Association between glymphatic dysfunction and neurocognitive decline in patients with frontal lobe epilepsy
Introduction
As the second most prevalent form of focal epilepsy behind temporal lobe epilepsy (TLE), frontal lobe epilepsy (FLE) comprises approximately 20–30% of all epilepsy cases (1). Neurocognitive deficits, a primary comorbidity in epilepsy, have emerged as a significant concern, due to their substantial impact on quality of life in individuals with chronic symptoms (2). Unlike TLE, which has shown notable deficits in memory and language (3), the pattern of neurocognitive deficits in FLE is quite heterogeneous, displaying considerable variability in extant literature (4,5). Multiple cognitive impairments and abnormality in motor-related networks have been frequently observed, although instances of preserved cognitive function have also been documented (4,5). The mechanism underlying the alteration of neurocognitive functions in epilepsy remains to be elucidated. Intrinsic epilepsy factors, such as seizure severity (6), age of onset (7), disease duration (8), and the impact of medications (9), are believed to contribute to the observed dysfunction. Moreover, extrinsic factors may also exert influence, particularly in patients who are newly diagnosed with epilepsy and already exhibit neurocognitive alterations (2).
The glymphatic (glial-lymphatic) system, which functions as a cerebral metabolite waste drainage mechanism, is driven by arterial pulsation and flow, facilitated by the astroglial water channel, aquaporin 4 (AQP4). This system enables the exchange of cerebrospinal fluid (CSF) with interstitial fluid (ISF) and facilitates drainage of extracellular soluble proteins and metabolites through perivascular spaces into cervical lymphatic vessels (10,11). Recent research has underscored the role of the glymphatic system in the elimination of neurotoxic compounds, such as excitatory neurotransmitters and protein aggregates. Interestingly, dysfunction of the glymphatic system may also play a part in the pathogenesis of neurodegenerative diseases (12) and epilepsy (13).
Considering the three-dimensional tubular geometry of perivascular spaces, water molecule diffusion in these spaces has been hypothesized to be anisotropic. This can be non-invasively assessed using diffusion imaging techniques (14). For instance, Taoka et al. (15) introduced the “analysis along the perivascular space” (ALPS) method, employing diffusion tensor imaging (DTI) as a non-invasive tool to evaluate the glymphatic system in humans, with results corroborated by an animal study (16). Various disorders of the central nervous system (CNS), including Alzheimer’s disease (AD) (15), mild cognitive impairment (MCI) (17), vascular cognitive impairment (VCI) (18), Parkinson’s disease (19), and epilepsy (20-24), exhibit lower diffusion tensor imaging analysis along the perivascular space (DTI-ALPS) indices, which have been significantly associated with the cognitive decline observed in these diseases (15,17,18). However, alteration in glymphatic function and its correlation with neurocognitive function in FLE is not well-defined. Our current study delves into differences in the DTI-ALPS index between patients with magnetic resonance imaging (MRI)-negative FLE and healthy controls (HCs), exploring the association between DTI-ALPS and clinical characteristics in FLE, and examining the neurocognitive performance in both groups. We present this article in accordance with the STROBE reporting checklist (available at https://qims.amegroups.com/article/view/10.21037/qims-24-375/rc).
Methods
Participants
The study was conducted in accordance with the Declaration of Helsinki (as revised in 2013), and it was approved by institutional ethics committee of the Third Xiangya Hospital (No. I-22254). Informed consent was obtained from all participants and parents of the participants under 18 years old. From January 2019 to December 2020, 58 consecutive patients of FLE were prospectively included from Department of Neurology, the Third Xiangya Hospital, Central South University, Changsha, which is a major epilepsy center and tertiary hospital in China.
Diagnoses were established by expert neurologists through a thorough evaluation of clinical history, 24-hour video-electroencephalogram (EEG) recordings, ictal semiology, and routine MRI examinations, adhering to International League Against Epilepsy (ILAE) guidelines (25). The patients underwent 24-hour scalp video-EEG recordings (EEG-1200C, Nihon Kohden, Tokyo, Japan), including overnight sessions. The utilized 16 electrodes placed per the 10–20 international standard, with a sampling rate of 256 Hz. All patients were on anti-epileptic drug (AED) therapy and received regular outpatient follow-up. Exclusion criteria included ictogenic structural abnormality on routine cranial MRI examination, prior brain surgery, alcoholic and drug abuse, pregnancy, patients with any psychiatric and/or systemic disease that affects cognitive functions, and primary sensory or motor impairment that could adversely affect the neurocognitive performance. All participants underwent further MRI examination and thorough neurocognitive tests, and those who refused to and/or were unable to finish the examinations were also excluded. Finally, the current study included 25 patients with FLE (age range, 14–45 years; mean age ± standard deviation (SD): 26.28±8.12 years, 10 females), and the flowchart was shown in Figure 1.
A group of 22 HC participants (age range, 17–38 years; average age ± SD: 25.86±6.15 years, 11 females), matched for age, sex, and education, were also recruited. All participants in the HC group had normal routine cranial MRI, without any history of neuro-psychiatric disorders and without alcohol or drug abuse.
Clinical information
Comprehensive clinical details were collected for each patient with FLE, including age at epilepsy onset, illness duration, varieties of epileptic seizures experienced, initial and follow-up EEGs, medication strategies, and the frequency of seizure episodes following treatment commencement. Severity of epilepsy was appraised using the National Hospital Seizure Severity Scale (NHS3) (26), which is a measure incorporating seven seizure-related factors, with a scoring range from 1 to 27. Post-treatment seizure attack frequency for each patient was systematically evaluated based on the criteria established by the ILAE scale (27).
Neuropsychological testing
All participants received a thorough neuropsychological evaluation, either during their hospital stay for seizure characterization or in an outpatient setting. Only the initial neuropsychological assessment was utilized for each patient. The evaluation comprised several tests (28): (I) Forward/Backward Digit Span Test (FDST/BDST), which assessed verbal working memory through two subtests; in the FDST, participants repeated a sequence of digits after hearing them, ending when the maximum sequence length was achieved or after two consecutive errors, while the BDST required reciting the digit sequence in reverse. (II) The Visual Recognition Task evaluated visual-spatial memory by having participants recall eight cards from a set of 28, including various graphics, Chinese characters, and mathematical symbols. (III) The Logical Memory Task measured verbal episodic memory, where participants recounted details from two stories immediately after they were read by the experimenter. (IV) The Trail Making Test (TMT), with Trails A (TMT-A) assessing basic speeded sequencing and Trails B (TMT-B) measuring divided visual attention and cognitive flexibility, involved connecting series of numbers and alternating between numbers and letters, respectively. Performance was scored based on completion time and error count. (V) Stroop Color and Word test (SCWT) assessed cognitive flexibility and the efficiency of the inhibitory process in the frontal lobe by having participants read characters, name colors, and name a color not matching the character as soon as possible, respectively. (VI) The similarity test (ST) examined higher-order executive functions, like abstract thinking and concept formation, requiring participants to identify similarities between pairs of words. Depressive and anxiety symptoms in all participants were assessed using the Beck Depression and Anxiety Inventories, respectively (28). In order to reduce confounding factors, participant with severe intelligence impairment was excluded by a rough estimation of ST and picture completion of Chinese version Wechsler Adult Intelligence Scale (WAIS-RC) (29,30).
Image acquisition
MRI data acquisition was conducted using a 3.0 T MRI scanner (Ingenia, Philips Medical Systems, Netherlands) equipped with a 15-channel receiver array head coil. Participants were instructed to lie still. To enhance image quality, earplugs were provided to reduce scanner noise and foam pads were utilized to limit head movement.
Structural imaging involved a three-dimensional turbo fast echo (3D-TFE) T1WI sequence to capture high-resolution images. The parameters were set as follows: repetition time (TR)/echo time (TE) =9.1/4.5 ms; number of slices =170; slice thickness =1 mm with no gap; flip angle =8°; acquisition matrix =256×256; and field of view (FOV) =240 mm × 240 mm.
For diffusion-weighted imaging, a single-shot gradient echo planar imaging (EPI) sequence was employed with these specific parameters: TR =6,578 ms; TE =85 ms; flip angle =90°; FOV =240 mm × 240 mm; voxel size =2.5×2.5×2.5 mm. The protocol included 32 volumes with noncollinear diffusion directions at a b value of 1,000 s/mm2 and one volume with a b value of 0, encompassing a total of 60 slices.
DTI data preprocessing
Careful evaluation of artifacts in all diffusion-weighted images was initially conducted by K.Z. and L.D., two senior neuroradiologists and experienced analysts, focusing on various aspects, such as total geometric distortion, signal loss, and volume motion. Subsequently, the origin positions of all diffusion-weighted images were adjusted to ensure accuracy. The preprocessing of diffusion-weighted images for all participants was conducted via the FMRIB Software Library (FSL) Package 6.0.1, accessible at (http://www.fmrib.ox.ac.uk/fsl) (31).
During preprocessing, non-brain voxels were removed utilizing the Brain Extraction Tool (BET) within FSL. Further, motion and eddy current corrections were performed using the same software suite. The refined data was then processed through DTI fitting, using FSL’s diffusion toolbox, facilitating the generation of fractional anisotropy (FA) and tensor 4D maps.
These processes enabled the creation of individual diffusivity maps for each participant. Diffusivity maps were generated in three primary directions: the x-axis (right-left, denoted as Dxx), y-axis (anterior-posterior, Dyy), and z-axis (inferior-superior, Dzz), thus providing a comprehensive analysis of diffusion properties across different brain regions.
Total intracranial volume (TIV) was estimated on 3D-TFE T1 data using Statistical Parametric Mapping (SPM)’s three region (gray matter, white matter, and CSF) segmentation, and TIV value was obtained by adding up the volume of gray matter, white matter, and CSF.
ALPS-index calculation
The ALPS index, which is indicative of glymphatic function, is based on water diffusion dynamics in white matter near the lateral ventricle body, primarily from perivascular spaces of medullary veins and white matter fiber tracts (e.g., projection and association fibers), as shown in Figure 2. The perpendicular orientation of these medullary veins to both the lateral ventricle wall and fiber tract enables precise analysis of perivascular water diffusivity.
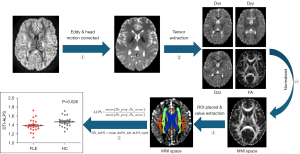
Adhering to methodologies established by Zhang et al. (17) and Song et al. (18), the DTI-ALPS index was calculated. Diffusivity maps in the x- (Dx), y- (Dy), and z-axis (Dz) were obtained for each subject using FSL, and co-plotted to the standard FA map template in the ICBM DTI-81 Atlas. Given the consistent orientation of medullary veins, region of interest (ROI) placement relied solely on DTI images. Four 5 mm-diameter spherical ROIs were placed on projection and association fibers in the uppermost layer of the lateral ventricle body. ROIs were manually adjusted as necessary to ensure accuracy and avoid non-specific lesions. The ALPS-index, representing the ratio of perivascular space water diffusivity (Dx_projc, Dx_assoc) to diffusivity in non-aligned directions (Dy_proj, Dz_assoc), quantifies glymphatic function.
The bilateral ALPS-index average served as a composite measure, representing the overall glymphatic function across subjects.
Statistical analyses
SPSS Statistics version 23.0 (IBM Corporation) was used to analyze data. Quantitative variables were expressed as mean ± standard deviation. Categorical variables were expressed as percentages [n (%)]. In order to measure inter-group difference, two-sample t-tests, Mann-Whitney U tests, and Fisher’s/chi-square tests were used for normally distributed quantitative, non-normally distributed quantitative, and categorical variables, respectively. False discovery rate (FDR) correction was performed for between-group two-tailed comparison of neurocognitive test results. Pearson’s and Spearman’s correlations were used to assess variable relationships for normally- and non-normally distributed variables respectively. A linear regression model with forward selection was used to evaluate associations with multiple cognition variables, including those significant in univariate logistic regression (P<0.1) or otherwise clinically relevant. Variance inflation factors (VIF) confirmed no multicollinearity (VIF <3). Statistical significance was set at P<0.05.
Results
Clinical characteristics and neurocognitive test results
Table 1 presents a detailed demonstration of the demographic information and neurocognitive test outcomes for all participants. The mean disease duration among the enrolled patients was recorded at 6.24±5.89 years, with a predominant manifestation of bilateral scalp EEG anomalies. When examining the neurocognitive performance disparities between the groups, it was noted that the scores in both the ST and FDST were significantly lower in the FLE group. Comparing to HC group, time to complete TMT-B was significantly longer with slightly but significantly more errors Moreover, the FLE group exhibited a substantial increase in the average completion time for the SCWT, although there was no marked difference in accuracy between the two groups. Furthermore, analysis revealed no significant divergence in levels of anxiety, depression, visual recognition abilities, or performance on the TMT-A between patients with FLE and HCs.
Table 1
Clinical characteristics | FLE patients (n=25) | HC (n=22) | P value |
---|---|---|---|
Age at examination/year, mean ± SD | 26.28±8.12 | 25.86±6.15 | 0.853 |
Sex: male, N [%] | 15 [60] | 11 [50] | 0.487 |
Education (year), mean ± SD | 12.72±2.70 | 14.23±2.94 | 0.074 |
Disease duration (year), mean ± SD | 6.24±5.89 | ||
Localization (left/right/bilateral/unknown), n | 3/6/16/0 | ||
Seizure frequency (grade 0/1/2/3), n | 6/12/0/7 | ||
Medication (mono/multi-medication), n | 20/5 | ||
Neurocognitive test†, mean ± SD | |||
TMT-A time (sec) | 41.24±16.06 | 39.50±17.85 | 0.283 |
TMT-A error | 0.12±0.33 | 0.09±0.43 | 0.268 |
TMT-B time (sec) | 106.48±34.52 | 82.91±27.82 | 0.035* |
TMT-B error | 0.80±1.38 | 0.14±0.35 | 0.028* |
SCWT average time (sec) | 49.69±20.37 | 37.59±10.78 | 0.027* |
SCWT average error | 1.48±2.81 | 1.00±2.14 | 0.057 |
FDST | 8.20±1.55 | 9.36±1.05 | 0.025* |
BDST | 5.48±1.74 | 6.23±1.69 | 0.080 |
ST | 9.60±2.96 | 11.50±2.50 | 0.029* |
Visual recognition | 9.96±2.85 | 11.18±3.19 | 0.089 |
Logical memory task | 8.54±2.82 | 9.91±2.53 | 0.056 |
Beck anxiety inventory | 30.12±7.75 | 29.02±8.02 | 0.271 |
Beck depression inventory | 9.16±5.84 | 6.68±7.49 | 0.096 |
*, P<0.05 was considered statistically significant; †, for between-group comparison of neurocognitive test results, FDR correction was performed. FLE, frontal lobe epilepsy; HC, healthy control; SD, standard deviation; TMT-A, Trail Making Test A; TMT-B, Trail Making Test B; SCWT, Stroop Color Word Test; FDST, Forward Digit Span Test; BDST, Backward Digit Span Test; ST, similarity test; FDR, false discovery rate.
Difference in DTI-ALPS index between FLE and HC groups
A statistically significant difference was evident with the DTI-ALPS index averaging 1.387±0.127 in the FLE group, in contrast to 1.468±0.114 in HCs (P=0.026). The 95% confidence interval for this disparity ranged from 0.010 to 0.151, with the test statistic t-value being 2.299, as illustrated in Figure 3. In addition, TIV was not significantly different between the two groups (mean ± SD: 1,493±138 mL in FLE vs. 1,481±164 mL in HCs group, two-tailed P=0.79).
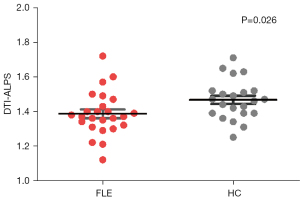
Association of DTI-ALPS with clinical and neurocognitive results
DTI-ALPS index demonstrated a significant correlation with disease duration in the FLE group (r=−0.415, P=0.039), as depicted in Figure 4A. Furthermore, this index was also significantly associated with performance in the ST (r=0.395, P=0.006) and FDST (r=0.399, P=0.005), across both groups, as illustrated in Figure 4B,4C. In the multivariate analysis, after adjusting for age, sex, education level, total cerebral volume, and disease duration, the DTI-ALPS index was found to be significantly related to the FDST and ST scores in both groups, as detailed in Table 2.
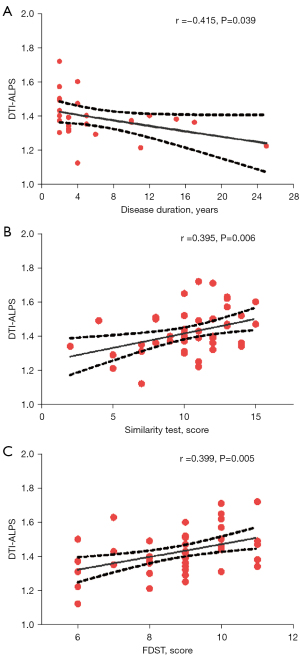
Table 2
DTI-ALPS | Model 1 | Model 2 | Model 3 | ||||||||
---|---|---|---|---|---|---|---|---|---|---|---|
R2 | Beta | P | R2 | Beta | P | R2 | Beta | P | |||
ST | 0.148 | 0.385 | 0.008 | 0.362 | 0.279 | 0.042 | 0.276 | 0.525 | 0.007 | ||
FDST | 0.186 | 0.431 | 0.003 | 0.270 | 0.285 | 0.044 | 0.254 | 0.534 | 0.006 |
Model 1, unadjusted; Model 2, adjusted for age, sex, education, TIV; Model 3, adjusted for age, sex, education. DTI-ALPS, diffusion tensor imaging analysis along the perivascular space; ST, similarity test; FDST, Forward Digit Span Test; TIV, total intercranial volume.
Discussion
The current study investigated differences in glymphatic function, as indicated by the DTI-ALPS index, and cognitive capabilities between patients with FLE and a group of age-, sex-, and education-matched HCs. Our findings revealed that the FLE group exhibited a significantly reduced ALPS-index and lower scores in tests of neurocognitive function when compared to the HC group. Additionally, the ALPS-index showed a significant correlation with the results of neurocognitive tests in both groups, particularly with FDST and ST scores. These results suggest that the functionality of the glymphatic system may be a contributing factor to cognitive dysfunction in patients with FLE.
Compared to tracer methodologies, the DTI-ALPS technique, developed by Taoka et al. (15), enables a non-invasive examination of glymphatic clearance function without the need for contrast administration. This method has gained widespread use for the study of diverse neurological disorders (17-23). However, findings regarding glymphatic function impairment in epilepsy, as indicated by the ALPS-index, have been inconsistent. A decrease in ALPS has been observed in generalized (20) and focal (22) epilepsy, as well as status epilepticus (23), while improvements have been observed in surgically treated individuals with TLE and a favorable prognosis (21). Conversely, some study has reported no significant differences in ALPS metrics (24) in newly diagnosed focal epilepsy compared to HCs. In our study, a markedly lower ALPS was observed in patients with FLE, and ALPS index scores were significantly correlated with disease duration, but not with attack frequency or NHS3 score. This discrepancy in findings could be attributed to variations in patient selection, suggesting that disease duration in epilepsy may contribute to glymphatic impairment.
Our study also identified a mild, yet significant decline in both executive function and memory in FLE patients, as shown by significantly lower FDST and ST scores, along with higher duration and error counts in the TMT-B test in the FLE group. Consistent with previous studies (4,5,32,33), these outcomes indicate that FLE patients may experience impairments across multiple neurocognitive domains, including basic and complex attention, working memory, semantic memory, and executive function. However, we found that the ALPS index was significantly associated only with FDST and ST scores in both FLE and HC groups. The DST assesses aspects of working memory maintenance, while the ST is an effective measure of memory performance, possibly due to its semantic encoding strategy, which enhances memory retention compared to simpler repetition strategies (34). Both tests are also effective in evaluating attention, which is closely linked to memory performance. Hence, we speculate that a decrease in the DTI-ALPS index may contribute to memory impairments in FLE.
Memory impairment has been observed in both pediatric and adult patients with FLE (32,33), ranging from mild encoding and retrieval difficulties in working memory, to more significant long-term memory issues; however, these memory difficulties have been observed less consistently in individuals with FLE than in individuals with TLE. Memory-task functional magnetic resonance imaging (fMRI) studies (35,36) have also revealed divergent activation patterns in patients with FLE, suggesting memory reliance on a network encompassing both the temporal and frontal lobes, rather than confined to specific brain structures, such as the medial temporal lobes. However, the heterogeneity in memory impairment patterns implies additional mechanisms beyond seizure propagation from the focal seizure onset area(s) and/or remote functional deficit zone(s). Given that short-term memories necessitate increased pre-synaptic glutamate release and changes in postsynaptic glutamatergic receptor activity (37,38), mediated by covalent modifications of existing proteins at preexisting synapses (39,40), glymphatic dysfunction could interfere with neurotransmitter clearance and associated neurocognition processes. In addition, neurocognitive adverse events related to anti-seizure medication (ASM) was also reported (41), and epilepsy duration and ASM administration duration may be the major risk factor (42). Thus, we postulate that glymphatic clearance system may also play a role in maintaining neurocognitive function in patients with FLE, but this certainly warrants further research for validation.
DTI-ALPS calculation is based on FA values. Previous studies (43,44) have shown that a decrease in FA in frontal and extra-frontal regions is significantly associated with cognitive alterations. Combined with our findings, it is plausible that a decrease in FA may indicate impairment in both white matter fiber integrity and in the micro-environment of the interstitial space. However, since the periventricular region of the glymphatic system, measured by DTI-ALPS, is predominantly located in the frontal lobe, it remains unclear whether DTI-ALPS is more susceptible to changes in frontal lobe function and whether glymphatic system function in other regions would also be associated. Additionally, the potential impact of sampling bias cannot be excluded, as the majority of participants in our study exhibited bilateral EEG abnormalities, suggesting either more extensive involvement of epileptogenic foci, or a greater propensity for bilateral propagation of epileptiform discharges.
The current study possesses some limitations that warrant discussion. First, the relatively small sample size and the cross-sectional nature of the data do not allow for the establishment of a causal relationship between lower DTI-ALPS and cognitive decline in FLE. This aspect may be more effectively explored in future studies. Additionally, as all participants were drawn from the same region within Changsha, China, the potential for sampling bias necessitates caution when generalizing the current findings. Second, while DTI-ALPS effectively isolates perivascular glymphatic diffusivity, the measurement is predominantly based on diffusion alterations parallel to the x-axis in frontoparietal region. It does not directly reflect glymphatic flow along other axes or glymphatic alterations in other brain regions, such as the nigrostriatal pathway. Therefore, future research with a larger sample size and multimodal assessment approaches should be conducted in epilepsies of other pathologies. Specifically, advances in MRI technology may eventually enable noninvasive glymphatic measurements across various anatomical structures, further enriching our understanding of this complex system.
Conclusions
Results of the current investigation revealed that the functionality of the glymphatic system may be compromised in individuals diagnosed with FLE, as evidenced by alterations in the DTI-ALPS index. Furthermore, a substantial correlation was established between DTI-ALPS index and diminished neurocognitive function. This finding suggests that glymphatic clearance mechanisms may be integral to the understanding of neurocognitive impairments associated with epilepsy. Consequently, the DTI-ALPS index is beginning to emerge as a pivotal biomarker, potentially playing a crucial role in the future exploration and understanding of the interplay between epilepsy and neurocognitive function.
Acknowledgments
Funding: This work was supported by
Footnote
Reporting Checklist: The authors have completed the STROBE reporting checklist. Available at https://qims.amegroups.com/article/view/10.21037/qims-24-375/rc
Conflicts of Interest: All authors have completed the ICMJE uniform disclosure form (available at https://qims.amegroups.com/article/view/10.21037/qims-24-375/coif). The authors have no conflicts of interest to declare.
Ethical Statement: The authors are accountable for all aspects of the work in ensuring that questions related to the accuracy or integrity of any part of the work are appropriately investigated and resolved. The study was conducted in accordance with the Declaration of Helsinki (as revised in 2013). The study was approved by institutional ethics committee of the Third Xiangya Hospital (No. I-22254) and informed consent was taken from all participants and parents of the participants under 18 years old.
Open Access Statement: This is an Open Access article distributed in accordance with the Creative Commons Attribution-NonCommercial-NoDerivs 4.0 International License (CC BY-NC-ND 4.0), which permits the non-commercial replication and distribution of the article with the strict proviso that no changes or edits are made and the original work is properly cited (including links to both the formal publication through the relevant DOI and the license). See: https://creativecommons.org/licenses/by-nc-nd/4.0/.
References
- Manford M, Fish DR, Shorvon SD. An analysis of clinical seizure patterns and their localizing value in frontal and temporal lobe epilepsies. Brain 1996;119:17-40. [Crossref] [PubMed]
- Vlooswijk MC, Jansen JF, de Krom MC, Majoie HM, Hofman PA, Backes WH, Aldenkamp AP. Functional MRI in chronic epilepsy: associations with cognitive impairment. Lancet Neurol 2010;9:1018-27. [Crossref] [PubMed]
- Allone C, Lo Buono V, Corallo F, Pisani LR, Pollicino P, Bramanti P, Marino S. Neuroimaging and cognitive functions in temporal lobe epilepsy: A review of the literature. J Neurol Sci 2017;381:7-15. [Crossref] [PubMed]
- Patrikelis P, Angelakis E, Gatzonis S. Neurocognitive and behavioral functioning in frontal lobe epilepsy: a review. Epilepsy Behav 2009;14:19-26. [Crossref] [PubMed]
- Arrotta K, Reyes A, Kaestner E, McDonald CR, Hermann BP, Barr WB, Sarmey N, Sundar S, Kondylis E, Najm I, Bingaman W, Busch RM. Cognitive phenotypes in frontal lobe epilepsy. Epilepsia 2022;63:1671-81. [Crossref] [PubMed]
- Dodrill CB. Progressive cognitive decline in adolescents and adults with epilepsy. Prog Brain Res 2002;135:399-407. [Crossref] [PubMed]
- Hermann B, Seidenberg M, Bell B, Rutecki P, Sheth R, Ruggles K, Wendt G, O'Leary D, Magnotta V. The neurodevelopmental impact of childhood-onset temporal lobe epilepsy on brain structure and function. Epilepsia 2002;43:1062-71. [Crossref] [PubMed]
- Jokeit H, Ebner A. Effects of chronic epilepsy on intellectual functions. Prog Brain Res 2002;135:455-63. [Crossref] [PubMed]
- Vermeulen J, Aldenkamp AP. Cognitive side-effects of chronic antiepileptic drug treatment: a review of 25 years of research. Epilepsy Res 1995;22:65-95. [Crossref] [PubMed]
- Tarasoff-Conway JM, Carare RO, Osorio RS, Glodzik L, Butler T, Fieremans E, Axel L, Rusinek H, Nicholson C, Zlokovic BV, Frangione B, Blennow K, Ménard J, Zetterberg H, Wisniewski T, de Leon MJ. Clearance systems in the brain-implications for Alzheimer disease. Nat Rev Neurol 2015;11:457-70. Erratum in: Nat Rev Neurol 2016;12:248. [Crossref] [PubMed]
- Rasmussen MK, Mestre H, Nedergaard M. The glymphatic pathway in neurological disorders. Lancet Neurol 2018;17:1016-24. [Crossref] [PubMed]
- Nedergaard M, Goldman SA. Glymphatic failure as a final common pathway to dementia. Science 2020;370:50-6. [Crossref] [PubMed]
- Christensen J, Yamakawa GR, Shultz SR, Mychasiuk R. Is the glymphatic system the missing link between sleep impairments and neurological disorders? Examining the implications and uncertainties. Prog Neurobiol 2021;198:101917. [Crossref] [PubMed]
- Sepehrband F, Cabeen RP, Choupan J, Barisano G, Law M, Toga AWAlzheimer's Disease Neuroimaging Initiative. Perivascular space fluid contributes to diffusion tensor imaging changes in white matter. Neuroimage 2019;197:243-54. [Crossref] [PubMed]
- Taoka T, Masutani Y, Kawai H, Nakane T, Matsuoka K, Yasuno F, Kishimoto T, Naganawa S. Evaluation of glymphatic system activity with the diffusion MR technique: diffusion tensor image analysis along the perivascular space (DTI-ALPS) in Alzheimer's disease cases. Jpn J Radiol 2017;35:172-8. [Crossref] [PubMed]
- Harrison IF, Siow B, Akilo AB, Evans PG, Ismail O, Ohene Y, Nahavandi P, Thomas DL, Lythgoe MF, Wells JA. Non-invasive imaging of CSF-mediated brain clearance pathways via assessment of perivascular fluid movement with diffusion tensor MRI. Elife 2018;7:e34028. [Crossref] [PubMed]
- Zhang W, Zhou Y, Wang J, Gong X, Chen Z, Zhang X, Cai J, Chen S, Fang L, Sun J, Lou M. Glymphatic clearance function in patients with cerebral small vessel disease. Neuroimage 2021;238:118257. [Crossref] [PubMed]
- Song H, Ruan Z, Gao L, Lv D, Sun D, Li Z, Zhang R, Zhou X, Xu H, Zhang J. Structural network efficiency mediates the association between glymphatic function and cognition in mild VCI: a DTI-ALPS study. Front Aging Neurosci 2022;14:974114. [Crossref] [PubMed]
- Cai X, Chen Z, He C, Zhang P, Nie K, Qiu Y, Wang L, Wang L, Jing P, Zhang Y. Diffusion along perivascular spaces provides evidence interlinking compromised glymphatic function with aging in Parkinson's disease. CNS Neurosci Ther 2023;29:111-21. [Crossref] [PubMed]
- Lee HJ, Lee DA, Shin KJ, Park KM. Glymphatic system dysfunction in patients with juvenile myoclonic epilepsy. J Neurol 2022;269:2133-9. [Crossref] [PubMed]
- Zhang C, Xu K, Zhang H, Sha J, Yang H, Zhao H, Chen N, Li K. Recovery of glymphatic system function in patients with temporal lobe epilepsy after surgery. Eur Radiol 2023;33:6116-23. [Crossref] [PubMed]
- Kim J, Lee DA, Lee HJ, Park KM. Glymphatic system dysfunction in patients with occipital lobe epilepsy. J Neuroimaging 2023;33:455-61. [Crossref] [PubMed]
- Lee DA, Lee J, Park KM. Glymphatic system impairment in patients with status epilepticus. Neuroradiology 2022;64:2335-42. [Crossref] [PubMed]
- Lee DA, Park BS, Ko J, Park SH, Park JH, Kim IH, Lee YJ, Park KM. Glymphatic system function in patients with newly diagnosed focal epilepsy. Brain Behav 2022;12:e2504. [Crossref] [PubMed]
- Engel J JrInternational League Against Epilepsy (ILAE). A proposed diagnostic scheme for people with epileptic seizures and with epilepsy: report of the ILAE Task Force on Classification and Terminology. Epilepsia 2001;42:796-803. [Crossref] [PubMed]
- O'Donoghue MF, Duncan JS, Sander JW. The National Hospital Seizure Severity Scale: a further development of the Chalfont Seizure Severity Scale. Epilepsia 1996;37:563-71. [Crossref] [PubMed]
- Wieser HG, Blume WT, Fish D, Goldensohn E, Hufnagel A, King D, Sperling MR, Lüders H, Pedley TACommission on Neurosurgery of the International League Against Epilepsy (ILAE). ILAE Commission Report. Proposal for a new classification of outcome with respect to epileptic seizures following epilepsy surgery. Epilepsia 2001;42:282-6.
- Daffner KR, Gale SA, Barrett AM, Boeve BF, Chatterjee A, Coslett HB, D'Esposito M, Finney GR, Gitelman DR, Hart JJ Jr, Lerner AJ, Meador KJ, Pietras AC, Voeller KS, Kaufer DI. Improving clinical cognitive testing: report of the AAN Behavioral Neurology Section Workgroup. Neurology 2015;85:910-8. [Crossref] [PubMed]
- Schoenberg MR, Scott JG, Duff K, Adams RL. Estimation of WAIS-III intelligence from combined performance and demographic variables: development of the OPIE-3. Clin Neuropsychol 2002;16:426-37. [Crossref] [PubMed]
- Ni C. Comparison of clinical application between full-scale and simplified version of Wechsler Adult Intelligence Scale. Zhong Guo Xing Wei Yi Xue Ke Xue 2005;14:1.
- Smith SM, Jenkinson M, Woolrich MW, Beckmann CF, Behrens TE, Johansen-Berg H, Bannister PR, De Luca M, Drobnjak I, Flitney DE, Niazy RK, Saunders J, Vickers J, Zhang Y, De Stefano N, Brady JM, Matthews PM. Advances in functional and structural MR image analysis and implementation as FSL. Neuroimage 2004;23:S208-19. [Crossref] [PubMed]
- Exner C, Boucsein K, Lange C, Winter H, Weniger G, Steinhoff BJ, Irle E. Neuropsychological performance in frontal lobe epilepsy. Seizure 2002;11:20-32. [Crossref] [PubMed]
- Nolan MA, Redoblado MA, Lah S, Sabaz M, Lawson JA, Cunningham AM, Bleasel AF, Bye AM. Memory function in childhood epilepsy syndromes. J Paediatr Child Health 2004;40:20-7. [Crossref] [PubMed]
- Spencer RJ, Reckow J, Drag LL, Bieliauskas LA. Incidental Learning: A Brief, Valid Measure of Memory Based on the WAIS-IV Vocabulary and Similarities Subtests. Cogn Behav Neurol 2016;29:206-11. [Crossref] [PubMed]
- Centeno M, Vollmar C, O'Muircheartaigh J, Stretton J, Bonelli SB, Symms MR, Barker GJ, Kumari V, Thompson PJ, Duncan JS, Richardson MP, Koepp MJ. Memory in frontal lobe epilepsy: an fMRI study. Epilepsia 2012;53:1756-64. [Crossref] [PubMed]
- Caciagli L, Paquola C, He X, Vollmar C, Centeno M, Wandschneider B, Braun U, Trimmel K, Vos SB, Sidhu MK, Thompson PJ, Baxendale S, Winston GP, Duncan JS, Bassett DS, Koepp MJ, Bernhardt BC. Disorganization of language and working memory systems in frontal versus temporal lobe epilepsy. Brain 2023;146:935-53. [Crossref] [PubMed]
- Shuster MJ, Camardo JS, Siegelbaum SA, Kandel ER. Cyclic AMP-dependent protein kinase closes the serotonin-sensitive K+ channels of Aplysia sensory neurones in cell-free membrane patches. Nature 1985;313:392-5. [Crossref] [PubMed]
- Siegelbaum SA, Camardo JS, Kandel ER. Serotonin and cyclic AMP close single K+ channels in Aplysia sensory neurones. Nature 1982;299:413-7. [Crossref] [PubMed]
- Siow TY, Toh CH, Hsu JL, Liu GH, Lee SH, Chen NH, Fu CJ, Castillo M, Fang JT. Association of Sleep, Neuropsychological Performance, and Gray Matter Volume With Glymphatic Function in Community-Dwelling Older Adults. Neurology 2022;98:e829-38. [Crossref] [PubMed]
- Kandel ER. The molecular biology of memory storage: a dialogue between genes and synapses. Science 2001;294:1030-8. [Crossref] [PubMed]
- Sarkis RA, Goksen Y, Mu Y, Rosner B, Lee JW. Cognitive and fatigue side effects of anti-epileptic drugs: an analysis of phase III add-on trials. J Neurol 2018;265:2137-42. [Crossref] [PubMed]
- Ogunjimi L, Alabi A, Aderinola A, Ogunjimi O, Oladunjoye Y, Kasali A, Kasumu E, Oyenuga I, Akinbodu S, Onipede M, Dele O, Adeyemo S, Osalusi B. Predictors and associated factors with adverse drug reaction in people with epilepsy. Epilepsy Behav 2024;150:109566. [Crossref] [PubMed]
- Widjaja E, Skocic J, Go C, Snead OC, Mabbott D, Smith ML. Abnormal white matter correlates with neuropsychological impairment in children with localization-related epilepsy. Epilepsia 2013;54:1065-73. [Crossref] [PubMed]
- Braakman HM, Vaessen MJ, Jansen JF, Debeij-van Hall MH, de Louw A, Hofman PA, Vles JS, Aldenkamp AP, Backes WH. Pediatric frontal lobe epilepsy: white matter abnormalities and cognitive impairment. Acta Neurol Scand 2014;129:252-62. [Crossref] [PubMed]