Muscle fat infiltration: a narrative review of the magnetic resonance (MR)-based evaluation methods and their clinical applications
Introduction
Muscle fat infiltration (MFI) is known to occur with the normal process of aging or in the presence of specific pathologies (1,2). These pathologies may be of varied origins, including neuromuscular, endocrinological, or musculoskeletal etiologies (3-5).
Two main processes have been identified that lead to the accumulation of fat in muscle tissue. The first process includes a direct route of accumulation of fat in myocytes, known as intra-muscular fat infiltration. This was shown to lead to a decrease in insulin sensitivity, increased inflammation, and decreased functional ability of the muscle tissue (6,7). The second process happens via the accumulation of adipocytes within the muscle fibers, also known as inter-muscular fat infiltration. This process might be caused by different conditions, such as muscle damage or glucocorticoid treatment. Both may lead to the differentiation of specific stem cells within the muscle, known as fibro/adipogenic progenitors, into adipocytes (8,9).
In both research and clinical settings, fat infiltration in skeletal muscle is mainly evaluated via medical imaging, such as magnetic resonance imaging (MRI), computed tomography (CT-scan), and ultrasonography (US). MRI represents the most efficient imaging modality in visualizing the soft tissues, especially the muscle, compared to CT-scans and US (10), while US allows dynamic assessment of the muscles. Furthermore, MRI is a non-irradiating imaging modality, unlike CT-scans, and can better visualize both superficial and deep muscles than US.
Several qualitative and quantitative methods have been implemented for the assessment of the degree of MFI. Qualitative grading methods have been used in the literature, such as the Goutallier classification which is mainly applied to assess the MFI of the rotator cuff (11), the Mercuri Classification in neuromuscular disorders (12), the Kjaer classification in paraspinal muscles (13,14), or the Bal-Lowe and Quartile classifications for hip muscles (15,16). Quantitative methods, such as pixel intensity-based thresholding, mean pixel-intensity or the Dixon technique, are used to better evaluate and quantify MFI.
The quantification of MFI was shown to be indicative of both the progression of disease and the response to treatment in different pathologies. For instance, a higher degree of fat infiltration has been shown to be associated with more severe symptoms in neuromuscular diseases, a worse clinical and functional status in neuromuscular disorders, and a higher risk of post-surgery complications during both the correction of spinal deformities and total hip replacement (16-22).
This review aimed to provide an overview of the different MRI applications for MFI assessment, their clinical relevance, and the techniques used for these evaluations. We present this article in accordance with the Narrative Review reporting checklist (available at https://qims.amegroups.com/article/view/10.21037/qims-24-177/rc).
Methods
Search strategy
The literature search was performed on the PubMed database for articles published between 2010 and end of January 2023. The keywords used were: (Fat infiltration of the muscle) AND [magnetic resonance imaging (MRI)].
Study selection
Articles were included if they were written in English, were full text, on human species, and included fat infiltration assessment of skeletal muscles regardless of the disease and system or muscle groups. Cross-sectional, retrospective, prospective and randomized controlled trials were included. Excluded studies were abstracts, reviews, systematic reviews, case studies, in vitro or animal studies, and articles not related to the research topic.
Study data extraction
For each included study, the main characteristics were extracted including the MFI evaluation method, the target pathology, the muscle(s) of interest, the size of the cohort, the software used (if applicable), the MRI sequences, and the magnet strength. The strategy of the narrative review is displayed in Table 1.
Table 1
Items | Specification |
---|---|
Date of search | 2nd of February 2023 |
Databases and other sources searched | PubMed |
Search terms used | (Fat infiltration of the muscle) and magnetic resonance imaging (MRI) |
Timeframe | 01 January 2010–31 January 2023 |
Inclusion and exclusion criteria | Inclusion criteria: written in English, full text, on human species, and included fat infiltration assessment of skeletal muscles regardless of the disease and system or muscle groups. Cross-sectional, retrospective, prospective and randomized controlled trials were included |
Exclusion criteria: abstracts, reviews, systematic reviews, case studies, in vitro or animal studies, and articles not related to the research topic | |
Selection process | Articles selection were conducted independently by two co-authors and consensus was obtained through consisted patterns |
Any additional considerations, if applicable | The following main characteristics were extracted from each selected article: muscle fat infiltration evaluation method, the target pathology, the muscle(s) of interest, the size of the cohort, the software used (if applicable), the MRI sequences, and the magnet strength |
Results
Literature search
Two researchers conducted an independent screening of the literature, based on the aforementioned inclusion and exclusion criteria. 615 publications were found on PubMed dated between January 2010 and January 2023. After applying the exclusion criteria, 471 articles were pre-selected. The two researchers independently underwent a screening of all titles and abstracts, then a detailed reading of full articles, effectively narrowing the literature down to a total of 159 papers. The detailed results of MFI evaluation methods and the associated conditions are presented hereafter. The search methodology was summarized in a flow chart (Figure 1) and a detailed table was added in https://cdn.amegroups.cn/static/public/qims-24-177-1.xlsx.
MFI evaluation methods
The 159 selected papers involved MFI evaluation methods that could be classified as qualitative or quantitative (Figure 2). Within the 159 papers, some of them included more than one method, resulting in 168 studies.
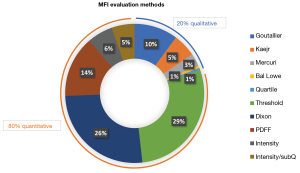
Qualitative methods (33 studies)
Goutallier classification (17 studies)
The Goutallier classification includes five grades. Grade 0: absence of fat infiltration in the muscle tissue; grade 1: some fat streaks in the muscle tissue; grade 2: lean muscle tissue exceeding the fat tissue; grade 3: lean muscle tissue equal to fat tissue; grade 4: fat deposits in the muscle tissue exceeding the lean muscle mass (Figure 3) (23).
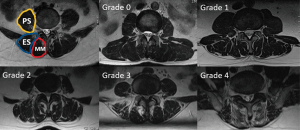
This classification was created to assess the MFI in rotator cuff pathologies using CT-scans (11). It was later modified for use in MRI studies and extended to other muscles, especially in the spine. The Goutallier classification is applied on T2 sequences.
Kjaer classification (9 studies)
The Kjaer classification is mainly used for the evaluation of the paraspinal muscles, especially the multifidus muscle, and includes three grades (Figure 4) (13,14,24). Grade 0: muscle with fat infiltration less than 10%; grade 1: fat infiltration between 10% and 50%; grade 2: muscle with fat infiltration more than 50%.
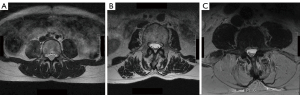
Mercuri grading scale (5 studies)
The Mercuri classification is mainly used for the assessment of thigh muscles in neuromuscular diseases and categorizes MFI into four grades (12). Grade 1: muscles with a normal appearance; grade 2: muscles with mild fatty infiltration (less than 30% of the volume of the original muscles); grade 3: muscles with moderate fatty infiltration (30% to 60% of the volume of the original muscles); grade 4: muscles with severe fatty infiltration (greater than 60% of the volume of the original muscles).
Bal Lowe classification (1 study)
The Bal Lowe classification aims to evaluate hip muscles after minimally invasive total hip arthroplasty (THA) and includes four grades (15,16). Grade 0: muscle with a normal appearance; grade 1: muscle with less than 30% of fatty infiltration; grade 2: muscle with fatty infiltration between 30% and 70%; grade 3: muscle with more than 70% of fatty infiltration.
Quartile classification (1 study)
The Quartile classification was first used to assess fat infiltration in the hip muscles and includes four grades. Grade 1: MFI less than 25%; grade 2: MFI between 25% and 50%; grade 3: MFI between >50% and 75%, grade 4: MFI more than 75% (25).
Table 2 displays the different qualitative methods used to evaluate MFI.
Table 2
Grade | Goutallier classification | Mercuri classification | Kjaer classification | Bal-Lowe classification | Quartile classification |
---|---|---|---|---|---|
0 | Normal appearance | N/A | N/A | Normal appearance | Normal appearance |
1 | Muscle contains some fatty streaks | Normal appearance | <10% MFI | <30% MFI | <25% MFI |
2 | Fatty infiltration, but still more muscle than fat | <30% MFI | 10–50% MFI | 30–70% MFI | 25–50% MFI |
3 | Equal amounts of muscle and fat | 30–60% MFI | >50% MFI | >70% MFI | >50–75% MFI |
4 | More fat than muscle | >60% MFI | N/A | N/A | >75% MFI |
MFI, muscle fat infiltration; N/A, not applicable.
Within the 168 studies, these 5 qualitative MIF classifications accounted for 20% of all MIF evaluation methods, with the Goutallier classification being the most represented.
Validity and reliability studies on these techniques were scarcely evaluated in the screened literature. Klemt et al. reported that the Bal Lowe, Goutallier and Quartile classifications demonstrated good reproducibility in assessing hip muscles with better results for the Quartile classification (16).
Quantitative methods (135 studies)
All the quantitative methods described hereafter involve the segmentation of the muscles of interest and the computation of the pixel intensity histogram.
Dixon method (68 studies)
Unlike other fat suppression methods, the Dixon acquisition principle takes advantage of a convenient fat and water chemical shift to generate fat-only and water-only images. Sequence terminology depends on the manufacturer: IDEAL (GE Healthcare, Waukesha, MI, USA); Dixon (Siemens Healthcare, Erlangen, Germany); mDIXON (Philips Medical Systems, Best, the Netherlands); and FatSep (Hitachi, Tokyo, Japan) (26,27).
Since the invention of this technique, numerous variations have been proposed to improve the quantification of the water and fat components.
The original 2-point Dixon (2PD) depends on two image acquisitions [in T1, T2, proton density weighted; spin echo (SE) or fast spin echo (FSE)] at two different echo times (TEs), where the water and fat magnetizations are in-phase and out-of-phase, respectively. Then, the water-only image is obtained by the sum of the in-phase and out-of-phase images, while the fat-only image is obtained by subtracting the out-of-phase from the in-phase images (26). This formulation is prone to misquantification caused by B0 inhomogeneities and ignores additional image weighting (T2* relaxation, diffusion, and flow) and other phase shift biases (eddy currents and B1 receive-field non-uniformity) (28).
To overcome 2PD sequence drawbacks, the original technique was modified to involve a third TE point to sample a second out-of-phase state. In the so-called three-point Dixon (3PD), B0 inhomogeneity can be removed using the two out-of-phase acquisitions for estimating the phase error. A generalized 3-point method was proposed in the late 90s, showing that pure in-phase and out-of-phase imaging was not required when 3 echoes were acquired (29). The 3PD technology provides more effective fat quantification, resulting in a better signal-to-noise ratio (SNR) and optimal spatial resolution. The fat fraction (FF) image is calculated as follows: IFF = If/ (If + Iw) × 100, where If, Iw, and IFF are the fat, water, and FF images, respectively.
The last two decades produced significant improvements due to advanced chemical shift imaging processes enabling the correction of some major confounding factors. First, the independence of T1 relaxation between fat and water can be avoided with very low flip angle values, less than 6 degrees (30). Second, the effect of T2*-related signal loss is corrected by using images acquired at flexible and multiple TEs (31,32), from 3 to up to 6 different TEs that can be exploited to fit the signal decay. Indeed, iron deposition in certain organs and tissues shortens T2* values. Finally, accurate water and fat separation requires a more sophisticated model including a fat multifrequency spectrum with the use of up to 6 fat peaks, rather than just a single methylene peak (32,33). Such quantitative Dixon sequences and the associated post-processing constitute a reliable method for fat quantification. Up to 6 quantitative images may be produced: fat-only, water-only, in-phase, and out-of-phase images, as well as proton density fat fraction (PDFF), and R2* maps (R2*=1/T2*) (Figure 5) (34). One can notice that the earlier signal FF refers to the fraction of MR signal attributable to fat. Conversely, the PDFF is the ratio of the density of mobile triglycerides to the total density of mobile triglycerides and mobile water and has an acute correlation with histological findings (35).
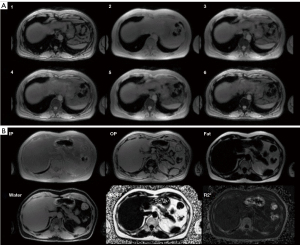
Thus, the quantification of the MFI is based on FF or PDFF images depending on the Dixon sequence available with the imaging system. The operator determines a region of interest (ROI) using segmentation software. The MFI is computed as the PDFF voxel average in the ROI.
The most frequently used software employing the Dixon technique are ImageJ (36,37), Osirix (38,39), MITK (40-44), ITK-SNAP (1,45-47), SiemensSyngo (48,49), AMRA (50), IntelliSpace (51), Mimics (52), MIPAV (53), MIAF (54,55), Analyzedirect-v11 (56-59), Aquarius-iNtuition-Edition (60), 3Dslicer (61), Horos (62), Muscl’X (5,63,64), PRIDE (3,65), Advantage-Window (4), Interactive Data Language (66), Intellispace (51), NiftyNet (67), Display1 (68), FSL software (69), IDEAL-IQ (18), MeVisLab (70), Myrian (71,72), Radionet (73) and Simple Ware (74-76).
Hiba et al. reported that the Dixon technique showed high reproducibility when measuring fat infiltration of the tibialis anterior in the setting of Myotonic Dystrophy type 1 (MD1) (77). Two research groups developed an automated thigh muscle segmentation using Dixon sequences. It was found to exhibit excellent accuracy and high reproducibility in FF estimation compared to manual segmentation (37,78).
Threshold-defined method (48 studies)
Three main methodologies were identified in order to define the cutoff between fat and muscle tissue, all applied on either T1 or T2 sequences without fat saturation.
- Fixed threshold: all pixels with a signal value higher than 120 (for pixels intensity ranging from 0 to 660) on the grayscale are defined as fatty tissue, while those lower than 120 are muscle tissue (79). The value of 120 was defined when the gray scale of muscle, fat, and bone tissues of the lower back muscles of 6 subjects were averaged, and this specific value was found to delimit the fat tissue from muscle and bones (80).
- Gaussian modeling: two Gaussian models are fitted on the pixel intensity histogram in order to identify the peaks of fat and muscle. The threshold between fat and muscle is then defined as the intersection between the two Gaussian models and used to classify pixels as fat or muscle tissue. This method is subject-specific (Figure 6) (81-84).
- Visual assessment: the operator is asked to specify the threshold to separate fat from muscle tissue on the histogram. The fat-muscle separation threshold is determined by locating the lower limit of the region-growing threshold at the base of the muscle peak. This is a subject-specific, but operator-dependent method (85,86).
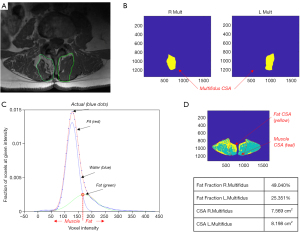
For all the previous methodologies, the MFI is computed as the proportion of the area of fat infiltration relative to the total area of the segmented muscle.
The software used in the 48 selected studies were 3Dslicer (87), AequitasIA (88), ImageJ (21,79,89-105), INFINIT (106), iSix (107), Osirix(17,24,82,84,108-111), ITK-SNAP (112-114), MIPAV (115), MIAF (55), Mimics (116), Surgimap (117,118), SliceOmatic (86,119), pMRI (120) and PiView (121).
Signal intensity (10 studies)
In this method, the mean signal intensity (MSI) of the segmented muscle is calculated. The intensity of this averaged grayscale increases (hypersignal) with the degree of fat infiltration in the region. For instance, Tabaraee et al. quantified multifidus fat infiltration following a 1-level minimally invasive lumbar discectomy to show the advantage of such interventions in reducing muscle degeneration compared to traditional open surgery (122).
The software used included PiView (123), OpaL (122), ImageJ (124,125), IDL (126,127) and MaZda (128).
Signal intensity/subQ (9 studies)
After computing the MSI of the segmented muscle, a region from the subcutaneous adipose tissue is selected and its MSI is calculated as well. MFI is then evaluated as the proportion of MSI of the muscle relative to the MSI of the subcutaneous adipose tissue (Figure 7) (129).
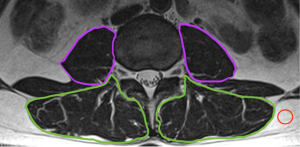
Software used for this method include Image J (20,130,131), PACS (132), OsiriX (129,133), AMIRA (134), ITK-SNAP (2) and Mimics (135).
Within the 168 studies, these 4 quantitative MFI evaluations accounted for 80% of all MIF evaluation methods, with the Dixon method being the most represented.
MFI applications in diseases
The most common diseases where MFI was explored were mainly neuromuscular disorders and musculoskeletal pathologies at the level of the spine, shoulders, hips and knees, in addition to studies in healthy subjects (Figure 8).
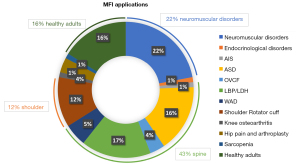
Neuromuscular disorders (37 studies)
Duchenne muscular dystrophy (DMD) (8 studies)
DMD is an X-linked, inherited disorder with an incidence of 4.8 per 100,000 (136). It is caused by mutations in the dystrophin gene, which lead to progressive weakening of skeletal muscle, loss of ambulation in adolescence, and death by early adulthood (137). DMD is first suspected by muscle biopsy and confirmed through genetic screening for the dystrophin gene mutation. The use of MRI is essential to assess disease progression and response to treatment. Pelvic, thigh, and calf muscles were the most studied muscles in this disease. Although patients with DMD present with hypertrophied calf muscles, studies reported an increased MFI that was found to be correlated to patient dysfunctional levels (such as the Gower’s sign, time to climb up four steps, and time to run 30 feet) (3,4,17). Three muscles showed the highest degree of MFI: adductor magnus and gluteus medius and magnus (138). The MFI of these muscles was positively correlated to age, height, and weight (138). Moreover, it was reported that using the intensity/subQ method for MFI quantification can show the multistep process of involvement of muscles in the lower limbs of DMD patients and allow the monitoring of disease progression (134).
Limb girdle muscular dystrophy type II (LGMD2) (10 studies)
LGMD2 is the most common form of heterogenous and genetically inherited LGMD. It is characterized by predominant proximal muscle weakness (hip girdle), elevated serum creatinine kinase, and muscle dystrophy on biopsy. LGMD2 is a recessive-autosomal form of LGMD, with a prevalence of 1.63 per 100,000 (139). Two studies have reported the quantification of MFI in this disease by evaluating its reliability (18,19). Thigh muscles were the most studied regions using Dixon sequences. MFI of thigh muscles in patients with LGMD2 correlated with clinical and functional status, such as the British medical research council scales, the motor function measure, muscle strength measurement using a dynamometer, the “timed up and go” test, and the 6-minute walk distance test (18,19).
One article studied muscle FF in calpainopathy, another type of LGMD (61).
MD1 (2 studies)
MD1 is an autosomal dominant multisystemic disorder and is one of the most frequently encountered muscular dystrophies in adults (140,141). It includes a wide range of symptoms such as myotonia, progressive muscle weakness and wasting, cardiac conduction defects, and endocrine and cognitive dysfunctions. In DM1 patients, motor impairment at the extremities progresses from distal to proximal. Although MRI has no role in the diagnosis of DM1, it is still used in research. Several studies have quantified the MFI of muscles in DMI patients using MRI, especially in the lower limbs. The use of the Dixon technique in the tibialis anterior muscle was capable of monitoring disease progression, as well as response to therapy (77). Other studies evaluated the MFI of trunk muscles using the Mercuri classification and indicated that MFI may be negatively correlated to muscle strength, trunk mobility, and balance. High MFI was also associated with decreased lung function (142).
Facioscapulohumeral muscular dystrophy (FSHD) (10 studies)
FSHD is the third most common inherited muscular disorder with an incidence of 3.95 per 100,000 (139). FSHD is characterized by a loss of repression of the DUX4 (protein that has toxic effects on muscle cells) (143). The decrease in muscle function during disease progression is strongly related to fat infiltration and muscle cell atrophy (36,144). MRI is mainly used in order to better understand the natural distribution of MFI, correlation with the disease, clinical status, severity, progression, and attenuation of specific muscle groups. The most commonly studied muscles in FSHD are thigh and leg muscles (145). Studies have shown that fatty infiltration begins in the distal end of affected muscles (49). The MFI was strongly correlated to the clinical status of FSHD and negatively correlated with manual testing of muscles and measures of muscle function (146,147). Furthermore, MFI measurement was shown to be a reproducible and sensitive biomarker to monitor the responsiveness of FSHD during aerobic exercise therapy (126).
Amyotrophic lateral sclerosis (ALS) (3 studies)
ALS is a rapidly progressive and fatal disease characterized by upper and lower motor neuron dysfunction, which leads to muscle degeneration (148). Its incidence ranges between 2.4 and 2.7 per 100,000 person per year (149). The initial clinical presentation may affect every segment of the body. The most common presentation is the asymmetric weakness of upper or lower limbs (148). Musculoskeletal MRI is not used for the diagnosis of ALS, but rather for research purposes. The MFI of shoulder muscles was shown to be correlated with the degree of brachial plexus alteration (150). In addition, the MFI of thigh and leg muscles using the quantitative Dixon technique was shown to be negatively correlated with disease functional status (ALS functional rating scale-revised). Also, MFI was shown to be a useful tool to differentiate between ALS and spino-bulbar muscular atrophy, another type of motor neuron disorder (45).
Four articles studied MFI in rare neuromuscular disorders: Becker muscular dystrophy (53), occulopharyngeal muscular dystrophy (151), Charcot-Marie-Tooth type 2 F (152), GNE myopathy (153).
Studies focusing on the MFI in neuromuscular disorders used mainly the Dixon and PDFF quantitative techniques. The qualitative Mercuri and Goutallier classifications were less used.
Endocrinological disorders (2 studies)
MFI has been used in the setting of two endocrinological disorders, acromegaly (65) and Cushing syndrome (3).
Acromegaly is characterized by elevated growth hormone (GH) secretion and insulin-like growth factor 1 (IGF1). Despite their lipolytic effects, the excess of GH and IGF1 is associated with increased MFI in skeletal muscles, which persists even after hormone levels have been controlled or the disease managed surgically (154,155). MFI of thigh muscles in patients with acromegaly is negatively correlated with muscle function (65).
Cushing syndrome is caused by hypercortisolism, which is associated with proximal myopathy and especially lower limb weakness (156). Musculoskeletal MRI has been used to evaluate muscle tissue evolution after blood cortisol levels have been controlled, and to search for relationships with muscle function. MFI of thigh muscles was negatively correlated with muscle strength and patient functional status. It was also shown that MFI remained elevated after blood cortisol levels were controlled (3).
The Dixon technique was used in these two pathologies.
Spine pathologies (72 studies)
Adolescent idiopathic scoliosis (AIS) (2 studies)
AIS is one of the most common 3D spinal deformities affecting adolescents, with a prevalence ranging between 1 to 4% with a female predominance (157). Despite many research efforts, the direct etiology behind AIS remains unclear. Many causes are involved, such as vertebral body deformation, paraspinal muscle defects leading to asymmetrical contractions, as well as biochemical and histopathological muscle alterations. Studies aimed to quantify the MFI of the multifidus and erector spinae muscles, mainly at the level of the apical vertebrae (83,89). Studies have found no correlations between increased MFI in multifidus and erector spinae muscles, the severity of radiographic scoliosis parameters, and curvature flexibility (83). However, a predominance of MFI on the concave side of the curvature has been reported (83,89). Furthermore, there was no significant difference regarding MFI of the multifidus and erector spinae muscles between subjects with AIS and controls.
Adult spinal deformity (ASD) (27 studies)
ASD encompasses a heterogeneous spectrum of deformities affecting the lumbar and thoracolumbar spine and includes de-novo scoliosis, degenerative thoracic kyphosis, degenerative lumbar lordosis, and spondylolisthesis (158). The incidence of ASD increases throughout the aging process, with a prevalence in individuals older than 65 years of age ranging from 38% to 68% (159,160). It is the result of complex-degenerative processes affecting the vertebral bodies, intervertebral discs, and paraspinal muscles, leading to spinal malalignment in the three planes, especially the sagittal plane with patients known to lean forward.
The most commonly studied muscles in ASD were the multifidus, erector spinae, psoas, and quadratus lumborum. Female patients with ASD tended to have an increased MFI of paraspinal muscles with increasing age, compared to their healthy counterparts (99,161).
Several studies investigated the correlation between MFI of paraspinal muscles and the severity of changes in radiographic parameters (96,97,123).
In degenerative lumbar scoliosis, the average MFI in the multifidus muscle was found to be higher on the concave than the convex side at the level of the apical vertebra. Additionally, the MFI of multifidus and erector spinae was found to be positively correlated to the Cobb angle and frontal malalignment (96,97). Furthermore, the MFI of paraspinal muscles showed a significant positive correlation with pelvic tilt and sagittal vertical axis, two radiographic parameters of sagittal alignment (114). Finally, spinopelvic sagittal malalignment could be predicted with a threshold of 43.9% of fat infiltration of multifidus and erector spinae (sensitivity =61.2%; specificity =59.4%) (112).
In degenerative lumbar lordosis patients, the increased MFI of paraspinal extensor muscles was found to be negatively correlated to the magnitude of the lumbar lordosis. Furthermore, the MFI of paraspinal extensor muscles was more correlated to dynamic parameters during walking than static parameters evaluated on radiographs (123).
Posterior interbody fusion is a very common treatment strategy in patients with ASD. The MFI of paravertebral muscles is considered a risk factor for post-operative complications. After lumbar fusion, the degree of MFI and the cross-sectional area of paravertebral muscles at the L4–L5 and L5–S1 levels were found to be important risk factors for the occurrence of adjacent segment disease (Figure 9) (20). Moreover, the MFI of paraspinal muscles preoperatively was found to be correlated to the loss of lumbar lordosis post-operatively (21).
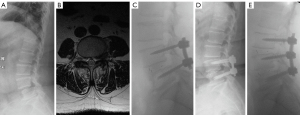
Osteoporotic vertebral compression fracture (OVCF) (6 studies)
The most common type of osteoporotic fracture is vertebral compression fracture (162). It generally results from minor trauma to the spine. This most commonly occurs at the midthoracic (T7–T8) and the thoracolumbar junctions (T12–L1). Many risk factors can cause OVCF, like aging, osteopenia, osteoporosis, corticosteroid treatment, weight loss, smoking, vitamin D deficiency, and depression (163,164).
OVCFs can lead to severe back pain, restricted physical activities, and impairments in activities of daily living, leading to a loss of independence, depression, chronic pain, and, in severe cases, spinal cord compression. Evaluation of OVCF is primarily done through clinical examination and X-rays with MRI being important diagnostic tool to help differentiate between malignant and benign fractures, and between chronic and acute fractures. Additionally, it is a useful tool to identify the MFI of paraspinal muscles or to evaluate the severity of vertebral fractures in the presence of posterior wall protrusion, posterior column extension, or spinal cord compression (165).
OVCFs result in a decrease in cross-sectional area and volume, and an increase in MFI of erector spinae and multifidus muscles, aggravating the risk of falling and occurrence of new OVCF (121,166,167).
Treatment can vary from conservative management (medications, bracing, physical therapy, and exercise) to percutaneous vertebroplasty or kyphoplasty (168). The MFI of paravertebral muscles correlated with radiographic parameters 6 months post-kyphoplasty (132). It was shown that, along with the increase in MFI of the erector spinae at L4, pelvic retroversion increased. Moreover, lower lumbar lordosis decreased with the increase of MFI of erector spinae and multifidus muscles at the L5 level.
Chronic low back pain (LBP) and lumbar disc disorders (28 studies)
LBP represents an important health problem. Approximately 80% of adults have experienced at least one episode of LBP, with degenerative disc disorders and lumbar disc herniation (LDH) being the most common causes (169). MRI remains the best imaging modality for the visualization and evaluation of intervertebral disc degeneration, using Pfirrmann classification (170,171). Several studies investigated the correlation between MFI in paravertebral muscles and spinal symptoms, such as radiculopathy, clinical presentation, and post-operative outcomes in patients with intervertebral disc disorders and LDH (103,105,109-111,122,125,172-174). In these studies, the MFI of the multifidus muscle at the same level of a herniated disc was found to be correlated with the severity of nerve compression and symptom duration. Moreover, the MFI of erector spinae and multifidus muscles was correlated with the Pfirrmann grade.
Whiplash-associated disorder (WAD) (9 studies)
Motor vehicle collisions may cause whiplash, which arises from rapid multiplanar acceleration/deceleration of the head and neck (175). WAD is a multifactorial clinical presentation (176) including neck movement disability, sensorimotor disturbances, distress, and cervical soft tissue degeneration (177). Deep extensor muscles, such as cervical multifidus and semispinalis cervicis, play an important role in maintaining cervical spine stability and postural control. Their degeneration, quantified by MFI, alters postural stability (Figure 10) (178-180). Many studies have shown the association between the increase of MFI and the increase of neck pain disability scores, proprioceptive deficits, chronic pain, and other functional impairments such as altered motor control and stiffness (57,58,67,178,181,182).
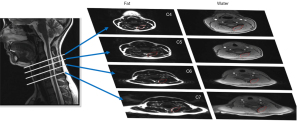
In spine pathologies, the main method used for MFI evaluation was the threshold method, except for the WAD investigation, where the Dixon technique was most frequent (Figure 10) (178).
Shoulder rotator cuff tears (20 studies)
The rotator cuff includes four muscles: supraspinatus, infraspinatus, teres minor, and subscapularis muscles. The supraspinatus is the most affected muscle in the rotator cuff. Rotator cuff tears affect around 20% to 30% of the population after the age of 60 (11). MFI represents the degenerative process of rotator cuff muscles (11). The analysis of MFI is primordial since the distribution of fat content of the supraspinatus muscle becomes heterogeneous once the global fat content of the muscle exceeds 10% (183). Several studies showed that MFI in the rotator cuff correlates with symptomatology, shoulder range of motion, and post-operative failure after tendon repair (60,184-186). One notable benefit when using the Dixon technique is its ability to perform a volumetric analysis of the muscle by analyzing several slices (187). In contrast, the Goutallier classification has limited reproducibility and it is restricted to a single slice (188).
The Goutallier classification was mainly used in the evaluation of MFI in the rotator cuff muscles in 9 studies. The Dixon technique was also applied (9 studies) for this muscle group and showed higher reliability and accuracy than the Goutallier classification (189).
Knee osteoarthritis (OA) (2 studies)
Knee OA is a painful degenerative disease affecting up to 6% of adults. Its prevalence increases with age, with over 40% of people aged over 75 years old being affected (190). Knee OA is associated with reduced strength of knee extensors and loss of muscle mass (191). It was shown that these patients have increased MFI of thigh muscles. It was thus suggested that the MFI of the quadriceps muscle may serve as a marker of the symptomatic and structural severity of the degenerative process (192). One study showed that sporting activities and lifestyle changes may decrease the MFI of the vastus medialis muscle, which is directly associated with a reduction in annual loss of cartilage volume and a reduction of the risk of knee OA (193).
Hip pain and arthroplasty (6 studies)
Hip muscles have a crucial role in maintaining hip joint mobility and stability, and in accomplishing activities of daily living such as standing, running, and walking (194). Hip muscle degeneration and especially the gluteus maximus can lead to hip joint diseases and accidental falls in the elderly (195,196). It was shown that a high MFI of hip muscles is associated with hip pain after physical activity. In addition, high fat infiltration of gluteus maximus was found to be associated with low physical activity, female gender, and obesity, even in healthy subjects (75). THA represents one of the most common procedures in patients with hip pain due to a variety of conditions. It can relieve pain, restore hip joint function, and improve quality of life (197). Patients with a history of high MFI of hip muscles presented a poor post-surgical outcome following THA (16,22).
Sarcopenia (2 studies)
Sarcopenia refers to the progressive loss of both muscle mass and function (198), appears commonly with aging and leads to an increased risk of falling and fractures among elderly patients. High intensity resistance training (HIRT) is strongly recommended by international guidelines, as it can improve muscle function and strength (199). MFI in thigh muscles after HIRT in elderly subjects was evaluated and was found to reduce sarcopenia and build lean muscle mass (54,200). Although only two studies have explicitly explored sarcopenia, investigations of the increase of MFI with age have been explored in healthy subjects.
Healthy subjects (27 studies)
Studies involving healthy subjects assessed the evolution of MFI either during the aging process, between young and older adults, or between genders. The association between bone mass density (BMD) and MFI as well as the integration of new automated algorithms for muscle segmentation were studied.
Through the aging process, MFI was found to increase mainly in the thigh and spine muscles (1,2,55,73,102,115). It was found to depend on gender and muscle groups (2,73). Higher MFI was more frequent in women than men and was found to be correlated to bone density loss (51,129). In thigh muscles, the muscles with most infiltration were the gracilis, the sartorius and the short head of biceps femoris (73).
Discussion
The evaluation of MFI using MRI is useful to establishing an accurate diagnosis, monitor disease progression, predict the severity of the disease, or to evaluate treatments in the setting of endocrinological diseases and neuromuscular or musculoskeletal disorders. This study presents a review of the methods available to evaluate MFI using MRI and their applications in multiple pathologies.
Qualitative methods, such as the Goutallier classification, are largely used in the context of rotator cuff tears and LBP. Based on a 2-to-4 score grading scale, they are known for their ease of use by clinicians. They can be applied to conventional T2-weighted images that are commonly prescribed by physicians for diagnostic purposes. However, they are used in only 20% of the referenced studies and are not subject-specific. Since the validity and reliability of these methods were scarcely evaluated, their clinical application should be approached with caution. Most of the investigations used quantitative methods (80%) requiring muscle segmentation, with the Dixon technique being the most widely used (45%). Studies utilizing the Dixon technique employed various modalities (2PD, 3PD, PDFF, etc.), and several software were used to quantify the percentage of fat signal in a specific ROI. Based on the needs and interests identified in the literature, automated muscle segmentation and calculation of MFI can be of utmost importance for physicians and researchers.
The evaluation of MFI seems to be particularly relevant in neuromuscular diseases, especially when evaluating the response to treatment, or objectively monitoring disease progression. It was also shown that MFI was predictive of the severity of these pathologies.
When evaluated for musculoskeletal diseases, MFI was correlated with the degree of shoulder range of motion in the presence of rotator cuff tears and failure of rotator cuff repair (60,184-186). MFI in the quadriceps was related to knee OA (193,201), whereas MFI in the hip muscles, especially the glutei, was related to post-operative complications after total hip replacement (16,22). Moreover, in the presence of LBP, MFI was more prevalent at the distal lumbar levels in all paraspinal muscles.
It is important to mention that muscles’ evaluation during physical examination is of paramount importance in clinical routine. The evaluation of MFI can give an additional insight, especially in the presence of complicated pathologies. For instance, in the setting of ASD, the MFI of the erector spinae was related to the severity of spinal malalignment both in the coronal and sagittal planes. Increased MFI in the trunk extensors was related to increased anterior shift of the trunk and pelvic retroversion. Complications and failure are known to occur in more than 50% of these cases (202). Several studies have attempted to understand the causes behind surgical failure that might occur due to over-correction of spinal malalignment (203,204). Spinal fusion is usually decided after a clinical examination of the patient and the evaluation of full standing X-rays on which spinopelvic and global alignment parameters are calculated. However, statis evaluations do not accurately represent activities of daily living, which emphasizes the importance of evaluating patients in other functional positions and during movement. More recently, studies have shown interest in quantifying the biomechanics of the spine in ASD patients in the sitting position and during movement (205-207), with recent reports exploring the risk of post-surgical mechanical complications based on the evaluation of spine flexibility (208). In order to complete the biomechanical assessment of the spine in ASD patients, an evaluation of the muscles, and more precisely the quality of the tissue, is important in order to estimate whether spinal fusion can be mechanically supported by the soft tissues. Thus, calculating the MFI can be a valuable predictor of muscle quality in ASD patients. As such, studies showed that increased MFI in the trunk was related to post-operative complications and increased risk of revision surgeries (20,209).
One of the major limitations of this study is related to the fact that the literature search did not use synonymous or Boolean algorithm. This may have resulted in overlooking some relevant articles. However, we believe this study still presents a relatively comprehensive and detailed review on the evaluation methods of MFI using MRI and their clinical applications (detailed literature table available in https://cdn.amegroups.cn/static/public/qims-24-177-1.xlsx). While the main objective of this review was to present the available techniques to evaluate MFI using MRI and the associated pathologies, this study did not focus on the correlation study between MFI and the severity of the pathology. General associations were presented in the text, and more in-depth analysis can be consulted in the listed articles.
Conclusions
The evaluation of MFI is important for diagnosis and can be representative of disease progression in several endocrinological, neuromuscular, or musculoskeletal diseases. The use of MFI assessment tools in the clinical setting seems to be important in spinal disorders, especially in ASD, to predict post-surgical complications. This review also showed the importance of automation of MFI assessment using quantitative MRI images, particularly Dixon sequences. Such automation could democratize the calculation of MFI, make its use easier in clinical settings and open MFI assessment applications to other diseases.
Acknowledgments
Funding: None.
Footnote
Reporting Checklist: The authors have completed the Narrative Review reporting checklist. Available at https://qims.amegroups.com/article/view/10.21037/qims-24-177/rc
Conflicts of Interest: All authors have completed the ICMJE uniform disclosure form (available at https://qims.amegroups.com/article/view/10.21037/qims-24-177/coif). A.A. is a consultant at Olea Medical. J.R., A.M. and S.C. are employees at Olea Medical in the research and scientific departments. The other authors have no conflicts of interest to declare.
Ethical Statement: The authors are accountable for all aspects of the work in ensuring that questions related to the accuracy or integrity of any part of the work are appropriately investigated and resolved.
Open Access Statement: This is an Open Access article distributed in accordance with the Creative Commons Attribution-NonCommercial-NoDerivs 4.0 International License (CC BY-NC-ND 4.0), which permits the non-commercial replication and distribution of the article with the strict proviso that no changes or edits are made and the original work is properly cited (including links to both the formal publication through the relevant DOI and the license). See: https://creativecommons.org/licenses/by-nc-nd/4.0/.
References
- Pinel S, Kelp NY, Bugeja JM, Bolsterlee B, Hug F, Dick TJM. Quantity versus quality: Age-related differences in muscle volume, intramuscular fat, and mechanical properties in the triceps surae. Exp Gerontol 2021;156:111594. [Crossref] [PubMed]
- Dallaway A, Hattersley J, Diokno M, Tallis J, Renshaw D, Wilson A, Wayte S, Weedall A, Duncan M. Age-related degeneration of lumbar muscle morphology in healthy younger versus older men. Aging Male 2020;23:1583-97. [Crossref] [PubMed]
- Martel-Duguech L, Alonso-Jiménez A, Bascuñana H, Díaz-Manera J, Llauger J, Nuñez-Peralta C, Biagetti B, Montesinos P, Webb SM, Valassi E. Thigh Muscle Fat Infiltration Is Associated With Impaired Physical Performance Despite Remission in Cushing's Syndrome. J Clin Endocrinol Metab 2020;105:dgz329. [Crossref] [PubMed]
- Yin L, Xie ZY, Xu HY, Zheng SS, Wang ZX, Xiao JX, Yuan Y. T2 Mapping and Fat Quantification of Thigh Muscles in Children with Duchenne Muscular Dystrophy. Curr Med Sci 2019;39:138-45. [Crossref] [PubMed]
- Moal B, Bronsard N, Raya JG, Vital JM, Schwab F, Skalli W, Lafage V. Volume and fat infiltration of spino-pelvic musculature in adults with spinal deformity. World J Orthop 2015;6:727-37. [Crossref] [PubMed]
- Rivas DA, McDonald DJ, Rice NP, Haran PH, Dolnikowski GG, Fielding RA. Diminished anabolic signaling response to insulin induced by intramuscular lipid accumulation is associated with inflammation in aging but not obesity. Am J Physiol Regul Integr Comp Physiol 2016;310:R561-9. [Crossref] [PubMed]
- Komolka K, Albrecht E, Wimmers K, Michal JJ, Maak S. Molecular heterogeneities of adipose depots - potential effects on adipose-muscle cross-talk in humans, mice and farm animals. J Genomics 2014;2:31-44. [Crossref] [PubMed]
- Uezumi A, Fukada S, Yamamoto N, Takeda S, Tsuchida K. Mesenchymal progenitors distinct from satellite cells contribute to ectopic fat cell formation in skeletal muscle. Nat Cell Biol 2010;12:143-52. [Crossref] [PubMed]
- Dong Y, Silva KA, Dong Y, Zhang L. Glucocorticoids increase adipocytes in muscle by affecting IL-4 regulated FAP activity. FASEB J 2014;28:4123-32. [Crossref] [PubMed]
- Engelke K, Museyko O, Wang L, Laredo JD. Quantitative analysis of skeletal muscle by computed tomography imaging-State of the art. J Orthop Translat 2018;15:91-103. [Crossref] [PubMed]
- Goutallier D, Postel JM, Bernageau J, Lavau L, Voisin MC. Fatty muscle degeneration in cuff ruptures. Pre- and postoperative evaluation by CT scan. Clin Orthop Relat Res 1994;78-83.
- Mercuri E, Pichiecchio A, Allsop J, Messina S, Pane M, Muntoni F. Muscle MRI in inherited neuromuscular disorders: past, present, and future. J Magn Reson Imaging 2007;25:433-40. [Crossref] [PubMed]
- Kjaer P, Bendix T, Sorensen JS, Korsholm L, Leboeuf-Yde C. Are MRI-defined fat infiltrations in the multifidus muscles associated with low back pain? BMC Med 2007;5:2. [Crossref] [PubMed]
- Solgaard Sorensen J, Kjaer P, Jensen ST, Andersen P. Low-field magnetic resonance imaging of the lumbar spine: reliability of qualitative evaluation of disc and muscle parameters. Acta Radiol 2006;47:947-53. [Crossref] [PubMed]
- Bal BS, Lowe JA. Muscle damage in minimally invasive total hip arthroplasty: MRI evidence that it is not significant. Instr Course Lect 2008;57:223-9.
- Klemt C, Simeone FJ, Melnic CM, Tirumala V, Xiong L, Kwon YM. MARS MRI assessment of fatty degeneration of the gluteal muscles in patients with THA: reliability and accuracy of commonly used classification systems. Skeletal Radiol 2021;50:665-72. [Crossref] [PubMed]
- Torriani M, Townsend E, Thomas BJ, Bredella MA, Ghomi RH, Tseng BS. Lower leg muscle involvement in Duchenne muscular dystrophy: an MR imaging and spectroscopy study. Skeletal Radiol 2012;41:437-45. [Crossref] [PubMed]
- Arrigoni F, De Luca A, Velardo D, Magri F, Gandossini S, Russo A, Froeling M, Bertoldo A, Leemans A, Bresolin N, D'angelo G. Multiparametric quantitative MRI assessment of thigh muscles in limb-girdle muscular dystrophy 2A and 2B. Muscle Nerve 2018;58:550-8. [Crossref] [PubMed]
- Willis TA, Hollingsworth KG, Coombs A, Sveen ML, Andersen S, Stojkovic T, Eagle M, Mayhew A, de Sousa PL, Dewar L, Morrow JM, Sinclair CD, Thornton JS, Bushby K, Lochmuller H, Hanna MG, Hogrel JY, Carlier PG, Vissing J, Straub V. Quantitative magnetic resonance imaging in limb-girdle muscular dystrophy 2I: a multinational cross-sectional study. PLoS One 2014;9:e90377. [Crossref] [PubMed]
- Gong Z, Li D, Zou F, Liu S, Wang H, Ma X. Low lumbar multifidus muscle status and bone mineral density are important risk factors for adjacent segment disease after lumbar fusion: a case-control study. J Orthop Surg Res 2022;17:490. [Crossref] [PubMed]
- Han G, Wang W, Zhou S, Li W, Zhang B, Sun Z, Li W. Paraspinal Muscle Degeneration as an Independent Risk for Loss of Local Alignment in Degenerative Lumbar Scoliosis Patients After Corrective Surgery. Global Spine J 2023;13:1186-93. [Crossref] [PubMed]
- Wang T, Shao L, Xu W, Chen H, Huang W. Comparison of morphological changes of gluteus medius and abductor strength for total hip arthroplasty via posterior and modified direct lateral approaches. Int Orthop 2019;43:2467-75. [Crossref] [PubMed]
- Guan J, Zhao D, Liu T, Yu X, Feng N, Jiang G, Li W, Yang K, Zhao H, Yang Y. Correlation between surgical segment mobility and paravertebral muscle fatty infiltration of upper adjacent segment in single-segment LDD patients: retrospective study at a minimum 2 years' follow-up. BMC Musculoskelet Disord 2023;24:28. [Crossref] [PubMed]
- Berry DB, Padwal J, Johnson S, Parra CL, Ward SR, Shahidi B. Methodological considerations in region of interest definitions for paraspinal muscles in axial MRIs of the lumbar spine. BMC Musculoskelet Disord 2018;19:135. [Crossref] [PubMed]
- Engelken F, Wassilew GI, Köhlitz T, Brockhaus S, Hamm B, Perka C. Diederichs uG. Assessment of fatty degeneration of the gluteal muscles in patients with THA using MRI: reliability and accuracy of the Goutallier and quartile classification systems. J Arthroplasty 2014;29:149-53. [Crossref] [PubMed]
- Dixon WT. Simple proton spectroscopic imaging. Radiology 1984;153:189-94. [Crossref] [PubMed]
- Özgen A. The Value of the T2-Weighted Multipoint Dixon Sequence in MRI of Sacroiliac Joints for the Diagnosis of Active and Chronic Sacroiliitis. AJR Am J Roentgenol 2017;208:603-8. [Crossref] [PubMed]
- Donato H, França M, Candelária I, Caseiro-Alves F. Liver MRI: From basic protocol to advanced techniques. Eur J Radiol 2017;93:30-9. [Crossref] [PubMed]
- Xiang QS, An L. Water-fat imaging with direct phase encoding. J Magn Reson Imaging 1997;7:1002-15. [Crossref] [PubMed]
- Liu CY, McKenzie CA, Yu H, Brittain JH, Reeder SB. Fat quantification with IDEAL gradient echo imaging: correction of bias from T(1) and noise. Magn Reson Med 2007;58:354-64. [Crossref] [PubMed]
- Hussain HK, Chenevert TL, Londy FJ, Gulani V, Swanson SD, McKenna BJ, Appelman HD, Adusumilli S, Greenson JK, Conjeevaram HS. Hepatic fat fraction: MR imaging for quantitative measurement and display--early experience. Radiology 2005;237:1048-55. [Crossref] [PubMed]
- Yu H, Shimakawa A, McKenzie CA, Brodsky E, Brittain JH, Reeder SB. Multiecho water-fat separation and simultaneous R2* estimation with multifrequency fat spectrum modeling. Magn Reson Med 2008;60:1122-34. [Crossref] [PubMed]
- Yokoo T, Bydder M, Hamilton G, Middleton MS, Gamst AC, Wolfson T, Hassanein T, Patton HM, Lavine JE, Schwimmer JB, Sirlin CB. Nonalcoholic fatty liver disease: diagnostic and fat-grading accuracy of low-flip-angle multiecho gradient-recalled-echo MR imaging at 1.5 T. Radiology 2009;251:67-76. [Crossref] [PubMed]
- Arango-Lievano M, Boutelier T, Brun L, Campana Tremblay S, Casagranda S, Gautier G, Geyler E, Girard F, Haxaire Y, Hermoso A, Kieusseyan N, Masi B, Mitulescu A, Papageorgakis C, Rasoanandrianina H, Rebbah H, Rouyer J, Sudre R. Spin to the Limit: MRI Principles & Physical Challenges. In: Djeridane F, Mitulescu A, Campana Tremplay SC, Arango-Lievano M, Bucciarelli B, Geyler E, editors. Publisher, Olea Medical, 2021.
- Bannas P, Kramer H, Hernando D, Agni R, Cunningham AM, Mandal R, Motosugi U, Sharma SD, Munoz del Rio A, Fernandez L, Reeder SB. Quantitative magnetic resonance imaging of hepatic steatosis: Validation in ex vivo human livers. Hepatology 2015;62:1444-55. [Crossref] [PubMed]
- Janssen BH, Voet NB, Nabuurs CI, Kan HE, de Rooy JW, Geurts AC, Padberg GW, van Engelen BG, Heerschap A. Distinct disease phases in muscles of facioscapulohumeral dystrophy patients identified by MR detected fat infiltration. PLoS One 2014;9:e85416. [Crossref] [PubMed]
- Mandić M, Rullman E, Widholm P, Lilja M, Dahlqvist Leinhard O, Gustafsson T, Lundberg TR. Automated assessment of regional muscle volume and hypertrophy using MRI. Sci Rep 2020;10:2239. [Crossref] [PubMed]
- Berry DB, Rodriguez-Soto AE, Englund EK, Shahidi B, Parra C, Frank LR, Kelly KR, Ward SR. Multiparametric MRI characterization of level dependent differences in lumbar muscle size, quality, and microstructure. JOR Spine 2020;3:e1079. [Crossref] [PubMed]
- Barnard AM, Lott DJ, Batra A, Triplett WT, Forbes SC, Riehl SL, Willcocks RJ, Smith BK, Vandenborne K, Walter GA. Imaging respiratory muscle quality and function in Duchenne muscular dystrophy. J Neurol 2019;266:2752-63. [Crossref] [PubMed]
- Schlaeger S, Sollmann N, Zoffl A, Becherucci EA, Weidlich D, Kottmaier E, Riederer I, Greve T, Montagnese F, Deschauer M, Schoser B, Zimmer C, Karampinos DC, Kirschke JS, Baum T. Quantitative Muscle MRI in Patients with Neuromuscular Diseases-Association of Muscle Proton Density Fat Fraction with Semi-Quantitative Grading of Fatty Infiltration and Muscle Strength at the Thigh Region. Diagnostics (Basel) 2021;11:1056. [Crossref] [PubMed]
- Greve T, Burian E, Zoffl A, Feuerriegel G, Schlaeger S, Dieckmeyer M, Sollmann N, Klupp E, Weidlich D, Inhuber S, Löffler M, Montagnese F, Deschauer M, Schoser B, Bublitz S, Zimmer C, Karampinos DC, Kirschke JS, Baum T. Regional variation of thigh muscle fat infiltration in patients with neuromuscular diseases compared to healthy controls. Quant Imaging Med Surg 2021;11:2610-21. [Crossref] [PubMed]
- Jung M, Rospleszcz S, Löffler MT, Walter SS, Maurer E, Jungmann PM, Peters A, Nattenmüller J, Schlett CL, Bamberg F, Kiefer LS, Diallo TD. Association of lumbar vertebral bone marrow and paraspinal muscle fat composition with intervertebral disc degeneration: 3T quantitative MRI findings from the population-based KORA study. Eur Radiol 2023;33:1501-12. [Crossref] [PubMed]
- Burian E, Syväri J, Dieckmeyer M, Holzapfel C, Drabsch T, Sollmann N, Kirschke JS, Rummeny EJ, Zimmer C, Hauner H, Karampinos DC, Baum T, Junker D. Age- and BMI-related variations of fat distribution in sacral and lumbar bone marrow and their association with local muscle fat content. Sci Rep 2020;10:9686. [Crossref] [PubMed]
- Dieckmeyer M, Inhuber S, Schläger S, Weidlich D, Mookiah MRK, Subburaj K, Burian E, Sollmann N, Kirschke JS, Karampinos DC, Baum T. Association of Thigh Muscle Strength with Texture Features Based on Proton Density Fat Fraction Maps Derived from Chemical Shift Encoding-Based Water-Fat MRI. Diagnostics (Basel) 2021;11:302. [Crossref] [PubMed]
- Klickovic U, Zampedri L, Sinclair CDJ, Wastling SJ, Trimmel K, Howard RS, Malaspina A, Sharma N, Sidle K, Emira A, Shah S, Yousry TA, Hanna MG, Greensmith L, Morrow JM, Thornton JS, Fratta P. Skeletal muscle MRI differentiates SBMA and ALS and correlates with disease severity. Neurology 2019;93:e895-907. [Crossref] [PubMed]
- Trevino JH 3rd, Gorny KR, Gomez-Cintron A, Zhao C, Giambini H. A quantitative alternative to the Goutallier classification system using Lava Flex and Ideal MRI techniques: volumetric intramuscular fatty infiltration of the supraspinatus muscle, a cadaveric study. MAGMA 2019;32:607-15. [Crossref] [PubMed]
- Giambini H, Hatta T, Gorny KR, Widholm P, Karlsson A, Leinhard OD, Adkins MC, Zhao C, An KN. Intramuscular fat infiltration evaluated by magnetic resonance imaging predicts the extensibility of the supraspinatus muscle. Muscle Nerve 2018;57:129-35. [Crossref] [PubMed]
- Lee SK, Jung JY, Kang YR, Jung JH, Yang JJ. Fat quantification of multifidus muscle using T2-weighted Dixon: which measurement methods are best suited for revealing the relationship between fat infiltration and herniated nucleus pulposus. Skeletal Radiol 2020;49:263-71. [Crossref] [PubMed]
- Heskamp L, Ogier A, Bendahan D, Heerschap A. Whole-muscle fat analysis identifies distal muscle end as disease initiation site in facioscapulohumeral muscular dystrophy. Commun Med (Lond) 2022;2:155. [Crossref] [PubMed]
- Mellion ML, Widholm P, Karlsson M, Ahlgren A, Tawil R, Wagner KR, Statland JM, Wang L, Shieh PB, van Engelen BGM, Kools J, Ronco L, Odueyungbo A, Jiang J, Han JJ, Hatch M, Towles J, Leinhard OD, Cadavid D. Quantitative Muscle Analysis in FSHD Using Whole-Body Fat-Referenced MRI: Composite Scores for Longitudinal and Cross-sectional Analysis. Neurology 2022;99:e877-89. [Crossref] [PubMed]
- Zhao Y, Huang M, Serrano Sosa M, Cattell R, Fan W, Li M, Chen J, Gao M, Zhou Q, Li S, Zhang X, Huang C. Fatty infiltration of paraspinal muscles is associated with bone mineral density of the lumbar spine. Arch Osteoporos 2019;14:99. [Crossref] [PubMed]
- Trevino Iii JH, Yuri T, Hatta T, Kiyoshige Y, Jacobs PM, Giambini H. Three-dimensional quantitative measurements of atrophy and fat infiltration in sub-regions of the supraspinatus muscle show heterogeneous distributions: a cadaveric study. Arch Orthop Trauma Surg 2022;142:1395-403. [Crossref] [PubMed]
- Wokke BH, Hooijmans MT, van den Bergen JC, Webb AG, Verschuuren JJ, Kan HE. Muscle MRS detects elevated PDE/ATP ratios prior to fatty infiltration in Becker muscular dystrophy. NMR Biomed 2014;27:1371-7. [Crossref] [PubMed]
- Ghasemikaram M, Chaudry O, Nagel AM, Uder M, Jakob F, Kemmler W, Kohl M, Engelke K. Effects of 16 months of high intensity resistance training on thigh muscle fat infiltration in elderly men with osteosarcopenia. Geroscience 2021;43:607-17. [Crossref] [PubMed]
- Engelke K, Ghasemikaram M, Chaudry O, Uder M, Nagel AM, Jakob F, Kemmler W. The effect of ageing on fat infiltration of thigh and paraspinal muscles in men. Aging Clin Exp Res 2022;34:2089-98. [Crossref] [PubMed]
- Elliott JM, Walton DM, Rademaker A, Parrish TB. Quantification of cervical spine muscle fat: a comparison between T1-weighted and multi-echo gradient echo imaging using a variable projection algorithm (VARPRO). BMC Med Imaging 2013;13:30. [Crossref] [PubMed]
- Elliott JM, Courtney DM, Rademaker A, Pinto D, Sterling MM, Parrish TB. The Rapid and Progressive Degeneration of the Cervical Multifidus in Whiplash: An MRI Study of Fatty Infiltration. Spine (Phila Pa 1976) 2015;40:E694-700. [Crossref] [PubMed]
- Karlsson A, Leinhard OD, Åslund U, West J, Romu T, Smedby Ö, Zsigmond P, Peolsson A. An Investigation of Fat Infiltration of the Multifidus Muscle in Patients With Severe Neck Symptoms Associated With Chronic Whiplash-Associated Disorder. J Orthop Sports Phys Ther 2016;46:886-93. [Crossref] [PubMed]
- Peolsson A, Karlsson A, Peterson G, Borén H, Zsigmond P, Elliott JM, Leinhard OD. Morphology and composition of the ventral neck muscles in individuals with chronic whiplash related disorders compared to matched healthy controls: a cross-sectional case-control study. BMC Musculoskelet Disord 2022;23:867. [Crossref] [PubMed]
- Davis DL, Almardawi R, Henn RF 3rd, Zhuo J, Mulligan ME, Resnik CS, Abdullah SB, Al Khalifah H, Gilotra MN, Hasan SA, Gullapalli RP. Correlation of Quantitative Versus Semiquantitative Measures of Supraspinatus Intramuscular Fatty Infiltration to Shoulder Range of Motion and Strength: A Pilot Study. Curr Probl Diagn Radiol 2021;50:629-36. [Crossref] [PubMed]
- Forsting J, Rohm M, Froeling M, Güttsches AK, Südkamp N, Roos A, Vorgerd M, Schlaffke L, Rehmann R. Quantitative muscle MRI captures early muscle degeneration in calpainopathy. Sci Rep 2022;12:19676. [Crossref] [PubMed]
- Emanuelsson EB, Berry DB, Reitzner SM, Arif M, Mardinoglu A, Gustafsson T, Ward SR, Sundberg CJ, Chapman MA. MRI characterization of skeletal muscle size and fatty infiltration in long-term trained and untrained individuals. Physiol Rep 2022;10:e15398. [Crossref] [PubMed]
- Boissière L, Moal B, Gille O, De-Roquefeuil E, Durieux M, Obeid I, Dousset V, Vital JM, Skalli W. Lumbar spinal muscles and spinal canal study by MRI three-dimensional reconstruction in adult lumbar spinal stenosis. Orthop Traumatol Surg Res 2017;103:279-83. [Crossref] [PubMed]
- Bao H, Moal B, Vira S, Bronsard N, Amabile C, Errico T, Schwab F, Skalli W, Dubousset J, Lafage V. Spino-femoral muscles affect sagittal alignment and compensatory recruitment: a new look into soft tissues in adult spinal deformity. Eur Spine J 2020;29:2998-3005. [Crossref] [PubMed]
- Martel-Duguech L, Alonso-Pérez J, Bascuñana H, Díaz-Manera J, Llauger J, Nuñez-Peralta C, Montesinos P, Webb SM, Valassi E. Intramuscular fatty infiltration and physical function in controlled acromegaly. Eur J Endocrinol 2021;185:167-77. [Crossref] [PubMed]
- Whitney DG, Singh H, Miller F, Barbe MF, Slade JM, Pohlig RT, Modlesky CM. Cortical bone deficit and fat infiltration of bone marrow and skeletal muscle in ambulatory children with mild spastic cerebral palsy. Bone 2017;94:90-7. [Crossref] [PubMed]
- Weber KA 2nd, Abbott R, Bojilov V, Smith AC, Wasielewski M, Hastie TJ, Parrish TB, Mackey S, Elliott JM. Multi-muscle deep learning segmentation to automate the quantification of muscle fat infiltration in cervical spine conditions. Sci Rep 2021;11:16567. [Crossref] [PubMed]
- Menezes-Reis R, Bonugli GP, Salmon CEG, Mazoroski D, Herrero CFPDS, Nogueira-Barbosa MH. Relationship of spinal alignment with muscular volume and fat infiltration of lumbar trunk muscles. PLoS One 2018;13:e0200198. [Crossref] [PubMed]
- Leporq B, Le Troter A, Le Fur Y, Salort-Campana E, Guye M, Beuf O, Attarian S, Bendahan D. Combined quantification of fatty infiltration, T (1)-relaxation times and T (2)*-relaxation times in normal-appearing skeletal muscle of controls and dystrophic patients. MAGMA 2017;30:407-15. [Crossref] [PubMed]
- Wieser K, Joshy J, Filli L, Kriechling P, Sutter R, Fürnstahl P, Valdivieso P, Wyss S, Meyer DC, Flück M, Gerber C. Changes of Supraspinatus Muscle Volume and Fat Fraction After Successful or Failed Arthroscopic Rotator Cuff Repair. Am J Sports Med 2019;47:3080-8. [Crossref] [PubMed]
- Kälin PS, Crawford RJ, Marcon M, Manoliu A, Bouaicha S, Fischer MA, Ulbrich EJ. Shoulder muscle volume and fat content in healthy adult volunteers: quantification with DIXON MRI to determine the influence of demographics and handedness. Skeletal Radiol 2018;47:1393-402. [Crossref] [PubMed]
- Crawford RJ, Filli L, Elliott JM, Nanz D, Fischer MA, Marcon M, Ulbrich EJ. Age- and Level-Dependence of Fatty Infiltration in Lumbar Paravertebral Muscles of Healthy Volunteers. AJNR Am J Neuroradiol 2016;37:742-8. [Crossref] [PubMed]
- Hogrel JY, Barnouin Y, Azzabou N, Butler-Browne G, Voit T, Moraux A, Leroux G, Behin A, McPhee JS, Carlier PG. NMR imaging estimates of muscle volume and intramuscular fat infiltration in the thigh: variations with muscle, gender, and age. Age (Dordr) 2015;37:9798. [Crossref] [PubMed]
- Belzunce MA, Henckel J, Fotiadou A, Di Laura A, Hart A. Automated measurement of fat infiltration in the hip abductors from Dixon magnetic resonance imaging. Magn Reson Imaging 2020;72:61-70. [Crossref] [PubMed]
- Belzunce MA, Henckel J, Di Laura A, Hart A. Intramuscular fat in gluteus maximus for different levels of physical activity. Sci Rep 2021;11:21401. [Crossref] [PubMed]
- Belzunce MA, Henckel J, Di Laura A, Hart AJ. Reference values for volume, fat content and shape of the hip abductor muscles in healthy individuals from Dixon MRI. NMR Biomed 2022;35:e4636. [Crossref] [PubMed]
- Hiba B, Richard N, Hébert LJ, Coté C, Nejjari M, Vial C, Bouhour F, Puymirat J, Janier M. Quantitative assessment of skeletal muscle degeneration in patients with myotonic dystrophy type 1 using MRI. J Magn Reson Imaging 2012;35:678-85. [Crossref] [PubMed]
- Ding J, Cao P, Chang HC, Gao Y, Chan SHS, Vardhanabhuti V. Deep learning-based thigh muscle segmentation for reproducible fat fraction quantification using fat-water decomposition MRI. Insights Imaging 2020;11:128. [Crossref] [PubMed]
- Miura K, Kadone H, Asada T, Koda M, Funayama T, Takahashi H, Noguchi H, Mataki K, Shibao Y, Sato K, Eto F, Kono M, Suzuki K, Yamazaki M. The fatty degeneration of the lumbar erector spinae muscles affects dynamic spinal compensation ability during gait in adult spinal deformity. Sci Rep 2021;11:18088. [Crossref] [PubMed]
- Ranson CA, Burnett AF, Kerslake R, Batt ME, O'Sullivan PB. An investigation into the use of MR imaging to determine the functional cross sectional area of lumbar paraspinal muscles. Eur Spine J 2006;15:764-73. [Crossref] [PubMed]
- Anderson B, Ordaz A, Zlomislic V, Allen RT, Garfin SR, Schuepbach R, Farshad M, Schenk S, Ward SR, Shahidi B. Paraspinal Muscle Health is Related to Fibrogenic, Adipogenic, and Myogenic Gene Expression in Patients with Lumbar Spine Pathology. BMC Musculoskelet Disord 2022;23:608. [Crossref] [PubMed]
- Shahidi B, Parra CL, Berry DB, Hubbard JC, Gombatto S, Zlomislic V, Allen RT, Hughes-Austin J, Garfin S, Ward SR. Contribution of Lumbar Spine Pathology and Age to Paraspinal Muscle Size and Fatty Infiltration. Spine (Phila Pa 1976) 2017;42:616-23. [Crossref] [PubMed]
- Berry DB, Grant CD, Farnsworth CL, Englund EK, Newton PO, Shahidi B. The influence of 3D curve severity on paraspinal muscle fatty infiltration in patients with adolescent idiopathic scoliosis. Spine Deform 2021;9:987-95. [Crossref] [PubMed]
- Berry DB, Padwal J, Johnson S, Englund EK, Ward SR, Shahidi B. The effect of high-intensity resistance exercise on lumbar musculature in patients with low back pain: a preliminary study. BMC Musculoskelet Disord 2019;20:290. [Crossref] [PubMed]
- Wong AK, Beattie KA, Min KK, Gordon C, Pickard L, Papaioannou A, Adachi JDCanadian Multicentre Osteoporosis Study. (CaMos) Research Group. Peripheral quantitative computed tomography-derived muscle density and peripheral magnetic resonance imaging-derived muscle adiposity: precision and associations with fragility fractures in women. J Musculoskelet Neuronal Interact 2014;14:401-10.
- Lorbergs AL, Noseworthy MD, Adachi JD, Stratford PW, MacIntyre NJ. Fat Infiltration in the Leg is Associated with Bone Geometry and Physical Function in Healthy Older Women. Calcif Tissue Int 2015;97:353-63. [Crossref] [PubMed]
- Jeong HJ, Kwon J, Rhee SM, Oh JH. New quantified measurement of fatty infiltration of the rotator cuff muscles using magnetic resonance imaging. J Orthop Sci 2020;25:986-91. [Crossref] [PubMed]
- Ali I, Ulbricht C, McGregor AH. Degeneration of the extensor muscle group in a surgical low back and leg pain population. J Back Musculoskelet Rehabil 2011;24:23-30. [Crossref] [PubMed]
- Wajchenberg M, Astur N, Fernandes EA, Paredes-Gamero EJ, Luciano RP, Schmidt B, Oliveira ASB, Martins DE. Assessment of fatty infiltration of the multifidus muscle in patients with adolescent idiopathic scoliosis through evaluation by magnetic resonance imaging compared with histological analysis: a diagnostic accuracy study. J Pediatr Orthop B 2019;28:362-7. [Crossref] [PubMed]
- Hida T, Eastlack RK, Kanemura T, Mundis GM Jr, Imagama S, Akbarnia BA. Effect of race, age, and gender on lumbar muscle volume and fat infiltration in the degenerative spine. J Orthop Sci 2021;26:69-74. [Crossref] [PubMed]
- Han G, Zou D, Liu Z, Zhang B, Gong C, Zhou S, Li W, Sun Z, Li W. Fat infiltration of paraspinal muscles as an independent risk for bone nonunion after posterior lumbar interbody fusion. BMC Musculoskelet Disord 2022;23:232. [Crossref] [PubMed]
- De Martino E, Hides J, Elliott JM, Hoggarth MA, Zange J, Lindsay K, Debuse D, Winnard A, Beard D, Cook JA, Salomoni SE, Weber T, Scott J, Hodges PW, Caplan N. The Effects of Reconditioning Exercises Following Prolonged Bed Rest on Lumbopelvic Muscle Volume and Accumulation of Paraspinal Muscle Fat. Front Physiol 2022;13:862793. [Crossref] [PubMed]
- Hayashi K, Upasani VV, Pawelek JB, Aubin CE, Labelle H, Lenke LG, Jackson R, Newton PO. Three-dimensional analysis of thoracic apical sagittal alignment in adolescent idiopathic scoliosis. Spine (Phila Pa 1976) 2009;34:792-7. [Crossref] [PubMed]
- Katsu M, Ohba T, Ebata S, Haro H. Comparative study of the paraspinal muscles after OVF between the insufficient union and sufficient union using MRI. BMC Musculoskelet Disord 2018;19:143. [Crossref] [PubMed]
- Xie D, Zhang J, Ding W, Yang S, Yang D, Ma L, Zhang J. Abnormal change of paravertebral muscle in adult degenerative scoliosis and its association with bony structural parameters. Eur Spine J 2019;28:1626-37. [Crossref] [PubMed]
- Tang Y, Yang S, Chen C, Luo K, Chen Y, Wang D, Tan J, Dai Q, Zhang C, Wu W, Xu J, Luo F. Assessment of the association between paraspinal muscle degeneration and quality of life in patients with degenerative lumbar scoliosis. Exp Ther Med 2020;20:505-11. [Crossref] [PubMed]
- Kiram A, Hu Z, Man GC, Ma H, Li J, Xu Y, Qian Z, Zhu Z, Liu Z, Qiu Y. The role of paraspinal muscle degeneration in coronal imbalance in patients with degenerative scoliosis. Quant Imaging Med Surg 2022;12:5101-13. [Crossref] [PubMed]
- Xia W, Fu H, Zhu Z, Liu C, Wang K, Xu S, Liu H. Association between back muscle degeneration and spinal-pelvic parameters in patients with degenerative spinal kyphosis. BMC Musculoskelet Disord 2019;20:454. [Crossref] [PubMed]
- Sun D, Wang Z, Mou J, Tian F, Cao J, Guo L, Liu P. Characteristics of paraspinal muscle degeneration in degenerative diseases of the lumbar spine at different ages. Clin Neurol Neurosurg 2022;223:107484. [Crossref] [PubMed]
- Tamai K, Grisdela P Jr, Romanu J, Paholpak P, Nakamura H, Wang JC, Buser Z. The Impact of Cervical Spinal Muscle Degeneration on Cervical Sagittal Balance and Spinal Degenerative Disorders. Clin Spine Surg 2019;32:E206-13. [Crossref] [PubMed]
- Pezolato A, de Vasconcelos EE, Defino HL, Nogueira-Barbosa MH. Fat infiltration in the lumbar multifidus and erector spinae muscles in subjects with sway-back posture. Eur Spine J 2012;21:2158-64. [Crossref] [PubMed]
- Takayama K, Kita T, Nakamura H, Kanematsu F, Yasunami T, Sakanaka H, Yamano Y. New Predictive Index for Lumbar Paraspinal Muscle Degeneration Associated With Aging. Spine (Phila Pa 1976) 2016;41:E84-90. [Crossref] [PubMed]
- Zhao X, Liang H, Hua Z, Li W, Li J, Wang L, Shen Y. The morphological characteristics of paraspinal muscles in young patients with unilateral neurological symptoms of lumbar disc herniation. BMC Musculoskelet Disord 2022;23:994. [Crossref] [PubMed]
- Liu Y, Liu Y, Hai Y, Liu T, Guan L, Chen X. Fat Infiltration in the Multifidus Muscle as a Predictor of Prognosis After Decompression and Fusion in Patients with Single-Segment Degenerative Lumbar Spinal Stenosis: An Ambispective Cohort Study Based on Propensity Score Matching. World Neurosurg 2019;128:e989-e1001. [Crossref] [PubMed]
- James G, Chen X, Diwan A, Hodges PW. Fat infiltration in the multifidus muscle is related to inflammatory cytokine expression in the muscle and epidural adipose tissue in individuals undergoing surgery for intervertebral disc herniation. Eur Spine J 2021;30:837-45. [Crossref] [PubMed]
- Lee YB, Yang CJ, Li CZ, Zhuan Z, Kwon SC, Noh KC. Can a Single Sagittal Magnetic Resonance Imaging Slice Represent Whole Fatty Infiltration in Chronic Rotator Cuff Tears at the Supraspinatus? Clin Orthop Surg 2018;10:55-63. [Crossref] [PubMed]
- Hoppe S, Maurer D, Valenzuela W, Benneker LM, Bigdon SF, Häckel S, Wangler S, Albers CE. 3D analysis of fatty infiltration of the paravertebral lumbar muscles using T2 images-a new approach. Eur Spine J 2021;30:2570-6. [Crossref] [PubMed]
- Faur C, Patrascu JM, Haragus H, Anglitoiu B. Correlation between multifidus fatty atrophy and lumbar disc degeneration in low back pain. BMC Musculoskelet Disord 2019;20:414. [Crossref] [PubMed]
- Fortin M, Lazáry À, Varga PP, McCall I, Battié MC. Paraspinal muscle asymmetry and fat infiltration in patients with symptomatic disc herniation. Eur Spine J 2016;25:1452-9. [Crossref] [PubMed]
- Urrutia J, Besa P, Lobos D, Andia M, Arrieta C, Uribe S. Is a single-level measurement of paraspinal muscle fat infiltration and cross-sectional area representative of the entire lumbar spine? Skeletal Radiol 2018;47:939-45. [Crossref] [PubMed]
- Urrutia J, Besa P, Lobos D, Campos M, Arrieta C, Andia M, Uribe S. Lumbar paraspinal muscle fat infiltration is independently associated with sex, age, and inter-vertebral disc degeneration in symptomatic patients. Skeletal Radiol 2018;47:955-61. [Crossref] [PubMed]
- Muellner M, Haffer H, Chiapparelli E, Dodo Y, Tan ET, Shue J, Zhu J, Sama AA, Cammisa FP, Girardi FP, Hughes AP. Differences in lumbar paraspinal muscle morphology in patients with sagittal malalignment undergoing posterior lumbar fusion surgery. Eur Spine J 2022;31:3109-18. [Crossref] [PubMed]
- Muellner M, Chiapparelli E, Moser M, Haffer H, Dodo Y, Adl Amini D, Carrino JA, Tan ET, Shue J, Zhu J, Sama AA, Cammisa FP, Girardi FP, Hughes AP. The effect of age on psoas and paraspinal muscle morphology in patients undergoing posterior lumbar fusion surgery. Eur Spine J 2022;31:2619-28. [Crossref] [PubMed]
- Muellner M, Haffer H, Moser M, Chiapparelli E, Dodo Y, Adl Amini D, Carrino JA, Tan ET, Shue J, Zhu J, Sama AA, Cammisa FP, Girardi FP, Hughes AP. Paraspinal musculature impairment is associated with spinopelvic and spinal malalignment in patients undergoing lumbar fusion surgery. Spine J 2022;22:2006-16. [Crossref] [PubMed]
- Yoshiko A, Hioki M, Kanehira N, Shimaoka K, Koike T, Sakakibara H, Oshida Y, Akima H. Three-dimensional comparison of intramuscular fat content between young and old adults. BMC Med Imaging 2017;17:12. [Crossref] [PubMed]
- Millett PJ, Schoenahl JY, Allen MJ, Motta T, Gaskill TR. An association between the inferior humeral head osteophyte and teres minor fatty infiltration: evidence for axillary nerve entrapment in glenohumeral osteoarthritis. J Shoulder Elbow Surg 2013;22:215-21. [Crossref] [PubMed]
- Passias PG, Vasquez-Montes D, Poorman GW, Protopsaltis T, Horn SR, Bortz CA, Segreto F, Diebo B, Ames C, Smith J, LaFage V, LaFage R, Klineberg E, Shaffrey C, Bess S, Schwab F. ISSG. Predictive model for distal junctional kyphosis after cervical deformity surgery. Spine J 2018;18:2187-94. [Crossref] [PubMed]
- Passias PG, Segreto FA, Horn SR, Lafage V, Lafage R, Smith JS, et al. Fatty infiltration of the cervical extensor musculature, cervical sagittal balance, and clinical outcomes: An analysis of operative adult cervical deformity patients. J Clin Neurosci 2020;72:134-41. [Crossref] [PubMed]
- Davison MJ, Maly MR, Adachi JD, Noseworthy MD, Beattie KA. Relationships between fatty infiltration in the thigh and calf in women with knee osteoarthritis. Aging Clin Exp Res 2017;29:291-9. [Crossref] [PubMed]
- Marunowski K, Świętoń D, Bzyl W, Grzywińska M, Bandosz P, Khrichenko D, Piskunowicz M. Reference values for MRI-derived psoas and paraspinal muscles and macroscopic fat infiltrations in paraspinal muscles in children. J Cachexia Sarcopenia Muscle 2022;13:2515-24. [Crossref] [PubMed]
- So KY, Kim DH, Choi DH, Kim CY, Kim JS, Choi YS. The influence of fat infiltration of back extensor muscles on osteoporotic vertebral fractures. Asian Spine J 2013;7:308-13. [Crossref] [PubMed]
- Tabaraee E, Ahn J, Bohl DD, Phillips FM, Singh K. Quantification of Multifidus Atrophy and Fatty Infiltration Following a Minimally Invasive Microdiscectomy. Int J Spine Surg 2015;9:25. [Crossref] [PubMed]
- Lee JH, Lee SH. Does lumbar paraspinal muscles improve after corrective fusion surgery in degenerative flat black? Indian J Orthop 2017;51:147-54. [Crossref] [PubMed]
- Umezawa H, Daimon K, Fujiwara H, Nishiwaki Y, Michikawa T, Okada E, Nojiri K, Watanabe M, Katoh H, Shimizu K, Ishihama H, Fujita N, Tsuji T, Nakamura M, Matsumoto M, Watanabe K. Changes in cross-sectional areas of posterior extensor muscles in thoracic spine: a 10-year longitudinal MRI study. Sci Rep 2022;12:14717. [Crossref] [PubMed]
- Colakoglu B, Alis D. Evaluation of lumbar multifidus muscle in patients with lumbar disc herniation: are complex quantitative MRI measurements needed? J Int Med Res 2019;47:3590-600. [Crossref] [PubMed]
- Janssen B, Voet N, Geurts A, van Engelen B, Heerschap A. Quantitative MRI reveals decelerated fatty infiltration in muscles of active FSHD patients. Neurology 2016;86:1700-7. [Crossref] [PubMed]
- Arpan I, Forbes SC, Lott DJ, Senesac CR, Daniels MJ, Triplett WT, Deol JK, Sweeney HL, Walter GA, Vandenborne K T. 2 mapping provides multiple approaches for the characterization of muscle involvement in neuromuscular diseases: a cross-sectional study of lower leg muscles in 5-15-year-old boys with Duchenne muscular dystrophy. NMR Biomed 2013;26:320-8. [Crossref] [PubMed]
- Mannil M, Burgstaller JM, Thanabalasingam A, Winklhofer S, Betz M, Held U, Guggenberger R. Texture analysis of paraspinal musculature in MRI of the lumbar spine: analysis of the lumbar stenosis outcome study (LSOS) data. Skeletal Radiol 2018;47:947-54. [Crossref] [PubMed]
- Yang Q, Yan D, Wang L, Li K, Liang W, Zhang W, Liu YD, Li XM, Blake GM, Konerth N, Cheng X, Tian W, Hind K. Muscle fat infiltration but not muscle cross-sectional area is independently associated with bone mineral density at the lumbar spine. Br J Radiol 2022;95:20210371. [Crossref] [PubMed]
- Sions JM, Coyle PC, Velasco TO, Elliott JM, Hicks GE. Multifidi Muscle Characteristics and Physical Function Among Older Adults With and Without Chronic Low Back Pain. Arch Phys Med Rehabil 2017;98:51-7. [Crossref] [PubMed]
- D'hooge R, Cagnie B, Crombez G, Vanderstraeten G, Dolphens M, Danneels L. Increased intramuscular fatty infiltration without differences in lumbar muscle cross-sectional area during remission of unilateral recurrent low back pain. Man Ther 2012;17:584-8. [Crossref] [PubMed]
- Li Q, Sun J, Cui X, Jiang Z, Li T. Analysis of correlation between degeneration of lower lumbar paraspinal muscles and spinopelvic alignment in patients with osteoporotic vertebral compression fracture. J Back Musculoskelet Rehabil 2017;30:1209-14. [Crossref] [PubMed]
- Ntilikina Y, Bahlau D, Garnon J, Schuller S, Walter A, Schaeffer M, Steib JP, Charles YP. Open versus percutaneous instrumentation in thoracolumbar fractures: magnetic resonance imaging comparison of paravertebral muscles after implant removal. J Neurosurg Spine 2017;27:235-41. [Crossref] [PubMed]
- Godi C, Ambrosi A, Nicastro F, Previtali SC, Santarosa C, Napolitano S, Iadanza A, Scarlato M, Natali Sora MG, Tettamanti A, Gerevini S, Cicalese MP, Sitzia C, Venturini M, Falini A, Gatti R, Ciceri F, Cossu G, Torrente Y, Politi LS. Longitudinal MRI quantification of muscle degeneration in Duchenne muscular dystrophy. Ann Clin Transl Neurol 2016;3:607-22. [Crossref] [PubMed]
- Greene KA, Tooze JA, Lenchik L, Weaver AA. Change in Lumbar Muscle Size and Composition on MRI with Long-Duration Spaceflight. Ann Biomed Eng 2022;50:816-24. [Crossref] [PubMed]
- Salari N, Fatahi B, Valipour E, Kazeminia M, Fatahian R, Kiaei A, Shohaimi S, Mohammadi M. Global prevalence of Duchenne and Becker muscular dystrophy: a systematic review and meta-analysis. J Orthop Surg Res 2022;17:96. [Crossref] [PubMed]
- Hoffman EP, Brown RH Jr, Kunkel LM. Dystrophin: the protein product of the Duchenne muscular dystrophy locus. Cell 1987;51:919-28. [Crossref] [PubMed]
- Kim HK, Merrow AC, Shiraj S, Wong BL, Horn PS, Laor T. Analysis of fatty infiltration and inflammation of the pelvic and thigh muscles in boys with Duchenne muscular dystrophy (DMD): grading of disease involvement on MR imaging and correlation with clinical assessments. Pediatr Radiol 2013;43:1327-35. [Crossref] [PubMed]
- Mah JK, Korngut L, Fiest KM, Dykeman J, Day LJ, Pringsheim T, Jette N. A Systematic Review and Meta-analysis on the Epidemiology of the Muscular Dystrophies. Can J Neurol Sci 2016;43:163-77. [Crossref] [PubMed]
- Udd B, Krahe R. The myotonic dystrophies: molecular, clinical, and therapeutic challenges. Lancet Neurol 2012;11:891-905. [Crossref] [PubMed]
- Lunt PW, Compston DA, Harper PS. Estimation of age dependent penetrance in facioscapulohumeral muscular dystrophy by minimising ascertainment bias. J Med Genet 1989;26:755-60. [Crossref] [PubMed]
- Solbakken G, Bjørnarå B, Kirkhus E, Nguyen B, Hansen G, Frich JC, Ørstavik K. MRI of trunk muscles and motor and respiratory function in patients with myotonic dystrophy type 1. BMC Neurol 2019;19:135. [Crossref] [PubMed]
- van der Maarel SM, Tawil R, Tapscott SJ. Facioscapulohumeral muscular dystrophy and DUX4: breaking the silence. Trends Mol Med 2011;17:252-8. [Crossref] [PubMed]
- Marra MA, Heskamp L, Mul K, Lassche S, van Engelen BGM, Heerschap A, Verdonschot N. Specific muscle strength is reduced in facioscapulohumeral dystrophy: An MRI based musculoskeletal analysis. Neuromuscul Disord 2018;28:238-45. [Crossref] [PubMed]
- Mul K, Lassche S, Voermans NC, Padberg GW, Horlings CG, van Engelen BG. What's in a name? The clinical features of facioscapulohumeral muscular dystrophy. Pract Neurol 2016;16:201-7. [Crossref] [PubMed]
- Mul K, Horlings CGC, Vincenten SCC, Voermans NC, van Engelen BGM, van Alfen N. Quantitative muscle MRI and ultrasound for facioscapulohumeral muscular dystrophy: complementary imaging biomarkers. J Neurol 2018;265:2646-55. [Crossref] [PubMed]
- Lareau-Trudel E, Le Troter A, Ghattas B, Pouget J, Attarian S, Bendahan D, Salort-Campana E. Muscle Quantitative MR Imaging and Clustering Analysis in Patients with Facioscapulohumeral Muscular Dystrophy Type 1. PLoS One 2015;10:e0132717. [Crossref] [PubMed]
- Rowland LP. Amyotrophic lateral sclerosis. Curr Opin Neurol 1994;7:310-5. [Crossref] [PubMed]
- Camacho-Soto A, Searles Nielsen S, Faust IM, Bucelli RC, Miller TM, Racette BA. Incidence of amyotrophic lateral sclerosis in older adults. Muscle Nerve 2022;66:289-96. [Crossref] [PubMed]
- Gerevini S, Agosta F, Riva N, Spinelli EG, Pagani E, Caliendo G, Chaabane L, Copetti M, Quattrini A, Comi G, Falini A, Filippi M. MR Imaging of Brachial Plexus and Limb-Girdle Muscles in Patients with Amyotrophic Lateral Sclerosis. Radiology 2016;279:553-61. [Crossref] [PubMed]
- Gloor M, Fasler S, Fischmann A, Haas T, Bieri O, Heinimann K, Wetzel SG, Scheffler K, Fischer D. Quantification of fat infiltration in oculopharyngeal muscular dystrophy: comparison of three MR imaging methods. J Magn Reson Imaging 2011;33:203-10. [Crossref] [PubMed]
- Gaeta M, Mileto A, Mazzeo A, Minutoli F, Di Leo R, Settineri N, Donato R, Ascenti G, Blandino A. MRI findings, patterns of disease distribution, and muscle fat fraction calculation in five patients with Charcot-Marie-Tooth type 2 F disease. Skeletal Radiol 2012;41:515-24. [Crossref] [PubMed]
- Fatehi F, Advani S, Okhovat AA, Ziaadini B, Shamshiri H, Nafissi S. Thigh and Leg Muscle MRI Findings in GNE Myopathy. J Neuromuscul Dis 2021;8:735-42. [Crossref] [PubMed]
- Reyes-Vidal CM, Mojahed H, Shen W, Jin Z, Arias-Mendoza F, Fernandez JC, Gallagher D, Bruce JN, Post KD, Freda PU. Adipose Tissue Redistribution and Ectopic Lipid Deposition in Active Acromegaly and Effects of Surgical Treatment. J Clin Endocrinol Metab 2015;100:2946-55. [Crossref] [PubMed]
- Freda PU, Shen W, Heymsfield SB, Reyes-Vidal CM, Geer EB, Bruce JN, Gallagher D. Lower visceral and subcutaneous but higher intermuscular adipose tissue depots in patients with growth hormone and insulin-like growth factor I excess due to acromegaly. J Clin Endocrinol Metab 2008;93:2334-43. [Crossref] [PubMed]
- Khaleeli AA, Edwards RH, Gohil K, McPhail G, Rennie MJ, Round J, Ross EJ. Corticosteroid myopathy: a clinical and pathological study. Clin Endocrinol (Oxf) 1983;18:155-66. [Crossref] [PubMed]
- Konieczny MR, Senyurt H, Krauspe R. Epidemiology of adolescent idiopathic scoliosis. J Child Orthop 2013;7:3-9. [Crossref] [PubMed]
- Good CR, Auerbach JD, O'Leary PT, Schuler TC. Adult spine deformity. Curr Rev Musculoskelet Med 2011;4:159-67. [Crossref] [PubMed]
- Kebaish KM, Neubauer PR, Voros GD, Khoshnevisan MA, Skolasky RL. Scoliosis in adults aged forty years and older: prevalence and relationship to age, race, and gender. Spine (Phila Pa 1976) 2011;36:731-6. [Crossref] [PubMed]
- Schwab F, Dubey A, Gamez L, El Fegoun AB, Hwang K, Pagala M, Farcy JP. Adult scoliosis: prevalence, SF-36, and nutritional parameters in an elderly volunteer population. Spine (Phila Pa 1976) 2005;30:1082-5. [Crossref] [PubMed]
- Suzuki K, Hasebe Y, Yamamoto M, Saita K, Ogihara S. Risk Factor Analysis for Fat Infiltration in the Lumbar Paraspinal Muscles in Patients With Lumbar Degenerative Diseases. Geriatr Orthop Surg Rehabil 2022;13:21514593211070688. [Crossref] [PubMed]
- Genant HK, Cooper C, Poor G, Reid I, Ehrlich G, Kanis J, et al. Interim report and recommendations of the World Health Organization Task-Force for Osteoporosis. Osteoporos Int 1999;10:259-64. [Crossref] [PubMed]
- LeBoff MS, Greenspan SL, Insogna KL, Lewiecki EM, Saag KG, Singer AJ, Siris ES. The clinician's guide to prevention and treatment of osteoporosis. Osteoporos Int 2022;33:2049-102. [Crossref] [PubMed]
- Whooley MA, Kip KE, Cauley JA, Ensrud KE, Nevitt MC, Browner WS. Depression, falls, and risk of fracture in older women. Study of Osteoporotic Fractures Research Group. Arch Intern Med 1999;159:484-90. [Crossref] [PubMed]
- McCarthy J, Davis A. Diagnosis and Management of Vertebral Compression Fractures. Am Fam Physician 2016;94:44-50.
- Habibi H, Takahashi S, Hoshino M, Takayama K, Sasaoka R, Tsujio T, Yasuda H, Kanematsu F, Kono H, Toyoda H, Ohyama S, Hori Y, Nakamura H. Impact of paravertebral muscle in thoracolumbar and lower lumbar regions on outcomes following osteoporotic vertebral fracture: a multicenter cohort study. Arch Osteoporos 2021;16:2. [Crossref] [PubMed]
- Kim JY, Chae SU, Kim GD, Cha MS. Changes of paraspinal muscles in postmenopausal osteoporotic spinal compression fractures: magnetic resonance imaging study. J Bone Metab 2013;20:75-81. [Crossref] [PubMed]
- Savage JW, Schroeder GD, Anderson PA. Vertebroplasty and kyphoplasty for the treatment of osteoporotic vertebral compression fractures. J Am Acad Orthop Surg 2014;22:653-64. [Crossref] [PubMed]
- Amin RM, Andrade NS, Neuman BJ. Lumbar Disc Herniation. Curr Rev Musculoskelet Med 2017;10:507-16. [Crossref] [PubMed]
- Pfirrmann CW, Metzdorf A, Zanetti M, Hodler J, Boos N. Magnetic resonance classification of lumbar intervertebral disc degeneration. Spine (Phila Pa 1976) 2001;26:1873-8. [Crossref] [PubMed]
- Urrutia J, Besa P, Campos M, Cikutovic P, Cabezon M, Molina M, Cruz JP. The Pfirrmann classification of lumbar intervertebral disc degeneration: an independent inter- and intra-observer agreement assessment. Eur Spine J 2016;25:2728-33. [Crossref] [PubMed]
- Zhong Y, Liu J, Zhou W, Yu D. Relationship between straight leg-raising test measurements and area of fat infiltration in multifidus muscles in patients with lumbar disc hernation. J Back Musculoskelet Rehabil 2020;33:57-63. [Crossref] [PubMed]
- Yazici A, Yerlikaya T. Investigation of the relationship between the clinical evaluation results of lumbar region muscles with cross-sectional area and fat infiltration. J Back Musculoskelet Rehabil 2022;35:1277-87. [Crossref] [PubMed]
- Yazici A, Yerlikaya T. The relationship between the degeneration and asymmetry of the lumbar multifidus and erector spinae muscles in patients with lumbar disc herniation with and without root compression. J Orthop Surg Res 2022;17:541. [Crossref] [PubMed]
- GAY JR. ABBOTT KH. Common whiplash injuries of the neck. J Am Med Assoc 1953;152:1698-704. [Crossref] [PubMed]
- Spitzer WO, Skovron ML, Salmi LR, Cassidy JD, Duranceau J, Suissa S, Zeiss E. Scientific monograph of the Quebec Task Force on Whiplash-Associated Disorders: redefining "whiplash" and its management. Spine (Phila Pa 1976) 1995;20:1S-73S.
- Treleaven J. Dizziness, Unsteadiness, Visual Disturbances, and Sensorimotor Control in Traumatic Neck Pain. J Orthop Sports Phys Ther 2017;47:492-502. [Crossref] [PubMed]
- Smith AC, Albin SR, Abbott R, Crawford RJ, Hoggarth MA, Wasielewski M, Elliott JM. Confirming the geography of fatty infiltration in the deep cervical extensor muscles in whiplash recovery. Sci Rep 2020;10:11471. [Crossref] [PubMed]
- Panjabi MM, Cholewicki J, Nibu K, Grauer J, Babat LB, Dvorak J. Critical load of the human cervical spine: an in vitro experimental study. Clin Biomech (Bristol, Avon) 1998;13:11-7. [Crossref] [PubMed]
- Elliott JM, Noteboom JT, Flynn TW, Sterling M. Characterization of acute and chronic whiplash-associated disorders. J Orthop Sports Phys Ther 2009;39:312-23. [Crossref] [PubMed]
- Abbott R, Peolsson A, West J, Elliott JM, Åslund U, Karlsson A, Leinhard OD. The qualitative grading of muscle fat infiltration in whiplash using fat and water magnetic resonance imaging. Spine J 2018;18:717-25. [Crossref] [PubMed]
- Elliott JM, Smith AC, Hoggarth MA, Albin SR, Weber KA 2nd, Haager M, Fundaun J, Wasielewski M, Courtney DM, Parrish TB. Muscle fat infiltration following whiplash: A computed tomography and magnetic resonance imaging comparison. PLoS One 2020;15:e0234061. [Crossref] [PubMed]
- Wallenberg RB, Belzer ML, Ramsey DC, Opel DM, Berkson MD, Gundle KR, Nagy ML, Boucher RJ, McCarron JA. MRI-based 3-dimensional volumetric assessment of fatty infiltration and muscle atrophy in rotator cuff tears. J Shoulder Elbow Surg 2022;31:1272-81. [Crossref] [PubMed]
- Goutallier D, Postel JM, Gleyze P, Leguilloux P, Van Driessche S. Influence of cuff muscle fatty degeneration on anatomic and functional outcomes after simple suture of full-thickness tears. J Shoulder Elbow Surg 2003;12:550-4. [Crossref] [PubMed]
- Thomazeau H, Boukobza E, Morcet N, Chaperon J, Langlais F. Prediction of rotator cuff repair results by magnetic resonance imaging. Clin Orthop Relat Res 1997;275-83.
- Lansdown DA, Lee S, Sam C, Krug R, Feeley BT, Ma CB A. Prospective, Quantitative Evaluation of Fatty Infiltration Before and After Rotator Cuff Repair. Orthop J Sports Med 2017;5:2325967117718537. [Crossref] [PubMed]
- Micevych PS, Garg A, Buchler LT, Marra G, Saltzman MD, Parrish TB, Seitz AL. Optimizing methods to quantify intramuscular fat in rotator cuff tears with normalization. Skeletal Radiol 2019;48:1111-8. [Crossref] [PubMed]
- Grimm A, Meyer H, Nickel MD, Nittka M, Raithel E, Chaudry O, Friedberger A, Uder M, Kemmler W, Quick HH, Engelke K. Evaluation of 2-point, 3-point, and 6-point Dixon magnetic resonance imaging with flexible echo timing for muscle fat quantification. Eur J Radiol 2018;103:57-64. [Crossref] [PubMed]
- Lee S, Lucas RM, Lansdown DA, Nardo L, Lai A, Link TM, Krug R, Ma CB. Magnetic resonance rotator cuff fat fraction and its relationship with tendon tear severity and subject characteristics. J Shoulder Elbow Surg 2015;24:1442-51. [Crossref] [PubMed]
- Michael JW, Schlüter-Brust KU, Eysel P. The epidemiology, etiology, diagnosis, and treatment of osteoarthritis of the knee. Dtsch Arztebl Int 2010;107:152-62. [Crossref] [PubMed]
- Gür H, Cakin N. Muscle mass, isokinetic torque, and functional capacity in women with osteoarthritis of the knee. Arch Phys Med Rehabil 2003;84:1534-41. [Crossref] [PubMed]
- Kumar D, Karampinos DC, MacLeod TD, Lin W, Nardo L, Li X, Link TM, Majumdar S, Souza RB. Quadriceps intramuscular fat fraction rather than muscle size is associated with knee osteoarthritis. Osteoarthritis Cartilage 2014;22:226-34. [Crossref] [PubMed]
- Teichtahl AJ, Wluka AE, Wang Y, Wijethilake PN, Strauss BJ, Proietto J, Dixon JB, Jones G, Forbes A, Cicuttini FM. Vastus medialis fat infiltration - a modifiable determinant of knee cartilage loss. Osteoarthritis Cartilage 2015;23:2150-7. [Crossref] [PubMed]
- Bartlett JL, Sumner B, Ellis RG, Kram R. Activity and functions of the human gluteal muscles in walking, running, sprinting, and climbing. Am J Phys Anthropol 2014;153:124-31. [Crossref] [PubMed]
- Zacharias A, Pizzari T, English DJ, Kapakoulakis T, Green RA. Hip abductor muscle volume in hip osteoarthritis and matched controls. Osteoarthritis Cartilage 2016;24:1727-35. [Crossref] [PubMed]
- Grimaldi A, Richardson C, Stanton W, Durbridge G, Donnelly W, Hides J. The association between degenerative hip joint pathology and size of the gluteus medius, gluteus minimus and piriformis muscles. Man Ther 2009;14:605-10. [Crossref] [PubMed]
- Sloan M, Premkumar A, Sheth NP. Projected Volume of Primary Total Joint Arthroplasty in the U.S., 2014 to 2030. J Bone Joint Surg Am 2018;100:1455-60. [Crossref] [PubMed]
- Cruz-Jentoft AJ, Bahat G, Bauer J, Boirie Y, Bruyère O, Cederholm T, Cooper C, Landi F, Rolland Y, Sayer AA, Schneider SM, Sieber CC, Topinkova E, Vandewoude M, Visser M, Zamboni M. Writing Group for the European Working Group on Sarcopenia in Older People 2 (EWGSOP2), and the Extended Group for EWGSOP2. Sarcopenia: revised European consensus on definition and diagnosis. Age Ageing 2019;48:16-31. [Crossref] [PubMed]
- Correa-de-Araujo R, Harris-Love MO, Miljkovic I, Fragala MS, Anthony BW, Manini TM. The Need for Standardized Assessment of Muscle Quality in Skeletal Muscle Function Deficit and Other Aging-Related Muscle Dysfunctions: A Symposium Report. Front Physiol 2017;8:87. [Crossref] [PubMed]
- Flor-Rufino C, Barrachina-Igual J, Pérez-Ros P, Pablos-Monzó A, Sanz-Requena R, Martínez-Arnau FM. Fat infiltration and muscle hydration improve after high-intensity resistance training in women with sarcopenia. A randomized clinical trial. Maturitas 2023;168:29-36. [Crossref] [PubMed]
- Teichtahl AJ, Urquhart DM, Wang Y, Wluka AE, Wijethilake P, O'Sullivan R, Cicuttini FM. Fat infiltration of paraspinal muscles is associated with low back pain, disability, and structural abnormalities in community-based adults. Spine J 2015;15:1593-601. [Crossref] [PubMed]
- Tretiakov PS, Lafage R, Smith JS, Line BG, Diebo BG, Daniels AH, et al. Calibration of a comprehensive predictive model for the development of proximal junctional kyphosis and failure in adult spinal deformity patients with consideration of contemporary goals and techniques. J Neurosurg Spine 2023;39:311-9. [Crossref] [PubMed]
- Katsuura Y, Lafage R, Kim HJ, Smith JS, Line B, Shaffrey C, Burton DC, Ames CP, Mundis GM Jr, Hostin R, Bess S, Klineberg EO, Passias PG, Lafage VInternational Spine Study Group (ISSG). Alignment Targets, Curve Proportion and Mechanical Loading: Preliminary Analysis of an Ideal Shape Toward Reducing Proximal Junctional Kyphosis. Global Spine J 2022;12:1165-74. [Crossref] [PubMed]
- Lafage R, Beyer G, Schwab F, Klineberg E, Burton D, Bess S, Kim HJ, Smith J, Ames C, Hostin R, Khalife M, Shaffrey C, Mundis G, Lafage V. Risk Factor Analysis for Proximal Junctional Kyphosis After Adult Spinal Deformity Surgery: A New Simple Scoring System to Identify High-Risk Patients. Global Spine J 2020;10:863-70. [Crossref] [PubMed]
- El Rachkidi R, Massaad A, Saad E, Kawkabani G, Semaan K, Abi Nahed J, Ghanem I, Lafage V, Skalli W, Assi A. Spinopelvic Adaptations in Standing and Sitting Positions in Patients With Adult Spinal Deformity. Cureus 2022;14:e28113. [Crossref] [PubMed]
- Semaan K, Rachkidi R, Saad E, Massaad A, Kawkabani G, Saliby RM, Mekhael M, Abi Karam K, Fakhoury M, Jaber E, Ghanem I, Skalli W, Lafage V, Assi A. Alterations of gait kinematics depend on the deformity type in the setting of adult spinal deformity. Eur Spine J 2022;31:3069-80. [Crossref] [PubMed]
- Saad E, Semaan K, Kawkabani G, Massaad A, Salibv RM, Mekhael M, Fakhoury M, Karam KA, Jaber E, Ghanem I, Lafage V, Skalli W, Rachkidi R, Assi A. Alteration of the Sitting and Standing Movement in Adult Spinal Deformity. Front Bioeng Biotechnol 2021;9:751193. [Crossref] [PubMed]
- Lovecchio F, Lafage R, Elysee JC, Huang A, Ang B, Bannwarth M, Kim HJ, Schwab F, Lafage V. The utility of supine radiographs in the assessment of thoracic flexibility and risk of proximal junctional kyphosis. J Neurosurg Spine 2021;35:110-6. [Crossref] [PubMed]
- Duan PG, Mummaneni PV, Guinn JMV, Rivera J, Berven SH, Chou D. Is the Goutallier grade of multifidus fat infiltration associated with adjacent-segment degeneration after lumbar spinal fusion? J Neurosurg Spine 2020;34:190-5. [Crossref] [PubMed]