The ability of virtual noncontrast images to remove intrinsic and external iodine from the thyroid via dual-layer spectral detector computed tomography
Introduction
Compared with traditional computed tomography (CT), the dual-layer spectral CT (DLCT) can provide various qualitative and quantitative analysis methods, such as virtual monoenergetic images (VMIs), virtual noncontrast (VNC) images, iodine density (ID) images, Z effective images, etc., to provide more valuable information for the qualitative diagnosis and quantitative analysis of diseases (1). VMIs area generated by applying a single-energy beam at the kiloelectronvolt (keV) level, which is a linear combination of basis images at 40–200 keV. DLCT directly utilizes spectral postprocessing of raw data, providing better single-energy reconstructions at low keV, with improved contrast-to-noise ratio (CNR) and signal-to-noise ratio (SNR). ID mapping and VNC technology leverage the significant spectral contrast between iodine and water, allowing for effective separation of the two substances. VNC images are obtained by removing the contribution of iodine material to CT values from the enhanced scan images, thereby obtaining images without iodine contrast agent. Studies have shown that VNC images can provide image quality comparable to that of true noncontrast (TNC) images in multiple areas, including the liver, coronary arteries, gastric tumors, kidneys, etc. (2-6). In this case, the noncontrast scan can be omitted in the CT scanning protocol, thereby reducing radiation dose. Unlike abdominal organs such as the liver and gastrointestinal tract, the thyroid stores approximately 70–80% of the body’s iodine, resulting in higher CT values on TNC images. Therefore, it is not appropriate to directly compare TNC images with VNC images generated from enhanced scans to validate VNC’s ability to suppress iodine in the thyroid. Moreover, the thyroid gland is significantly more enhanced compared to other organs due to the large in flow of contrast through a rich vascular network. Thus, a pair of VNC images must be created based on noncontrast and contras-enhanced conventional images (CI) by suppressing the attenuation attributed to intrinsic iodine on noncontrast images and suppressing the attenuation caused by the substantial contrast agent influx on contrast-enhanced images, which can only be achieved with dual-energy CT. Therefore, we attempted to assess the feasibility of VNC by comparing the CT attenuation in thyroid VNC images and ID generated before and after contrast agent injection.
Methods
Patients
A retrospective analysis was conducted on patients who underwent neck scans via spectral CT at Zhongshan City People’s Hospital from October 2021 to October 2022. The inclusion criteria were as follows: (I) thyroid or neck nonenhanced scans and enhanced scans were performed, and the scans covered the entire thyroid gland; (II) no significant artifacts were in the thyroid region that could affect lesion evaluation and identification, with a short diameter of the lesion ≥5 mm; (III) thyroid surgery performed within 1 month after CT scans, with clear immunohistochemical results. Meanwhile, the exclusion criteria were as follows: (I) poor image quality with significant artifacts, (II) images showing purely cystic lesions or nodules with extensive cystic changes, (III) extensive calcification of the lesion or calcification affecting measurements, and (IV) pathological findings suggestive of nodular goiter (NG) with papillary carcinoma or inconclusive pathological results. A total of 115 nodules from 99 patients were included in the study (16 patients with 2 nodules each), with 19 males and 80 females, aged 19–81 years, with an average age of 45±13 years. All nodules were confirmed by surgical pathology and included 81 cases of papillary thyroid carcinoma (PTC) and 34 cases of NG. This study was conducted in accordance with the Declaration of Helsinki (as revised in 2013) and was approved by the Ethics Committee of Zhongshan City People’s Hospital (No. K2021-159). The requirement for individual consent for this retrospective analysis was waived.
CT image acquisition
A dual-layer spectral detector CT scanner (IQon Spectral CT; Philips Healthcare, Amsterdam, the Netherlands) was used for thyroid nonenhanced and enhanced scans. The patient was placed in the supine position with a pillow removed, and the scan range extended from the submandibular region to the upper margin of the chest. A homemade saline bag was placed in the middle of the neck to reduce artifacts during scanning. The patient was instructed to lift the chin and avoid swallowing movements, while the shoulders were lowered to minimize interference from the clavicle. The scanning parameters were as follows: a tube voltage of 120 kVp, tube current automatically adjusted using automatic tube current modulation technology (ranging from 70 to 185 mAs), a collimator width of 64×0.625 mm, an X-ray tube rotation speed of 0.33 s/rotation, and a pitch of 1.164. The contrast agent used was iohexol injection (350 mg I/mL; Jiangsu Hengrui Pharmaceuticals Co., Ltd., Lianyungang, China), which was administered via a high-pressure injector (CT Motion, Ulrich Medical, Ulm, Germany) into the median cubital vein at a dose of 1.5 mL/kg and an injection rate of 3.0–3.5 mL/s. Enhanced scanning was performed 50 seconds after the start of contrast agent injection, with the same scan range as that of the plain scan. After completion of the scan, spectral reconstruction was performed to generate spectral-based images (SBI), with a slice thickness and interval of 1 mm.
Image analysis
After scanning, the SBI data package was transferred to the Philips IntelliSpace Portal (Philips Healthcare) for analysis. The SBI data package was opened in the Spectral Viewer postprocessing workstation, which has the function of synchronously presenting and measuring conventional, VNC, and ID images. The display layer was manually adjusted to layer with the largest nodule area. The CT attenuation values of the nodules, normal thyroid, and corresponding cervical arteries at the same selected layer were measured in the CI and VNC image reconstructed by both the nonenhanced and enhanced data packages, as was ID at the respective locations. Measurements were performed by a radiologist with over 5 years of experience in thyroid diagnosis. On the TNC image, a region of interest (ROI) was selected, covering an area larger than one-third of the nodule. The ROI was positioned to avoid areas of calcification, blood vessels, or cysts. Three measurements were taken at the same level, and the average value was calculated. The ROI was then duplicated, and the corresponding CT attenuation values and ID were measured on the VNC and ID images, respectively. In the nonenhanced images, the CT attenuation values of CI images and VNC images were recorded as conventional images of nonenhanced scans (CIn) and VNC images from nonenhanced scans (VNCn), the iodine density of CI was recorded as iodine density of nonenhanced scans (IDn). In the enhanced images, the CT attenuation values of conventional enhanced images and VNC images were recorded as conventional images of enhanced scans (CIe) and VNC images from enhanced scans (VNCe), the iodine density of enhanced images was recorded as iodine density of enhanced scans (IDe).
The following values were calculated based on the above measurements: ΔHUn = CIn – VNCn, reflecting the CT attenuation values of intrinsic iodine in the ROI in the nonenhanced image; ΔHUe = CIe – VNCe, reflecting the CT attenuation values of iodine contrast agent concentration and intrinsic iodine content in the ROI after contrast injection; ΔHU = ΔHUe – ΔHUn, reflecting the CT attenuation values of external iodine content in the ROI after contrast injection; and ΔID = IDe – IDn, reflecting the concentration of external iodine in the ROI after contrast injection.
Statistical analysis
The data were analyzed using SPSS 27.0 statistical software (IBM Corp., Armonk, NY, USA). The Kolmogorov-Smirnov test was performed to assess the normality of the continuous data. Normally distributed data are presented as [mean ± standard deviation (SD)], while skewed data are presented as the median with interquartile range. The Wilcoxon rank-sum test was used to compare the differences in ΔHUn and ΔHU values between the two groups of nodules, normal thyroid tissue, and the carotid artery and to compare the differences of CT values between the VNCn and VNCe images within each group, from which paired scatter plots were generated. The Spearman rank correlation test was used to examine the correlations between ΔHUn and ΔIDn and between ΔHU and ΔID within each group. Bland-Altman scatter plots were created, with the mean as the X-axis and the difference as the Y-axis, to evaluate the agreement of CT values between VNCn and VNCe images at different limits. The limits of agreement (LOA) were defined as the median of the differences (mean) ± 1.96 SD. All tests were two-sided, and P values lower than 0.05 were considered statistically significant.
Results
Differential analysis
There was no statistically significant difference in the CT attenuation values of the normal thyroid in VNCn and VNCe images (P>0.05), while the CT attenuation values of the nodules and carotid artery in the VNCn and VNCe images in the two groups were significantly different (P<0.05) (Table 1, Figure 1).
Table 1
Parameter | PTC (n=81) | NG (n=34) | |||||
---|---|---|---|---|---|---|---|
Nodule | Normal thyroid | Carotid artery | Nodule | Normal thyroid | Carotid artery | ||
VNCn (HU) | 44.70 (39.10, 49.20) | 61.50 (55.1, 66.1) | 41.4 (38.35, 44.90) | 55.10 (42.93, 58.83) | 62.40 (58.50, 69.50) | 41.30 (38.90, 45.60) | |
VNCe (HU) | 46.30 (40.60, 53.60) | 61.1 (56.7, 67.10) | 51.60 (45.05, 58.15) | 59.00 (46.98, 63.90) | 60.00 (57.98, 66.95) | 51.90 (46.8, 54.6) | |
Z | −2.378 | −0.193 | −6.636 | −2.556 | −1.548 | −4.711 | |
P | 0.018 | 0.850 | <0.001 | 0.011 | 0.120 | <0.001 |
Data are presented as median (interquartile range). CT, computed tomography; VNCn, virtual noncontrast images from nonenhanced scans; VNCe, virtual noncontrast images from enhanced scans; PTC, papillary thyroid carcinoma; NG, nodular goiter.
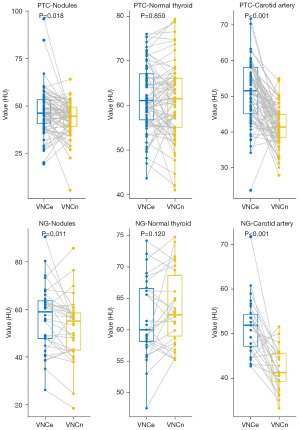
The result showed that there was no significant difference in the intrinsic iodine content (ΔHUn) between nodules, normal thyroid, and the carotid artery in the PTC and NG groups (P>0.05). There was a difference in the external iodine content (ΔHU) of nodules between the PTC and NG groups (P<0.05), but no significant difference in external iodine content (ΔHU) between the normal thyroid and carotid artery (P>0.05) (Table 2).
Table 2
Group | ΔHUn (HU) | ΔHU (HU) | |||||
---|---|---|---|---|---|---|---|
Nodule | Normal thyroid | Carotid artery | Nodule | Normal thyroid | Carotid artery | ||
PTC (n=81) | 10.60 (5.10, 18.20) | 34.12 (25.51, 42.42) | 3.60 (1.95, 5.55) | 69.80 (55.00, 83.90) | 72.60 (63.30, 88.95) | 113.90 (100.95, 126.85) | |
NG (n=34) | 11.20 (6.80, 23.02) | 30.50 (14.90, 38.15) | 4.05 (3.07, 5.83) | 53.65 (25.08, 74.83) | 74.50 (56.95, 86.70) | 106.45 (84.02, 125.07) | |
Z | −1.232 | −2.016 | −0.279 | 3.037 | −0.570 | −1.894 | |
P | 0.218 | 0.054 | 0.780 | 0.002 | 0.569 | 0.058 |
Data are presented as median (interquartile range). ΔHUn = CIn – VNCn is the CT attenuation values of intrinsic iodine in the ROI in the nonenhanced image; ΔHU = ΔHUe – ΔHUn is the CT attenuation values of external iodine content in the ROI after contrast injection. HU, Hounsfield unit; PTC, papillary thyroid carcinoma; NG, nodular goiter; CIn, conventional images of nonenhanced scans; VNCn, virtual noncontrast images from nonenhanced scans; CT, computed tomography; ROI, region of interest.
Correlation analysis
In the PTC group, there was a strong correlation between the CT attenuation values of the nodules, normal thyroid, and carotid artery for the intrinsic iodine content (ΔHUn) and ID (IDn) (r=0.919, r=0.951, and r=0.592, respectively; P<0.001). Similarly, in the NG group, there was a strong correlation between the CT attenuation values of the nodules, normal thyroid, and carotid artery for the intrinsic iodine content (ΔHUn) and ID (IDn) (r=0.925, r=0.973, and r=0.721, respectively; P<0.001) (Table 3).
Table 3
Parameter | PTC (n=81) | NG (n=34) | |||||
---|---|---|---|---|---|---|---|
Nodules | Normal thyroid | Carotid artery | Nodules | Normal thyroid | Carotid artery | ||
ΔHUn (HU) | 10.60 (5.10, 18.20) | 34.12 (25.51, 42.42) | 3.60 (1.95, 5.55) | 11.20 (6.80, 23.02) | 30.5 (14.90, 38.15) | 4.05 (3.07, 5.83) | |
IDn (mg/mL) | 0.29 (0.17, 0.54) | 1.15 (0.90, 1.40) | 0.04 (0.00, 0.15) | 0.36 (0.19, 0.90) | 1.00 (0.51, 1.19) | 0.01 (0, 0.04) | |
r | 0.919 | 0.951 | 0.592 | 0.925 | 0.973 | 0.721 | |
P | <0.001 | <0.001 | <0.001 | <0.001 | <0.001 | <0.001 |
Data are presented as median (interquartile range). ΔHUn = CIn –VNCn is the CT attenuation values of intrinsic iodine in the ROI in nonenhanced images; IDn is the intrinsic iodine density within the ROI. CT, computed tomography; PTC, papillary thyroid carcinoma; NG, nodular goiter; HU, Hounsfield unit; IDn, iodine density of nonenhanced scans; CIn, conventional images of nonenhanced scans; VNCn, virtual noncontrast images from nonenhanced scans; ROI, region of interest.
In the PTC group, there was a good correlation between the CT attenuation values of the nodules, normal thyroid, and carotid artery for the extrinsic iodine content (ΔHU) and ID (ΔID) (r=0.960, r=0.965, and r=0.904, respectively; P<0.001). In the NG group, there was a good correlation between the CT attenuation values of the nodules, normal thyroid, and carotid artery for the extrinsic iodine content (ΔHU) and ID (ΔID) (r=0.979, r=0.967, r=0.963; P<0.001) (Table 4).
Table 4
Parameter | PTC (n=81) | NG (n=34) | |||||
---|---|---|---|---|---|---|---|
Nodules | Normal thyroid | Carotid artery | Nodules | Normal thyroid | Carotid artery | ||
ΔHU (HU) | 69.80 (55.00, 83.90) | 72.60 (63.30, 88.95) | 113.90 (100.95, 126.85) | 53.65 (25.08, 74.83) | 74.50 (56.95, 86.70) | 106.45 (84.02, 125.07) | |
ΔID (mg/mL) | 2.46 (1.98, 3.12) | 2.57 (2.23, 3.12) | 4.15 (3.79, 4.55) | 1.96 (0.90, 2.60) | 2.51 (2.01, 2.91) | 3.91 (3.08, 4.46) | |
r | 0.960 | 0.965 | 0.904 | 0.979 | 0.967 | 0.963 | |
P | <0.001 | <0.001 | <0.001 | <0.001 | <0.001 | <0.001 |
Data are presented as median (interquartile range). ΔHU = ΔHUe – ΔHUn is the CT attenuation values of external iodine content in the ROI after contrast injection; ΔID = IDe – IDn is the concentration of external iodine in the ROI after contrast injection. CT, computed tomography; PTC, papillary thyroid carcinoma; NG, nodular goiter; HU, Hounsfield unit; IDe, iodine density of enhanced scans; IDn, iodine density of nonenhanced scans; ROI, region of interest.
Consistency analysis
The Bland-Altman scatter plot showed that the proportion of data points in the PTC group with differences in CT attenuation values of the nodule, normal thyroid, and carotid artery in VNCn and VNCe images outside the LOA was 6.17%, 6.17%, and 7.41%, respectively (Table 5, Figure 2).
Table 5
Differential index | PTC (n=81) | NG (n=34) | |||||
---|---|---|---|---|---|---|---|
Nodule | Normal thyroid | Carotid artery | Nodules | Normal thyroid | Carotid artery | ||
Mean difference between VNCn and VNCe | −3.2 | −0.2 | −9.6 | −4.2 | 1.4 | −9.3 | |
The upper limit of the difference between VNCn and VNCe in LOA | 19.8 | 13.3 | 8.4 | 15.1 | 10.4 | 6.1 | |
The lower limit of the difference between VNCn and VNCe in LOA | −26.3 | −13.8 | −27.6 | −23.6 | −7.5 | −24.7 | |
Difference between VNCn and VNCe > LOA | 6.17% | 6.17% | 7.41% | 5.89% | 2.94% | 5.89% |
CT, computed tomography; VNCe, virtual noncontrast images from enhanced scans; VNCn, virtual noncontrast images from nonenhanced scans; PTC, papillary thyroid carcinoma; NG, nodular goiter; LOA, limits of agreement.
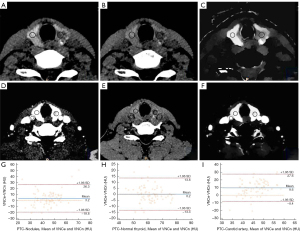
In NG group, the proportion of data points with differences in CT attenuation values in the VNCn and VNCe images of the normal thyroid outside the LOA was 2.94%, while the proportion for the nodules and carotid artery was 5.89% and 5.89%, respectively (Table 5, Figure 3).
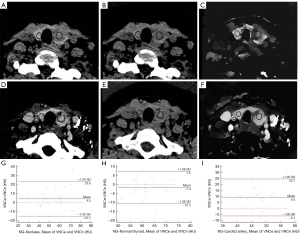
Discussion
In this study, the difference between CIn and VNCn, represented as ΔHUn, was used to reflect the CT attenuation values of intrinsic iodine content. And the CT attenuation values of intrinsic iodine was highly correlated with IDn, suggesting that VNCn images have a certain effectiveness in removing intrinsic iodine. The ΔHUe after enhancement reflects the CT attenuation values of both intrinsic and external iodine agents. The difference between ΔHUe and ΔHUn, referred to as ΔHU, can represent the CT attenuation values of the external iodine agent and is highly correlated with ΔID (the difference in ID between enhanced and nonenhanced scans), indicating that VNCe has some capacity to remove external iodine. The consistency analysis of VNCn and VNCe shows that, except for the normal thyroid in the NG group, the consistency of other indices in the two groups was poor, suggesting a possibility of inconsistent effectiveness.
The thyroid follicle is the basic structure and functional unit of the thyroid gland. During the process of synthesis, storage, and secretion of thyroid hormones, the thyroid follicular epithelial cells obtain iodine from the blood, which is the main source of intrinsic iodine in the thyroid gland (7). In contrast-enhanced CT scans, nonionic contrast agents are commonly used, and iodine ions reach the thyroid gland via the blood during enhancement. While the compound forms may differ, iodine exists in the thyroid gland in the form of I-. VNC images reconstructed using spectral CT use high- and low-energy information to identify iodine substances and remove them from the image, resulting in an iodine-free image (8). In the PTC and NG groups of this study, there was no significant difference in the ΔHUn value of the nodules, suggesting that there was not much difference in intrinsic iodine content between the PTC and NG nodules and that it is not possible to distinguish between these two types of nodules based solely on iodine concentration or CT value. There was a significant difference in the ΔHU value of the nodules between the two groups after enhancement, indicating an increased difference in iodine concentration in the pathological tissues that can aid in distinguishing between the two types of nodules.
The cytological characteristic of PTC is the presence of numerous papillary structures within the lesion. The fibrovascular axis of these papillae serves as the main blood supply for PTC, resulting in significant enhancement of most PTC lesions (9,10). In contrast, in NG lesions, factors such as follicular epithelial cell hyperplasia and residual colloid within the follicular lumen can lead to insufficient blood supply within the lesion. This can result in necrosis, hemorrhage, atrophy of follicular epithelial cells, and continuous proliferation of new follicular epithelial cells in the surrounding vascular-rich areas. Therefore, on CT scans, NG lesions often exhibit central hemorrhage and necrosis, with proliferative thyroid tissue distributed around the lesion (11-14). In this study, the external iodine content after enhancement was found to be higher in PTC nodules compared to NG nodules, indicating a more pronounced enhancement in PTC nodules. Specifically, 9 cases of PTC nodules showed iodine concentrations exceeding 4.0 mg/mL, with the highest concentration reaching 6.4 mg/mL. In comparison, there were 5 cases of NG nodules with iodine concentrations exceeding 4.0 mg/mL, with the highest iodine concentration reaching 5.02 mg/mL.
There was no statistically significant difference in the CT attenuation values of the normal thyroid in VNCn and VNCe images. However, the Bland-Altman plot suggested that the proportion of observations outside the consistency limits for NG-normal thyroid was 2.94%, which is less than 5%. Combining this with the pairwise scatter plots, we believe that the consistency of the observed indicators VNCn and VNCe was poor in both groups. Park et al. compared the CT values of normal thyroid parenchyma on VNC images and enhanced VNC images, and the results showed significant differences, with a proportion of out-of-range data points of 10.9% when the consistency limit was set to 10 HU (15). The results of our study are consistent with the results of Park et al., and we also compared thyroid lesions and carotid arteries. The carotid artery itself does not contain iodine. The VNCn image was similar to the original image, while the enhanced VNCc image obtained after enhancement could reflect the level of iodine contrast agent removal, with poorer consistency compared to VNCn, suggesting incomplete removal of iodine in VNCc. Zhou et al. retrospectively analyzed imaging data from 96 patients with PTC and reported that although VNC images reduced the radiation dose in patients with PTC, there were significant differences in CT values, SNR, and CNR compared to TNC images, and the detection rate of calcification decreased (16). Toepker et al. based on the results of a dual-source spectral system in the abdomen, showed that the difference in CT attenuation values between TNC and enhanced VNC images was higher than 10 HU in adipose tissue, the aorta, and spleen among the 2637 ROIs of various organs and tissues (including the liver, pancreas, spleen, kidney, muscle, fat, etc.) (17). More studies have shown that in regions with high iodine concentration, such as the renal cortex, main portal vein, hepatic vein, and inferior vena cava, iodine cannot be effectively suppressed by VNC, and under low-dose scanning, there is even a tendency for it to be exaggerated (18-20). Therefore, we believe that due to the iodine content in the thyroid itself and its rich blood supply, iodine accumulation is more obvious after contrast agent injection, and VNC cannot completely suppress the iodine, which also leads to significant differences in CT values on VNC images after nonenhanced and enhanced reconstruction. This indicates that enhanced VNC images of the thyroid cannot replace conventional nonenhanced images.
The limitations of this study are as follows: First, we employed a small-sample, single-center design, and the results still need to be validated in larger samples and multiple centers. Second, although fast CT scanning was performed, there may still be incomplete matching between plain and enhanced images due to the influence of respiration or motion, resulting in incomplete consistency of the measured ROIs. Third, the boundaries of the nodules on VNC images were not clear, which had a slight impact on the measured values, and the quality scoring of VNC images should be further investigated and discussed.
Conclusions
VNC images obtained from dual-layer detector spectral CT have some effectiveness in removing both intrinsic and external iodine contrast agents in the thyroid, but their removal efficiency is inconsistent. Therefore, conventional plain scans should still be included in CT examinations of the thyroid to aid in nodule diagnosis.
Acknowledgments
Funding: None.
Footnote
Conflicts of Interest: All authors have completed the ICMJE uniform disclosure form (available at https://qims.amegroups.com/article/view/10.21037/qims-24-116/coif). W.W.D. and X.M.L. are employees of Philips HealthCare. The other authors have no conflicts of interest to declare.
Ethical Statement: The authors are accountable for all aspects of the work in ensuring that questions related to the accuracy or integrity of any part of the work are appropriately investigated and resolved. This study was conducted in accordance with the Declaration of Helsinki (as revised in 2013) and approved by the Ethics Committee of Zhongshan City People’s Hospital (No. K2021-159). The requirement for individual consent for this retrospective analysis was waived.
Open Access Statement: This is an Open Access article distributed in accordance with the Creative Commons Attribution-NonCommercial-NoDerivs 4.0 International License (CC BY-NC-ND 4.0), which permits the non-commercial replication and distribution of the article with the strict proviso that no changes or edits are made and the original work is properly cited (including links to both the formal publication through the relevant DOI and the license). See: https://creativecommons.org/licenses/by-nc-nd/4.0/.
References
- Chinese Journal of Radiology. Dual-layer Detector Spectral CT Clinical Application Collaborative Group. China expert consensus on clinical application of dual-layer spectral detector CT. Chinese Journal of Radiology 2020;54:635-43.
- Megibow AJ, Kambadakone A, Ananthakrishnan L. Dual-Energy Computed Tomography: Image Acquisition, Processing, and Workflow. Radiol Clin North Am 2018;56:507-20. [Crossref] [PubMed]
- Hua CH, Shapira N, Merchant TE, Klahr P, Yagil Y. Accuracy of electron density, effective atomic number, and iodine concentration determination with a dual-layer dual-energy computed tomography system. Med Phys 2018;45:2486-97. [Crossref] [PubMed]
- Durieux P, Gevenois PA, Muylem AV, Howarth N, Keyzer C. Abdominal Attenuation Values on Virtual and True Unenhanced Images Obtained With Third-Generation Dual-Source Dual-Energy CT. AJR Am J Roentgenol 2018;210:1042-58. [Crossref] [PubMed]
- Sun H, Hou XY, Xue HD, Li XG, Jin ZY, Qian JM, Yu JC, Zhu HD. Dual-source dual-energy CT angiography with virtual non-enhanced images and iodine map for active gastrointestinal bleeding: image quality, radiation dose and diagnostic performance. Eur J Radiol 2015;84:884-91. [Crossref] [PubMed]
- Jing M, Sun J, Xi H, Liu Z, Zhang S, Deng L, Han T, Zhang B, Lin X, Zhou J. Abdominal virtual non-contrast images derived from energy spectrum CT to evaluate chemotherapy-related fatty liver disease. Quant Imaging Med Surg 2023;13:669-81. [Crossref] [PubMed]
- Jhaveri K, Shroff MM, Fatterpekar GM, Som PM CT. AJNR Am J Neuroradiol 2003;24:143-6. [PubMed]
- Goo HW, Goo JM, Dual-Energy CT. New Horizon in Medical Imaging. Korean J Radiol 2017;18:555-69. [Crossref] [PubMed]
- Lam AK. Papillary Thyroid Carcinoma: Current Position in Epidemiology, Genomics, and Classification. Methods Mol Biol 2022;2534:1-15. [Crossref] [PubMed]
- Zou Y, Zheng M, Qi Z, Guo Y, Ji X, Huang L, Gong Y, Lu X, Ma G, Xia S. Dual-energy computed tomography could reliably differentiate metastatic from non-metastatic lymph nodes of less than 0.5 cm in patients with papillary thyroid carcinoma. Quant Imaging Med Surg 2021;11:1354-67. [Crossref] [PubMed]
- Knobel M. Etiopathology, clinical features, and treatment of diffuse and multinodular nontoxic goiters. J Endocrinol Invest 2016;39:357-73. [Crossref] [PubMed]
- Wang HB, Shu YY, Han ZJ, Ding JW. Value of CT in evaluating the risk of benign and malignant thyroid nodules. Zhonghua Yi Xue Za Zhi 2017;97:2766-9. [PubMed]
- Li Z, Zhang H, Chen W, Li H. Contrast-Enhanced CT-Based Radiomics for the Differentiation of Nodular Goiter from Papillary Thyroid Carcinoma in Thyroid Nodules. Cancer Manag Res 2022;14:1131-40. [Crossref] [PubMed]
- Li M, Zheng X, Li J, Yang Y, Lu C, Xu H, Yu B, Xiao L, Zhang G, Hua Y. Dual-energy computed tomography imaging of thyroid nodule specimens: comparison with pathologic findings. Invest Radiol 2012;47:58-64. [Crossref] [PubMed]
- Park A, Lee YH, Seo HS. Could both intrinsic and extrinsic iodine be successfully suppressed on virtual non-contrast CT images for detecting thyroid calcification? Jpn J Radiol 2021;39:580-8. [Crossref] [PubMed]
- Zhou J, Zhou Y, Hu H, Shen MP, Ge YQ, Tao XW, Xu XQ, Su GY, Wu FY. Feasibility study of using virtual non-contrast images derived from dual-energy CT to replace true non-contrast images in patients diagnosed with papillary thyroid carcinoma. J Xray Sci Technol 2021;29:711-20. [Crossref] [PubMed]
- Toepker M, Moritz T, Krauss B, Weber M, Euller G, Mang T, Wolf F, Herold CJ, Ringl H. Virtual non-contrast in second-generation, dual-energy computed tomography: reliability of attenuation values. Eur J Radiol 2012;81:e398-405. [Crossref] [PubMed]
- Jamali S, Michoux N, Coche E, Dragean CA. Virtual unenhanced phase with spectral dual-energy CT: Is it an alternative to conventional true unenhanced phase for abdominal tissues? Diagn Interv Imaging 2019;100:503-11. [Crossref] [PubMed]
- Borhani AA, Kulzer M, Iranpour N, Ghodadra A, Sparrow M, Furlan A, Tublin ME. Comparison of true unenhanced and virtual unenhanced (VUE) attenuation values in abdominopelvic single-source rapid kilovoltage-switching spectral CT. Abdom Radiol (NY) 2017;42:710-7. [Crossref] [PubMed]
- Sellerer T, Noël PB, Patino M, Parakh A, Ehn S, Zeiter S, Holz JA, Hammel J, Fingerle AA, Pfeiffer F, Maintz D, Rummeny EJ, Muenzel D, Sahani DV. Dual-energy CT: a phantom comparison of different platforms for abdominal imaging. Eur Radiol 2018;28:2745-55. [Crossref] [PubMed]