High-resolution magnetic resonance imaging features of time-of-flight magnetic resonance angiography signal loss and its relevance to ischemic stroke
Introduction
Intracranial atherosclerotic stenosis (ICAS) is one of the leading causes of ischemic stroke worldwide, especially in Asian populations (1). The middle cerebral artery (MCA) is most frequently involved and accounts for approximately 40–70% of ICAS cases (2). Time-of-flight magnetic resonance angiography (TOF-MRA) is a widely used noninvasive imaging technique for evaluating ICAS. Focal signal loss usually occurs in the intracranial arteries on three-dimensional (3D) TOF-MRA, with its distal vessels being relatively well visualized. This type of signal loss on MRA usually occurs in vessels with stenosis >50% (3). Previous studies on carotid artery and MCA stenosis, using digital subtraction angiography (DSA) as the standard, concluded that signal loss on MRA represents moderate-or-severe stenosis of vessels (4,5) and thus is often referred to as a “false positive” in clinical practice. However, focal signal loss does not occur in all arteries with moderate or severe stenosis, and There are no studies have examined what differentiates moderate-to-severe stenosis with signal loss from that without signal loss. Dual screening of TOF-MRA and high-resolution magnetic resonance imaging (HR-MRI) can ensure that the focal signal loss is at the point of arterial plaque formation and at the narrowest part of the lumen. HR-MRI, or more precisely, high-resolution intracranial vessel wall MRI (VW-MRI) (6), is a noninvasive magnetic resonance (MR) intracranial vascular wall imaging technique that can assess arterial stenosis and plaque characteristics (7-10). It is regarded as a reliable technique due to its high consistency with DSA for the diagnosis of intracranial arterial stenosis (11).
In this study, we focused on the M1 segment of MCA and used HR-MRI to investigate the vessel wall characteristics and clinical value of focal signal loss on 3D-TOF-MRA maximal intensity projection (MIP) images. We present this article in accordance with the STROBE reporting checklist (available at https://qims.amegroups.com/article/view/10.21037/qims-24-329/rc).
Methods
Patients
In this cross-sectional study, data from patients with MCA M1 segment focal signal loss or stenosis (50–99%) detected with TOF-MRA between January 2018 and December 2022 at the China-Japan Union Hospital of Jilin University Neurology Department were retrospectively reviewed. All patients fulfilled the following criteria: (I) completion of routine brain MRI [T1-, T2-weighted, fluid-attenuated inversion recovery, and diffusion-weighted imaging (DWI)], with HR-MRI being performed within 2 weeks; (II) lack of coexistent >70% ipsilateral extracranial or intracranial internal carotid artery stenosis; (III) no clinical evidence of cardioembolism (recent myocardial infarction within less than 3 weeks, atrial fibrillation, mitral stenosis or prosthetic valve, dilated cardiomyopathy, sick sinus syndrome, acute bacterial endocarditis, patent foramen ovale, etc.); (IV) good image quality for vessel wall and lumen identification; and (V) complete medical registration and treatment records. The following general clinical information was collected from all patients: age, sex, history of hypertension, diabetes mellitus, hyperlipidemia, hyperhomocysteinemia, smoking, and alcohol consumption.
In total, 401 patients with moderate-to-severe stenosis (50–99%) of the MCA M1 segment on 3D-TOF-MRA MIP images were included in this study, comprising 417 stenosis lesions. Finally, 414 cases of atherosclerotic stenosis were included in the analysis, including 231 stenotic lesions with hyperhomocysteinemia and 183 stenotic lesions without focal signal loss in the MCA.
As shown on HR-MRI, 275 out of these 401 patients had severe stenosis in the M1 segment of the MCA, and they were divided into a symptomatic (n=151) and an asymptomatic (n=124) group based on whether they had recent ischemic stroke (a diagnosis of ischemic stroke within the preceding 2 weeks confirmed by DWI showing high signal and low or equal signal lesions based on the apparent diffusion coefficient at the corresponding locations in the brain parenchyma of the MCA blood supply area) (12,13). All the recent stroke survivors enrolled received systematic drug treatment after diagnosis, but none received endovascular stent treatment.
The study was conducted in accordance with the Declaration of Helsinki (as revised in 2013) and was approved by the Ethics Committee of China-Japan Union Hospital, Jilin University (No. 220506014). Written informed consent was obtained from all patients.
Imaging protocol
Imaging was performed using a 3-T system (MAGNETOM Skyra; Siemens Healthineers, Erlangen, Germany) equipped with a standard 8-channel head coil. The protocol consisted of 3D-TOF-MRA and pre- and postcontrast 3-dimensional T1-weighted HR-MRI. The 3D-TOF-MRA locator selected the MIP image and the source image of the 3D TOF-MRA as the topographic map to ensure that the cross-sectional image was perpendicular to the M1 segment of the MCA. The imaging parameters for high-resolution vessel wall imaging were as follows: repetition time/echo time, 900/15 ms; field of view, 127×164 mm2; 192 slices; layer thickness, 0.63 mm; and matrix size, 256×256. The imaging parameters for TOF-MRA were as follows: repetition time/echo time, 20/3.69 ms; field of view, 200×200 mm2, 158 slices; field of view, 200×200 mm2; and matrix size, 352×256. Postcontrast HR-MRI was performed 5 minutes after the injection of a single dose (0.1 mmol/kg of body weight) of gadolinium-based contrast agent.
Image analysis
All image analysis and parameter measurements were performed using a research picture archiving communications system workstation (Philips, Amsterdam, the Netherlands). Stenosis was categorized as mild (0–49% stenosis), moderate (50–69% stenosis), severe (70–99% stenosis), and occluded. The following equation was used to determine the percent stenosis of an MCA: percent stenosis = (1 − Dstenosis/Dnormal) × 100%, where Dstenosis is the diameter of the artery at the site with the most severe degree of stenosis, and Dnormal is the diameter of the proximal normal artery. Severe stenosis was defined as focalized signal reduction or loss in the MRA where Dstenosis could not be measured with calipers (14).
Whole-brain HR-MRI was reviewed for a detailed analysis of the MCA M1 segment with moderate-to-severe stenosis in each patient. HR-MRI images were evaluated by two experienced neuroradiologists who were blinded to the identity and clinical information of the patients. Any disagreements were resolved through discussion. T1-weighted pre- and postcontrast images in three planes (axial, coronal, and sagittal) were analyzed to determine the presence of atherosclerotic plaques or other vascular lesions. Plaque was defined as a thickening of the vessel wall with reference to an adjacent proximal, distal, or contralateral vessel segment. Meanwhile, the culprit plaque was defined as the only lesion present within the vascular territory of the stroke or the most stenotic lesion when multiple plaques were present within the same vascular territory of the stroke (15).
The plaque characteristics were also evaluated. First, the degree of stenosis of the MCA on HR-MRI was calculated using the following formula: (1 − LAnarrow/LAreference) × 100% (13). The sagittal precontrast T1-weighted images (T1WIs) were magnified to 300%, and the lumen area (LA) of the stenotic MCA was measured at the most narrow LA (LAnarrow) and the LA at the reference site (LAreference). The reference site was defined as the nearest proximal segment of the MCA stenosis that was free of plaque or contained the least amount of lesion. When a proximal reference site was not available, the adjacent distal reference site was used. Second, plaque distribution was characterized as eccentric if wall involvement was <50% and concentric if it was >50% (16). Third, intraplaque hemorrhage was defined as a high signal on T1-weighted fat-suppressed images that was 150% higher than the signal of the adjacent brain tissue (17,18). Fourth, the plaque enhancement grade was classified as follows: grade 0 if the enhancement was not greater than that of the intracranial arterial walls without plaque in the same individual, grade 1 if the enhancement was greater than grade 0 but less than that of the pituitary infundibulum, and grade 2 if the enhancement was not less than that of the pituitary infundibulum. The degree of contrast enhancement (CE) was quantified using the following equation: CE = (SIpostcontrast − SIprecontrast)/SIprecontrast × 100, where SIpostcontrast and SIprecontrast are the plaque signal intensity (SI) after and before the standardized enhancement scan, respectively. Plaque SI was normalized by manual measurement the SI of the adjacent brain parenchyma (area of 15 mm2) (19). Finally, in the HR-MRI evaluation of intracranial artery dissection, the pathognomonic radiological findings of intracranial artery dissection included mural hematoma, intimal flap, and double lumen (20).
Statistical analysis
SPSS 25 (IBM Corp., Armonk, NY, USA) for Windows was used for statistical analyses. Statistical significance was set at P<0.05. Nonnormally distributed continuous variables were compared using the median and interquartile ranges, and categorical variables were expressed as values and percentages. The differences between the groups were analyzed using the Chi-squared test or Fisher exact test for categorical variables and the Mann-Whitney test for nonnormally distributed continuous variables. Independent predictors of focal signal loss in the M1 segment of the MCA and independent risk factors for recent ischemic stroke were analyzed using binary logistic regression analysis. Factors with P<0.1 in the univariate analysis or that were considered as being closely related clinically to the dependent variable were included as covariates in the multivariate analysis.
Results
Patient characteristics
In total, 401 patients with moderate-to-severe stenosis (50–99%) of the MCA M1 segment on 3D-TOF-MRA MIP images were included in this study, comprising 417 stenosis lesions, including 233 stenotic lesions with and 184 stenotic lesions without focal signal loss in the MCA. On HR-MRI, there were 231 atherosclerotic plaques and 2 intercalations in the group with signal loss, and there were 183 atherosclerotic plaques and 1 intercalation in the group without signal loss. Finally, 414 cases of atherosclerotic stenosis were included in the analysis. Table 1 presents the patient demographics and the main risk factors of atherosclerosis. The differences in age and hypertension between the two groups were statistically significant (P<0.05), as determined through univariate analysis.
Table 1
Characteristic | Signal loss (n=231) | Without signal loss (n=183) | P value |
---|---|---|---|
Male, n (%) | 157 (68.0) | 111 (32.0) | 0.122 |
Age (years), median [IQR] | 56 [45–66] | 59 [52–66] | 0.014 |
Hypertension, n (%) | 120 (51.9) | 113 (61.7) | 0.046 |
Hyperlipidemia, n (%) | 219 (94.8) | 169 (92.3) | 0.306 |
Hyperhomocysteinemia, n (%) | 98 (42.4) | 87 (48.5) | 0.298 |
Diabetes mellitus, n (%) | 93 (40.3) | 78 (42.6) | 0.628 |
Smoker, n (%) | 104 (45.0) | 71 (38.8) | 0.203 |
Drinker, n (%) | 60 (26.0) | 48 (26.2) | 0.953 |
Symptomatic†, n (%) | 134 (58.0) | 54 (29.5) | <0.001 |
†, a recent ischemic stroke. The P values were calculated using the Mann-Whitney test for continuous data and the Chi-square test for categorical data. IQR, interquartile range.
Plaque features in the two types of stenosis
The two groups were significantly different in terms of degree of stenosis (P<0.001), plaque enhancement grade (P<0.001), and plaque morphology (P<0.001). The focal signal loss group, as compared to the no-signal loss group, were more likely to have grade 2 enhanced plaques (82.3% vs. 28.4%, P<0.001) and concentric plaques (63.2% vs. 34.4%, P<0.001) (Table 2). Similar results were observed in patients with severe stenosis as shown on HR-MRI, with the the signal loss group showing a higher prevalence of grade 2 enhanced plaques (P<0.001) and concentric plaques (P=0.002) than the no-signal loss group (Table 2). A representative case is shown in Figure 1.
Table 2
HR-MRI feature | Signal loss (n=231) | Without signal loss (n=183) | P value | Signal losssevere (n=181) | Without signal losssevere (n=94) | Psevere value |
---|---|---|---|---|---|---|
Stenosis degree, n (%) | <0.001 | NA | NA | NA | ||
Mild | 9 (3.9) | 25 (13.7) | ||||
Moderate | 39 (16.9) | 64 (35.0) | ||||
Severe | 181 (78.4) | 94 (51.4) | ||||
Occluded | 2 (0.9) | 0 (0.0) | ||||
Plaque enhancement grade, n (%) | <0.001 | <0.001 | ||||
Grade 0 | 8 (3.4) | 97 (53.0) | 2.8% | 39.4% | ||
Grade 1 | 33 (14.3) | 34 (18.6) | 13.8% | 20.2% | ||
Grade 2 | 190 (82.3) | 52 (28.4) | 83.4% | 40.4% | ||
Grade 2 vs. non-grade 2† | 190/41 | 52/131 | <0.001 | 151/30 | 38/56 | <0.001 |
IPH, n (%) | 3 (1.3) | 0 (0) | 0.258 | NA | NA | NA |
Plaque distribution, n (%) | <0.001 | 0.002 | ||||
Concentric | 146 (63.2) | 63 (34.4) | 66.3% | 46.8% | ||
Eccentric | 85 (36.8) | 120 (65.6) | 33.7% | 53.2% | ||
Mean stenosis ratio (%) | NA | NA | 89.2% | 84.5% |
†, item is expressed in numerical values only. The P values for categorical data were calculated using the Chi-square test and Fisher exact test. HR-MRI, high-resolution magnetic resonance imaging; NA, not available; MCA, middle cerebral artery; IPH, intraplaque hemorrhage.
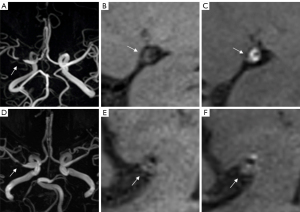
Association of plaque features with signal loss
Age, hypertension, and plaque characteristics were used as input variables for the logistic regression analysis. The degree of stenosis, grade 2 enhanced plaques, and concentric plaques remained significant in the multivariate analysis (P=0.025, P<0.001, and P=0.004, respectively), with odds ratios (ORs) of 1.600 [95% confidence interval (CI): 1.062–2.411], 8.552 (95% CI: 5.230–13.984), and 2.039 (95% CI: 1.257–3.307), respectively (Table 3).
Table 3
Characteristic | OR (95% CI) | P value |
---|---|---|
Age | 0.991 (0.971–1.011) | 0.390 |
Hypertension | 0.687 (0.419–1.126) | 0.137 |
Grade 2 enhanced plaque, n (%) | 8.552 (5.230–13.984) | <0.001 |
Concentric plaque, n (%) | 2.039 (1.257–3.307) | 0.004 |
Stenosis degree | 1.600 (1.062–2.411) | 0.025 |
OR, odds ratio; CI, confidence interval.
Associations of signal loss with recent ischemic stroke
The incidence of recent ischemic stroke among patients with severe stenosis on HR-MRI was higher in the group with signal loss than in that without signal loss (62.4% vs. 40.4%; P<0.001) (Table S1). A representative case is shown in Figure 1. The details of patient demographics, main atherosclerosis risk factors, and stenosis types on MRA are summarized in Table 4. Multifactorial logistic regression analysis showed that focal signal loss in the MCA M1 segment on MRA was an independent risk factor for recent ischemic stroke (OR =2.547; P<0.001) (Table S2).
Table 4
Characteristic | Symptomatic (n=151) | Asymptomatic (n=124) | P value |
---|---|---|---|
Male, n (%) | 98 (64.9) | 85 (68.5) | 0.524 |
Age (years), median [IQR] | 56 [48–66] | 57 [49–65] | 0.725 |
Hypertension, n (%) | 82 (54.3) | 70 (56.5) | 0.722 |
Hyperlipidemia, n (%) | 146 (96.7) | 120 (96.8) | 0.968 |
Hyperhomocysteinemia, n (%) | 74 (49.0) | 44 (35.5) | 0.024 |
Diabetes mellitus, n (%) | 67 (44.4) | 51 (41.1) | 0.589 |
Smoker, n (%) | 69 (45.7) | 46 (37.1) | 0.150 |
Drinker, n (%) | 42 (27.8) | 29 (23.4) | 0.404 |
3D-TOF-MRA signal loss, n (%) | 113 (74.8) | 68 (54.8) | <0.001 |
The P values were calculated using the Mann-Whitney test for categorical data with the Chi-square test for continuous data. 3D-TOF-MRA, three-dimensional time-flight method magnetic resonance angiography; IQR, interquartile range.
Discussion
In this study, we compared the wall characteristics of vessels with signal loss and those without signal loss using HR-MRI. In addition to the degree of stenosis, we found the vulnerability and morphology of plaques to be independently associated with vessel signal loss. The stroke occurrence in the group with vessel signal loss was significantly higher than that in the group without signal loss.
The focal signal loss phenomenon was thought to be a “false positive” or “flow void” owing to the velocity and direction of blood flow during the imaging process, reflecting moderate or severe stenosis (5,21). As the lumen diameter of the artery decreases, blood flow velocity increases as blood passes through the stenotic segment. Moreover, a jet with subsequent turbulent flow can be observed near the vessel wall in the poststenotic segment. In the TOF sequence, the loss of phase coherence of moving spins occurs with fast and turbulent flow, eventually leading to reduced flow SI (5,21). In this study, the degree of stenosis was independently associated with focal signal loss, which is consistent with previous findings (4,5).
MRA signal loss has been reported to be correlated with stenosis shape. Sharp-edged stenosis is more likely to lead to signal loss than is smoothly shaped stenosis when the severity of stenosis and flowrate are equivalent (22). Plaque enhancement is usually considered to be a sign of unstable plaque (23,24), which is often characterized by surface ulceration (25,26). The surface irregularities of enhanced plaques or the unsmooth edges of the stenosis wall caused by ulceration may be the reasons for signal loss (22). We found that compared to the group without signal loss, the group with signal loss had a higher proportion of grade 2-enhanced plaque, which was independently correlated with focal signal loss. Nevertheless, to further clarify the impact of factors other than the degree of stenosis on signal loss, we compared the data from patients with severe stenosis as confirmed by HR-MRI. Although the ratio of stenosis of the two groups was highly similar (Table 2), the results obtained were similar to those mentioned. Therefore, grade 2 contrast-enhanced plaque may be a more influential factor in signal loss than the degree of stenosis. Furthermore, a similar analysis was performed on mild stenosis as confirmed with HR-MRI in both the signal loss and the stenosis group (9 and 25 cases, respectively). Consequently, a higher proportion of plaque enhancement was observed in the signal loss group (P=0.002; Table S3). This finding is of note despite the small sample size it is derived from, but we did not investigate it further in our study.
An orifice shape in stenosis has been reported to contribute to signal loss when stenosis severity and flowrate are equivalent (22). In comparison with eccentric plaques, concentric plaques may easily cause orifice-type stenosis in the vessel lumen. In this study, an independent association with focal signal loss was observed for concentric plaque. Similar results were also found in the HR-MRI severe stenosis data, with the proportion of concentric plaque being higher in the signal loss group. Therefore, concentric plaque may be another factor that is more influential than the degree of stenosis in signal loss.
Our findings have certain clinical implications. Luminal stenosis with intracranial atherosclerotic plaque >50% is currently considered to be an independent predictor of ischemic stroke (27). However, studies have reported no significant differences in the degree of stenosis between symptomatic and asymptomatic groups of patients with moderate-to-severe MCA stenosis (13,28). The influence of collateral circulation status, plaque stability, hemodynamic effects, and other potential factors in predicting the risk of subsequent recurrent events may outweigh that of the degree of stenosis. A series of studies have confirmed that plaque CE and concentric plaques are closely related to the occurrence and recurrence of acute ischemic stroke; furthermore, it has been demonstrated that concentric plaques involve a wider area of the vessel wall and are more likely to develop into unstable plaques (19,29-34). This was confirmed in our study, as grade 2 enhanced plaques were more common in concentric than in eccentric plaques and concentric plaque was more prevalent in the focal signal loss group, which may explain the higher frequency of recent ischemic stroke in this group.
Although HR-MRI is superior in analyzing atherosclerotic plaque features and particularly important for the risk stratification of ischemic stroke due to intracranial arterial stenosis (35,36), it has obvious limitations in clinical application, such as the need for specialized imaging equipment and sequences and long scanning times. In this study, we showed that 3D-TOF-MRA, which is a relatively simple technique, can predict some of the wall features while obtaining information on the lumen of intracranial arteries. These results support the value of 3D-TOF-MRA MIP in the risk stratification of ischemic stroke in patients with intracranial vascular stenosis. Furthermore, DSA remains the gold standard for assessment of arterial stenosis. This study used the highly consistent characteristics of HR-MRI and DSA to evaluate stenosis (11), but DSA was not used for in-depth validation, which could be implemented in future research.
This study involved certain limitations which should be acknowledged. First, as we employed a retrospective design, future cohort studies can be devised to produce higher-quality evidence. Second, HR-MRI imaging scans were performed to be as perpendicular to the M1 segment of the stenotic MCA as possible; however, the tortuous shape of the MCA vessel alignment might have slightly affected the observations and measurements in this study. Third, unfortunately, the evaluation of the degree of vascular stenosis in this study was not been confirmed by DSA. Fourth, studies involving intraindividual comparisons with contrast-enhanced computed tomography angiography are needed in the future. Finally, further pathological confirmation regarding the vessel wall condition at the site of signal loss was not obtained.
Conclusions
Our findings suggest that focal signal loss on MRA can indicate the stability of atherosclerotic plaques and plaque morphology and be used to effectively assess ischemic stroke. In addition, patients with MRA focal signal loss in the MCA M1 segment had a higher incidence of recent ischemic stroke than those with moderate-to-severe—and even severe—stenosis. This may provide valuable insight into the risk stratification of patients with severe stenosis in the MCA.
Acknowledgments
The authors thank all those at the Department of Neurology and Radiology, China-Japan Union Hospital of Jilin University, who participated in this study, as well as the patients who were involved in this study.
Funding: This study was supported by
Footnote
Reporting Checklist: The authors have completed the STROBE reporting checklist. Available at https://qims.amegroups.com/article/view/10.21037/qims-24-329/rc
Conflicts of Interest: All authors have completed the ICMJE uniform disclosure form (available at https://qims.amegroups.com/article/view/10.21037/qims-24-329/coif). The authors have no conflicts of interest to declare.
Ethical Statement:
Open Access Statement: This is an Open Access article distributed in accordance with the Creative Commons Attribution-NonCommercial-NoDerivs 4.0 International License (CC BY-NC-ND 4.0), which permits the non-commercial replication and distribution of the article with the strict proviso that no changes or edits are made and the original work is properly cited (including links to both the formal publication through the relevant DOI and the license). See: https://creativecommons.org/licenses/by-nc-nd/4.0/.
References
- Zhang DF, Chen YC, Chen H, Zhang WD, Sun J, Mao CN, Su W, Wang P, Yin X. A High-Resolution MRI Study of Relationship between Remodeling Patterns and Ischemic Stroke in Patients with Atherosclerotic Middle Cerebral Artery Stenosis. Front Aging Neurosci 2017;9:140. [Crossref] [PubMed]
- Teng Z, Peng W, Zhan Q, Zhang X, Liu Q, Chen S, Tian X, Chen L, Brown AJ, Graves MJ, Gillard JH, Lu J. An assessment on the incremental value of high-resolution magnetic resonance imaging to identify culprit plaques in atherosclerotic disease of the middle cerebral artery. Eur Radiol 2016;26:2206-14. [Crossref] [PubMed]
- Fürst G, Hofer M, Sitzer M, Kahn T, Müller E, Mödder U. Factors influencing flow-induced signal loss in MR angiography: an in vitro study. J Comput Assist Tomogr 1995;19:692-9. [Crossref] [PubMed]
- Nederkoorn PJ, van der Graaf Y, Eikelboom BC, van der Lugt A, Bartels LW, Mali WP. Time-of-flight MR angiography of carotid artery stenosis: does a flow void represent severe stenosis? AJNR Am J Neuroradiol 2002;23:1779-84.
- You SH, Kim B, Yang KS, Kim BK, Woo S, Park SE. Development and validation of visual grading system for stenosis in intracranial atherosclerotic disease on time-of-flight magnetic resonance angiography. Eur Radiol 2022;32:2781-90. [Crossref] [PubMed]
- Mandell DM, Mossa-Basha M, Qiao Y, Hess CP, Hui F, Matouk C, Johnson MH, Daemen MJ, Vossough A, Edjlali M, Saloner D, Ansari SA, Wasserman BA, Mikulis DJVessel Wall Imaging Study Group of the American Society of Neuroradiology. Intracranial Vessel Wall MRI: Principles and Expert Consensus Recommendations of the American Society of Neuroradiology. AJNR Am J Neuroradiol 2017;38:218-29. [Crossref] [PubMed]
- Zhu XJ, Wang W, Liu ZJ. High-resolution Magnetic Resonance Vessel Wall Imaging for Intracranial Arterial Stenosis. Chin Med J (Engl) 2016;129:1363-70. [Crossref] [PubMed]
- Vranic JE, Hartman JB, Mossa-Basha M. High-Resolution Magnetic Resonance Vessel Wall Imaging for the Evaluation of Intracranial Vascular Pathology. Neuroimaging Clin N Am 2021;31:223-33. [Crossref] [PubMed]
- Zhang D, Wang M, Wu L, Zhao Y, Wang S, Yin X, Wu X. Assessing the characteristics and diagnostic value of plaques for patients with acute stroke using high-resolution magnetic resonance imaging. Quant Imaging Med Surg 2022;12:1529-38. [Crossref] [PubMed]
- Luo J, Bai X, Tian Q, Li L, Wang T, Xu R, Gong H, Wang Y, Chen Y, Gao P, Chen J, Yang B, Ma Y, Dmytriw AA, Jiao L. Patterns and implications of artery remodeling based on high-resolution vessel wall imaging in symptomatic severe basilar artery stenosis. Quant Imaging Med Surg 2023;13:2098-108. [Crossref] [PubMed]
- Gong Y, Cao C, Guo Y, Chang B, Sheng Z, Shen W, Zou Y, Lu X, Xing J, Xia S. Quantification of intracranial arterial stenotic degree evaluated by high-resolution vessel wall imaging and time-of-flight MR angiography: reproducibility, and diagnostic agreement with DSA. Eur Radiol 2021;31:5479-89. [Crossref] [PubMed]
- Ryu CW, Jahng GH, Shin HS. Gadolinium enhancement of atherosclerotic plaque in the middle cerebral artery: relation to symptoms and degree of stenosis. AJNR Am J Neuroradiol 2014;35:2306-10. [Crossref] [PubMed]
- Xu WH, Li ML, Gao S, Ni J, Zhou LX, Yao M, Peng B, Feng F, Jin ZY, Cui LY. In vivo high-resolution MR imaging of symptomatic and asymptomatic middle cerebral artery atherosclerotic stenosis. Atherosclerosis 2010;212:507-11. [Crossref] [PubMed]
- Samuels OB, Joseph GJ, Lynn MJ, Smith HA, Chimowitz MI. A standardized method for measuring intracranial arterial stenosis. AJNR Am J Neuroradiol 2000;21:643-6.
- Wang M, Wu F, Yang Y, Miao H, Fan Z, Ji X, Li D, Guo X, Yang Q. Quantitative assessment of symptomatic intracranial atherosclerosis and lenticulostriate arteries in recent stroke patients using whole-brain high-resolution cardiovascular magnetic resonance imaging. J Cardiovasc Magn Reson 2018;20:35. [Crossref] [PubMed]
- Lu SS, Ge S, Su CQ, Xie J, Mao J, Shi HB, Hong XN. MRI of plaque characteristics and relationship with downstream perfusion and cerebral infarction in patients with symptomatic middle cerebral artery stenosis. J Magn Reson Imaging 2018;48:66-73. [Crossref] [PubMed]
- Xu WH, Li ML, Gao S, Ni J, Yao M, Zhou LX, Peng B, Feng F, Jin ZY, Cui LY. Middle cerebral artery intraplaque hemorrhage: prevalence and clinical relevance. Ann Neurol 2012;71:195-8. [Crossref] [PubMed]
- Yang WQ, Huang B, Liu XT, Liu HJ, Li PJ, Zhu WZ. Reproducibility of high-resolution MRI for the middle cerebral artery plaque at 3T. Eur J Radiol 2014;83:e49-55. [Crossref] [PubMed]
- Qiao Y, Zeiler SR, Mirbagheri S, Leigh R, Urrutia V, Wityk R, Wasserman BA. Intracranial plaque enhancement in patients with cerebrovascular events on high-spatial-resolution MR images. Radiology 2014;271:534-42. [Crossref] [PubMed]
- Debette S, Compter A, Labeyrie MA, Uyttenboogaart M, Metso TM, Majersik JJ, et al. Epidemiology, pathophysiology, diagnosis, and management of intracranial artery dissection. Lancet Neurol 2015;14:640-54. [Crossref] [PubMed]
- Ota H, Takase K, Rikimaru H, Tsuboi M, Yamada T, Sato A, Higano S, Ishibashi T, Takahashi S. Quantitative vascular measurements in arterial occlusive disease. Radiographics 2005;25:1141-58. [Crossref] [PubMed]
- Oshinski JN, Ku DN, Pettigrew RI. Turbulent fluctuation velocity: the most significant determinant of signal loss in stenotic vessels. Magn Reson Med 1995;33:193-9. [Crossref] [PubMed]
- Ibrahim T, Makowski MR, Jankauskas A, Maintz D, Karch M, Schachoff S, Manning WJ, Schömig A, Schwaiger M, Botnar RM. Serial contrast-enhanced cardiac magnetic resonance imaging demonstrates regression of hyperenhancement within the coronary artery wall in patients after acute myocardial infarction. JACC Cardiovasc Imaging 2009;2:580-8. [Crossref] [PubMed]
- Millon A, Boussel L, Brevet M, Mathevet JL, Canet-Soulas E, Mory C, Scoazec JY, Douek P. Clinical and histological significance of gadolinium enhancement in carotid atherosclerotic plaque. Stroke 2012;43:3023-8. [Crossref] [PubMed]
- Migdalski A, Jawien A. New insight into biology, molecular diagnostics and treatment options of unstable carotid atherosclerotic plaque: a narrative review. Ann Transl Med 2021;9:1207. [Crossref] [PubMed]
- Troyer A, Saloner D, Pan XM, Velez P, Rapp JHAssessment of Carotid Stenosis by Comparison with Endarterectomy Plaque Trial Investigators. Major carotid plaque surface irregularities correlate with neurologic symptoms. J Vasc Surg 2002;35:741-7. [Crossref] [PubMed]
- Wang Y, Zhao X, Liu L, Soo YO, Pu Y, Pan Y, Wang Y, Zou X, Leung TW, Cai Y, Bai Q, Wu Y, Wang C, Pan X, Luo B, Wong KSCICAS Study Group. Prevalence and outcomes of symptomatic intracranial large artery stenoses and occlusions in China: the Chinese Intracranial Atherosclerosis (CICAS) Study. Stroke 2014;45:663-9. [Crossref] [PubMed]
- Shi MC, Wang SC, Zhou HW, Xing YQ, Cheng YH, Feng JC, Wu J. Compensatory remodeling in symptomatic middle cerebral artery atherosclerotic stenosis: a high-resolution MRI and microemboli monitoring study. Neurol Res 2012;34:153-8. [Crossref] [PubMed]
- Yang WJ, Abrigo J, Soo YO, Wong S, Wong KS, Leung TW, Chu WC, Chen XY. Regression of Plaque Enhancement Within Symptomatic Middle Cerebral Artery Atherosclerosis: A High-Resolution MRI Study. Front Neurol 2020;11:755. [Crossref] [PubMed]
- Vakil P, Vranic J, Hurley MC, Bernstein RA, Korutz AW, Habib A, Shaibani A, Dehkordi FH, Carroll TJ, Ansari SA. T1 gadolinium enhancement of intracranial atherosclerotic plaques associated with symptomatic ischemic presentations. AJNR Am J Neuroradiol 2013;34:2252-8. [Crossref] [PubMed]
- Dieleman N, Yang W, Abrigo JM, Chu WC, van der Kolk AG, Siero JC, Wong KS, Hendrikse J, Chen XY. Magnetic Resonance Imaging of Plaque Morphology, Burden, and Distribution in Patients With Symptomatic Middle Cerebral Artery Stenosis. Stroke 2016;47:1797-802. [Crossref] [PubMed]
- Wu F, Ma Q, Song H, Guo X, Diniz MA, Song SS, Gonzalez NR, Bi X, Ji X, Li D, Yang Q, Fan Z. WISP Investigators. Differential Features of Culprit Intracranial Atherosclerotic Lesions: A Whole-Brain Vessel Wall Imaging Study in Patients With Acute Ischemic Stroke. J Am Heart Assoc 2018;7:e009705. [Crossref] [PubMed]
- Fakih R, Roa JA, Bathla G, Olalde H, Varon A, Ortega-Gutierrez S, Derdeyn C, Adams HP Jr, Hasan DM, Leira EC, Samaniego EA. Detection and Quantification of Symptomatic Atherosclerotic Plaques With High-Resolution Imaging in Cryptogenic Stroke. Stroke 2020;51:3623-31. [Crossref] [PubMed]
- Tandon V, Senthilvelan S, Sreedharan SE, Kesavadas C, Vt J, Sylaja PN. High-resolution MR vessel wall imaging in determining the stroke aetiology and risk stratification in isolated middle cerebral artery disease. Neuroradiology 2022;64:1569-77. [Crossref] [PubMed]
- Leng X, Wong KS, Liebeskind DS. Evaluating intracranial atherosclerosis rather than intracranial stenosis. Stroke 2014;45:645-51. [Crossref] [PubMed]
- Zhao JJ, Lu Y, Cui JY, Ma LQ, Zhang RP, Xu Z. Characteristics of symptomatic plaque on high-resolution magnetic resonance imaging and its relationship with the occurrence and recurrence of ischemic stroke. Neurol Sci 2021;42:3605-13. [Crossref] [PubMed]