Assessment of the re-entry tear index as a prognostic indicator for renal perfusion improvement after thoracic endovascular aortic repair in patients with acute and subacute Stanford type b aortic dissection with renal artery involvement
Introduction
Aortic dissection is the most prevalent acute aortic syndrome and is associated with a relatively high mortality rate (1,2). The International Registry of Acute Aortic Dissection (IRAD) reported that the incidence of aortic dissection has been estimated to be from 5 to 30 per 1 million people per year. Overall, in-hospital mortality is 27.4%, the mortality of patients with type A dissection managed surgically is 26%, and among those not receiving surgery, the mortality is 58%. The mortality of patients with type B dissection treated medically is 10.7%, surgery is performed in 20% of patients with type B dissection, and the mortality of those with type B dissection who underwent surgery is 31.4% (3).
The extensively employed Stanford classification delineates aortic dissection by differentiating between involvement with the ascending aorta (type A) and the absence of such involvement (type B) (4). In cases of complicated type B aortic dissection, thoracic endovascular aortic repair (TEVAR) is the preferred therapeutic approach (5). Among the visceral branches affected in aortic dissection, renal artery involvement is the most common, and renal malperfusion significantly contributes to an unfavorable prognosis in affected patients (6). The efficacy of vascular repair hinges on the identification of the primary entry tear of aortic dissection, with the size and location of re-entry tears exerting a direct impact on the enhancement of postoperative blood flow in branch arteries originating from the false lumen (7). Despite this, prior studies have not conducted a comprehensive analysis encompassing both morphology and hemodynamics, nor have they quantitatively assessed the location and size of re-entry tears. Clinical studies have suggested that the distal re-entry tears play a significant role in the remodeling of the true lumen and thrombosis of the false lumen following TEVAR. Of particular note, the tear count, area, and maximum distance of re-entry tears have been suggested as the risk factors of incomplete thrombosis in the post-TEVAR false lumen (8,9). As re-entry tears are the only channel connecting the true lumen and false lumen, they are a key determinant of the postoperative treatment effect received by patients. A computational fluid dynamics simulation studies found out that an increase in the number of re-entry tears can lead to an increase in the total area of re-entry tears. When tear count and total area are fixed, this may increase the distance between tears, which will lead to an unfavorable hemodynamic environment for the initiation and formation of thrombosis, resulting in a decrease in the thrombosis rate in the false lumen following TEVAR (10). Consequently, in this study, we aimed to ascertain whether a comprehensive quantitative analysis of the re-entry tear index (RTI) can serve as a predictive indicator for postoperative improvement in renal perfusion. The RTI for each re-entry tear was calculated as the ratio of the maximum diameter of the re-entry tear (b) to the distance between the re-entry tear and the involved renal artery (a), as depicted in Figure 1. We present this article in accordance with the STROBE reporting checklist (available at https://qims.amegroups.com/article/view/10.21037/qims-24-206/rc).
Methods
Study design and patients
Patients diagnosed with acute or subacute type B aortic dissection with concomitant renal artery involvement who underwent TEVAR at Anzhen Hospital in Beijing from October 2017 to November 2021 were enrolled in this study. The inclusion criteria were as follows: (I) diagnosis of acute (1–14 days) or subacute (15–90 days) Stanford type B aortic dissection based on established criteria (11,12); (II) specific classifications of type B aortic dissection, namely B38, B39, B310, and B311 (13); (III) presence of renal artery involvement, defined as the extension of aortic dissection to the level of the renal artery and beyond; (IV) a scheduled TEVAR procedure; and (V) the renal arteries affected are predominantly or entirely supplied by the false lumen. Meanwhile, the exclusion criteria were as follows: (I) a history of renal disease; (II) prior aortic surgery; (III) presence of coronary artery disease or cardiac insufficiency; (IV) chronic obstructive pulmonary disease; or (V) autoimmune disorders. A total of 137 eligible patients, comprising of 93 males and 44 females, were included in the study. This study was conducted in accordance with the Declaration of Helsinki (as revised in 2013) and was approved by the ethics committee of Beijing Anzhen Hospital (No. 2023123X). Informed consent was obtained from all participating patients.
Procedures
Clinical data
Medical records, encompassing demographic data, clinical characteristics, medical history, pharmacological history, laboratory findings, imaging results, and treatment strategies at discharge, were collected. Body mass index (BMI) was calculated by dividing the weight in kilograms by the square of the height in meters (kg/m2). Hypertension was defined as blood pressure ≥140/90 mmHg without the use of antihypertensive drugs (14). Dyslipidemia was defined as fasting levels of triglycerides >1.7 mmol/L, total cholesterol >5.2 mmol/L, low-density lipoprotein cholesterol (LDL-C) >3.4 mmol/L, and/or high-density lipoprotein cholesterol (HDL-C) <1.0 mmol/L (15). Diabetes mellitus was defined as blood glucose levels ≥7.0 mmol/L under fasting conditions, ≥11.1 mmol/L at 2 hours postmeal or at a random time, and/or levels of glycosylated hemoglobin (A1C) ≥6.5 (16). Smoking history was classified as either being a former smoker or a current smoker. The estimated glomerular filtration rate (eGFR) was calculated using the following formula: 186 × serum creatinine (mg/dL) − 1.154 × age − 0.203 × 0.742 (if female) (mL/min/1.73 m2) (17). Biochemical tests were conducted using standard methods in an AU5400 chemistry analyzer (Beckman Coulter, Danaher, Brea, CA, USA), and serum creatinine measurements were obtained through an isotope dilution mass spectrometry-traceable method. Serum creatinine levels and eGFR were assessed 24 hours before, 24 hours after, and 6 months after TEVAR.
Imaging data
Aortic computed tomography angiography (CTA) examinations were conducted within 3 days prior to TEVAR and at 3 days, 1 month, and 6 months following TEVAR. Measurements of the diameter of the abdominal aorta, true lumen, and false lumen at the level of the left renal artery were concurrently obtained. The diameter of the primary entry tear was assessed preoperatively. Six months after TEVAR, the observation of false-lumen remodeling encompassed the stent segments, distal thoracic aorta segments, and abdominal aorta segments, involving the formation and absorption of thrombus within the false lumen. Inadequate remodeling indicated the presence of unabsorbed false lumen thrombosis, partial thrombosis, or the absence of thrombosis. Two vascular ultrasound doctors who had worked for more than 10 years performed aortic ultrasound examination within 24 hours preceding and following TEVAR and mutually confirmed that the ultrasound images and measurement results met the inclusion criteria. The study flowchart is depicted in Figure 2. CTA was performed using a multidetector computed tomography (CT) scanner (SOMATOM Definition FLASH, Siemens Healthineers, Erlangen, Germany) with a tube voltage of 120 kV and a current of 400 mA. Images were acquired with a slice thickness of 0.5 or 0.75 mm. An iopramide (350 mg/mL) contrast agent was administered at a rate of 4.0 mL/s. The volume data were transmitted to Vitrea 2.0 workstation (Canon Medical Systems, Tokyo, Japan) for image analysis through virtual reality and multiplanar reformation technologies.
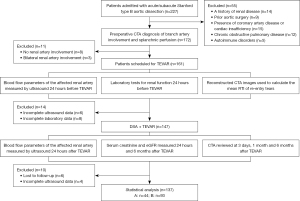
Ultrasonographic quantification of renal perfusion
The software of the ultrasonography system (EchoPAC, GE HealthCare, Chicago, IL, USA) was used to acquire the following hemodynamic parameters for the main renal artery and ascending aorta (AAO): peak systolic velocity (PSV), end-diastolic velocity (EDV), mean diastolic velocity (MDV), time-averaged maximum velocity (TAMAX), time-averaged velocity (TAMEAN), volume flow (VolFlow), resistance index (RI), heart rate (HR), and inner diameter (D) (Figure 3). Quantitative estimation of renal blood flow, representing the average volume of blood flowing through the main renal artery per minute, was conducted using the following formula (18): VolFlow (mL/min) = TAMEAN (cm/s) × [D (cm)/2]2 × π × 60. The measured site for the renal artery was the distal normal trunk of the involved segment. Aortic blood flow was assessed at the aortic valve.
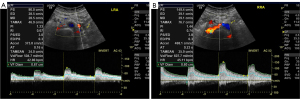
Because the renal artery blood flow after TEVAR changes from that before operation, most of the blood flow will increase, and a small part of the blood flow will decrease. Based on the ultrasound findings of renal artery flow, which indicated whether the flow exhibited an increase or decrease subsequent to TEVAR, patients were classified into two groups: group A (postoperative VolFlow reduced compared to preoperative VolFlow) and group B (postoperative VolFlow increased compared to preoperative VolFlow). Ultrasound examinations were performed with a Vivid 9 ultrasound system (GE HealthCare) equipped with a 4C-D transducer (frequency range, 1.6–6.0 MHz) and a M5S-D transducer (frequency range, 1.5–4.5 MHz). Doppler settings were standardized with a gain of 50, a pulse repetition frequency of 5 MHz, and a sample volume of 2–32 mm.
Calculation of RTI
The calculation of RTI was conducted based on preoperatively obtained reconstructed CTA images (Figure 4). All re-entry tears present in the aortic trunk were included in the analysis. The number of re-entry tears was documented, and for each re-entry tear, both the maximum diameter and the distance from the involved renal artery were measured. The RTI for each re-entry tear was computed as the ratio of the maximum diameter of the re-entry tear (a) to the distance between the re-entry tear and the involved renal artery (b). The mean RTI was determined as the average of individual RTI values; i.e., (RTI1 + RTI2 + RTI3 + … + RTIn)/n.
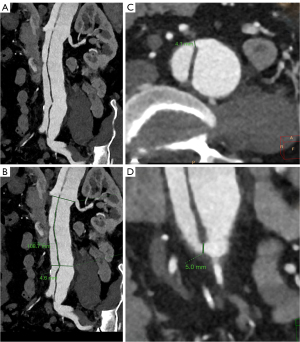
TEVAR procedure
A stent graft, measuring approximately 15–20 cm in length and with an oversizing of about 10%, was primarily deployed to cover the proximal entry tear, ensuring proximal landing zones of at least 1.5 cm. The distal landing zones of the stent graft were consistently positioned above the diaphragm, providing coverage from zone 3 to zone 5 (19). If the proximal landing zones were <1.5 cm, adjunctive procedures such as chimney stenting or bypass surgery were deemed necessary. Technical success was considered achieved when the stent graft was accurately deployed, effectively covering the primary tear without causing proximal endoleak, severe complications, or intraoperative death. In this study, membrane-covered stents, specifically the Valiant Captivia stents (Medtronic, Minneapolis, MN, USA), were used in TEVAR.
Statistical analysis
Numerical data conforming to a normal distribution were presented as the mean ± standard deviation, with group comparisons being conducted with the Student t-test. If the data did not conform to a normal distribution, the nonparametric test method was used. Categorical data were expressed as frequency counts and percentages, and group comparisons were conducted via the chi-square test. Univariate and multivariate logistic regression analyses were undertaken to assess risk factors associated with heightened renal arterial blood flow subsequent to TEVAR. Inclusion of variables in the multivariate analysis was determined based on the results of the univariate analysis with a P value <0.1 and clinical significance. Receiver operating characteristic (ROC) curve analysis, along with DeLong test, was conducted to ascertain the optimal cutoff value and the predictive efficacy of the RTI in enhancing renal arterial blood flow. A two-sided P value <0.05 indicated a statistically significant difference. The analyses were conducted using SPSS version 22.0 (IBM Corp., Armonk, NY, USA).
Results
Baseline characteristics of patients
In this study, a group of 137 patients diagnosed with acute (n=32) or subacute (n=105) type B aortic dissection and renal artery involvement underwent TEVAR. The TEVAR procedure was successful in all cases, resulting in a 67.9% increase in renal blood flow after TEVAR. Among the enrolled patients, 83 (60.6%) had concurrent hypertension, 78 (56.9%) had a history of smoking, 71 (51.8%) had diabetes mellitus, and 80 (58.4%) had dyslipidemia (Table 1).
Table 1
Variables | Group A (n=44) | Group B (n=93) | P value |
---|---|---|---|
Clinical data | |||
Age (years) | 53.14±10.63 | 51.32±11.25 | 0.036 |
Male | 27 (61.4) | 66 (71.0) | 0.553 |
BMI (kg/m2) | 25.11±7.30 | 23.05±7.25 | 0.231 |
SBP (mmHg) | 145±36 | 153±22 | 0.212 |
DBP (mmHg) | 94±26 | 83±19 | 0.056 |
Medical history | |||
Hypertension | 26 (59.1) | 57 (61.3) | 0.031 |
Dyslipidemia | 29 (65.9) | 51 (54.8) | 0.555 |
Diabetes | 19 (43.2) | 52 (55.9) | 0.677 |
Smoking | 33 (75.0) | 45 (48.4) | 0.91 |
Data are presented as mean ± SD or n (%). Group A: postoperative VolFlow reduced compared to preoperative VolFlow; group B: postoperative VolFlow increased compared to preoperative VolFlow. BMI, body mass index; SBP, systolic blood pressure; DBP, diastolic blood pressure; SD, standard deviation; VolFlow, volume flow.
The preoperative CTA examination revealed a total of 308 re-entry tears, representing an average of 2.25 re-entry tears per patient. The distribution of these re-entry tears was as follows: 60 (19.5%) between the primary entry tear and the abdominal trunk, 156 (50.6%) between the abdominal trunk artery and the right renal artery, and 92 (29.9%) below the right renal artery.
Among the patients, 44 (32.1%) were assigned to group A and 93 (67.9%) to group B (Table 1). The laboratory data (serum creatinine and eGFR) (Table 2), CTA data (such as whether the false lumen entirely supplied the renal artery and the number of re-entry tears) (Table 3), ultrasound data (PSV, EDV, TAMEAN, VolFlow) (Table 4), covered stent for TEVAR, and false-lumen remodeling (Table 5) were collected. Compared to group A, patients in group B exhibited a younger age (P=0.036) and lower preoperative 24-hour serum creatinine levels (P=0.022) among patients with acute/subacute type B aortic dissection and renal artery involvement. Group B also displayed a higher prevalence of a history of hypertension (P=0.031), complete renal artery supply from the false lumen (P=0.034), and a higher RTI value of (P=0.005). Moreover, poorer remodeling, specifically partial thrombosis (P=0.014) in the false lumen of abdominal aorta segments was more frequently observed in group B than in group A.
Table 2
Variables | Group A (n=44) | Group B (n=93) | P value |
---|---|---|---|
Serum creatinine (μmol/L) | |||
24 hours preoperation | 81.20±26.80 | 78.70±36.30 | 0.022 |
24 hours postoperation | 82.55±25.54 | 94.2±62.30 | 0.443 |
6 months postoperation | 72.35±28.14 | 70.1±27.30 | 0.755 |
eGFR (mL/min/1.73 m2) | |||
24 hours preoperation | 105.90±21.30 | 90.10±18.20 | 0.877 |
24 hours postoperation | 91.10±19.20 | 86.10±18.50 | 0.255 |
6 months postoperation | 119.90±26.8 | 101.10±17.30 | 0.092 |
Data are presented as mean ± SD. Group A: postoperative VolFlow reduced compared to preoperative VolFlow; group B: postoperative VolFlow increased compared to preoperative VolFlow. eGFR, estimated glomerular filtration rate; SD, standard deviation; VolFlow, volume flow.
Table 3
Variables | Group A (n=44) | Group B (n=93) | P value |
---|---|---|---|
False-lumen supply for the renal artery | |||
Whole from the false lumen | 15 (34.1) | 38 (40.9) | 0.034 |
Partial from the false lumen | 29 (65.9) | 55 (59.1) | 0.228 |
Re-entry tear | |||
One | 5 (11.4) | 11(11.8) | 0.094 |
Two | 13 (29.5) | 13 (14.0) | 0.771 |
More than two | 26 (59.1) | 69 (74.2) | 0.087 |
RTI | 0.030±0.029 | 0.034±0.023 | 0.005 |
Data are presented as n (%) or mean ± SD. Group A: postoperative VolFlow reduced compared to preoperative VolFlow; group B: postoperative VolFlow increased compared to preoperative VolFlow. One, one re-entry tear; two, two re-entry tears; more than two, more than two re-entry tears. CTA, computed tomography angiography; RTI, re-entry tear index; SD, standard deviation; VolFlow, volume flow.
Table 4
Variables | Group A | Group B | P value |
---|---|---|---|
Preoperative 24 hours | |||
PSV (cm/s) | 112±23 | 91±24 | 0.441 |
EDV (cm/s) | 26±9 | 25±4 | 0.076 |
TAMEAN (cm/s) | 31±7 | 29±7 | 0.332 |
VolFlowRA (mL/min) | 468±119 | 392±125 | 0.221 |
VolFlowAAO (mL/min) | 3,706±944 | 3,755±989 | 0.937 |
VolFlowRA/VolFlowAAO | 0.165±0.062 | 0.163±0.079 | 0.811 |
Postoperative 24 hours | |||
PSV (cm/s) | 104±19 | 97±23 | 0.233 |
EDV (cm/s) | 26±5 | 26±6 | 0.655 |
TAMEAN (cm/s) | 28±6 | 33±7 | 0.066 |
VolFlowRA (mL/min) | 489±157 | 420±189 | 0.330 |
VolFlowAAO (mL/min) | 3,725±901 | 3,925±1,001 | 0.337 |
VolFlowRA/VolFlowAAO | 0.164±0.077 | 0.167±0.066 | 0.091 |
Data are presented as mean ± SD. Group A: postoperative VolFlow reduced compared to preoperative VolFlow; group B: postoperative VolFlow increased compared to preoperative VolFlow. PSV, peak systolic velocity; EDV, end-diastolic velocity; TAMEAN, time-averaged velocity; VolFlow, volume flow; VolFlowAAO, ascending aorta volume flow; VolFlowRA, renal artery volume flow; SD, standard deviation.
Table 5
Variables | Group A (n=44) | Group B (n=93) | P value |
---|---|---|---|
TEVAR covered stent | |||
Proximal diameter (mm) | 0.661 | ||
28 | 6 (13.6) | 11 (11.8) | |
30/32/34 | 38 (86.4) | 78 (83.9) | |
36/38/40 | 0 | 4 (4.3) | |
Length (mm) | 0.544 | ||
200 | 40 (90.9) | 85 (91.4) | |
150 | 4 (9.1) | 8 (8.6) | |
False-lumen remodeling | |||
Stent segment | |||
Complete absorption | 31 (70.5) | 81 (87.1) | 0.778 |
Poor remodeling | |||
Unabsorbed thrombosis | 13 (29.5) | 11 (11.8) | 0.902 |
Partial thrombosis | 0 | 1 (1.1) | 0.445 |
No thrombosis | 0 | 0 | – |
Distal thoracic aorta segment | |||
Complete absorption | 15 (34.1) | 49 (52.7) | 0.667 |
Poor remodeling | |||
Unabsorbed thrombosis | 7 (15.9) | 8 (8.6) | 0.551 |
Partial thrombosis | 17 (38.6) | 19 (20.4) | 0.9 |
No thrombosis | 5 (11.4) | 17 (18.3) | 0.732 |
Abdominal aorta segment | |||
Complete absorption | 10 (22.7) | 20 (21.5) | 0.554 |
Poor remodeling | |||
Unabsorbed thrombosis | 4 (9.1) | 8 (8.6) | 0.335 |
Partial thrombosis | 22 (50.0) | 54 (58.1) | 0.014 |
No thrombosis | 8 (18.2) | 11 (11.8) | 0.096 |
Abdominal aorta (preoperation, left renal artery plane) | |||
TL diameter (cm) | 11.3±3.0 | 12.7±3.9 | – |
PSVTL (cm/s) | 63±34 | 72±39 | – |
FL diameter (cm) | 14.2±5.7 | 15.6±5.4 | – |
PSVFL (cm/s) | 71±29 | 65±39 | – |
Abdominal aorta (24 hours postoperation, left renal artery plane) | |||
TL diameter (cm) | 14.7±4.9 | 14.3±4.1 | – |
PSVTL (cm/s) | 73±44 | 73±32 | – |
FL diameter (cm) | 12.6±4.4 | 14.2±5.7 | – |
PSVFL (cm/s) | 45±19 | 51±22 | – |
Data are presented as n (%) or mean ± SD. Group A: postoperative VolFlow reduced compared to preoperative VolFlow; group B: postoperative VolFlow increased compared to preoperative VolFlow. TEVAR, thoracic endovascular aortic repair; TL, true lumen; PSVTL, peak systolic velocity of true lumen; FL, false lumen; PSVFL, peak systolic velocity of false lumen; SD, standard deviation; VolFlow, volume flow.
In the comparison of measures before operation to those after operation, significant statistical differences were observed in the true-lumen diameter (P=0.01), false-lumen diameter (P=0.030), true-lumen velocity (P=0.011), and false-lumen velocity (P=0.043). Specifically, the true lumen exhibited an enlargement with an increased flow velocity, while the false lumen displayed a reduction in size accompanied by a decreased flow velocity. Notably, no new re-entries were detected in group A, whereas 15 patients in group B exhibited the emergence of new re-entries.
Six months after TEVAR, 71 patients (51.8%) (59 from group B and 12 from group A) exhibited a decrease in serum creatinine along with an increase in eGFR, indicating improved renal function. Conversely, 66 patients (48.2%) experienced an increase in serum creatinine paired with a decrease in eGFR, reflecting no significant improvement in renal function. The mean value of serum creatinine reached the peak value and eGFR decreased to the lowest value at 24 hours after operation in both groups (Table 2).
In group A, ultrasound examination revealed that the AAO VolFlow was 3,706±944 mL/min preoperatively and 3,725±901 mL/min postoperatively (P=0.937). The VolFlow renal artery:AAO ratio, representing the ratio of arterial blood flow to aortic blood flow in the affected kidney, was 0.165±0.062 preoperatively and 0.164±0.077 postoperatively (P=0.811). Conversely, in group B, ultrasound examination revealed that the AAO VolFlow was 3,755±989 mL/min preoperatively and 3,925±1,001 mL/min postoperatively (P=0.337). The VolFlow renal artery:AAO ratio was 0.163±0.079 preoperatively and 0.167±0.066 postoperatively (P=0.091) (Table 4).
Risk factor analysis for increased renal arterial blood flow post-TEVAR
The univariate statistical analysis of age, gender, BMI, medical history (hypertension, dyslipidemia, diabetes, smoking), serum creatinine, whether the involved renal artery arose partially or wholly from the false lumen, proximal diameter and length of covered stent, diameter of the primary entry tear, RTI, etc. was completed. To identify risk factors associated with increased renal arterial blood flow after TEVAR, a multivariate analysis was conducted. This analysis incorporated age, preoperative serum creatinine levels at 24 hours, a history of hypertension, complete renal artery supply from the false lumen, poorer remodeling (partial thrombosis), primary entry tear diameter, and RTI. eGFR was not included in the multivariate analysis, as it was calculated from serum creatinine. In the multivariate analysis, the independent risk factors for renal artery involvement in acute and subacute type B aortic dissection were a history of hypertension [odds ratio =5.87; 95% confidence interval (CI): 1.39–11.77; P=0.032] and RTI (odds ratio =14.70; 95% CI: 3.13–66.55; P=0.020) (Table 6).
Table 6
Preoperative parameters | Univariate analysis | Multivariate analysis | |||||
---|---|---|---|---|---|---|---|
Odds ratio | 95% CI | P value | Odds ratio | 95% CI | P value | ||
Clinical data | |||||||
Age | 1.10 | 1.06–1.15 | 0.074 | 0.95 | 0.53–3.07 | 0.220 | |
Male | 0.71 | 0.73–2.31 | 0.931 | ||||
BMI | 0.12 | 0.91–1.13 | 0.773 | ||||
Medical history | |||||||
Hypertension | 3.76 | 0.56–1.07 | 0.021 | 5.87 | 1.39–11.77 | 0.032 | |
Dyslipidemia | 0.94 | 0.81–1.53 | 0.171 | 0.21 | 0.05–0.77 | 0.472 | |
Diabetes | 0.33 | 0.90–1.33 | 0.172 | ||||
Smoking | 1.00 | 1.01–1.33 | 0.371 | ||||
Laboratory data | |||||||
Serum creatinine | 1.41 | 0.38–5.21 | 0.031 | 0.41 | 0.08–3.21 | 0.561 | |
eGFR | 0.65 | 0.48–7.03 | 0.370 | ||||
False-lumen supply | |||||||
Whole from false lumen | 3.09 | 0.78–5.21 | 0.051 | 1.22 | 0.67–4.21 | 0.120 | |
Partial from false lumen | 0.45 | 0.18–1.03 | 0.370 | ||||
Covered stent | |||||||
Proximal diameter | 0.65 | 0.07–3.15 | 0.120 | 0.44 | 0.28–1.21 | 0.061 | |
Length | 0.60 | 0.25–2.23 | 0.341 | ||||
Diameter of primary entry tear | 1.21 | 0.40–2.11 | 0.044 | 0.39 | 0.21–2.20 | 0.332 | |
RTI | 8.34 | 3.03–11.87 | 0.011 | 14.70 | 3.13–66.55 | 0.020 |
TEVAR, thoracic endovascular aortic repair; CI, confidence interval; BMI, body mass index; eGFR, estimated glomerular filtration rate; RTI, re-entry tear index.
ROC curve analysis revealed that the area under the ROC curve (AUC) of RTI for identifying increased renal arterial blood flow after TEVAR in patients with no history of hypertension was 0.789 (95% CI: 0.599–0.960; P=0.011). The optimal cutoff value was determined to be 0.033, providing 90.9% sensitivity and 54.5% specificity (Figure 5A). Similarly, in patients with a history of hypertension, RTI yielded a 96.6% sensitivity and a 60.0% specificity in identifying increased renal arterial blood flow (AUC =0.792; 95% CI: 0.643–0.941; P=0.021) in acute/subacute type B aortic dissection with renal artery involvement (Figure 5B), and the optimal cutoff value was also 0.033. The ROC curve of RTI in the whole cohort had a sensitivity of 100% and a specificity of 50% (AUC =0.750; 95% CI: 0.540–0.960; P=0.048), and the cutoff value was 0.033 (Figure 6).
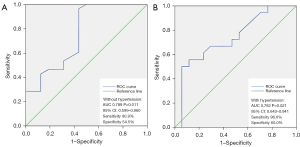
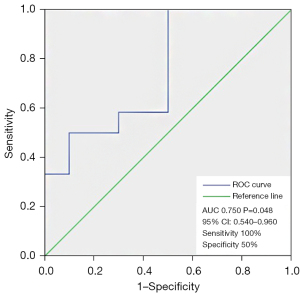
The relationship between mean RTI and the increase in post-TEVAR renal blood flow appeared roughly linear (Figure 7). At the 6-month follow-up after surgery, two cases demonstrated a reduction in kidney volume, with kidney lengths decreasing from 9.5 and 9.3 cm before surgery to 8.5 and 8.3 cm after surgery, respectively, with the corresponding RTI values being 0.034 and 0.011.
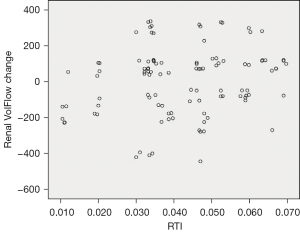
Discussion
The objective of this study was to investigate the predictive capacity of the RTI in assessing the enhancement of postoperative renal perfusion among patients with acute or subacute type B aortic dissection with renal artery involvement. Our findings indicate that RTI can function as an independent risk factor influencing changes in renal perfusion, providing valuable insights into the potential improvement of compromised renal perfusion. Notably, patients with a history of hypertension demonstrated heightened sensitivity and specificity at the same RTI cutoff value (0.033) compared to their counterparts without hypertension. This phenomenon can be attributed to the diminished elasticity of vessel walls in patients who are hypertensive and to the attenuated remodeling capacity of vessel walls following surgery. Consequently, the redistribution of blood flow pressure between the true and false lumens becomes more contingent on the passage between the two, namely the re-entry tears.
Despite the recognized significance of the number of re-entry tears as a potential determinant influencing the progression of type B aortic dissection, previous studies have been limited in achieving real-time quantitative analyses of the relationship between re-entry tears and hemodynamic shifts in arterial branches (20,21). The evidence indicates that an escalation in the number of tears facilitates the redistribution of flow from the false lumen to the true lumen, providing additional pathways for blood flow between the lumens (20-23). This redistribution results in changes in velocity patterns, including changes in velocity magnitudes and VolFlow in the aorta and its branches, thereby directly impacting branch perfusion. However, our study revealed no significant changes in the ratio of VolFlow renal artery:AAO ratio after surgery in the two groups, potentially due to the absence of comprehensive renal artery dissection in these patients. Although following surgery, the renal VolFlow decreased in group A but increased in group B, the VolFlow renal artery:AAO ratio remained relatively stable in both groups. This observation prompts further investigation in subsequent studies.
Our study demonstrated a roughly linear correlation between mean RTI and the augmentation in post-TEVAR renal blood flow increase. Larger re-entry tear diameters, coupled with shorter distances from the implicated renal artery, corresponded to elevated RTI values (>0.033), which is indicative of an improvement in blood flow to the affected renal artery subsequent to surgery. Notably, patients with subacute type B aortic dissection who underwent TEVAR exhibited positive clinical outcomes.
Furthermore, distal re-entry tears were observed in all patients, with the positioning of the trunk abdominal artery when compared to the double renal artery exhibiting a multifaceted pattern. This observation may be attributed to the profusion of visceral artery branches in this anatomical region. As blood descends subsequent to the primary breach into the middle layer, it encounters these branches, prompting changes in the blood flow direction and consequent inner membrane tears of the visceral artery at the opening. Consequently, a higher prevalence of re-entry tears was identified between the abdominal trunk and renal arteries, potentially explaining the observed enhancement in renal artery flow after TEVAR in most patients (67.9%) within this group.
Injuries to branches of the abdominal aorta can receive blood supply from either a single true lumen, a single false lumen, or both true and false lumens. After TEVAR, the diameter of the false lumen in the abdominal aorta shrinks, gradually leading to thrombosis and diminished patency. These factors may contribute to inadequate blood supply to arterial branches fed by the false lumen. There have been clinical cases reported of kidney atrophy and necrosis following TEVAR (24). Rectifying all branches of the abdominal aorta through intervention to supply blood from the true lumen after TEVAR requires considerable time and effort, and this approach is not recommended in the relevant guidelines. Hence, preoperative assessment of renal artery risk stratification through noninvasive imaging assumes particular significance. Given the relatively low incidence of cases and the heterogeneity of type B aortic dissection subtypes, formulating risk-stratification tools for this condition poses challenges but remains imperative for refining personalized and cost-effective treatment selection. In this context, both morphological and hemodynamic assessments are rapidly evolving. Our study integrated morphology (location and size of re-entry tears observed through CTA) and hemodynamics (quantitative analysis of changes in renal arterial blood flow through ultrasound) to clarify the intrinsic relationship between these two facets.
The results from our study revealed a significant correlation between the size and location of distal re-entry tears and the improvement in renal artery blood flow following TEVAR. The underlying mechanism involves the false lumen-perfused visceral arteries, which induce negative aortic remodeling by sustaining continuous patency of the false lumen (11,12,18,25). Previous research has demonstrated that patients with false-lumen perfusion of the visceral arteries exhibit more extensive dissection, a larger false-lumen volume, and a greater prevalence of distal re-entry tears (26). In cases of aortic dissection involving the renal artery level, distal re-entry tears frequently manifest in the region above the celiac trunk to the termination of the abdominal aorta. These tears serve as significant conduits for blood flow from the true lumen to the false lumen, resulting in the reversal of perfusion in the affected renal artery after TEVAR. This pathophysiological alteration, characterized by retrograde blood supply through the distal re-entry tear of the dissection, constitutes the pathological foundation for enhancing the blood supply to the implicated renal artery following surgery.
The study conducted by Magee et al. demonstrated a high patency rate of branch vessels after TEVAR for type B aortic dissection (27). Notably, there was no substantial difference in the patency rate between branch vessels originating from the false lumen or both the true and false lumen and those originating solely from the true lumen (27). Our present study similarly observed an increase in postoperative blood flow in the involved renal artery for most patients. Moreover, the outcomes of our semiquantitative analysis of re-entry tears using the RTI indicated that the RTI cutoff value has high sensitivity but low specificity in predicting whether renal artery blood flow can increase after TEVAR, resulting in an amount of false positives and false negatives.
It is worth noting that certain patients in our study had abnormal renal function before surgery. Despite the RTI values being greater than the cutoff, there was no increase in renal arterial blood flow 24 hours following surgery, and no enhancement in renal function was observed at the 6-month follow-up, leading to instances of false positives. This may be attributed to a significantly lower preoperative renal artery blood flow compared to that of a normal physiological state. Prolonged exposure to a low hemodynamic state can result in diminished renal perfusion pressure, causing damage to glomeruli and tubules. Consequently, when renal tissue regains blood supply following acute ischemia and hypoxia, renal ischemia-reperfusion injury may occur, impeding the return to normal and potentially exacerbating dysfunction and structural damage. Therefore, in patients with preoperative renal dysfunction and renal malperfusion identified via ultrasound, the prognosis after TEVAR is unfavorable, and the sole use of the RTI cutoff value is insufficient for determining outcomes. Among the patients in our study, 71 (51.8%) exhibited improved renal function, while 66 (48.2%) demonstrated no improvement at 6 months following TEVAR. The week following TEVAR included the poorest renal function compared to 24 hours before surgery and 6 months after surgery. The substantial use of contrast agent during surgery likely impacted the swift recovery of renal function (28,29). Contrast nephropathy is being increasingly acknowledged as a major contributor to medically induced renal insufficiency, with no current effective treatment. With the improvement of renal blood perfusion, renal function gradually recovers. Therefore, the judicious selection of isotonic contrast agents with minimal renal injury according to preoperative renal function is crucial to safeguarding renal function.
Following the release of the Investigation of Stent Grafts in Aortic Dissection (INSTEAD) XL trial, numerous centers have adopted the practice of using TEVAR for the treatment of uncomplicated subacute type B aortic dissection, with the aim of achieving long-term remodeling and enhanced survival (30). Our study included both acute and subacute cases, with RTI potentially providing additional predictive insights. Further investigations in patients specifically diagnosed with acute type B aortic dissection are imperative to assess the utility of RTI in predicting postoperative renal perfusion improvement in this subgroup.
This study was subject to several limitations which should be addressed. First, the relatively low incidence of cases and the heterogeneity of type B aortic dissection subtypes pose challenges in developing risk stratification tools. The number of patients with acute type B aortic dissection was relatively limited, primarily due to the emergency nature of treatment for these patients, precluding sufficient time for measurements tailored for research purposes. Additionally, the RTI threshold used in this study displayed low specificity in determining whether renal artery flow increased after TEVAR, resulting in some false positives and false negatives. These limitations may be a result of the sample size and the stochastic nature of the data or may be inherent to the methodology itself. Consequently, further large-scale studies are warranted to validate the clinical significance of these findings.
Conclusions
RTI is a promising predictor for the improvement of renal malperfusion subsequent to TEVAR in cases of aortic dissection affecting the renal artery. Patients with preoperative renal dysfunction and malperfusion on ultrasound and CTA may experience an unfavorable prognosis following TEVAR, with a diminished likelihood that the renal malperfusion can be resolved.
Acknowledgments
Funding: This study was supported by
Footnote
Reporting Checklist: The authors have completed the STROBE reporting checklist. Available at https://qims.amegroups.com/article/view/10.21037/qims-24-206/rc
Conflicts of Interest: All authors have completed the ICMJE uniform disclosure form (available at https://qims.amegroups.com/article/view/10.21037/qims-24-206/coif). The authors have no conflicts of interest to declare.
Ethical Statement: The authors are accountable for all aspects of the work in ensuring that questions related to the accuracy or integrity of any part of the work are appropriately investigated and resolved. This study was conducted in accordance with the Declaration of Helsinki (as revised in 2013) and was approved by the ethics committee of Beijing Anzhen Hospital (No. 2023123X). Informed consent was obtained from all patients.
Open Access Statement: This is an Open Access article distributed in accordance with the Creative Commons Attribution-NonCommercial-NoDerivs 4.0 International License (CC BY-NC-ND 4.0), which permits the non-commercial replication and distribution of the article with the strict proviso that no changes or edits are made and the original work is properly cited (including links to both the formal publication through the relevant DOI and the license). See: https://creativecommons.org/licenses/by-nc-nd/4.0/.
References
- Pape LA, Awais M, Woznicki EM, Suzuki T, Trimarchi S, Evangelista A, Myrmel T, Larsen M, Harris KM, Greason K, Di Eusanio M, Bossone E, Montgomery DG, Eagle KA, Nienaber CA, Isselbacher EM, O'Gara P. Presentation, Diagnosis, and Outcomes of Acute Aortic Dissection: 17-Year Trends From the International Registry of Acute Aortic Dissection. J Am Coll Cardiol 2015;66:350-8. [Crossref] [PubMed]
- Patel AY, Eagle KA, Vaishnava P. Acute type B aortic dissection: insights from the International Registry of Acute Aortic Dissection. Ann Cardiothorac Surg 2014;3:368-74. [PubMed]
- Hagan PG, Nienaber CA, Isselbacher EM, Bruckman D, Karavite DJ, Russman PL, et al. The International Registry of Acute Aortic Dissection (IRAD): new insights into an old disease. JAMA 2000;283:897-903. [Crossref] [PubMed]
- Tozzi P. Thoracic endovascular aortic repair to treat uncomplicated Stanford type B aortic dissection: The surgeon's dilemma to preventing future complications. Eur J Prev Cardiol 2018;25:24-31. [Crossref] [PubMed]
- Canaud L, Ozdemir BA, Patterson BO, Holt PJ, Loftus IM, Thompson MM. Retrograde aortic dissection after thoracic endovascular aortic repair. Ann Surg 2014;260:389-95. [Crossref] [PubMed]
- Hansen CJ, Bui H, Donayre CE, Aziz I, Kim B, Kopchok G, Walot I, Lee J, Lippmann M, White RA. Complications of endovascular repair of high-risk and emergent descending thoracic aortic aneurysms and dissections. J Vasc Surg 2004;40:228-34. [Crossref] [PubMed]
- Rudenick PA, Bijnens BH, García-Dorado D, Evangelista A. An in vitro phantom study on the influence of tear size and configuration on the hemodynamics of the lumina in chronic type B aortic dissections. J Vasc Surg 2013;57:464-474.e5. [Crossref] [PubMed]
- Tsai TT, Evangelista A, Nienaber CA, Myrmel T, Meinhardt G, Cooper JV, Smith DE, Suzuki T, Fattori R, Llovet A, Froehlich J, Hutchison S, Distante A, Sundt T, Beckman J, Januzzi JL Jr, Isselbacher EM, Eagle KA. Partial thrombosis of the false lumen in patients with acute type B aortic dissection. N Engl J Med 2007;357:349-59. [Crossref] [PubMed]
- Wan Ab Naim WN, Ganesan PB, Sun Z, Lei J, Jansen S, Hashim SA, Ho TK, Lim E. Flow pattern analysis in type B aortic dissection patients after stent-grafting repair: Comparison between complete and incomplete false lumen thrombosis. Int J Numer Method Biomed Eng 2018;34:e2961. [Crossref] [PubMed]
- Li D, Zheng T, Liu Z, Li Y, Yuan D, Fan Y. Influence of Distal Re-entry Tears on False Lumen Thrombosis After Thoracic Endovascular Aortic Repair in Type B Aortic Dissection Patients: A Computational Fluid Dynamics Simulation. Cardiovasc Eng Technol 2021;12:426-37. [Crossref] [PubMed]
- Tolenaar JL, Kern JA, Jonker FH, Cherry KJ, Tracci MC, Angle JF, Sabri S, Trimarchi S, Strider D, Alaiwaidi G, Upchurch GR Jr. Predictors of false lumen thrombosis in type B aortic dissection treated with TEVAR. Ann Cardiothorac Surg 2014;3:255-63. [PubMed]
- Sultan I, Siki MA, Bavaria JE, Dibble TR, Savino DC, Kilic A, Szeto W, Vallabhajosyula P, Fairman RM, Jackson BM, Wang GJ, Desai ND. Predicting Distal Aortic Remodeling After Endovascular Repair for Chronic DeBakey III Aortic Dissection. Ann Thorac Surg 2018;105:1691-6. [Crossref] [PubMed]
- Lombardi JV, Hughes GC, Appoo JJ, Bavaria JE, Beck AW, Cambria RP, Charlton-Ouw K, Eslami MH, Kim KM, Leshnower BG, Maldonado T, Reece TB, Wang GJ. Society for Vascular Surgery (SVS) and Society of Thoracic Surgeons (STS) reporting standards for type B aortic dissections. J Vasc Surg 2020;71:723-47. [Crossref] [PubMed]
- Jones NR, McCormack T, Constanti M, McManus RJ. Diagnosis and management of hypertension in adults: NICE guideline update 2019. Br J Gen Pract 2020;70:90-1. [Crossref] [PubMed]
- 2016 Chinese guidelines for the management of dyslipidemia in adults. J Geriatr Cardiol 2018;15:1-29. [PubMed]
- Lian F, Ni Q, Shen Y, Yang S, Piao C, Wang J, Wei J, Duan J, Fang Z, Lu H, Yang G, Zhao L, Song J, Li Q, Zheng Y, Lyu Y, Tong X. International traditional Chinese medicine guideline for diagnostic and treatment principles of diabetes. Ann Palliat Med 2020;9:2237-50. [Crossref] [PubMed]
- Soares AA, Eyff TF, Campani RB, Ritter L, Camargo JL, Silveiro SP. Glomerular filtration rate measurement and prediction equations. Clin Chem Lab Med 2009;47:1023-32. [Crossref] [PubMed]
- Birjiniuk J, Veeraswamy RK, Oshinski JN, Ku DN. Intermediate fenestrations reduce flow reversal in a silicone model of Stanford Type B aortic dissection. J Biomech 2019;93:101-10. [Crossref] [PubMed]
- MacGillivray TE, Gleason TG, Patel HJ, Aldea GS, Bavaria JE, Beaver TM, Chen EP, Czerny M, Estrera AL, Firestone S, Fischbein MP, Hughes GC, Hui DS, Kissoon K, Lawton JS, Pacini D, Reece TB, Roselli EE, Stulak J. The Society of Thoracic Surgeons/American Association for Thoracic Surgery Clinical Practice Guidelines on the Management of Type B Aortic Dissection. Ann Thorac Surg 2022;113:1073-92. [Crossref] [PubMed]
- Girish A, Padala M, Kalra K, McIver BV, Veeraswamy RK, Chen EP, Leshnower BG. The Impact of Intimal Tear Location and Partial False Lumen Thrombosis in Acute Type B Aortic Dissection. Ann Thorac Surg 2016;102:1925-32. [Crossref] [PubMed]
- Karmonik C, Bismuth J, Shah DJ, Davies MG, Purdy D, Lumsden AB. Computational study of haemodynamic effects of entry- and exit-tear coverage in a DeBakey type III aortic dissection: technical report. Eur J Vasc Endovasc Surg 2011;42:172-7. [Crossref] [PubMed]
- Armour C, Guo B, Saitta S, Guo D, Liu Y, Fu W, Dong Z, Xu XY. The Role of Multiple Re-Entry Tears in Type B Aortic Dissection Progression: A Longitudinal Study Using a Controlled Swine Model. J Endovasc Ther 2024;31:104-14. [Crossref] [PubMed]
- Saitta S, Guo B, Pirola S, Menichini C, Guo D, Shan Y, Dong Z, Xu XY, Fu W. Qualitative and Quantitative Assessments of Blood Flow on Tears in Type B Aortic Dissection With Different Morphologies. Front Bioeng Biotechnol 2021;9:742985. [Crossref] [PubMed]
- Yu YT, Ren XS, An YQ, Yin WH, Zhang J, Wang X, Lu B. Changes in the renal artery and renal volume and predictors of renal atrophy in patients with complicated type B aortic dissection after thoracic endovascular aortic repair. Quant Imaging Med Surg 2022;12:5198-208. [Crossref] [PubMed]
- Kamman AV, Brunkwall J, Verhoeven EL, Heijmen RH, Trimarchi S. Predictors of aortic growth in uncomplicated type B aortic dissection from the Acute Dissection Stent Grafting or Best Medical Treatment (ADSORB) database. J Vasc Surg 2017;65:964-971.e3. [Crossref] [PubMed]
- Ge Y, Lv X, Ge X, Miao J, Fan W, Rong D, Guo W. The Number of Preoperative Abdominal False Lumen-perfused Small Branches Is Related to Abdominal Aortic Remodeling after Thoracic Endovascular Aortic Repair for Type B Aortic Dissection. Ann Vasc Surg 2021;71:56-64. [Crossref] [PubMed]
- Magee GA, Plotkin A, Dake MD, Starnes BW, Han SM, Ding L, Weaver FA. Branch Vessel Patency after Thoracic Endovascular Aortic Repair for Type B Aortic Dissection. Ann Vasc Surg 2021;70:152-61. [Crossref] [PubMed]
- Zhang F, Lu Z, Wang F. Advances in the pathogenesis and prevention of contrast-induced nephropathy. Life Sci 2020;259:118379. [Crossref] [PubMed]
- Luk L, Steinman J, Newhouse JH. Intravenous Contrast-Induced Nephropathy-The Rise and Fall of a Threatening Idea. Adv Chronic Kidney Dis 2017;24:169-75. [Crossref] [PubMed]
- Nienaber CA, Kische S, Rousseau H, Eggebrecht H, Rehders TC, Kundt G, Glass A, Scheinert D, Czerny M, Kleinfeldt T, Zipfel B, Labrousse L, Fattori R, Ince H. INSTEAD-XL trial. Endovascular repair of type B aortic dissection: long-term results of the randomized investigation of stent grafts in aortic dissection trial. Circ Cardiovasc Interv 2013;6:407-16. [Crossref] [PubMed]