μg/cm3) | 17.52±3.75 | 14.78±4.04 | 0.001 |
NIC DP | 0.75 (0.69, 0.87) | 0.62 (0.50, 0.74) | 0.000* |
Slope DP | 2.13±0.45 | 1.79±0.49 | 0.000 |
Eff-Z DP | 8.63±0.21 | 8.48±0.23 | 0.001 |
ECV DP | 0.40 (0.32, 0.46) | 0.32 (0.28, 0.39) | 0.001* |
Quantitative data are reported as means ± standard deviations or medians with interquartile ranges; using the t-test (* using Mann-Whitney U test). CT, computed tomography; ECV, extracellular volume; LVI/PNI, lymphovascular invasion/perineural invasion; IC, iodine concentration; AP, arterial phase; NIC, normalized iodine concentration; Slope, slope of spectral Hounsfield unit curve; Eff-Z, effective atomic number; VP, venous phase; DP, delayed phase.
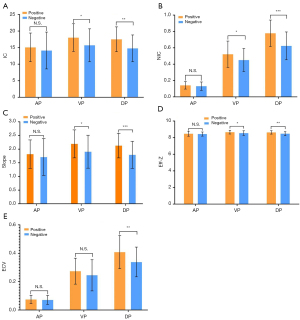
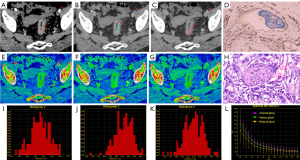
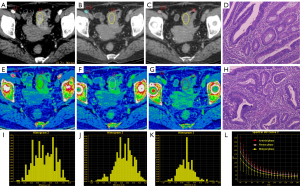
ROC-curve analysis
The discriminative capacities of the parameters that exhibit statistical disparities are demonstrated in Table 4 and Figure 5. The T stage showed high sensitivity (84.7%) and specificity (93.9%). The N stage exhibited an AUC of 0.664, with a sensitivity of 52.9% and a specificity of 78.8%. Although the histological grade had low sensitivity at 24.7%, its specificity remained high at 97%. Notably, NIC in DP achieved the highest AUC value of 0.750, accompanied by corresponding sensitivity and specificity of 74.1% and 66.7%, respectively.
Table 4
Variables | AUC (95% CI) | Youden index | Cut-off value | Sensitivity (95% CI) (%) | Specificity (95% CI) (%) |
---|---|---|---|---|---|
Clinical characteristics | |||||
CEA (ng/mL) | 0.607 (0.496–0.719) | 0.215 | 1.50 | 51.8 (41.3–62.1) | 69.7 (52.7–82.6) |
Histological grade | 0.631 (0.526–0.736) | 0.217 | 2.50 | 24.7 (16.3–35.5) | 97.0 (82.5–99.8) |
T stage | 0.650 (0.536–0.765) | 0.241 | 2.50 | 84.7 (74.9–91.3) | 93.9 (78.4–98.9) |
N stage | 0.664 (0.560–0.768) | 0.317 | 0.50 | 52.9 (41.9–63.7) | 78.8 (60.6–90.4) |
Dual-energy CT parameters | |||||
IC VP (100 μg/cm3) | 0.623 (0.510–0.737) | 0.263 | 16.95 | 56.5 (45.3–67.1) | 69.7 (51.1–83.8) |
NIC VP | 0.647 (0.527–0.768) | 0.314 | 0.49 | 64.7 (53.5–74.6) | 66.7 (48.1–81.5) |
Slope VP | 0.629 (0.516–0.741) | 0.317 | 2.23 | 52.9 (41.9–63.8) | 78.8 (60.6–90.4) |
Eff-Z VP | 0.611 (0.498–0.723) | 0.220 | 8.61 | 55.3 (44.2–66.0) | 66.7 (48.1–81.5) |
IC DP (100 μg/cm3) | 0.686 (0.576–0.796) | 0.353 | 16.84 | 56.5 (45.3–67.1) | 78.8 (60.6–90.4) |
NIC DP | 0.750 (0.648–0.852) | 0.408 | 0.70 | 74.1 (63.3–82.7) | 66.7 (48.1–81.5) |
Slope DP | 0.686 (0.578–0.792) | 0.339 | 2.01 | 61.2 (50.0–71.4) | 72.7 (54.2–86.1) |
Eff-Z DP | 0.687 (0.579–0.795) | 0.330 | 8.66 | 48.2 (37.4–59.3) | 84.9 (67.3–94.3) |
ECV DP | 0.689 (0.580–0.798) | 0.329 | 0.33 | 75.3 (64.5–83.7) | 57.6 (39.4–74.1) |
AUC, area under the curve; CI, confidence interval; CEA, carcinoembryonic antigen; IC, iodine concentration; VP, venous phase; NIC, normalized iodine concentration; Slope, slope of spectral Hounsfield unit curve; Eff-Z, effective atomic number; DP, delayed phase; ECV, extracellular volume.
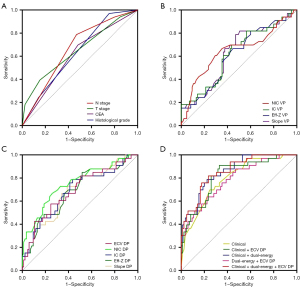
The diagnostic efficacies of the combinations of clinical parameters, dual-energy parameters, or ECV DP were found to be higher than those of individual parameters (Table 5, Figure 5). The combination of ECV DP with clinical and dual-energy parameters demonstrated the highest discriminative capability [AUC =0.857 (95% CI: 0.786–0.928); F1 score =0.833; accuracy =77.97%; sensitivity =76.47% (95% CI: 65.80–84.71%); specificity =81.82% (95% CI: 63.92–93.38%); PPV =91.55% (95% CI: 81.89–96.52%); NPV =57.45% (95% CI: 42.26–71.43%), +RL =4.21 (95% CI: 2.02–8.77), and −RL =0.29 (95% CI: 0.19–0.43)]. The combination of clinical and dual-energy parameters also demonstrated a significant evaluation value [AUC =0.854 (95% CI: 0.784–0.925); F1 score =0.836; accuracy =66.22%; sensitivity =77.65% (95% CI: 67.07–85.68%); specificity =78.79% (95% CI: 60.60–90.37%); PPV =90.41% (95% CI: 80.76–95.73%); NPV =57.78% (95% CI: 42.24–72.01%); +RL =3.66 (95% CI: 1.88–7.13); −RL =0.28 (95% CI: 0.19–0.43)]. Additionally, the combination of clinical parameters and ECV DP demonstrated the highest accuracy (79.19%) and specificity [90.90% (95% CI: 74.53–97.62%)].
Table 5
Models | Clinical | Dual-energy + ECV DP |
Clinical + dual-energy |
Clinical + ECV DP |
Clinical + dual-energy + ECV DP |
---|---|---|---|---|---|
AUC | 0.792 (0.705–0.879) | 0.784 (0.693–0.876) | 0.854 (0.784–0.925) | 0.819 (0.735–0.902) | 0.857 (0.786–0.928) |
Cut-off value | 0.682 | 0.679 | 0.726 | 0.786 | 0.743 |
F1 score | 0.805 | 0.810 | 0.836 | 0.737 | 0.833 |
Accuracy (%) | 64.19 | 66.18 | 66.22 | 79.19 | 77.97 |
Sensitivity (%) | 75.29 (64.53–83.73) | 77.65 (67.07–85.68) | 77.65 (67.07–85.68) | 67.06 (55.92–76.64) | 76.47 (65.80–84.71) |
Specificity (%) | 69.70 (51.12–83.80) | 63.64 (45.14–79.04) | 78.79 (60.60–90.37) | 90.90 (74.53–97.62) | 81.82 (63.92–93.38) |
PPV (%) | 86.51 (76.13–93.0) | 84.62 (74.27–91.46) | 90.41 (80.76–95.73) | 95.04 (85.18–98.70) | 91.55 (81.89–96.52) |
NPV (%) | 52.32 (36.91–67.33) | 15.38 (8.54–25.73) | 57.78 (42.24–72.01) | 51.72 (38.35–64.87) | 57.45 (42.26–71.43) |
+LR | 2.49 (1.46–4.23) | 2.14 (1.34–3.40) | 3.66 (1.88–7.13) | 7.38 (2.48–21.92) | 4.21 (2.02–8.77) |
−LR | 0.35 (0.24–0.53) | 0.35 (0.23–0.54) | 0.28 (0.19–0.43) | 0.36 (0.27–0.49) | 0.29 (0.19–0.43) |
Numbers in parentheses are 95% confidence intervals. Clinical = CEA + Histological grade + T stage + N stage; Dual-energy = IC VP + NIC VP + Eff-Z VP + Slope VP + IC DP + NIC DP + Eff-Z DP + Slope DP; ECV, extracellular volume; DP, delayed phase; AUC, area under the curve; F1 score = 2 × precision × recall/(precision + recall); PPV, positive predictive value; NPV, negative predictive value; +LR, positive likelihood ratio; −LR, negative likelihood ratio; CEA, carcinoembryonic antigen.
Discussion
Preoperative prediction of LVI and PNI status in CRC is helpful in selecting patients requiring appropriate adjuvant therapy and evaluating prognosis (8,10). Our study revealed the clinicopathological variables (CEA level, histological grade, T and N stages), ssDECT-derived parameters (IC, NIC, slope and Eff-Z) in the VP and DP, as well as ECV in the DP, were all associated with the LVI/PNI status in patients with CRC. The integration of multiple ssDECT imaging parameters and clinicopathological variables yielded a relatively high diagnostic accuracy in distinguishing the presence of LVI/PNI from its absence.
We found that LVI/PNI was associated with advanced CRC (including more advanced T and N stages), poor tumour differentiation and higher serum CEA level. These results are consistent with those of previous studies (3,7). A study has also confirmed that as the degree of differentiation decreases, tumour microvascular infiltration increases because the aggressiveness of tumour cells is inversely proportional to the degree of differentiation (25). In addition, it has been reported that CEA plays a key role in mediating the CEA-specific T-cell responses which facilitate the process of tumour growth and invasion by inducing a loss of mucosal integrity with increased epithelial leakiness (26). Therefore, preoperative serum CEA level, histological grade, T and N stages may contribute to the evaluation of LVI/PNI status in CRC.
The study investigated the value of ssDECT-derived parameters in predicting the presence of LVI and PNI in patients with CRC. The results demonstrated significant increases in ssDECT-derived parameters both in the VP and DP among tumours positive for LVI/PNI compared to those negative for LVI/PNI. Angiogenesis is fundamental to the growth, invasion and metastasis of CRC, which can be reflected by the iodine content measurement in ssDECT (27). Generally, the higher IC and NIC values indicate the higher blood supply to lesions (28). It has been confirmed by Chen et al. (29) that the biphasic NICs showed a significant positive linear relationship with MVD in primary gastric carcinoma. A previous study (30) has reported a significant association between LVI-positive tumours and tumour angiogenesis, indicating that the incomplete walls of tumour neovascularization exhibit higher permeability compared to healthy vessels. This further enhances the blood supply to the tumour. Hence, we concluded that the higher IC and NIC values are indicators of LVI/PNI. Moreover, in the VP and DP, the diagnostic performance of NIC values was higher than that of IC values, possibly because NIC reduced technical or physiological variabilities in iodine load within the tissue of interest caused by changes in phase times and cardiac output. The IC is static and reflects the iodine content in the tumour, while the spectral HU curve shows changes in tumour attenuation after contrast enhancement. The steepness of the spectral HU curve is positively correlated with the iodine content within the tumour. Several studies (31,32) reported that malignant lesions have steeper curves and higher slope values in contrast to benign lesions, and our results are similar to these findings. Eff-Z reflects the average atomic number of compounds composed of different components: the denser the compound, the higher the Eff-Z (33). The values of Eff-Z in the VP and DP were found to be significantly higher in the LVI/PNI-positive group compared to the LVI/PNI-negative group in our study. These differences may be explained by tumour thrombus formation in small lymphatic or vascular channels in LVI-positive patients caused by the accumulation of tumour cells or polymerisation with platelets and red blood cells (34). Tumour thrombi increase the mass of lesions, leading to changes in Eff-Zs. Therefore, Eff-Z potentially reflects the risks associated with LVI and PNI. Moreover, the error in the Eff-Z calculation by ssDECT (1.7%) is minimal, and the Eff-Z has high accuracy in evaluating tissue components (35).
The triple-phasic enhancement is the mainstream protocol for contrast-enhanced CT in CRC. In theory, the triple-phasic IC values can reflect the comprehensive blood supply of CRC (29). However, previous studies have reported inconsistent results regarding the predictive value of contrast-enhanced CT parameters in different phases (16,20,30,36). Therefore, in this study, we conducted a comparative analysis of the triple-phasic ssDECT-derived parameters and observed significant differences between the LVI/PNI-positive and -negative groups in terms of ssDECT-derived parameters in the VP and DP. Furthermore, ssDECT-derived parameters in DP showed higher predictive performance than those in VP. On the contrary, no significant difference was observed in ssDECT-derived parameters in AP between these two groups. This can be explained by the fact that CRC is a well-known tumour with abundant fibrosis, resulting in persistent enhancement following administration of a contrast agent (19). While ssDECT-derived parameters in the AP predominantly reflect blood supply from major vessels and functional capillary density, those derived from the VP and DP more accurately depict dysfunctional neovascularization as well as contrast medium diffusion within the interstitial space. A previous study (36) also demonstrated that IC DP/NIC DP outperformed IC VP/NIC VP in predicting the occurrence of PNI for patients with locally advanced gastric cancer. This indicates clinical value and potential application prospects for using DP parameters to predict LVI/PNI.
The TME is a complex system composed of tumour cells, various immune and stromal cells, the ECM, capillaries and signaling molecules, which plays a key role in cancer aggressiveness (37). Kalluri (38) proposed that cancer-associated fibroblasts with high caveolin 1 expression could facilitate tumour invasion via ECM remodelling. Kim et al. (39) reported that the presence of PNI was closely associated with the expression of gelsolin, which promotes tumour cell proliferation and migration by degrading the ECM, subsequently contributing to the systemic recurrence of CRC. Thus, we can infer that changes in ECM in the TME are correlated with cancer aggressiveness. The ECV fraction represents the ECM volume fraction in the TME, and the ECV measured by imaging examination is consistent with the ECM measured by tissue biopsy (22); therefore, the ECV value can be used to quantitatively represent the ECM of lesions. Our results showed that the ECV in the DP of the LVI/PNI-positive group was elevated, which may be due to enhanced tumour aggressiveness accompanied by increased inflammatory cell infiltration and fibroblast activation in the TME, ultimately leading to ECM deposition (37). Furthermore, the neovascularization network of malignant tumours is abundant but immature, and the blood flow, blood volume, and vascular permeability increase significantly, resulting in more contrast agents entering the tissue space, which also leads to an increase in the ECV (40).
Although our findings indicate that the ssDECT imaging parameters in the VP and DP exhibit discriminative ability between LVI/PNI-positive and -negative tumours, the highest AUC is only 0.750 (NIC DP), which suggests that relying solely on imaging parameters may not be sufficient from a clinical perspective. Hence, we explored the predictive performance of several parameter combinations, in which the clinicopathological variables combined with ssDECT-derived parameters and ECV DP showed the highest differentiating ability (AUC =0.857). The integration of clinicopathological variables and imaging parameters yields remarkable accuracy and PPV, enhancing the preoperative recognition of patients at a heightened risk of LVI/PNI and enabling surgeons to adopt a more assertive surgical strategy when necessary. Although NCCN clinical practice guidelines recommend transanal endoscopic microsurgery for stage cT1N0 CRC patients, Stage T1 patients with LVI/PNI-positive should undergo radical mesenteric resection surgery due to their heightened risk of recurrence (41). Furthermore, predicting the LVI/PNI status in CRC through preoperative CT scans could help clinicians tailor their perioperative treatment. Patients with node-negative disease, particularly those in stage II, are arguably the most important group that may benefit from identifying LVI or PNI. These patients may not generally be considered candidates for adjuvant chemotherapy but can potentially benefit significantly from it (7,8). Studies suggest that adding chemotherapy improves disease-free survival significantly in PNI-positive patients (2,8).
Our study had limitations. First, this was a single-centre retrospective study. Future prospective studies with large sample sizes involving multiple centers are required to split the datasets and perform multiple cross-validations in order to improve the stability and generalization capacity of models. Second, although there may have been bias in manually sketching the ROI, we managed to keep the error within an acceptable range by averaging it, and the ICC values showed good consistency with our measurements. Third, the haematocrit values of the patients included in this study were collected within 3 days before the ssDECT examination. Different time windows may affect the accuracy of ECV calculations, which should be confirmed in future studies.
Conclusions
In conclusion, our study suggests that multiple ssDECT parameters and the ECV fraction may serve as imaging markers, and their combination with clinical variables can provide relatively high diagnostic accuracy for preoperative evaluation of LVI/PNI status in CRC.
Acknowledgments
Funding: This study was supported by grants of
Footnote
Reporting Checklist: The authors have completed the STARD reporting checklist. Available at https://qims.amegroups.com/article/view/10.21037/qims-24-76/rc
Conflicts of Interest: All authors have completed the ICMJE uniform disclosure form (available at https://qims.amegroups.com/article/view/10.21037/qims-24-76/coif). The authors have no conflicts of interest to declare.
Ethical Statement: The authors are accountable for all aspects of the work in ensuring that questions related to the accuracy or integrity of any part of the work are appropriately investigated and resolved. The study was conducted in accordance with the Declaration of Helsinki (as revised in 2013). The study was approved by the Ethics Committee of The Second Hospital of Lanzhou University (No. 2022A-007) and individual consent for this retrospective analysis was waived.
Open Access Statement: This is an Open Access article distributed in accordance with the Creative Commons Attribution-NonCommercial-NoDerivs 4.0 International License (CC BY-NC-ND 4.0), which permits the non-commercial replication and distribution of the article with the strict proviso that no changes or edits are made and the original work is properly cited (including links to both the formal publication through the relevant DOI and the license). See: https://creativecommons.org/licenses/by-nc-nd/4.0/.
References
- Sung H, Ferlay J, Siegel RL, Laversanne M, Soerjomataram I, Jemal A, Bray F. Global Cancer Statistics 2020: GLOBOCAN Estimates of Incidence and Mortality Worldwide for 36 Cancers in 185 Countries. CA Cancer J Clin 2021;71:209-49. [Crossref] [PubMed]
- Knijn N, Mogk SC, Teerenstra S, Simmer F, Nagtegaal ID. Perineural Invasion is a Strong Prognostic Factor in Colorectal Cancer: A Systematic Review. Am J Surg Pathol 2016;40:103-12. [Crossref] [PubMed]
- Jiang HH, Zhang ZY, Wang XY, Tang X, Liu HL, Wang AL, Li HG, Tang EJ, Lin MB. Prognostic significance of lymphovascular invasion in colorectal cancer and its association with genomic alterations. World J Gastroenterol 2019;25:2489-502. [Crossref] [PubMed]
- Achen MG, Stacker SA. Tumor lymphangiogenesis and metastatic spread-new players begin to emerge. Int J Cancer 2006;119:1755-60. [Crossref] [PubMed]
- Watanabe J, Ichimasa K, Kataoka Y, Miki A, Someko H, Honda M, Tahara M, Yamashina T, Yeoh KG, Kawai S, Kotani K, Sata N. Additional staining for lymphovascular invasion is associated with increased estimation of lymph node metastasis in patients with T1 colorectal cancer: Systematic review and meta-analysis. Dig Endosc 2024;36:533-45. [Crossref] [PubMed]
- Saidak Z, Clatot F, Chatelain D, Galmiche A. A gene expression profile associated with perineural invasion identifies a subset of HNSCC at risk of post-surgical recurrence. Oral Oncol 2018;86:53-60. [Crossref] [PubMed]
- Al-Sukhni E, Attwood K, Gabriel EM, LeVea CM, Kanehira K, Nurkin SJ. Lymphovascular and perineural invasion are associated with poor prognostic features and outcomes in colorectal cancer: A retrospective cohort study. Int J Surg 2017;37:42-9. [Crossref] [PubMed]
- Sun Q, Liu T, Liu P, Luo J, Zhang N, Lu K, Ju H, Zhu Y, Wu W, Zhang L, Fan Y, Liu Y, Li D, Zhu Y, Liu L. Perineural and lymphovascular invasion predicts for poor prognosis in locally advanced rectal cancer after neoadjuvant chemoradiotherapy and surgery. J Cancer 2019;10:2243-9. [Crossref] [PubMed]
- Rönnow CF, Arthursson V, Toth E, Krarup PM, Syk I, Thorlacius H. Lymphovascular Infiltration, Not Depth of Invasion, is the Critical Risk Factor of Metastases in Early Colorectal Cancer: Retrospective Population-based Cohort Study on Prospectively Collected Data, Including Validation. Ann Surg 2022;275:e148-54. [Crossref] [PubMed]
- Zhang L, Deng Y, Liu S, Zhang W, Hong Z, Lu Z, Pan Z, Wu X, Peng J. Lymphovascular invasion represents a superior prognostic and predictive pathological factor of the duration of adjuvant chemotherapy for stage III colon cancer patients. BMC Cancer 2023;23:3. [Crossref] [PubMed]
- Ma J, Guo D, Miao W, Wang Y, Yan L, Wu F, Zhang C, Zhang R, Zuo P, Yang G, Wang Z. The value of (18)F-FDG PET/CT-based radiomics in predicting perineural invasion and outcome in non-metastatic colorectal cancer. Abdom Radiol (NY) 2022;47:1244-54. [Crossref] [PubMed]
- Chen Q, Cui Y, Xue T, Peng H, Li M, Zhu X, Duan S, Gu H, Feng F. Computed tomography-based radiomics nomogram for the preoperative prediction of perineural invasion in colorectal cancer: a multicentre study. Abdom Radiol (NY) 2022;47:3251-63. [Crossref] [PubMed]
- Yang YS, Qiu YJ, Zheng GH, Gong HP, Ge YQ, Zhang YF, Feng F, Wang YT. High resolution MRI-based radiomic nomogram in predicting perineural invasion in rectal cancer. Cancer Imaging 2021;21:40. [Crossref] [PubMed]
- Wu CC, Lee RC, Chang CY. Prediction of lymphovascular invasion in rectal cancer by preoperative CT. AJR Am J Roentgenol 2013;201:985-92. [Crossref] [PubMed]
- Chen XL, Chen GW, Pu H, Yin LL, Li ZL, Song B, Li H. DWI and T2-Weighted MRI Volumetry in Resectable Rectal Cancer: Correlation With Lymphovascular Invasion and Lymph Node Metastases. AJR Am J Roentgenol 2019;212:1271-8. [Crossref] [PubMed]
- Gong HX, Zhang KB, Wu LM, Baigorri BF, Yin Y, Geng XC, Xu JR, Zhu J. Dual Energy Spectral CT Imaging for Colorectal Cancer Grading: A Preliminary Study. PLoS One 2016;11:e0147756. [Crossref] [PubMed]
- Yang Z, Zhang X, Fang M, Li G, Duan X, Mao J, Shen J. Preoperative Diagnosis of Regional Lymph Node Metastasis of Colorectal Cancer With Quantitative Parameters From Dual-Energy CT. AJR Am J Roentgenol 2019;213:W17-25. [Crossref] [PubMed]
- Li S, Yuan L, Yue M, Xu Y, Liu S, Wang F, Liu X, Wang F, Deng J, Sun Q, Liu X, Xue C, Lu T, Zhang W, Zhou J. Early evaluation of liver metastasis using spectral CT to predict outcome in patients with colorectal cancer treated with FOLFOXIRI and bevacizumab. Cancer Imaging 2023;23:30. [Crossref] [PubMed]
- Wu J, Lv Y, Wang N, Zhao Y, Zhang P, Liu Y, Chen A, Li J, Li X, Guo Y, Wu T, Liu A. The value of single-source dual-energy CT imaging for discriminating microsatellite instability from microsatellite stability human colorectal cancer. Eur Radiol 2019;29:3782-90. [Crossref] [PubMed]
- Ren T, Zhang W, Li S, Deng L, Xue C, Li Z, Liu S, Sun J, Zhou J. Combination of clinical and spectral-CT parameters for predicting lymphovascular and perineural invasion in gastric cancer. Diagn Interv Imaging 2022;103:584-93. [Crossref] [PubMed]
- Jiang X, Ma Q, Zhou T, Feng Q, Yang W, Zhou X, Huang W, Lin X, Li J, Zhang X, Liu S, Xin X, Fan L. Extracellular volume fraction as a potential predictor to differentiate lung cancer from benign lung lesions with dual-layer detector spectral CT. Quant Imaging Med Surg 2023;13:8121-31. [Crossref] [PubMed]
- Bluemke DA, Kawel-Boehm N. Can a MR Imaging Scanner Accurately Measure Hematocrit to Determine ECV Fraction? JACC Cardiovasc Imaging 2016;9:64-6. [Crossref] [PubMed]
- Fukukura Y, Kumagae Y, Higashi R, Hakamada H, Nakajo M, Maemura K, Arima S, Yoshiura T. Extracellular volume fraction determined by equilibrium contrast-enhanced dual-energy CT as a prognostic factor in patients with stage IV pancreatic ductal adenocarcinoma. Eur Radiol 2020;30:1679-89. [Crossref] [PubMed]
- Song X, Xie D, Tan F, Zhou Y, Li Y, Zhou Z, Pei Q, Pei H. Intravascular emboli relates to immunosuppressive tumor microenvironment and predicts prognosis in stage III colorectal cancer. Aging (Albany NY) 2021;13:20609-28. [Crossref] [PubMed]
- Chuang-Bo Y, Tai-Ping H, Hai-Feng D, Yong-Jun J, Xi-Rong Z, Guang-Ming M, Chenglong R, Jun W, Yong Y. Quantitative assessment of the degree of differentiation in colon cancer with dual-energy spectral CT. Abdom Radiol (NY) 2017;42:2591-6. [Crossref] [PubMed]
- Scurr MJ, Brown CM, Costa Bento DF, Betts GJ, Rees BI, Hills RK, Gallimore A, Godkin A. Assessing the prognostic value of preoperative carcinoembryonic antigen-specific T-cell responses in colorectal cancer. J Natl Cancer Inst 2015;107:djv001. [Crossref] [PubMed]
- Moding EJ, Clark DP, Qi Y, Li Y, Ma Y, Ghaghada K, Johnson GA, Kirsch DG, Badea CT. Dual-energy micro-computed tomography imaging of radiation-induced vascular changes in primary mouse sarcomas. Int J Radiat Oncol Biol Phys 2013;85:1353-9. [Crossref] [PubMed]
- Mulé S, Pigneur F, Quelever R, Tenenhaus A, Baranes L, Richard P, Tacher V, Herin E, Pasquier H, Ronot M, Rahmouni A, Vilgrain V, Luciani A. Can dual-energy CT replace perfusion CT for the functional evaluation of advanced hepatocellular carcinoma? Eur Radiol 2018;28:1977-85. [Crossref] [PubMed]
- Chen XH, Ren K, Liang P, Chai YR, Chen KS, Gao JB. Spectral computed tomography in advanced gastric cancer: Can iodine concentration non-invasively assess angiogenesis? World J Gastroenterol 2017;23:1666-75. [Crossref] [PubMed]
- Ma Z, Liang C, Huang Y, He L, Liang C, Chen X, Huang X, Xiong Y, Liu Z. Can lymphovascular invasion be predicted by preoperative multiphasic dynamic CT in patients with advanced gastric cancer? Eur Radiol 2017;27:3383-91. [Crossref] [PubMed]
- Kim JE, Kim HO, Bae K, Cho JM, Choi HC, Choi DS. Differentiation of small intrahepatic mass-forming cholangiocarcinoma from small liver abscess by dual source dual-energy CT quantitative parameters. Eur J Radiol 2017;92:145-52. [Crossref] [PubMed]
- Zhu Q, Sun J, Zhu W, Chen W, Ye J. Spectral CT imaging versus conventional CT post-processing technique in differentiating malignant and benign renal tumors. Br J Radiol 2023;96:20230147. [Crossref] [PubMed]
- Mileto A, Allen BC, Pietryga JA, Farjat AE, Zarzour JG, Bellini D, Ebner L, Morgan DE. Characterization of Incidental Renal Mass With Dual-Energy CT: Diagnostic Accuracy of Effective Atomic Number Maps for Discriminating Nonenhancing Cysts From Enhancing Masses. AJR Am J Roentgenol 2017;209:W221-30. [Crossref] [PubMed]
- Lagarde SM, Phillips AW, Navidi M, Disep B, Immanuel A, Griffin SM. The presence of lymphovascular and perineural infiltration after neoadjuvant therapy and oesophagectomy identifies patients at high risk for recurrence. Br J Cancer 2015;113:1427-33. [Crossref] [PubMed]
- Goo HW, Goo JM, Dual-Energy CT. New Horizon in Medical Imaging. Korean J Radiol 2017;18:555-69. [Crossref] [PubMed]
- Li J, Xu S, Wang Y, Fang M, Ma F, Xu C, Li H. Spectral CT-based nomogram for preoperative prediction of perineural invasion in locally advanced gastric cancer: a prospective study. Eur Radiol 2023;33:5172-83. [Crossref] [PubMed]
- Ao T, Mochizuki S, Kajiwara Y, Yonemura K, Shiraishi T, Nagata K, Shinto E, Okamoto K, Nearchou IP, Shimazaki H, Kishi Y, Okada Y, Ueno H. Cancer-associated fibroblasts at the unfavorable desmoplastic stroma promote colorectal cancer aggressiveness: Potential role of ADAM9. Int J Cancer 2022;150:1706-21. [Crossref] [PubMed]
- Kalluri R. The biology and function of fibroblasts in cancer. Nat Rev Cancer 2016;16:582-98. [Crossref] [PubMed]
- Kim JC, Ha YJ, Tak KH, Roh SA, Kwon YH, Kim CW, Yoon YS, Lee JL, Park Y, Kim SK, Kim SY, Cho DH, Kim YS. Opposite functions of GSN and OAS2 on colorectal cancer metastasis, mediating perineural and lymphovascular invasion, respectively. PLoS One 2018;13:e0202856. [Crossref] [PubMed]
- Jain RK. Normalization of tumor vasculature: an emerging concept in antiangiogenic therapy. Science 2005;307:58-62. [Crossref] [PubMed]
- Benson AB, Venook AP, Al-Hawary MM, Cederquist L, Chen YJ, Ciombor KK, et al. Rectal Cancer, Version 2.2018, NCCN Clinical Practice Guidelines in Oncology. J Natl Compr Canc Netw 2018;16:874-901. [Crossref] [PubMed]