Potential of quantitative T1 mapping to serve as a novel prognostic predictor of clear cell renal cell carcinoma after nephrectomy
Introduction
Clear cell renal cell carcinoma (ccRCC) is the most common subtype of renal cell carcinomas (RCCs), which accounts for about 75% of all RCCs (1,2). At present, surgical resection followed by observation has been a clinical standard treatment for patients with non-metastatic ccRCCs (3). However, up to 60% of high-risk patients with localized ccRCCs will experience recurrence or metastatic disease within 5 years (3,4). Therefore, there is a clinical need for accurate risk stratification of patients with ccRCCs before surgery to enable personalized treatment. The traditional prognostic indicators are tumor grade (5), sarcomatoid transformations, tumor necrosis (6), microvascular infiltration, and the presence of lymph node metastases. Diagnosing these factors needs a biopsy or surgical resection, but they are invasive, time- consuming, and costly. Thus, a non-invasive preoperative imaging method has more potential to guide the clinical decision-making.
Currently, noninvasive imaging methods, including computed tomography (CT) and magnetic resonance imaging (MRI), are employed for early detection of ccRCCs. MRI has an excellent soft-tissue contrast and, unlike CT, does not involve radiation exposure (7). MRI has been routinely used for noninvasive diagnosis (8,9), treatment planning, and treatment response assessment of ccRCCs (10,11). However, conventional MRI can only analyze the characteristics of tumors qualitatively, and the overlapping characteristics of lesions on imaging is another key problem.
The longitudinal (spin-lattice) relaxation time (T1) is a quantifiable property of the tissue in a magnetic field that is related to water content and mobility. T1 mapping is a technique that can provide an absolute value of T1 for quantitative analyses of biological tissue characteristics. In addition, T1 mapping is linearly correlated to the concentration of gadolinium diethylenetriaminepentaacetic acid (GD-DTPA) in both blood and tissue. It could accurately demonstrate the blood supply of ccRCCs. Evidence has shown that T1 mapping may reflect the extracellular expansion and indicate the pathophysiological processes of the lesions (12,13) such as edema, inflammation, and fibrosis (14). Previous studies have reported the usefulness of T1 mapping in grading the ccRCCs (15), distinguishing ccRCCs from fat-poor angiomyolipomas (16), and detecting the severity of renal function and fibrosis (17-19). However, the prognostic value of T1 mapping in patients with ccRCCs remains unclear.
In this study, we aimed to determine whether the quantitative T1 mapping may be used as an imaging biomarker to predict the prognosis of ccRCCs preoperatively. We present this article in accordance with the STROBE reporting checklist (available at https://qims.amegroups.com/article/view/10.21037/qims-23-1829/rc).
Methods
Patients
The study was conducted in accordance with the Declaration of Helsinki (as revised in 2013). This retrospective cohort study obtained approval from the Institutional Review Board of Zhongshan Hospital (No. B2020-214R). Since this is a retrospective review study, the requirement for written informed consent was waived. The data in renal neoplasms of inpatients were collected consecutively and retrospectively from the period between September 2014 and September 2021. All renal incidentalomas discovered by abdominal ultrasonography or CT examinations were confirmed by MRI examinations for further clinical diagnosis. Surgical procedures include laparoscopic or open, radical, or partial nephrectomy. The inclusion criteria were as follows: (I) pathologically confirmed ccRCCs with nephrectomy; (II) MRI performed within 1 month before resection, and the MRI scanning protocol was complete (including T1 mapping before and after contrast enhancement). The exclusion criteria were as follows: (I) patients receiving preoperative neoadjuvant therapy; (II) poor imaging quality with serious artifacts affecting the observation; (III) complete cystic ccRCCs without measurable solid components. The flowchart of this study population is presented in Figure 1.
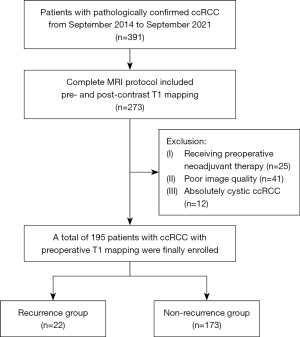
Renal MRI
All patients with renal lesions were scanned with the same 1.5T MRI system (Magnetom Area; Siemens Healthineers, Erlangen, Germany) using an 18-channel body array coil. A routine multiparametric renal magnetic resonance (MR) protocol was scanned in the transverse position, including diffusion-weighted imaging (DWI) (b values, 0, 50, and 800 s/mm2), 3-dimensional (3D) in- and out-of-phase T1-weighted interpolated examination imaging (T1WI), T2-weighted fast spin-echo pulse sequence with fat suppression imaging (T2WI), and pre-and post-contrasted dynamic 3D T1-weighted volumetric interpolated examination at corticomedullary phase (CMP, 20–25 s), nephrographic phase (NP, 80–90 s), and excretory phase (EP, 180 s) after injection of 0.1 mmol/kg administration GD-DTPA at a rate of 2 mL/s. The native and enhanced T1 mapping sequences were performed before and at 5 minutes after the administration of GD-DTPA, with a breath-holding interval of 15 seconds, respectively. The parameters were as follows: echo time (TE), 4.38 ms; repetition time (TR), 1.93 ms; slice thickness, 3mm; matrix size 174×288, field of view (FOV) 300 mm × 380 mm; flip angle, 2° and 12°.
Quantitative MR image analysis
Observers (L.Z. with 5 years of experience and Y.D. with 12 years of experience in abdominal MR imaging diagnosis) who were blinded to the pathological diagnosis of tumors independently delineated the regions of interest (ROI) of the lesions. A circular ROI was manually placed in the most avidly enhanced area of the lesions 3 times and an average value was taken for further analysis. All the ROIs were first delineated on the CMP image and then copied to the corresponding unenhanced phase (UP), NP, apparent diffusion coefficient (ADC), and T1 maps. When the tumor was entirely homogeneous, ROIs were outlined around it as large as possible. To minimize the selection bias, we avoided sites adjacent to the renal parenchyma and perinephric fat, as well as heterogeneous compositions (including necrosis, cystic, hemorrhagic, and calcified areas). The reduction rate of T1 relaxation time (T1r) and enhancement rate of corticomedullary phase (CMPr) and nephrographic phase (NPr) were calculated as follows: T1r = (native T1 value − enhanced T1 value)/native T1 value × 100%; CMPr or NPr = (SICMP or SINP − SIUP)/SIUP × 100%, where SIUP, SICMP, and SINP were the signal intensity of the lesions on UP, CMP, and NP, respectively.
Follow-up
All patients were followed-up until the end of December 2022. Progression-free survival (PFS) was measured from the date of surgery until the date of confirmed tumor recurrence and metastasis. PFS was censored at the date of death due to other causes or last follow-up at which the patient was alive and recurrence-free (20).
Statistical analysis
Univariate and multivariable Cox proportional hazard regression analyses were conducted to assess risk factors related to the development of recurrence and metastasis. Factors with a P value of 0.05 or less in the univariate regression analysis were all entered into a multivariate model. The forward stepwise method (Forward: LR) variable selection method was used to obtain significant variables for the final model. Subsequently, a comprehensive nomogram based on the findings of multivariable Cox regression was built to predict the 1-, 3-, and 5-year PFS of patients with ccRCC. We used the Schoenfeld residuals to check the proportional hazards assumption in the Cox model. The a priori level of significance was set at 0.05, and the hypothesis tests were 2-sided. The interclass correlation coefficient (ICC) was calculated between 2 observers for T1 mapping parameters. An ICC less than 0.40 was considered indicative of poor interobserver reliability, 0.40–0.59 as fair, 0.60–0.74 as good, and 0.75–1.00 as excellent (21). Kaplan-Meier plots and the log-rank test were used to evaluate the PFS among different independent risk factors. The optimal cutoff value of risk factors was calculated by the receiver operator characteristic (ROC) curve and the Youden index. Statistical analyses were performed with SPSS (version 26.0; IBM Corp., Armonk, NY, USA).
Results
Clinical and pathological characteristics
Table 1 shows the clinical and pathological characteristics of the enrolled patients. Finally, a total of 195 patients with pathologically confirmed ccRCCs were analyzed. Among them, 133 (68%) were male and 62 (32%) were female patients. The mean age was 56.0±12.0 years (range, 26.0–83.0 years). A total of 10 (5%) patients had Fuhrman grade 1 ccRCCs, 151 (77%) had grade 2, 34 (17%) had grade 3, and none (0%) had grade 4. The mean size of the lesions was 4.1±2.1 cm (range, 1.2–10.7 cm).
Table 1
Variable | All patients (n=195) |
---|---|
Gender | |
Male | 133 [68] |
Female | 62 [32] |
Mean age (years)a | 56.0±12.0 (26.0–83.0) |
Surgery type | |
Partial nephrectomy | 113 [58] |
Radical nephrectomy | 82 [42] |
Fuhrman/ISUP grade | |
Grade 1 | 10 [5] |
Grade 2 | 151 [77] |
Grade 3 | 34 [17] |
Grade 4 | 0 [0] |
Mean size (cm)a | 4.1±2.1 (1.2–10.7) |
Location | |
Laterality | |
Left | 98 [50] |
Right | 97 [50] |
Polar | |
Upper | 63 [32] |
Middle | 66 [34] |
Lower | 66 [34] |
Growth pattern | |
Endophytic | 36 [18] |
Mixed | 91 [47] |
Exophytic | 68 [35] |
a, data are mean ± standard deviation, and data in parentheses are range. Other data are numbers of patients, with percentages in parentheses.
The median follow-up period was 27.6 months (range, 1–88 months). During the follow-up period, progression of ccRCCs was observed in 22 (11.3%) of 195 patients. Among 22 patients with progression, local recurrence occurred in 2 (9.1%) patients and the time to tumor recurrence was 22 and 53 months, respectively. Distant metastases occurred in 20 patients. Among this group, 13 (59%) patients had lung metastases (median, 14.3 months; range, 1–41 months); 3 (13.6%) patients had bone metastases and the time to tumor recurrence was 11, 33, and 48 months, respectively; 2 (9.1%) patients had retroperitoneal lymph node metastases and the time to tumor recurrence was 43 and 45 months, respectively; 1 (4.5%) patient had metastases in the liver after 30 months follow-up and 1 (4.5%) in the pancreas after 1 month follow-up.
Risk factors of recurrence
Univariate and multivariate Cox regression analyses were applied to identify the preoperative risk factors between the progression group and the non-progression group. At univariate Cox analysis, male gender, older age, larger tumor diameter, irregular shape, tumor necrosis, incomplete capsule, no-defined margin, hypointense T2WI signal intensity, lower ADC value, signal intensity drop-out in out-of-phase T1WI, the enhancement pattern of “wash-in and wash-out” and “delayed enhancement”, the higher post-contrasted T1 relaxation time, and the lower T1r were statistically correlated to tumor recurrence. However, the CMPr and NPr were not statistically different between the progression group and the non-progression group of ccRCCs.
According to multivariate Cox analysis, only the incomplete tumor capsule [P<0.001; hazard ratio (HR), 7.849; 95% confidence interval (CI): 2.614–23.570] and the higher post-contrasted T1 relaxation time (P=0.001; HR, 2.077; 95% CI: 1.350–3.196) were independently associated with ccRCC recurrence. According to multivariate analysis, ADC was not a significant risk factor (Table 2). The prognostic nomograms for 1-, 3-, and 5-year PFS were established by independent risk factors identified in the multivariate Cox analysis. The enhanced T1 relaxation time showed the maximum weight of the points. The age of the patients had a moderate impact on PFS. Capsule completeness showed the minimum weight of the points (Figure 2).
Table 2
Variable | Univariate analysis | Multivariate analysis | |||
---|---|---|---|---|---|
HR (95% CI) | P | HR (95% CI) | P | ||
Gender (male/female) | 5.093 (1.188–21.837) | 0.028* | – | – | |
Mean age (years) | 2.198 (1.326–3.645) | 0.002* | 1.811 (1.001–3.276) | 0.050* | |
Mean size (cm) | 2.244 (1.628–3.092) | 0.000* | – | – | |
Shape (round/ irregular) | 8.110 (2.966–22.178) | 0.000* | – | – | |
Composition | |||||
Predominantly solid | Reference | 0.568 | – | – | |
Mixed solid and cystic | 1.200 (0.465–3.096) | 0.143 | – | – | |
Predominantly cystic | 0.380 (0.050–2.882) | 0.349 | – | – | |
Hemorrhage (Y:N) | 1.641 (0.709–3.802) | 0.248 | – | – | |
Necrosis | |||||
Absent | Reference | 0.038* | – | – | |
<50% | 5.691 (1.314–24.651) | 0.020* | – | – | |
≥50% | 9.185 (1.530–55.143) | 0.015* | – | – | |
Capsule (intact/incomplete) | 10.285 (3.447–30.691) | 0.000* | 7.849 (2.614–23.570) | 0.000* | |
Margin (well-defined/no) | 9.413 (3.926–22.572) | 0.000* | – | – | |
T2 signal (hyper-/hypointense) | 0.379 (0.154–0.930) | 0.034* | – | – | |
ADC value | 0.623 (0.412–0.940) | 0.024* | – | – | |
Out-of-phase T1 (signal dropout/no) | 4.911 (1.661–14.521) | 0.004* | – | – | |
Enhancement degree | |||||
Low | Reference | 0.444 | – | – | |
Middle | 0.038 (0.000–22.710) | 0.317 | – | – | |
High | 0.038 (0.000–124.429) | 0.429 | – | – | |
Enhancement pattern | |||||
Wash-in and wash-out | Reference | 0.026* | – | – | |
Persistent enhancement | 0 | 0.957 | – | – | |
Delayed enhancement | 0.286 (0.116–0.709) | 0.007* | – | – | |
T1 relaxation time | |||||
Pre-contrasted | 0.811 (0.508–1.296) | 0.381 | – | – | |
Post-contrasted | 2.328 (1.507–3.596) | 0.000* | 2.077 (1.350–3.196) | 0.001* | |
T1r | 0.626 (0.450–0.870) | 0.005* | – | – | |
CMPr | 0.967 (0.663–1.409) | 0.861 | – | – | |
NPr | 0.819 (0.552–1.213) | 0.319 | – | – |
*, P≤0.05. PFS, progression-free survival; ccRCC, clear cell renal cell carcinoma; HR, hazard ratio; CI, confidence interval; Y:N, yes:no; ADC, apparent diffusion coefficient; T1r, reduction rate of T1 relaxation time; CMPr, enhancement rate of corticomedullary phase; NPr, enhancement rate of nephrographic phase.
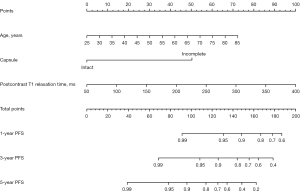
Diagnostic performance of T1 relaxation time
The agreement on the T1 relaxation time between the 2 observers was excellent. The ICC of pre-contrasted, post-contrasted, and T1r were 0.821 (95% CI: 0.770–0.862), 0.963 (95% CI: 0.951–0.972), and 0.913 (95% CI: 0.886–0.934), respectively. The mean post-contrasted T1 relaxation time in the recurrence group was significantly higher (273.99±38.55 ms) than those in the non-recurrence group (209.42±61.26 ms), and the T1r was lower (84.15%±4.66% vs. 87.83%±5.29%) (Figure 3).
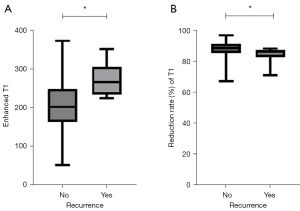
PFS for different risk factors
Based on the ROC curve analysis, the optimal cut-off point for post-contrasted T1 relaxation time was 222.73 ms [P<0.001; area under the curve (AUC) =0.814; 95% CI: 0.749–0.879]. Patients with higher post-contrasted T1 relaxation time showed worse PFS than those with lower T1 values (log-rank test, χ2=25.612, P<0.001), and the sensitivity and specificity for predicting tumor progression were 100% and 61.8%, respectively. Worse outcomes were shown in patients with incomplete tumor capsules (log-rank test, χ2=26.475, P<0.001) (Figure 4).
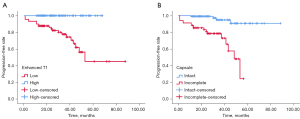
Discussion
Identifying predictors of tumor progression would assist in determining an optimal treatment strategy for patients with ccRCCs. Our study demonstrated that the higher post-contrasted T1 relaxation time and the presence of incomplete capsules of the tumors may predict the worse PFS of ccRCCs after nephrectomy. Figure 5 shows an example of such a case.
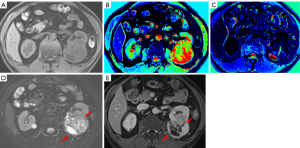
The paramagnetic effect of contrast agents (CAs) can reduce the T1 relaxation time, which means the higher the concentration of CAs in the lesions, the lower the post-contrasted T1 relaxation time will be obtained. We observed that the high-risk group of ccRCCs showed a lower concentration of CAs. There are 3 possible reasons for this phenomenon. Firstly, more highly aggressive ccRCCs were observed with more complex cell structures and immature malformed vessels, secondarily decreasing its microvascular density (MVD) (22). Similarly, Yhee et al. (23) and Hemmerlein et al. (24) have shown a significant decrease in MVD from histopathological G1 to G3 RCCs. We speculated that the prolonged post-contrasted T1 relaxation time would be due to the reduction of MVD in the higher aggressive ccRCCs. According to the study of Imao et al. (22), high-grade RCCs enlarge more rapidly, exceeding their vascular network, and neovascularization cannot keep up with tumor cell proliferation. Secondly, most of the ccRCCs display heterogeneity. Although we had done our best to avoid ROIs with the macroscopic areas of tumor degeneration, it may still be difficult to exclude the microscopic necrosis and cystic zones (25-27), which potentially negatively affect the concentration of CAs in aggressive ccRCCs. Thirdly, it was found that tumor progression of ccRCCs was related to the upregulation of extracellular matrix genes and proteins, particularly in higher-grade ccRCCs (28,29). Poorly differentiated ccRCCs were composed of relatively large and irregular tumor cells, resulting in higher cell density and narrow extravascular or interstitial spaces (30), which may partially explain a lower CAs uptake in more highly aggressive ccRCCs. Furthermore, a study by Peng et al. (31) demonstrated that the higher Edmondson-Steiner grade of hepatocellular carcinoma (HCC), the higher the T1 values of the lesions after gadolinium ethoxybenzyl-diethylenetriaminepentaacetic acid (Gd-EOB-DTPA) administration. It was considered that poorly differentiated HCC could result in the loss of part of liver cell functions, leading to a reduced CAs uptake, which may also explain the process in ccRCCs. In addition, our results showed that the CMPr and NPr were not useful in predicting the PFS of ccRCC. This may indicate that T1 mapping can reflect the internal characteristics of tumors, which has advantages over semi-quantitative signal intensity change of the lesions.
We also found that the presence of tumor incomplete capsules was an independent prognostic factor for tumor progression, which was consistent with the results of the previous studies (32). In our cohort, a total of 68 (35%) cases were identified as incomplete capsules, this was in accordance with the results obtained by the previous studies (33,34). The fibrous capsule is considered a result of the expansive growth of the tumor. The presence of a fibrous capsule is a crucial element in determining the tumor’s minimal margin during nephron-sparing surgery. Invasion beyond the renal capsule was a prognostic parameter and was noted to be associated with a poor outcome in patients with renal tumors (35). CcRCCs with infiltration beyond the renal capsule into the peri-renal fat were categorized as T3a stage by the tumor, node, metastasis (TNM) staging system (36). Xi et al. indicated that the absence of pseudocapsule on CT significantly increased the risk of adverse clinical outcomes in RCCs (37). Papalia et al. showed that the characterization of pseudocapsule invasion in renal tumors using MRI could reach a sensitivity of 93.75%, a specificity of 95%, and an AUC of 0.94 (38). A large amount of cohort studies are warranted to further evaluate the prognostic value of fibrosis capsules in ccRCCs.
Previous studies have shown that ADC value derived from functional DWI may effectively help predict the recurrence of RCCs (39,40). However, our results revealed that ADC value was not an independent risk factor for PFS of RCCs. A possible explanation might be the different b values we selected. Furthermore, ADC value can be affected by various factors, including but not limited to MRI scanners, field strengths, and MRI protocols (41).
With the development of novel technologies and artificial intelligence, quantitative imaging has several potential applications in grading renal malignancy. Choi et al. (42) reported that MR radiomics can accurately predict the tumor stage, size, grade, and necrosis (SSIGN) score of ccRCCs, and achieve the highest AUC score at 0.94. Zhao et al. (43) found that a deep learning model based on conventional MRI can predict the histological grade of stage I and II RCCs, and achieved a test accuracy of 0.88. However, there is still a paucity of follow-up information on RCCs in those current published studies.
There are several limitations in our study. Firstly, a sampling bias cannot be excluded; the study included 195 patients with ccRCCs (22 patients with tumor progression and 175 patients without progression). The prognosis of early-stage RCCs is relatively good (44). Secondly, only one 1.5-T MRI scanner was used and the variation of rescanning was not tested, which means that the results of this study may not be generalized to all institutions. Thirdly, only ccRCCs were included in our study; more renal malignant masses may be further investigated, such as papillary and chromophobe subtypes of RCCs. Fourthly, we failed to establish artificial intelligence models, specifically convolutional neural networks (CNN), because of technical limitations. Such research would be the next step in our research program.
Conclusions
Quantitative T1 mapping parameters may be a potential prognostic marker in ccRCCs. The higher enhanced T1 relaxation time could be a predictor of poor prognosis in patients with ccRCCs. In any event, further studies with larger sample sizes are needed to explore the full potential of T1 mapping in ccRCCs.
Acknowledgments
We would like to acknowledge the reviewers for their helpful comments on this paper.
Funding: This study was supported by
Footnote
Reporting Checklist: The authors have completed the STROBE reporting checklist. Available at https://qims.amegroups.com/article/view/10.21037/qims-23-1829/rc
Conflicts of Interest: All authors have completed the ICMJE uniform disclosure form (available at https://qims.amegroups.com/article/view/10.21037/qims-23-1829/coif). The authors have no conflicts of interest to declare.
Ethical Statement: The authors are accountable for all aspects of the work in ensuring that questions related to the accuracy or integrity of any part of the work are appropriately investigated and resolved. The study was conducted in accordance with the Declaration of Helsinki (as revised in 2013). This retrospective cohort study obtained approval from the Institutional Review Board of Zhongshan Hospital (No. B2020-214R). Since this is a retrospective review study, the requirement for written informed consent was waived.
Open Access Statement: This is an Open Access article distributed in accordance with the Creative Commons Attribution-NonCommercial-NoDerivs 4.0 International License (CC BY-NC-ND 4.0), which permits the non-commercial replication and distribution of the article with the strict proviso that no changes or edits are made and the original work is properly cited (including links to both the formal publication through the relevant DOI and the license). See: https://creativecommons.org/licenses/by-nc-nd/4.0/.
References
- Marconi L, Dabestani S, Lam TB, Hofmann F, Stewart F, Norrie J, Bex A, Bensalah K, Canfield SE, Hora M, Kuczyk MA, Merseburger AS, Mulders PFA, Powles T, Staehler M, Ljungberg B, Volpe A. Systematic Review and Meta-analysis of Diagnostic Accuracy of Percutaneous Renal Tumour Biopsy. Eur Urol 2016;69:660-73. [Crossref] [PubMed]
- Muglia VF, Prando A. Renal cell carcinoma: histological classification and correlation with imaging findings. Radiol Bras 2015;48:166-74. [Crossref] [PubMed]
- Staehler M, Motzer RJ, George DJ, Pandha HS, Donskov F, Escudier B, Pantuck AJ, Patel A, DeAnnuntis L, Bhattacharyya H, Ramaswamy K, Zanotti G, Lin X, Lechuga M, Serfass L, Paty J, Ravaud A. Adjuvant sunitinib in patients with high-risk renal cell carcinoma: safety, therapy management, and patient-reported outcomes in the S-TRAC trial. Ann Oncol 2018;29:2098-104. [Crossref] [PubMed]
- Lane BR, Kattan MW. Prognostic models and algorithms in renal cell carcinoma. Urol Clin North Am 2008;35:613-25. vii. [Crossref] [PubMed]
- Delahunt B, Cheville JC, Martignoni G, Humphrey PA, Magi-Galluzzi C, McKenney J, Egevad L, Algaba F, Moch H, Grignon DJ, Montironi R, Srigley JRMembers of the ISUP Renal Tumor Panel. The International Society of Urological Pathology (ISUP) grading system for renal cell carcinoma and other prognostic parameters. Am J Surg Pathol 2013;37:1490-504. [Crossref] [PubMed]
- Frank I, Blute ML, Cheville JC, Lohse CM, Weaver AL, Zincke H. An outcome prediction model for patients with clear cell renal cell carcinoma treated with radical nephrectomy based on tumor stage, size, grade and necrosis: the SSIGN score. J Urol 2002;168:2395-400. [Crossref] [PubMed]
- Hundshammer C, Braeuer M, Müller CA, Hansen AE, Schillmaier M, Düwel S, Feuerecker B, Glaser SJ, Haase A, Weichert W, Steiger K, Cabello J, Schilling F, Hövener JB, Kjær A, Nekolla SG, Schwaiger M. Simultaneous characterization of tumor cellularity and the Warburg effect with PET, MRI and hyperpolarized (13)C-MRSI. Theranostics 2018;8:4765-80. [Crossref] [PubMed]
- Wang W, Cao K, Jin S, Zhu X, Ding J, Peng W. Differentiation of renal cell carcinoma subtypes through MRI-based radiomics analysis. Eur Radiol 2020;30:5738-47. [Crossref] [PubMed]
- Deng Y, Pan L, Xing W, Zhou Z, Chen J. Application of BOLD-MRI-based radiomics in differentiating malignant from benign renal tumors. Zhong Nan Da Xue Xue Bao Yi Xue Ban 2021;46:1010-7. [Crossref] [PubMed]
- Wang C, Padgett KR, Su MY, Mellon EA, Maziero D, Chang Z. Multi-parametric MRI (mpMRI) for treatment response assessment of radiation therapy. Med Phys 2022;49:2794-819. [Crossref] [PubMed]
- Zhou C, Ban X, Lv J, Cheng L, Xu J, Shen X. Role of computed tomography features in the differential diagnosis of chromophobe renal cell carcinoma from oncocytoma and angiomyolipoma without visible fat. Quant Imaging Med Surg 2022;12:2332-43. [Crossref] [PubMed]
- Haaf P, Garg P, Messroghli DR, Broadbent DA, Greenwood JP, Plein S. Cardiac T1 Mapping and Extracellular Volume (ECV) in clinical practice: a comprehensive review. J Cardiovasc Magn Reson 2016;18:89. [Crossref] [PubMed]
- De Cecco CN, Monti CB. Use of Early T1 Mapping for MRI in Acute Myocarditis. Radiology 2020;295:326-7. [Crossref] [PubMed]
- Hueper K, Peperhove M, Rong S, Gerstenberg J, Mengel M, Meier M, Gutberlet M, Tewes S, Barrmeyer A, Chen R, Haller H, Wacker F, Hartung D, Gueler F. T1-mapping for assessment of ischemia-induced acute kidney injury and prediction of chronic kidney disease in mice. Eur Radiol 2014;24:2252-60. [Crossref] [PubMed]
- Adams LC, Ralla B, Jurmeister P, Bressem KK, Fahlenkamp UL, Hamm B, Busch J, Makowski MR. Native T1 Mapping as an In Vivo Biomarker for the Identification of Higher-Grade Renal Cell Carcinoma: Correlation With Histopathological Findings. Invest Radiol 2019;54:118-28. [Crossref] [PubMed]
- Wang S, Li J, Zhu D, Hua T, Zhao B. Contrast-enhanced magnetic resonance (MR) T1 mapping with low-dose gadolinium-diethylenetriamine pentaacetic acid (Gd-DTPA) is promising in identifying clear cell renal cell carcinoma histopathological grade and differentiating fat-poor angiomyolipoma. Quant Imaging Med Surg 2020;10:988-98. [Crossref] [PubMed]
- Jiang K, Ponzo TA, Tang H, Mishra PK, Macura SI, Lerman LO. Multiparametric MRI detects longitudinal evolution of folic acid-induced nephropathy in mice. Am J Physiol Renal Physiol 2018;315:F1252-60. [Crossref] [PubMed]
- Tewes S, Gueler F, Chen R, Gutberlet M, Jang MS, Meier M, Mengel M, Hartung D, Wacker F, Rong S, Hueper K. Functional MRI for characterization of renal perfusion impairment and edema formation due to acute kidney injury in different mouse strains. PLoS One 2017;12:e0173248. [Crossref] [PubMed]
- Wei CG, Zeng Y, Zhang R, Zhu Y, Tu J, Pan P, Ma Q, Wei LY, Zhao WL, Shen JK. Native T(1) mapping for non-invasive quantitative evaluation of renal function and renal fibrosis in patients with chronic kidney disease. Quant Imaging Med Surg 2023;13:5058-71. [Crossref] [PubMed]
- Zhang S, Song M, Zhao Y, Xu S, Sun Q, Zhai G, Liang D, Wu G, Li ZC. Radiomics nomogram for preoperative prediction of progression-free survival using diffusion-weighted imaging in patients with muscle-invasive bladder cancer. Eur J Radiol 2020;131:109219. [Crossref] [PubMed]
- Pérez Boal E, Martin-Villa C, Becerro de Bengoa Vallejo R, Losa Iglesias ME, Trevissón Redondo B, Casado Hernández I, Calvo Lobo C, Rodríguez Sanz D. Intra and Inter-Observer Reliability and Repeatability of Metatarsus Adductus Angle in Recreational Football Players: A Concordance Study. J Clin Med 2022;11:2043. [Crossref] [PubMed]
- Imao T, Egawa M, Takashima H, Koshida K, Namiki M. Inverse correlation of microvessel density with metastasis and prognosis in renal cell carcinoma. Int J Urol 2004;11:948-53. [Crossref] [PubMed]
- Yhee JY, Yu CH, Kim JH, Im KS, Kim NH, Brodersen BW, Doster AR, Sur JH. Angiogenesis and expression of vascular endothelial growth factor, tumour necrosis factor-α and hypoxia inducible factor-1α in canine renal cell carcinoma. J Comp Pathol 2012;147:129-38. [Crossref] [PubMed]
- Hemmerlein B, Kugler A, Ozisik R, Ringert RH, Radzun HJ, Thelen P. Vascular endothelial growth factor expression, angiogenesis, and necrosis in renal cell carcinomas. Virchows Arch 2001;439:645-52. [Crossref] [PubMed]
- Čechová M, Chocholatý M, Háček J, Schmidt M, Koldová M, Babjuk M. Histologic tumor necrosis rate as a predictor of recurrence after surgical treatment for localized clear cell renal cell carcinoma. Rozhl Chir 2022;101:114-8. [Crossref] [PubMed]
- Kuroe T, Watanabe R, Kojima M, Morisue R, Sugano M, Kuwata T, Masuda H, Kusuhara S, Matsubara N, Oda S, Ushiku T, Ishii G. Evaluation of the morphological features and unfavorable prognostic impact of dirty necrosis in renal cell carcinoma. J Cancer Res Clin Oncol 2021;147:1089-100. [Crossref] [PubMed]
- Delahunt B, McKenney JK, Lohse CM, Leibovich BC, Thompson RH, Boorjian SA, Cheville JC. A novel grading system for clear cell renal cell carcinoma incorporating tumor necrosis. Am J Surg Pathol 2013;37:311-22. [Crossref] [PubMed]
- Klatt MG, Kowalewski DJ, Schuster H, Di Marco M, Hennenlotter J, Stenzl A, Rammensee HG, Stevanović S. Carcinogenesis of renal cell carcinoma reflected in HLA ligands: A novel approach for synergistic peptide vaccination design. Oncoimmunology 2016;5:e1204504. [Crossref] [PubMed]
- Wan F, Wang H, Shen Y, Zhang H, Shi G, Zhu Y, Dai B, Ye D. Upregulation of COL6A1 is predictive of poor prognosis in clear cell renal cell carcinoma patients. Oncotarget 2015;6:27378-87. [Crossref] [PubMed]
- Dagher J, Delahunt B, Rioux-Leclercq N, Egevad L, Varma M, Samaratunga H. Percentage grade 4 tumour predicts outcome for clear cell renal cell carcinoma. Pathology 2019;51:349-52. [Crossref] [PubMed]
- Peng Z, Jiang M, Cai H, Chan T, Dong Z, Luo Y, Li ZP, Feng ST. Gd-EOB-DTPA-enhanced magnetic resonance imaging combined with T1 mapping predicts the degree of differentiation in hepatocellular carcinoma. BMC Cancer 2016;16:625. [Crossref] [PubMed]
- Abou Elkassem AM, Lo SS, Gunn AJ, Shuch BM, Dewitt-Foy ME, Abouassaly R, Vaidya SS, Clark JI, Louie AV, Siva S, Grosu AL, Smith AD. Role of Imaging in Renal Cell Carcinoma: A Multidisciplinary Perspective. Radiographics 2021;41:1387-407. [Crossref] [PubMed]
- Xi W, Wang J, Liu L, Xiong Y, Qu Y, Lin Z, Chen X, Wang Z, Xu J, Guo J. Evaluation of Tumor Pseudocapsule Status and its Prognostic Significance in Renal Cell Carcinoma. J Urol 2018;199:915-20. [Crossref] [PubMed]
- Takagi T, Yoshida K, Kondo T, Kobayashi H, Iizuka J, Okumi M, Ishida H, Nagashima Y, Tanabe K. Peritumoral pseudocapsule status according to pathological characteristics from robot-assisted laparoscopic partial nephrectomy for localized renal cell carcinoma. Int J Urol 2019;26:446-50. [Crossref] [PubMed]
- Delahunt B, Eble JN, Samaratunga H, Thunders M, Yaxley JW, Egevad L. Staging of renal cell carcinoma: current progress and potential advances. Pathology 2021;53:120-8. [Crossref] [PubMed]
- Guo P, Wang Y, Han Y, Wei D, Zhao J, Li M, Jiang Y, Luo Y. Oncological Outcomes of Patients With Different Pathological Features of pT3a Renal Tumor: A Systematic Review and Quantitative Synthesis. Front Oncol 2021;11:678459. [Crossref] [PubMed]
- Xi W, Tan Q, Hou Y, Hu X, Wang H, Liu L, Xia Y, Bai Q, Wang J, Zhou J, Guo J. No Detection of Pseudocapsule of Tumor-Parenchyma Interface on Multidetector Computed Tomography (MDCT) Images: Clinical Significance and Histological Reflections in Renal Cell Carcinoma. Cancer Manag Res 2021;13:5403-11. [Crossref] [PubMed]
- Papalia R, Panebianco V, Mastroianni R, Del Monte M, Altobelli E, Faiella E, Grasso FR, Bellangino M, Simone G, Ciccozzi M, Angeletti S, D'ovidio G, Catalano C, Gallucci M, Scarpa RM, Muto G. Accuracy of magnetic resonance imaging to identify pseudocapsule invasion in renal tumors. World J Urol 2020;38:407-15. [Crossref] [PubMed]
- Mytsyk Y, Borzhiyevskyy A, Dutka I, Shulyak A, Kowal P, Vorobets D, Skrzypczyk M, Borzhiyevs'kyy O, Górecki A, Matskevych V. Local recurrence of renal cell carcinoma after partial nephrectomy: applicability of the apparent diffusion coefficient of MRI as an imaging marker - a multicentre study. Pol J Radiol 2022;87:e325-32. [Crossref] [PubMed]
- Nakamura T, Yoshizako T, Araki H, Maruyama M, Uchida K, Tamaki Y, Ishikawa N, Shiina H, Kitagaki H. The relation between apparent diffusion coefficient and clinical stage of clear-cell renal cell carcinoma. Clin Imaging 2015;39:72-5. [Crossref] [PubMed]
- Driessen JP, van Kempen PM, van der Heijden GJ, Philippens ME, Pameijer FA, Stegeman I, Terhaard CH, Janssen LM, Grolman W. Diffusion-weighted imaging in head and neck squamous cell carcinomas: a systematic review. Head Neck 2015;37:440-8. [Crossref] [PubMed]
- Choi JW, Hu R, Zhao Y, Purkayastha S, Wu J, McGirr AJ, Stavropoulos SW, Silva AC, Soulen MC, Palmer MB, Zhang PJL, Zhu C, Ahn SH, Bai HX. Preoperative prediction of the stage, size, grade, and necrosis score in clear cell renal cell carcinoma using MRI-based radiomics. Abdom Radiol (NY) 2021;46:2656-64. [Crossref] [PubMed]
- Zhao Y, Chang M, Wang R, Xi IL, Chang K, Huang RY, Vallières M, Habibollahi P, Dagli MS, Palmer M, Zhang PJ, Silva AC, Yang L, Soulen MC, Zhang Z, Bai HX, Stavropoulos SW. Deep Learning Based on MRI for Differentiation of Low- and High-Grade in Low-Stage Renal Cell Carcinoma. J Magn Reson Imaging 2020;52:1542-9. [Crossref] [PubMed]
- Tang J, Wang J, Pan X, Liu X, Zhao B. A Web-Based Prediction Model for Cancer-Specific Survival of Middle-Aged Patients With Non-metastatic Renal Cell Carcinoma: A Population-Based Study. Front Public Health 2022;10:822808. [Crossref] [PubMed]