Multi-echo three-dimensional (3D) Dixon sequence combined with disodium gadolinium for risk stratification of advanced fibrosis in metabolic dysfunction-associated steatotic liver disease
Introduction
Metabolic dysfunction-associated steatotic liver disease (MASLD) is the most common cause of chronic liver disease, and the incidence of MASLD in Asian adults ranges from 15% to 30% (1). The presence of fibrosis is an important prognostic factor for MASLD and is associated (2,3) with the ultimate outcome and mortality of liver disease. Monitoring the progression of liver fibrosis and risk assessment is particularly important for developing appropriate treatment (4) during the disease course. Pathological biopsy is the gold standard for the diagnosis of MASLD, however, it is not suitable for MASLD screening and follow-up due to its invasiveness, sampling error of biopsy tissue samples, and poor reproducibility (5). Among the noninvasive imaging diagnosis methods, magnetic resonance imaging (MRI) is recognized as the best imaging method. The accuracy of MRI proton density fat fraction (MRI-PDFF) in quantifying fat is high. magnetic resonance elastography (MRE) has been confirmed as a biomarker of liver fibrosis, and has high application value in the diagnosis of non-alcoholic fatty liver disease (NAFLD) and tracking of disease progression (1,6).
Gadolinum ethoxybenzyl diethylenetriaminepentaacetic acid (Gd-EOB-DTPA) is a type of liver-cell-specific contrast agent that can be absorbed by liver cells specifically after intravenous injection (7). This agent can be used to quantitatively evaluate hepatic function status by taking advantage of the fact that hepatocytes can absorb and increase the contrast agent’s effect at different rates and degrees (8). A study has shown that Gd-EOB-DTPA has high value in liver-enhanced MRI, the change in liver function can be reflected by the signal-intensity enhancement (SE) calculated after measuring the signal intensity (SI) of the liver during the pre-contrast scan and hepatobiliary phase, respectively (8).
Fat fraction (FF) map and R2* map can be generated by multi-echo three-dimensional (3D) dixon sequence (Q-dixon). FF map can quantitatively analyze liver fat deposition, and R2* is the reciprocal of T2*, reflecting the attenuation of T2*, which is commonly used for the quantification of iron deposition. Gd-EOB-DTPA can shorten tissue T2* relaxation time, and measuring the corresponding T2* value using T2* weighted images can reflect the functional changes of the liver (9). If the change of R2* before and after enhancement can reflect the situation of liver fibrosis, then Q-dixon can assess steatosis, iron deposition and liver fibrosis in a one-stop manner, which has important application value for the evaluation of MASLD. This study analyzed the difference of T2* relaxation-time enhancement (RE) in patients with liver fibrosis at different risk levels, and explored the potential application value of Q-dixon combined with Gd-EOB-DTPA in the risk stratification of liver fibrosis in MASLD. We present this article in accordance with the STARD reporting checklist (available at https://qims.amegroups.com/article/view/10.21037/qims-24-555/rc).
Methods
General information
This retrospective study included 138 MASLD patients who underwent Gd-EOB-DTPA MRI examination in The First Affiliated Hospital of Chongqing Medical University from June 2020 to December 2021. The criteria for MASLD were that the FF of the patient’s MRI was more than 5%, accompanied by at least 1 abnormality in body mass index (BMI), triglycerides (TG), high-density lipoprotein (HDL), blood pressure (BP), and blood glucose (GLU). Exclusion criteria were as follows: history of liver or biliary tract surgery, severe ascites, liver tumors or severe diffuse lesions, long history of alcohol consumption, long use of drugs that can lead to fatty liver, history of viral hepatitis or other chronic hepatitis, incomplete examination data, severe image artifacts due to poor breath holding, and incomplete real-time shear-wave elastic imaging of the liver. Finally, 90 MASLD patients were included, including 52 males and 38 females, aged 48.53±13.12 years (Figure 1). The study was conducted in accordance with the Declaration of Helsinki (as revised in 2013). The study was approved by the Ethics Committee of The First Affiliated Hospital of Chongqing Medical University (No. 2021-129), and individual consent for this retrospective analysis was waived.
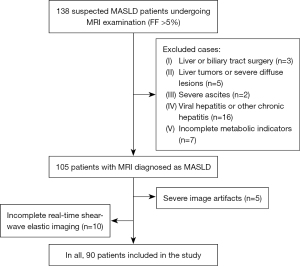
Equipment and methods
MR scanner (MAGNETOM Skyra, 3.0T) with an 18-channel body matrix coil and a 32-channel spine matrix coil was used. After a multi-echo 3D chemical shift imaging sequence (Q-Dixon) and gradient-echo T1WI (Vibe-Dixon), a multiphase dynamic enhanced scan was performed, followed by a free-breath diffusion weighted echo-planar imaging sequence and a diaphragm-navigated T2-weighted fast-spin-echo sequence. Finally, Q-Dixon and Vibe-Dixon sequences were used again to scan the hepatobiliary phase. The Vibe-Dixon sequence parameters were as follows: echo time (TE) =2.56 ms, repetition time (TR) =4.66 ms, repetition angle =12°, field of view (FOV) =380 mm × 380 mm, layer thickness =3 mm, 88 layers, voxel size =1.2×1.2×3 mm, excitation number =1, and parallel acquisition technique (PAT) factor =4. Q-Dixon is a gradient-echo sequence of six TEs with the following parameters: TR/TE1/ΔTE =9/1.05/1.4 ms; receiver bandwidth =1,080 kHz; FOV =45 cm; voxel =1.4×1.4×3.5 mm; layer thickness =3.5 mm; 64 layers, flip angle =4, scan time =13 s, excitation time =1, and PAT =4.
Gd-EOB-DTPA (Primovist; Bayer, Berlin, Germany) and a high-pressure syringe (Spectris MR, MEDRAD) were used for bolus injection. The injection flow rate was 1 mL/s, and the total injection volume was 0.1 mL/kg. 20 mL of normal saline was used to flush the tube for dynamic scanning. Vibe Dixon sequence was used to obtain late arterial phase, portal venous phase, equilibrium phase and delayed phase images at 24, 50, 70 and 180 s after contrast injection. The scanning Q-Dixon sequence and Vibe-Dixon sequence were triggered in the hepatobiliary phase with a delay of 20 min, and the actual acquisition delay time range was 20–22 min.
Blood fibrosis test
The non-alcoholic fatty liver Disease Fibrosis Score (NAFLD fibrosis score; NFS) formula (10) was calculated as follows:
The Fibros-4 index (FIB4) formula (11) was calculated as follows:
Liver stiffness measurement (LSM)
The LSM was obtained by performing real-time shear-wave elastography (SWE) (Aixplorer; Supersonic Imagine, France).
Liver-fibrosis risk stratification
The degree of fibrosis risk was assessed according to the two-step approach (4,12) proposed in the guidelines of the European Association for the Study of the Liver (EASL), which uses either NFS or FIB-4 as the first-line assessment: FIB-4 <1.3 or NFS <−1.455 is low risk; FIB-4 <1.3 to approximately 3.25 or NFS =−1.455 to approximately 0.672 is moderate risk, and FIB-4 >3.25 or NFS >0.672 is high risk. When the first-line assessment suggested medium or high risk, LSM was performed for the second-line assessment: LSM <8 kPa is a low risk, 8 kPa to approximately 10 kPa is a moderate risk, and ≥10 kPa is a high risk of liver fibrosis.
Image analysis
The Vibe-Dixon and Q-Dixon images of the pre-contrast scan and hepatobiliary stage were analyzed by two MR physicians who used a Siemens Syngo.via postprocessing workstation. Three regions of interest (ROIs) were placed in the right posterior lobe of the liver for measurement. The ROI area was 180 to 220 mm2, and focal lesions, bile ducts, and blood vessels were avoided if possible during measurement (Figure 2). R2* values were measured in the Q-Dixon sequence, and SI was measured in the Vibe-Dixon sequence. The ROI was copied and pasted to ensure consistency of the measurement positions before and after enhancement.
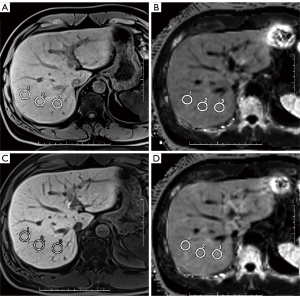
Statistical analysis
SPSS (IBM SPSS statistics for windows; IBM Corp., Armonk, NY, USA) was used for statistical analysis. All measured data were expressed as mean ± standard deviation. The interobserver correlation coefficient (ICC) was used to evaluate the consistency between observers. ICC value >0.80 indicated very good consistency. The differences between SE and RE of liver fibrosis at different risk levels were analyzed. The K-S test was used for normality test, and the single factor t-test was used when the data were normally distributed; When data were not normally distributed, Mann Whitney U test was used. The area under the curve (AUC) of the receiver operating characteristic (ROC) curve was analyzed by Delong test. The point closest to the upper left corner of the ROC curve was the best critical point, and the corresponding coordinates represented the sensitivity and specificity, respectively. A two-sided test P<0.05 indicated statistical significance.
Results
Among the 90 patients ultimately included, 55 cases (61.1%) belonged to the low-risk group of liver fibrosis, and 35 cases (38.9%) belonged to the medium and high-risk groups. The comparison of general information for patients with different risk groups is shown in Table 1. There is a significant difference in the proportion of diabetes mellitus (DM) and hypertension (P=0.036, 0.027), and there is a significant difference in TG between the two groups of patients (P=0.002). There is no significant difference in other general information (P>0.05).
Table 1
Characteristics | Low risk fibrosis | Medium-to-high risk fibrosis | P value |
---|---|---|---|
Number | 55 (61.11) | 35 (38.89) | – |
Age (years) | 48.18±13.36 | 45.66±12.48 | 0.468 |
Male | 32 (58.18) | 22 (62.86) | 0.506 |
BMI (kg/m2) | 25.76±3.02 | 26.98±2.84 | 0.235 |
Hypertension | 8 (14.55) | 12 (34.28) | 0.036* |
DM | 6 (10.90) | 13 (37.14) | 0.027* |
TG (mmol/L) | 1.78±0.86 | 2.21±1.02 | 0.002* |
HDL (mmol/L) | 1.19±0.36 | 1.08±0.29 | 0.356 |
Data are represented as mean ± standard deviation or number (%). *, indicates significant differences (P<0.05). BMI, body mass index; DM, diabetes mellitus; TG, triglyceride; HDL, high-density lipoprotein.
Interobserver assessment of objective data measurement revealed excellent agreement [ICC 0.987; 95% confidence interval (CI): 0.970 to 0.994]. The FF value of pre contrast scan in 90 patients suggested steatosis, with an average value of 11.49 (5.19, 34.36); the average R2* value was 71.19 (29.1, 369.38), of which 11 cases suggested iron deposition. As shown in Table 2, the mean values of RE and SE in the low-risk group were 1.23±0.15 and 1.57±0.23, whereas the values of RE and SE in the medium-to-high risk group decreased significantly to 0.99±0.09 and 1.38±0.21, respectively, with significant differences (P<0.01).
Table 2
Index | Low risk | Medium-to-high risk | T-value | P value |
---|---|---|---|---|
RE | 1.23±0.15 | 0.99±0.09 | 8.34 | <0.01 |
SE | 1.57±0.23 | 1.38±0.21 | 3.98 | <0.01 |
Data are represented as mean ± standard deviation. P<0.05 was significant. RE, relaxation-time enhancement; SE, signal-intensity enhancement.
The ROC curve data are shown in Figure 3. The curves for identifying liver-fibrosis risk stratification by RE and SE values are all above the reference line, as shown in Table 3. The AUC value corresponding to RE is 0.922, the diagnostic value of RE for risk is very high, and the corresponding optimal cutoff value is 0.713. The sensitivity was 0.852 and the specificity was 0.861. The AUC value corresponding to SE was 0.724, the diagnostic value of SE for risk is high, and the corresponding optimal cutoff value was 0.352, the sensitivity was 0.519, and the specificity was 0.833. The AUC difference between RE and SE for the predictive value of different risk assessments was 0.198, and the 95% CI of the difference was 0.084–0.312. The Delong test showed that P=0.001, the difference was significant, so there was a significant difference between the two indicators for the predictive effect of different risk assessments, and RE was significantly better than SE.
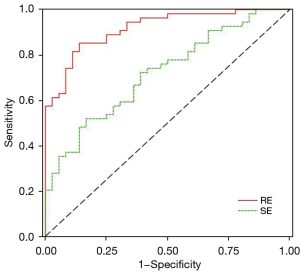
Table 3
Index | Optimal cutoff value | Cutoff | AUC | Sensitivity | Specificity |
---|---|---|---|---|---|
RE | 0.713 | 1.070 | 0.922 | 0.852 | 0.861 |
SE | 0.352 | 1.580 | 0.724 | 0.519 | 0.833 |
AUC, area under the curve; RE, relaxation-time enhancement; SE, signal-intensity enhancement.
Discussion
In this study, we used a two-step approach to stratify the liver-fibrosis risk, with reference to NFS/FIB-4 and LSM, and divided the study subjects into a medium-to-high-risk fibrosis group and a low-risk group. Both NFS (13) and FIB-4 (14,15) indices have previously been shown to be stable, and LSM is used clinically to monitor the severity of liver fibrosis and is recommended (4,16) in the guidelines for the diagnosis and treatment of MASLD. Risk assessment of fibrosis in MASLD is essential for follow-up monitoring of patients. Most conventional methods for staging evaluation of liver fibrosis involve needle biopsy, and its invasive nature and patient acceptance limit its clinical application. In this context, studies on noninvasive evaluation methods have been widely conducted.
Gd-EOB-DTPA is formed by chelating the lipophilic EOB group on the basis of the structure of the traditional extracellular contrast agent Gd-DTPA, which increases the tissue T1 relaxation rate and enhances the liver MR signal (17,18). Gd-EOB-DTPA can also shorten the T2* relaxation time of tissues (9), and R2* is the reciprocal of T2*, showing an increase in R2*. Studies have shown that the decreased level of adenosine triphosphate (ATP) in fibrotic or damaged liver cells reduces (19,20) the uptake of EOB groups by hepatocytes, which is manifested by the decreased SI and R2* of liver parenchyma in MRI hepatobiliary stage images. This study strictly implemented the breath holding training of patients before scanning to avoid the delay time difference caused by Q-dixon and vibe Dixon repeated scanning in the 20-minute hepatobiliary phase. The results showed that the RE and SE values were significantly lower for the patients in the medium- to high-risk group than that in the low-risk group of liver fibrosis, indicating that the signal changes and magnetic relaxation-time changes based on Gd-EOB-DTPA could reflect the differences in the degree of liver fibrosis among different risk groups.
The ROC curve for the efficacy of RE and SE values in differentiating risk stratification of liver fibrosis showed that the curves of both indexes were above the reference line, indicating good diagnostic value for risk differentiation. However, the sensitivity of SE was low, only 0.519, mainly because SE is an index calculated on the basis of signal strength, which is a relative value. It cannot directly reflect the concentration of contrast agent and tissue characteristics, and it is easily affected by many factors, such as scanning equipment and receiving coils. The results showed that the accuracy, sensitivity, and specificity for identification of liver fibrosis risk were higher for RE than for SE, and the diagnostic efficiency was higher. Because RE is an index calculated based on R2* map, which can quantitatively analyze the T2* relaxation time difference caused by the ability of hepatocytes to take up Gd-EOB-DTPA, the relaxation measurement can more objectively reflect the characteristics of tissues, making the results reproducible.
The degree of hepatic steatosis and fibrosis is important for the progression of MASLD or other liver diseases complicated with MASLD. Noninvasive diagnosis methods of liver fibrosis mainly include serum and imaging examination. Serum indicators are widely used in clinical practice, but their sensitivity and specificity for early fibrosis are low. The diagnostic efficacy of SWE is better than serum indicators, but obesity has a greater impact on it. MRE is considered to be a more accurate method to evaluate liver fibrosis, but it requires additional mechanical wave driving device, has high requirements for the hardware of the institution, and has limitations in clinical promotion. The multi-echo 3D chemical shift imaging sequence is equipped on most 1.5T or 3.0TMR scanners produced in recent years. The sequence names of different manufacturers are different, it is routinely used to quantitatively evaluate hepatic steatosis and iron deposition. This study proposed to combine Gd-EOB-DTPA to calculate the RE value to evaluate the risk stratification of liver fibrosis, which is an expansion of the application value of this sequence for obtaining more quantitative information. The feasibility of T1 map combined with Gd-EOB-DTPA in the assessment of liver fibrosis has been confirmed (21),but study did not compare the accuracy of T1 map and R2* map in the diagnosis of liver fibrosis, which is also the content of our future research.
The limitations of this study were as follows: first, the cases in this study usually had mild clinical symptoms, due to the study design, liver biopsy was not possible. We used magnetic resonance fat quantification as a noninvasive diagnostic standard for steatosis, and used the two-step method (4,22) proposed in the guidelines of the EASL to group the fibrosis risk of MASLD patients, the experimental results may be different from other pathological grouping studies. Second, R2* values were in the normal range in most of the cases in this study, and measurements of R2* values may be biased in patients with iron deposits. Third, the time interval between image acquisition in the normal scan and the hepatobiliary phase was relatively long, which may be caused by the inconsistency in the breath-holding state, resulting in inconsistent selection of layers, and there was also some deviation in the placement of the three ROIs. To reduce the error as much as possible, we adopted the copy-paste ROI method before and after enhancement. We repeatedly trained patients to practice end-expiratory breath-holding to the same degree in each breath-holding sequence to minimize positioning errors.
Conclusions
In summary, magnetic relaxation-time enhancement was found to have higher diagnostic effectiveness for identification of liver fibrosis risk. A multi-echo water-lipid separation 3D sequence combined with Gd-EOB-DTPA provided a simplified assessment of MASLD steatosis and fibrosis, with good clinical application value.
Acknowledgments
Funding: This study was supported by
Footnote
Reporting Checklist: The authors have completed the STARD reporting checklist. Available at https://qims.amegroups.com/article/view/10.21037/qims-24-555/rc
Conflicts of Interest: All authors have completed the ICMJE uniform disclosure form (available at https://qims.amegroups.com/article/view/10.21037/qims-24-555/coif). The authors have no conflicts of interest to declare.
Ethical Statement: The authors are accountable for all aspects of the work in ensuring that questions related to the accuracy or integrity of any part of the work are appropriately investigated and resolved. The study was conducted in accordance with the Declaration of Helsinki (as revised in 2013). The study was approved by the Ethics Committee of The First Affiliated Hospital of Chongqing Medical University (No. 2021-129), and individual consent for this retrospective analysis was waived.
Open Access Statement: This is an Open Access article distributed in accordance with the Creative Commons Attribution-NonCommercial-NoDerivs 4.0 International License (CC BY-NC-ND 4.0), which permits the non-commercial replication and distribution of the article with the strict proviso that no changes or edits are made and the original work is properly cited (including links to both the formal publication through the relevant DOI and the license). See: https://creativecommons.org/licenses/by-nc-nd/4.0/.
References
- Rockey DC, Bell PD, Hill JA. Fibrosis--a common pathway to organ injury and failure. N Engl J Med 2015;372:1138-49. [Crossref] [PubMed]
- Angulo P, Kleiner DE, Dam-Larsen S, Adams LA, Bjornsson ES, Charatcharoenwitthaya P, Mills PR, Keach JC, Lafferty HD, Stahler A, Haflidadottir S, Bendtsen F. Liver Fibrosis, but No Other Histologic Features, Is Associated With Long-term Outcomes of Patients With Nonalcoholic Fatty Liver Disease. Gastroenterology 2015;149:389-97.e10. [Crossref] [PubMed]
- Patel PJ, Cheng JC, Banh X, Gracen L, Radford-Smith D, Hossain F, Horsfall LU, Hayward KL, Williams S, Johnson T, Brown NN, Saad N, Stuart KA, Russell AW, Valery PC, Clouston AD, Irvine KM, Bernard A, Powell EE. Clinically Significant Fibrosis Is Associated With Longitudinal Increases in Fibrosis-4 and Nonalcoholic Fatty Liver Disease Fibrosis Scores. Clin Gastroenterol Hepatol 2020;18:710-8.e4. [Crossref] [PubMed]
- European Association for Study of Liver. Asociacion Latinoamericana para el Estudio del Higado. EASL-ALEH Clinical Practice Guidelines: Non-invasive tests for evaluation of liver disease severity and prognosis. J Hepatol 2015;63:237-64. [Crossref] [PubMed]
- Festi D, Schiumerini R, Marasco G, Scaioli E, Pasqui F, Colecchia A. Non-invasive diagnostic approach to non-alcoholic fatty liver disease: current evidence and future perspectives. Expert Rev Gastroenterol Hepatol 2015;9:1039-53. [Crossref] [PubMed]
- Castera L, Friedrich-Rust M, Loomba R. Noninvasive Assessment of Liver Disease in Patients With Nonalcoholic Fatty Liver Disease. Gastroenterology 2019;156:1264-81.e4. [Crossref] [PubMed]
- Murakami T, Sofue K, Hori M. Diagnosis of Hepatocellular Carcinoma Using Gd-EOB-DTPA MR Imaging. Magn Reson Med Sci 2022;21:168-81. [Crossref] [PubMed]
- Araki K, Harimoto N, Kubo N, Watanabe A, Igarashi T, Tsukagoshi M, Ishii N, Tsushima Y, Shirabe K. Functional remnant liver volumetry using Gd-EOB-DTPA-enhanced magnetic resonance imaging (MRI) predicts post-hepatectomy liver failure in resection of more than one segment. HPB (Oxford) 2020;22:318-27. [Crossref] [PubMed]
- Katsube T, Okada M, Kumano S, Imaoka I, Kagawa Y, Hori M, Ishii K, Tanigawa N, Imai Y, Kudo M, Murakami T. Estimation of liver function using T2* mapping on gadolinium ethoxybenzyl diethylenetriamine pentaacetic acid enhanced magnetic resonance imaging. Eur J Radiol 2012;81:1460-4. [Crossref] [PubMed]
- Vilar-Gomez E, Chalasani N. Non-invasive assessment of non-alcoholic fatty liver disease: Clinical prediction rules and blood-based biomarkers. J Hepatol 2018;68:305-15. [Crossref] [PubMed]
- Chan WK, Treeprasertsuk S, Goh GB, Fan JG, Song MJ, Charatcharoenwitthaya P, Duseja A, Dan YY, Imajo K, Nakajima A, Ho KY, Goh KL, Wong VW. Optimizing Use of Nonalcoholic Fatty Liver Disease Fibrosis Score, Fibrosis-4 Score, and Liver Stiffness Measurement to Identify Patients With Advanced Fibrosis. Clin Gastroenterol Hepatol 2019;17:2570-80.e37. [Crossref] [PubMed]
- Blond E, Disse E, Cuerq C, Drai J, Valette PJ, Laville M, Thivolet C, Simon C, Caussy C. EASL-EASD-EASO clinical practice guidelines for the management of non-alcoholic fatty liver disease in severely obese people: do they lead to over-referral? Diabetologia 2017;60:1218-22. [Crossref] [PubMed]
- Angulo P, Hui JM, Marchesini G, Bugianesi E, George J, Farrell GC, Enders F, Saksena S, Burt AD, Bida JP, Lindor K, Sanderson SO, Lenzi M, Adams LA, Kench J, Therneau TM, Day CP. The NAFLD fibrosis score: a noninvasive system that identifies liver fibrosis in patients with NAFLD. Hepatology 2007;45:846-54. [Crossref] [PubMed]
- Sterling RK, Lissen E, Clumeck N, Sola R, Correa MC, Montaner J. S Sulkowski M, Torriani FJ, Dieterich DT, Thomas DL, Messinger D, Nelson M; Mark Nelson; APRICOT Clinical Investigators. Development of a simple noninvasive index to predict significant fibrosis in patients with HIV/HCV coinfection. Hepatology 2006;43:1317-25. [Crossref] [PubMed]
- Sumida Y, Yoneda M, Hyogo H, Itoh Y, Ono M, Fujii H, Eguchi Y, Suzuki Y, Aoki N, Kanemasa K, Fujita K, Chayama K, Saibara T, Kawada N, Fujimoto K, Kohgo Y, Yoshikawa T, Okanoue TJapan Study Group of Nonalcoholic Fatty Liver Disease (JSG-NAFLD). Validation of the FIB4 index in a Japanese nonalcoholic fatty liver disease population. BMC Gastroenterol 2012;12:2. [Crossref] [PubMed]
- Suzuki K, Yoneda M, Imajo K, Kirikoshi H, Nakajima A, Maeda S, Saito S. Transient elastography for monitoring the fibrosis of non-alcoholic fatty liver disease for 4 years. Hepatol Res 2013;43:979-83. [Crossref] [PubMed]
- Van Beers BE, Pastor CM, Hussain HK. Primovist, Eovist: what to expect? J Hepatol 2012;57:421-9. [Crossref] [PubMed]
- Wang C, Yuan XD, Wu N, Sun WR, Tian Y. Optimization of hepatobiliary phase imaging in gadoxetic acid-enhanced magnetic resonance imaging: a narrative review. Quant Imaging Med Surg 2023;13:1972-82. [Crossref] [PubMed]
- Verloh N, Utpatel K, Haimerl M, Zeman F, Fellner C, Fichtner-Feigl S, Teufel A, Stroszczynski C, Evert M, Wiggermann P. Liver fibrosis and Gd-EOB-DTPA-enhanced MRI: A histopathologic correlation. Sci Rep 2015;5:15408. [Crossref] [PubMed]
- Lee S, Choi D, Jeong WK. Hepatic enhancement of Gd-EOB-DTPA-enhanced 3 Tesla MR imaging: Assessing severity of liver cirrhosis. J Magn Reson Imaging 2016;44:1339-45. [Crossref] [PubMed]
- Breit HC, Vosshenrich J, Heye T, Gehweiler J, Winkel DJ, Potthast S, Merkle EM, Boll DT. Assessment of hepatic function employing hepatocyte specific contrast agent concentrations to multifactorially evaluate fibrotic remodeling. Quant Imaging Med Surg 2023;13:4284-94. [Crossref] [PubMed]
- Chan WK, Nik Mustapha NR, Mahadeva S. A novel 2-step approach combining the NAFLD fibrosis score and liver stiffness measurement for predicting advanced fibrosis. Hepatol Int 2015;9:594-602. [Crossref] [PubMed]