Using CT features of cystic airspace to predict lung adenocarcinoma invasiveness
Introduction
The use of computed tomography (CT) screening to reduce lung cancer mortality has increased the detection of lung nodules (1,2). However, due to their atypical and rare features, the accurate diagnosis of these lung nodules remains challenging for radiologists. Recent analyses of lung cancer screening trials have shown that 22.7% (5/22) of missed or delayed lung cancer diagnoses are associated with the cystic airspace appearing as a “bulla with wall thickening or a mural nodule” (3). A cystic airspace is defined as a round, parenchymal, gas-containing lesion with a well-defined interface with normal lung tissue (4). The definition was first standardized by the Fleischner Society in 1996 and later updated in 2008 and 2017 (4-6).
Lung cancer associated with cystic airspace (LCCA) was once considered an uncommon manifestation of lung adenocarcinoma (LUAD); however, it is being observed more frequently in clinical practice (7). Data from the International Early Lung Cancer Action Program (I-ELCAP) showed that cystic airspaces presented in 3.7% (26/706) of lung cancer cases (7). The key diagnostic features of malignancy include wall thickness, mural nodules, and wall components (8), and any changes in these features during follow-up (4,9). Due to the rarity of LCCAs, their high-resolution computed tomography (HRCT) features remain underrecognized. Regular clinical follow-up examinations are required to detect wall thickening or the appearance/increase of a nodule inside or outside the cystic airspace, which may indicate lung cancer; however, such examinations place economic and psychological burdens on patients (7,9). The pathological types of LCCAs include adenocarcinoma, squamous cell carcinoma, poorly differentiated carcinoma, and small cell carcinoma. Lung adenocarcinoma is the most commonly histological type associated with cystic airspaces (4,9,10). To date, little research has been conducted on the prevalence and morphology of LUAD associated with cystic airspace (LACA) features on HRCT (8,11), especially in terms of the degree of invasiveness. Thus, this study sought to investigate the prevalence of LACA and compare the HRCT features of LACA in patients with varying degrees of invasiveness. We present this article in accordance with the STROBE reporting checklist (available at https://qims.amegroups.com/article/view/10.21037/qims-24-912/rc).
Methods
Patients
The Institutional Review Board of the Shanghai Chest Hospital, Shanghai Jiao Tong University School of Medicine, approved this retrospective, single-center study [No. KS(Y)21293]. The study was conducted in accordance with the Declaration of Helsinki (as revised in 2013), and the requirement of individual consent for this retrospective analysis was waived. In total, 1,525 lesions were retrospectively identified in 1,406 consecutive patients newly diagnosed with LUADs ≤3 cm in diameter at this tertiary care hospital between January 2016 and May 2016. The patients were aged 17–85 years (average: 56.5±10.8 years), and 474 were male and 932 were female. Among the patients, 407 underwent wedge resection, 246 underwent segmentectomy, 871 underwent lobectomy, and one underwent combined segmentectomy and lobectomy. The lesions were distributed across the lung lobes as follows: right upper lobe: 564; right middle lobe: 127; right lower lobe: 236; left upper lobe: 407; left lower lobe: 189, and right upper and lower cross-lobe: 2. There were 455 preinvasive lesions (PLs), including 32 atypical adenomatous hyperplasia (AAH) lesions, 423 adenocarcinomas in situ (AISs), 392 minimally invasive adenocarcinomas (MIAs), and 678 invasive adenocarcinomas (IACs). Additionally, 52 patients had lymph node metastases and 88 had visceral pleural metastases.
Patients were excluded from the study if they met any of the following exclusion criteria: (I) displayed airspace in the center of a previously solid lesion suggesting cavitation; (II) displayed airspace indistinguishable from surrounding emphysema, bronchiectasis, or cystic interstitial lung disease; (III) had an incomplete CT target scan; (IV) the resected lesion did not correspond to the CT target scan; (V) the lesion had been proven to be LUAD by histopathology, but had not been confirmed to be the primary lesion.
CT scanning
An unenhanced routine CT with continuous thin sections over the entire lung volume was performed with a multi-slice (16-, 64-, 128-, and 256-slice) spiral CT scanner (Brilliance, Ingenuity, iCT, Philips, USA; Discovery CT750, GE, USA; uCT510, UIH, China) using a helical technique at the end of inspiration during a single breath-hold. Scanning parameters included 64 mm × 0.625 mm detector collimation, 1.08 pitch, 5.0 mm section thickness and interval, 5–7 s scan time, 512×512 matrix, 400 mm field of view (FOV), 120 kVp, and 250 mA. When a lung nodule was detected, a HRCT target scan was conducted with the following parameters: 64 mm × 0.625 mm collimation, 0.64 pitch, 1.0 mm section thickness and interval, 1–3 s scan time, 1024×1024 (Brilliance/Ingenuity/iCT) matrix or 512×512 matrix (Discovery CT750/uCT510), 180 mm FOV, 120 kVp, and 300 mA. The reconstruction algorithms for the routine CT and targeted HRCT scans were “standard” and “sharp”, respectively.
Evaluation of CT features
Cystic airspace was defined as a circumscribed parenchymal air-containing region with a well-defined wall at the interface inside or outside the lesion (12). The presence of the cystic airspace for each nodule was evaluated two times (eight months later for the second time) by one radiologist (Y.Z., who had 12 years of experience in chest imaging). Pulmonary parenchyma was evaluated for the presence or absence of emphysema in the lobe containing LUAD, particularly surrounding the lesion. The cystic airspace outside the lesion was not recorded when emphysema occurred in the background of the lung parenchyma. The HRCT findings for each nodule were analyzed in terms of: (I) density [pure ground-glass nodule (PGGN) or mixed ground-glass nodule (MGGN), or solid nodule]; (II) location; (III) surgery type (wedge resection, segmentectomy, or lobectomy); (IV) multiplicity (the presence of two or more ground-glass nodules (GGNs) in an individual); and (V) diameter. When cystic airspaces were confirmed, two radiologists (Y.Z. and L.Z., who had 12 and 22 years of experience in chest imaging, respectively), who were blinded to the invasiveness of the nodules, assessed the HRCT images of the LACAs in a lung window (width: 1,450 HUs; level: –520 HUs). The decisions as to the CT findings were reached by consensus.
In relation to the characteristics of the cystic airspace, the HRCT qualitative findings were analyzed in terms of the: (I) pattern (type I: the cystic airspace was entirely within the nodule; type II: more than half of the cystic airspace was within the nodule; or type III: more than half of the cystic airspace was outside the nodule); (II) number (one, two, or multiple locules); (III) wall component density (PGGN, MGGN, or solid nodule); (IV) distribution (eccentric or centric); (V) inner surface (smooth or irregular); (VI) mural nodules (yes/no); (VII) septa (yes/no); and (VIII) vessels passing through the cystic airspace. When multiple patterns of cystic airspaces were present in the nodules, only the largest cystic airspace was analyzed.
The quantitative measurements of the cystic airspace included the following: (I) cystic airspace diameter (the largest cystic airspace diameter on the axial section); (II) wall thickness (the largest diameter on the axial section, running perpendicular to the tangent of the cystic airspace, from the border of the cystic airspace to that of the ground-glass opacity/solid component); and (III) thin-wall proportion [circumferential thickening <4 mm of the cystic airspace as calculated in degrees; 0° represented thickening of the entire wall of the airspace and 360° represented no focal thickening (6)]. If a patient underwent several preoperative CT examinations at our department that revealed the same LACA, the radiological features of the latest CT images of this patient were analyzed.
Pathological evaluation
The surgically removed specimens were fixed in 10% formalin, embedded in paraffin, sectioned with a microtome, and stained with hematoxylin and eosin. According to the 2015 international multidisciplinary classification of LUADs, the nodules underwent histopathological analysis and were classified by two experienced pulmonary pathologists.
Statistical analysis
The HRCT findings of positive and negative cystic lung nodules were compared using the Pearson χ2 test or Fisher’s exact test as appropriate for the categorical variables, and a one-way analysis of variance or the Mann-Whitney U test for the continuous variables. A P value <0.05 was considered statistically significant. Multiple comparisons of the three groups (i.e., the PL, MIA, and IAC groups) were performed using the Pearson χ2 test or Fisher’s exact test as appropriate for the categorical variables, and a P value <0.017 indicated a statistically significant difference. Multiple comparisons of the three groups were performed using the Bonferroni test (P<0.05) or Kruskal-Wallis rank-sum test (P<0.017) as appropriate for the continuous variables. All the tests were two-sided and analyzed by SPSS (version 22.0; IBM Corp, Armonk, NY, USA).
Results
Of the LUAD nodules (Figure 1), 176 of 1,525 (11.5%) showed cystic airspace on HRCT as follows: PL: 4.6% (21/455); AAH: 3.1% (1/32); AIS: 4.7% (20/423); MIA: 8.4% (33/392); and IAC: 18.0% (122/678). Table 1 sets out the demographics and HRCT features of the LACAs; LACAs were more common than LUADs without cystic airspaces in older male patients (P=0.036 and P<0.001, respectively). Cystic airspace was observed in 7.1% (36/505) of the PGGNs, 13.5% (112/830) of the MGGNs, and 14.7% (28/190) of the solid nodules (P=0.001). The prevalence of cystic airspaces was significantly higher in the MGGNs than the PGGNs (P<0.001), and in the solid nodules than the PGGNs (P=0.002). The surgical procedures for the LACAs differed significantly (P=0.012), such that the patients were more likely to undergo lobectomy than wedge resection (P=0.006). The incidence of LACAs increased as lesion diameter increased (13.1 vs. 19.8 mm) (P<0.001). The prevalence of LACAs increased as LUAD invasiveness increased (P<0.001) and was higher in IACs than PLs and MIAs (P<0.001, and P<0.001, respectively), and slightly higher in MIAs than PLs but the difference was not statistically significant (P=0.024, >0.017). However, the occurrence of LACAs was not found to be related to lesion distribution, multiplicity, lymph node metastasis, visceral pleural metastasis, or biological indicators.

Table 1
Variables | Cystic airspace | P value | |
---|---|---|---|
Negative (n=1,349) | Positive (n=176) | ||
Gender | <0.001 | ||
Male | 410 (81.3) | 94 (18.7) | |
Female | 939 (92.0) | 82 (8.0) | |
Age (years) | 56.2±10.8 | 58.2±10.3 | 0.036† |
Density | 0.001 | ||
PGGN | 469 (92.9) | 36 (7.1) | |
MGGN | 718 (86.5) | 112 (13.5) | |
Solid | 162 (85.3) | 28 (14.7) | |
Location | 0.892‡ | ||
Right upper lobe | 503 (89.2) | 61 (10.8) | |
Right middle lobe | 113 (89.0) | 14 (11.0) | |
Right lower lobe | 209 (88.6) | 27 (11.4) | |
Left upper lobe | 359 (88.2) | 48 (11.8) | |
Left lower lobe | 163 (86.2) | 26 (13.8) | |
Right upper and lower lobe | 2 (100.0) | 0 | |
Surgery | 0.012‡ | ||
Wedge resection | 373 (91.6) | 34 (8.4) | |
Segmentectomy | 224 (91.1) | 22 (8.9) | |
Lobectomy | 751 (86.2) | 120 (13.8) | |
Segmentectomy and lobectomy | 1 (100.0) | 0 | |
Multiply | 589 (87.6) | 83 (12.4) | 0.379 |
Lymph node metastasis§ | 0.108 | ||
Negative | 1068 (88.2) | 143 (11.8) | |
Positive | 42 (80.8) | 10 (19.2) | |
Visceral pleural metastasis§ | 0.072 | ||
Negative | 401 (88.7) | 51 (11.3) | |
Positive | 72 (81.8) | 16 (18.2) | |
Biological indicators§ | 0.351 | ||
Negative | 818 (89.1) | 100 (10.9) | |
Positive | 93 (86.1) | 15 (13.9) | |
Diameter (mm) | 13.1±6.3 | 19.8±6.2 | <0.001† |
Pathology | <0.001 | ||
PL | 434 (95.4) | 21 (4.6) | |
MIA | 359 (91.6) | 33 (8.4) | |
IAC | 556 (82.0) | 122 (18.0) |
Data are presented as n (%) and mean ± standard deviation. †, Mann-Whitney rank-sum test; ‡, Fisher’s exact test; §, there were missing cases. LACA, lung adenocarcinomas associated with cystic airspace; PGGN, pure ground-glass nodule; MGGN, mixed ground-glass nodule; PL, preinvasive lesions; MIA, minimally invasive adenocarcinomas; IAC, invasive adenocarcinomas.
Table 2 sets out the HRCT characteristics of the cystic airspaces in the PL, MIA, and IAC groups. Statistically significant differences were observed in terms of the wall component density, distribution, septa, vessels passing through the cystic airspace, cystic airspace diameter, wall thickness, and thin-wall proportion (P<0.001, P=0.024, P=0.001, P=0.025, P=0.001, P<0.001, and P<0.001, respectively); however, no statistically significant differences were observed in terms of the pattern, number, inner surface, and mural nodules (P=0.758, P=0.316, P>0.99, and P=0.382, respectively). Table 3 sets out the results of multiple comparisons among the three groups for the above statistically significant parameters. Wall component density increased as invasiveness increased (P<0.001). On HRCT, wall component density was more likely to appear as MGGNs in MIA than PL, for which it mainly appeared as PGGNs (P=0.01) (Figure 2), while wall component density was more likely to appear as MGGNs or solid nodules in IAC than PL and MIA (P<0.001 and P<0.001, respectively) (Figure 3). The cystic airspaces in PL typically lacked septa, unlike those in MIA and IAC (P=0.001 and P<0.001, respectively) (Figure 4). IAC had a larger cystic airspace diameter than PL (6.5 vs. 3.7 mm) (P<0.001) (Figures 5,6). The wall thickness of the cystic airspace was thicker in IAC than PL and MIA (11.8 vs. 6.8 mm, 11.8 vs. 8.3 mm) (P<0.001 and P<0.001, respectively) (Figures 5,6). The thin-wall proportion was smaller in IAC than PL and MIA (181.5° vs. 264.8°, 181.5° vs. 223.8°) (P<0.001, and P=0.039, respectively) (Figure 6).
Table 2
Variables | PL | MIA | IAC | P value |
---|---|---|---|---|
Pattern | 0.758† | |||
Type I | 13 (11.4) | 21 (18.4) | 80 (70.2) | |
Type II | 6 (13.3) | 7 (15.6) | 32 (71.1) | |
Type III | 2 (11.8) | 5 (29.4) | 10 (58.8) | |
Number | 0.316† | |||
One | 18 (14.9) | 24 (19.8) | 79 (65.3) | |
Two | 2 (8.3) | 5 (20.8) | 17 (70.8) | |
Multiple | 1(3.2) | 4 (12.9) | 26 (83.9) | |
Wall component density | <0.001† | |||
PGGN | 17 (35.4) | 15 (31.3) | 16 (33.3) | |
MGGN | 4 (4.0) | 18 (18.0) | 78 (78.0) | |
Solid | 0 (0.0) | 0 (0.0) | 28 (100.0) | |
Distribution | 0.024 | |||
Eccentric | 13 (9.7) | 21 (15.7) | 100 (74.6) | |
Centric | 8 (19.0) | 12 (28.6) | 22 (52.4) | |
Inner surface | >0.99† | |||
Smooth | 18 (11.8) | 29 (19.1) | 105 (69.1) | |
Irregular | 3 (12.5) | 4 (16.7) | 17 (70.8) | |
Mural nodules | 0 (0.0) | 0 (0.0) | 7 (100.0) | 0.382† |
Septa | 1 (1.4) | 15 (20.3) | 58 (78.4) | 0.001 |
Vessels passing through the cystic airspace | 4 (6.2) | 8 (12.3) | 53 (81.5) | 0.025 |
Cystic airspace diameter (mm) | 3.7±1.8 | 5.6±3.9 | 6.5±4.3 | 0.001‡ |
Wall thickness (mm) | 6.8±2.7 | 8.3±4.0 | 11.8±4.3 | <0.001 |
Thin-wall proportion (°) | 264.8±76.2 | 223.8±88.1 | 181.5±86.7 | <0.001 |
Data are presented as n (%) and mean ± standard deviation. †, Fisher’ exact test; ‡, Kruskal-Wallis rank-sum test. HRCT, high-resolution computed tomography; PL, preinvasive lesions; MIA, minimally invasive adenocarcinomas; IAC, invasive adenocarcinomas; PGGN, pure ground-glass nodule; MGGN, mixed ground-glass nodule.
Table 3 P
Variables | PL vs. MIA | PL vs. IAC | MIA vs. IAC |
---|---|---|---|
Wall component density | 0.01 | <0.001† | <0.001 |
Distribution | 0.898 | 0.046† | 0.024 |
Septa | 0.001 | <0.001 | 0.831 |
Vessels passing through the cystic airspace | 0.747† | 0.035 | 0.045 |
Cystic airspace diameter | 0.049 | <0.001 | 0.151 |
Wall thickness | 0.567‡ | <0.001‡ | <0.001‡ |
Thin-wall proportion | 0.268‡ | <0.001‡ | 0.039‡ |
A P value <0.017 was considered to be statistically significant difference. †, Fisher’ exact test; ‡, a P value <0.05 was considered to be statistically significant according to the Bonferroni test. PL, preinvasive lesions; MIA, minimally invasive adenocarcinomas; IAC, invasive adenocarcinomas.
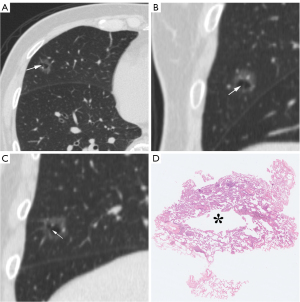
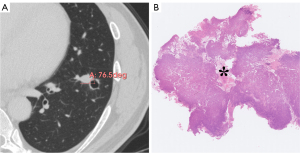
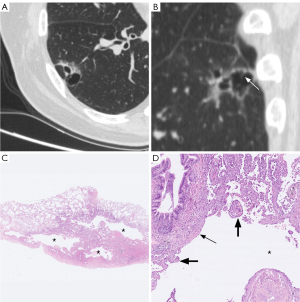
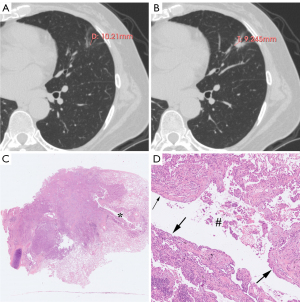
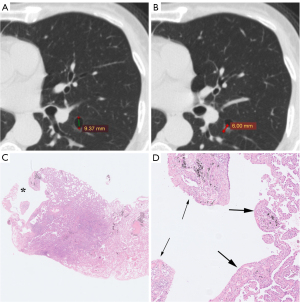
Discussion
In this large cohort study, the prevalence of cystic airspace was 11.5% (176/1,525) on HRCT target scans in patients with LUADs ≤3 cm in diameter. Further, the wall component density, septa, cystic airspace diameter, wall thickness, and thin-wall proportion were found to be significant predictors of LUAD invasiveness. LCCAs were observed in 3.7% of cases in an I-ELCAP study, in 1% (30/2,954) of non-small cell lung cancer cases in a study by Fintelmann et al., and in 1.1% (117/10,835) of lung cancer cases in recent years (4,7,8). We found a higher prevalence of LACAs than previous studies, but our findings are consistent with other reports (11). The higher prevalence reported in the present study might be due to the LCCA pathology, wall thickness of the cystic airspace, and the diameters of the LUADs in the enrolled patients. In the above-mentioned studies, the most common pathological subtype was LUAD, which suggests that cystic airspace is more common in LUAD than other pathological subtypes (13). In the present study, only patients with LUADs were included, which resulted in a higher prevalence. Additionally, many previous studies only included patients with thin-walled cystic airspaces (13,14). However, in the present study, patients were included regardless of the thickness of the cystic airspace wall, which led to a higher prevalence of LACAs.
Previous studies of LCCAs have reported a stepwise process in which the cystic airspace first displays wall thickening and then transforms into a mural nodule, or in which the cystic airspace is replaced by a solid component. This indicates that cystic airspace is a part of lung cancer, and the incidence of cystic airspace will decrease as the lesion develops (4,8,9). Alternately, the higher prevalence of LACAs observed in the present study might be related to the enrolment criteria; all the patients had LUADs ≤3 cm in diameter, which is not too large, resulting in the cystic airspace being replaced by a solid mass. Pathologically, it may be that tumor cells develop in the alveolar wall and grow toward the bronchiole or tumor cells directly developed in the bronchiole first, and then form a check-valve due to the lack of cartilage in the bronchiole, and the accumulated gases enter the alveoli, which then ruptures and fuses into solitary cystic airspace. This cystic airspace may gradually increase as the inner pressure continually increases. Additionally, the tumor components grow irregularly and induce the asymmetric thickening of the wall (14). Finally, the cystic airspace may be totally replaced by a solid component, in which case, the prevalence of cystic airspaces would decrease.
The characteristics of cystic airspaces differ among clinical cases. Maki et al. used a three-type classification system to describe the CT morphologic features of LCCAs as follows: type I: a nodule or mass extruding from the wall of the cystic airspace; type II: a nodule or mass confined in the cystic airspace; and type III: soft-tissue density extending along the wall of the cystic airspace (15). Mascalchi et al. added another type to describe the morphological CT features of LCCAs; that is, type IV: soft-tissue density intermixed in clusters of cystic airspaces (9). The various subtypes can transform into each other, or can be replaced by solid lesions during follow-up. Subsequent studies considered additional factors in an effort to improve diagnosis. For example, a thin-walled cystic airspace indicates a lesion with a maximum wall thickness of 4 mm (14). The density of a nodule, mural nodule, and wall thickening on HRCT were also considered as diagnostic factors. Fintelmann et al. divided cystic airspaces into unilocular or multilocular (4). A mural nodule in the cystic airspace (type III), a part-solid/solid component in the wall, and an irregular inner surface in a cyst were shown to be independent risk factors for the moderately/poorly (M/P)-differentiated subtype (8,16). Type III LCCAs were shown to have the worst survival rate (8,16). However, these studies partially sought to categorize LCCA based on its appearance. Conversely, the present study explored the CT morphology of LACAs based on their qualitative features and included quantitative variables. We found that the wall component density, cystic airspace diameter, septa, wall thickness, and thin-wall proportion could be used to predict the invasiveness of LUAD.
The wall component density in the PLs mainly appeared as PGGNs, but increasingly appeared as MGGNs in the MIAs; no patient manifested a solid density of wall components in the PLs or MIAs. As invasiveness increased, the wall component density in the IACs appeared as either MGGNs or solid nodules on HRCT. The stepwise radiologic progression of LUAD, including the transformation of ground-glass opacity from non-solid nodules to part-solid nodules and then entire-solid nodules, is well known (17-20). Wall component density demonstrated a similar stepwise disease progression in the LACAs. Unlike those in the MIAs and IACs, cystic airspaces in PLs typically lacked septa. A microscopic observation by Tan et al. showed that septa in the cystic airspace on CT were composed of many types of tissue, including fibrous tissue produced by tumor cells, bronchus, or blood vessels (13). Jung et al. proposed a stepwise progression model of subsolid LACAs as follows: phase I, cystic airspaces appear in the middle of non-solid nodules; phase II, the cystic airspaces enlarge; phase III, a solid component emerges on the border of the cystic airspaces; and phase IV, the solid component gradually encompasses the cystic airspaces and becomes thicker, and ultimately, the cystic airspaces shrink or disappear (13,21). Thus, as cystic airspaces develop, the cystic airspace diameter can be used to diagnose LUAD invasiveness in some phases. We found that the cystic airspace diameter was larger in the IACs than PLs (6.5 vs. 3.7 mm).
We also found that the wall thickness of the cystic airspace was thicker in the IACs than the PLs and MIAs (11.8 vs. 6.8 mm; 11.8 vs. 8.3 mm). A previous study found that the thickness of the wall surrounding the cystic airspaces was associated with prognosis (22). Subsequent research found that only solid wall thickness was closely related to the recurrence for subsolid nodules (21). In relation to lung nodules, PL and MIA have a homologous prognosis (23). Similarly, we found insignificant differences in the wall thickness between the PLs and MIAs. As the wall thickness of the cystic airspace is frequently heterogeneous (13,21), we measured the thickest and the proportion of the thin-wall to further assess cystic airspace performance. The thin-wall proportion is much smaller in the IACs than the PLs and MIAs. This is consistent with a previous study that showed that patients with thin-walled LACAs had significantly longer overall survival than those with thick-walled LACAs in stage I (22).
This study found that observations of cystic airspaces are not uncommon in LUADs, and may be transiently observed as cancers develop. Further knowledge of the spectrum of cystic LUAD morphology is key to improving diagnostic accuracy and LUAD management (24). Our study also showed that specific guidelines need to be developed for the follow-up and staging of LACAs. Standardized protocols incorporating both traditional imaging techniques and advanced artificial intelligence (AI) analyses could enhance early detection and treatment planning. Regular follow-up with continuous HRCT scans, combined with AI analyses, could improve the accuracy of detecting changes in cystic airspaces, thereby aiding in the timely and precise staging of the disease.
This study had a number of limitations. First, no analysis was conducted using AI tools, which are increasingly being employed to enhance the predictive accuracy of CT features. Future studies should incorporate AI methodologies to provide more robust and precise assessments of LUAD characteristics. Second, this study was limited by its retrospective nature; thus, there might be some bias in the estimate of the overall prevalence of the LACAs. The nodules examined in this study were diagnosed by surgery and pathology; however, those diagnosed by biopsy or other methods were excluded, as were those that required follow-up. Additionally, the correlations between the HRCT findings for cystic airspace and pathology, such as the relationship between the wall pathological composition and HRCT features, were not examined individually. However, we examined the wall pathological composition of the tumor cells and fibrous tissues in several cases. We did not assess the LACA growth pattern and prognosis. Finally, this was a transversal study; thus, more continuous HRCT should be performed preoperatively and postoperatively.
Conclusions
The prevalence and features of cystic airspaces on HRCT can be used to predict the invasiveness of LUADs ≤3 cm in diameter.
Acknowledgments
Funding: This study was supported by grants from
Footnote
Reporting Checklist: The authors have completed the STROBE reporting checklist. Available at https://qims.amegroups.com/article/view/10.21037/qims-24-912/rc
Conflicts of Interest: All authors have completed the ICMJE uniform disclosure form (available at https://qims.amegroups.com/article/view/10.21037/qims-24-912/coif). The authors have no conflicts of interest to declare.
Ethical Statement: The authors are accountable for all aspects of the work in ensuring that questions related to the accuracy or integrity of any part of the work are appropriately investigated and resolved. The Institutional Review Board of the Shanghai Chest Hospital, Shanghai Jiao Tong University School of Medicine, approved this retrospective, single-center study [No. KS(Y)21293]. The study was conducted in accordance with the Declaration of Helsinki (as revised in 2013), and the requirement of individual consent for this retrospective analysis was waived.
Open Access Statement: This is an Open Access article distributed in accordance with the Creative Commons Attribution-NonCommercial-NoDerivs 4.0 International License (CC BY-NC-ND 4.0), which permits the non-commercial replication and distribution of the article with the strict proviso that no changes or edits are made and the original work is properly cited (including links to both the formal publication through the relevant DOI and the license). See: https://creativecommons.org/licenses/by-nc-nd/4.0/.
References
- Henschke CI, Boffetta P, Gorlova O, Yip R, Delancey JO, Foy M. Assessment of lung-cancer mortality reduction from CT Screening. Lung Cancer 2011;71:328-32. [Crossref] [PubMed]
- de Koning HJ, van der Aalst CM, de Jong PA, Scholten ET, Nackaerts K, Heuvelmans MA, et al. Reduced Lung-Cancer Mortality with Volume CT Screening in a Randomized Trial. N Engl J Med 2020;382:503-13. [Crossref] [PubMed]
- Scholten ET, Horeweg N, de Koning HJ, Vliegenthart R, Oudkerk M, Mali WP, de Jong PA. Computed tomographic characteristics of interval and post screen carcinomas in lung cancer screening. Eur Radiol 2015;25:81-8. [Crossref] [PubMed]
- Fintelmann FJ, Brinkmann JK, Jeck WR, Troschel FM, Digumarthy SR, Mino-Kenudson M, Shepard JO. Lung Cancers Associated With Cystic Airspaces: Natural History, Pathologic Correlation, and Mutational Analysis. J Thorac Imaging 2017;32:176-88. [Crossref] [PubMed]
- Austin JH, Müller NL, Friedman PJ, Hansell DM, Naidich DP, Remy-Jardin M, Webb WR, Zerhouni EA. Glossary of terms for CT of the lungs: recommendations of the Nomenclature Committee of the Fleischner Society. Radiology 1996;200:327-31. [Crossref] [PubMed]
- Hansell DM, Bankier AA, MacMahon H, McLoud TC, Müller NL, Remy J. Fleischner Society: glossary of terms for thoracic imaging. Radiology 2008;246:697-722. [Crossref] [PubMed]
- Farooqi AO, Cham M, Zhang L, Beasley MB, Austin JH, Miller A, Zulueta JJ, Roberts H, Enser C, Kao SJ, Thorsen MK, Smith JP, Libby DM, Yip R, Yankelevitz DF, Henschke CI. Lung cancer associated with cystic airspaces. AJR Am J Roentgenol 2012;199:781-6. [Crossref] [PubMed]
- Shen Y, Xu X, Zhang Y, Li W, Dai J, Jiang S, Wu T, Cai H, Sihoe A, Shi J, Jiang G. Lung cancers associated with cystic airspaces: CT features and pathologic correlation. Lung Cancer 2019;135:110-5. [Crossref] [PubMed]
- Mascalchi M, Attinà D, Bertelli E, Falchini M, Vella A, Pegna AL, Ambrosini V, Zompatori M. Lung cancer associated with cystic airspaces. J Comput Assist Tomogr 2015;39:102-8. [Crossref] [PubMed]
- Ma Z, Wang S, Zhu H, Li Y, Zhang Y. Comprehensive investigation of lung cancer associated with cystic airspaces: predictive value of morphology. Eur J Cardiothorac Surg 2022;62:ezac297. [Crossref] [PubMed]
- Zhu H, Zhang L, Huang Z, Chen J, Sun L, Chen Y, Huang G, Chen Q, Yu H. Lung adenocarcinoma associated with cystic airspaces: Predictive value of CT features in assessing pathologic invasiveness. Eur J Radiol 2023;165:110947. [Crossref] [PubMed]
- Bankier AA, MacMahon H, Colby T, Gevenois PA, Goo JM, Leung ANC, Lynch DA, Schaefer-Prokop CM, Tomiyama N, Travis WD, Verschakelen JA, White CS, Naidich DP. Fleischner Society: Glossary of Terms for Thoracic Imaging. Radiology 2024;310:e232558. [Crossref] [PubMed]
- Tan Y, Gao J, Wu C, Zhao S, Yu J, Zhu R, Zhang Q, Wu G, Xue X, Wu J. CT Characteristics and Pathologic Basis of Solitary Cystic Lung Cancer. Radiology 2019;291:495-501. [Crossref] [PubMed]
- Xue X, Wang P, Xue Q, Wang N, Zhang L, Sun J, Wang K, Yang B, Wang J. Comparative study of solitary thin-walled cavity lung cancer with computed tomography and pathological findings. Lung Cancer 2012;78:45-50. [Crossref] [PubMed]
- Maki D, Takahashi M, Murata K, Sawai S, Fujino S, Inoue S. Computed tomography appearances of bronchogenic carcinoma associated with bullous lung disease. J Comput Assist Tomogr 2006;30:447-52. [Crossref] [PubMed]
- Shen Y, Zhang Y, Guo Y, Li W, Huang Y, Wu T, Jiang G, Dai J. Prognosis of lung cancer associated with cystic airspaces: A propensity score matching analysis. Lung Cancer 2021;159:111-6. [Crossref] [PubMed]
- Zhang Y, Qiang JW, Ye JD, Ye XD, Zhang J. High resolution CT in differentiating minimally invasive component in early lung adenocarcinoma. Lung Cancer 2014;84:236-41. [Crossref] [PubMed]
- Zhang Y, Shen Y, Qiang JW, Ye JD, Zhang J, Zhao RY. HRCT features distinguishing pre-invasive from invasive pulmonary adenocarcinomas appearing as ground-glass nodules. Eur Radiol 2016;26:2921-8. [Crossref] [PubMed]
- Min JH, Lee HY, Lee KS, Han J, Park K, Ahn MJ, Lee SJ. Stepwise evolution from a focal pure pulmonary ground-glass opacity nodule into an invasive lung adenocarcinoma: an observation for more than 10 years. Lung Cancer 2010;69:123-6. [Crossref] [PubMed]
- Kato T, Iwano S, Hanamatsu Y, Nakaguro M, Emoto R, Okado S, Sato K, Noritake O, Nakanishi K, Kadomatsu Y, Ueno H, Ozeki N, Nakamura S, Fukumoto K, Takeuchi T, Karube K, Matsui S, Chen-Yoshikawa TF. Prognostic impact of highly solid component in early-stage solid lung adenocarcinoma. Quant Imaging Med Surg 2023;13:5641-52. [Crossref] [PubMed]
- Jung W, Cho S, Yum S, Chung JH, Lee KW, Kim K, Lee CT, Jheon S. Stepwise Disease Progression Model of Subsolid Lung Adenocarcinoma with Cystic Airspaces. Ann Surg Oncol 2020;27:4394-403. [Crossref] [PubMed]
- Watanabe Y, Kusumoto M, Yoshida A, Shiraishi K, Suzuki K, Watanabe SI, Tsuta K. Cavity Wall Thickness in Solitary Cavitary Lung Adenocarcinomas Is a Prognostic Indicator. Ann Thorac Surg 2016;102:1863-71. [Crossref] [PubMed]
- Russell PA, Wainer Z, Wright GM, Daniels M, Conron M, Williams RA. Does lung adenocarcinoma subtype predict patient survival? A clinicopathologic study based on the new International Association for the Study of Lung Cancer/American Thoracic Society/European Respiratory Society international multidisciplinary lung adenocarcinoma classification. J Thorac Oncol 2011;6:1496-504. [Crossref] [PubMed]
- Byrne D, English JC, Atkar-Khattra S, Lam S, Yee J, Myers R, Bilawich AM, Mayo JR, Mets OM. Cystic Primary Lung Cancer: Evolution of Computed Tomography Imaging Morphology Over Time. J Thorac Imaging 2021;36:373-81. [Crossref] [PubMed]