Correlation between clinical characteristics and epicardial adipose tissue features in acute myocarditis patients using coronary computed tomography (CT) vascular imaging: a case-control study with retrospective data collection
Introduction
Myocarditis is an inflammatory disease of the myocardium, mostly caused by viral infection, resulting in inflammatory infiltrates within the myocardium (1). It has been established that adipokines secreted by adipose tissue, which can be either pro-inflammatory or anti-inflammatory, play a role in linking obesity to various cardiovascular diseases, including myocarditis and inflammatory cardiomyopathy (2-4). For instance, adiponectin protects the heart from acute viral infection and subsequent pathological cardiac remodeling (5,6). Epicardial adipose tissue (EAT) is a unique type of visceral adipose tissue without distinct boundaries, sharing the same microcirculation with myocardium (7). And it also serves as a source of adipokines that have an impact on both coronary vessels and the myocardium (8). Nevertheless, there has been limited research into the imaging characteristics of EAT in patients with acute myocarditis (AM).
Volume (EAT-Vol) and attenuation (EAT-At) of EAT, as measured on computed tomography (CT) scans, serve as biomarkers for cardiometabolic diseases, including but not limited to type 2 diabetes mellitus, coronary artery disease (CAD), heart failure, and atrial fibrillation (9-13). These parameters provide insights into both the quantity and composition of EAT. Due to the intimate anatomical and functional connection between EAT and the myocardium, as mentioned earlier, our hypothesis was that EAT characteristics derived from cardiac CT imaging in patients with AM would exhibit abnormalities when compared with those of the control group. This study aimed to assess the distinctions in imaging features of EAT between AM and control groups. Furthermore, we examined the correlation between clinical characteristics and EAT features in AM patients, which would have the potential to provide new imaging markers for the diagnosis and prognostic evaluation of myocarditis. Taking into account that attenuation of EAT is associated with fat composition (14) and recognizing the non-uniform nature of this composition, we conducted histogram analysis of EAT-At to obtain quantitative parameters for all participants. This analysis helps us gain a better understanding of the distribution and characteristics of fat within the EAT, providing valuable insights into its composition and potential relevance to AM. We present this article in accordance with the STROBE reporting checklist (available at https://qims.amegroups.com/article/view/10.21037/qims-23-1407/rc).
Methods
Population and design
This is a single-center retrospective case-control study. We screened 156 consecutive adult patients admitted to the hospital for new-onset symptoms and clinically suspected myocarditis from January 2019 to December 2022. Complete baseline blood examinations for cardiac biomarkers (T-troponin, N-terminal pro-B-type natriuretic peptide) and inflammatory indexes, continuous 12-lead electrocardiography (ECG) telemonitoring, transthoracic color Doppler echocardiogram and cardiac magnetic resonance (CMR) were collected from all of the patients. To diagnose AM, we referred to the algorithm of the European Society of Cardiology guidelines (1). Clinically suspected AM was diagnosed when symptomatic patients with chest pain fulfilled one or more diagnostic criteria (new ECG modification, elevated troponin, wall motion abnormalities with preserved left ventricular ejection fractions at echocardiography) or when asymptomatic patients had two or more diagnostic criteria. Clinical history, physical examination, and laboratory results were further analyzed, and those with CAD, systemic immune-mediated or toxic myocarditis were excluded. A definite diagnosis of AM was made in the event of two or more CMR Lake Louise criteria (myocardial edema, hyperemia, and late gadolinium enhancement (LGE) or pathologically diagnosed with AM after endomyocardial biopsy (EMB) (1). Characterizations of AM, including the common symptoms of chest pain and fever, ST-segment and T-wave (ST/T) changes, pericardial effusion (PE), impairment of systolic function, and the need for intensive care during hospitalization, were recorded. The study was conducted in accordance with the Declaration of Helsinki (as revised in 2013). The study was approved by ethics board of Zhongshan Hospital of Fudan University (No. B2020-088R) and informed consent was taken from all the patients.
A control group consisted of age-, sex- and body mass index (BMI)-matched participants who were selected from all subjects who underwent coronary CT angiography (CCTA) for health check-ups in our center with the same CT scanner from January 2017 to August 2020. The detailed inclusion criteria were as follows: (I) Negative coronary vessels observed from CCTA; (II) normal morphology and size of the heart; (III) no history of surgery or invasive procedures involving the lung, mediastinum or heart; and (IV) absence of PE and marked pericardial thickening (>5 mm).
The study proceeded in two main steps. First, EAT imaging measurements were compared between the AM group and the control group. In the second step, the AM group was further stratified into subgroups based on the presence or absence of the six clinical features. Subsequently, EAT-Vol and EAT-At were compared within these six pairs of subgroups. If any clinical features were determined to have a significant association with changes in EAT, then the corresponding subgroups were also compared with the control group for further analysis. An overview of this study is presented in the form of a flow chart, as depicted in Figure 1.
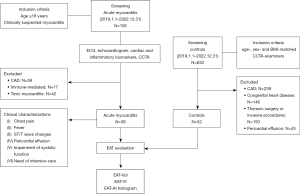
Scan protocol and image reconstruction
All the CCTA scans were performed on the same CT scanner (Aquilion ONE; Toshiba Medical Systems Corporation, Japan) with 320 detector rows. Patients with heart rate >65 beats/min took metoprolol orally approximately 1–1.5 hours before CCTA examination. Sublingual nitroglycerine (0.5 mg) was administered 5 min before scanning, except in the case of contraindications. Intravenous injection of iodinated contrast medium was injected through the right cubital vein with a double-cylinder high-pressure syringe (370 mgI/mL, flow rate 4.0–5.0 mL/s, total amount of injection 0.8 mL/kg) followed by saline (25 mL) injection at the same flow rate. The scanning parameters were as follows: tube voltage 120 kV, current automatically adapted to the patient’s BMI and thoracic anatomy, z-coverage 120–160 mm.
Quantification of EAT
All the images were imported from picture archiving and communication system (PACS) to the postprocessing workstation (uWS-CT, R004, Shanghai United Imaging Healthcare, China). EAT was defined as the visceral fat between the surface of the myocardium and the visceral layer of the pericardium. The pericardium itself was delineated manually, starting from the bifurcation of the pulmonary trunk and extending to the end of the pericardial sac. To ensure measurement consistency, this step was performed by a radiologist (C.Y.) with 8 years of experience. A volume of interest (VOI) encompassing the entire heart was established, and a frequency distribution table of CT attenuation unit value within the VOI was extracted and then exported to a personal computer. To identify fat-containing voxels, a threshold ranging from −190 to −30 Hounsfield units (HU) was applied. The EAT-Vol was calculated as the product of the voxel volume and the count of fat-containing voxels. To account for variations in heart size among individuals and mitigate errors arising from these differences, the volume index of EAT volume (EAT-VI) was defined as the ratio of EAT-Vol to heart volume (Heart-V). The Heart-V equaled the difference between the volume of heart VOI and EAT-Vol.
Histogram analysis of EAT
The histogram analysis of EAT was performed in SPSS v. 19.0.0 software (SPSS, Inc., Chicago, Illinois, USA) using the frequency distribution table which mentioned in the previous step. The frequency distribution data within the interval of (−190, −30 HU) which represented EAT being extracted and imported into the SPSS software for histogram analysis and the following parameters were obtained: mean value (EAT-At-mean), standard deviation (EAT-At-SD), interquartile range (EAT-At-IQR), 5th-percentile value (EAT-At5%), 10th-percentile value (EAT-At10%), 25th-percentile value (EAT-At25%), 75th-percentile value (EAT-At75%), 90th-percentile value (EAT-At90%), 95th-percentile value (EAT-At95%) of the EAT attenuation (Figure 2).
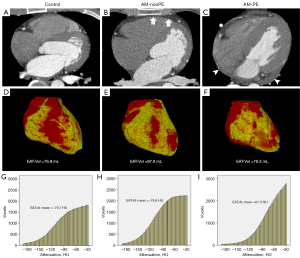
Statistical analysis
Statistical analyses were performed with SPSS v. 19.0.0 software (SPSS, Inc., Chicago, Illinois, USA). Normally distributed variables were presented as mean ± SD. Nonnormally distributed variables were presented as median (25th–75th percentile). Comparisons of characteristics between different groups of patients were performed using the t-test for two groups. Nonnormally distributed continuous variables were compared between patient subgroups using the Mann-Whitney U-test. Categorical variables were compared using the Chi-squared test and P<0.05 (two-sided) was considered indicative of a statistically significant difference. If differences exist, further subdivide the subjects into subgroups based on clinical characteristics and the analysis of variance (ANOVA) test with post-hoc Bonferroni correction was applied.
Results
General characteristics of the enrolled participants
As presented in Table 1, a total of 38 patients with AM and 52 control subjects were included in this study (Table 1). Within the AM group, 22 patients (58%) reported experiencing chest pain, and 17 (45%) had fever during the onset of disease. The other symptoms included cough, dyspnea, fatigue, diarrhea, and abdominal pain. ECG findings indicated ST/T changes, including ST-segment elevation or depression and T-wave inversions, presented in 27 (71%) AM patients. All patients underwent echocardiography, revealing regional left ventricular and/or right ventricular dysfunction in 11 (29%) patients, while PE was observed in 11 (29%) patients. During hospitalization, 21 (55%) patients required intensive care, among which one female patient died during hospitalization.
Table 1
Characteristics | Control group (N=52) | AM group (N=38) | P value |
---|---|---|---|
Male | 34 [65] | 29 [76] | 0.215 |
Age (years) | 31.8±6.8 | 31.5±9.9 | 0.871 |
BMI (kg/m2) | 24.3±3.6 | 24.8±3.2 | 0.510 |
Clinical characteristics | |||
Chest pain | – | 22 [58] | – |
Fever | – | 17 [45] | – |
T wave abnormalities | – | 27 [71] | – |
Impaired systolic function | – | 11 [29] | – |
Pericardial effusion | – | 11 [29] | – |
Critical illness | – | 21 [55] | – |
EAT features | |||
Heart-V (mL) | 703.5±123.3 | 730.8±141.6 | 0.332# |
EAT-VI | 0.11±0.04 | 0.09±0.03 | 0.032* |
EAT-Vol (mL) | 64.7±26.0 | 75.2±22.8 | 0.049* |
EAT-At-mean (HU) | −77.9±5.0 | −75.8±4.4 | 0.044* |
EAT-At-SD (HU) | 33.1±2.4 | 32.4±2.1 | 0.179 |
EAT-At-IQR (HU) | 47.6±4.6 | 46.7±3.9 | 0.309 |
EAT-At 5% (HU) | −139.7±8.7 | −136.6±7.8 | 0.077 |
EAT-At 10% (HU) | −123.4±8.7 | −120.7±7.5 | 0.114 |
EAT-At 25% (HU) | −98.8±7.5 | −96.3±6.5 | 0.091 |
EAT-At 50% (HU) | −73.2±6.2 | −70.9±5.1 | 0.064 |
EAT-At 75% (HU) | −51.2±3.8 | −49.6±3.0 | 0.033* |
EAT-At 90% (HU) | −38.2±1.7 | −37.4±1.4 | 0.019* |
EAT-At 95% (HU) | −33.8±0.8 | −33.5±0.8 | 0.111 |
Data are shown as mean ± SD or number [%]. #, Mann-Whitney U-test, while t-tests were utilized for intergroup comparisons of other quantitative data; *, significance of P value (P<0.05). T wave abnormalities include ST-segment elevation or depression, T-wave inversions. EAT, epicardial adipose tissue; AM, acute myocarditis; BMI, body mass index; EAT-VI, epicardial adipose tissue volume index; EAT-Vol, epicardial adipose tissue volume; EAT-At, epicardial adipose tissue attenuation; HU, Hounsfield units; EAT-At-SD, standard deviation of EAT-At; EAT-At-IQR, interquartile range of EAT-At; SD, standard deviation.
Comparison of EAT measurements between the AM and control groups
The comparison of EAT measurements between the AM and control groups revealed the following findings. The EAT-Vol of the AM group was 75.2±22.8 mL, which was significantly larger than that of the control group (64.7±26.0 mL, P=0.049). Moreover, the EAT-VI remained significantly different between the two groups even when normalized to the volume of the heart (AM vs. control: 0.09±0.03 vs. 0.11±0.04, P=0.032). Regarding EAT-At, the mean EAT-At value in the AM group was −75.8±4.4 HU, which was significantly higher than that in the control group (−77.9±5.0 HU, P=0.044). Further histogram analysis revealed that this change was primarily driven by the increase in EAT-At75% (−49.6±3.0 vs. −51.2±3.8 HU, P=0.033<0.05) and EAT-At90% (−37.4±1.4 vs. −38.2±1.7 HU, P=0.019) in the AM group (Table 1). Through histogram analysis, it was observed that the histogram curves of EAT for all subjects exhibited a pattern of lower values on the left side and higher values on the right side (Figure 2G-2I).
EAT evaluation based on patient characteristics
The mean EAT-At of the AM with PE (AM-PE) subgroup (−71.9±4.0 HU) was significantly higher than that in the AM without PE (AM-nonPE) subgroup (−77.4±3.5 HU, P<0.001). This indicates a significant increase in EAT-At when PE was present. The EAT-Vol was not significantly different between the two subgroups (AM-PE vs. AM-nonPE: 72.2±28.3 vs. 76.4±20.6 mL, P=0.613), even after normalizing to the volume of the heart, as indicated by EAT-VI (0.10±0.04 vs. 0.11±0.03, P=0.584). There were no significant differences observed in EAT-At, EAT-Vol or EAT-VI between AM patients with and without chest pain, fever, ST/T changes, impairment of systolic function or the need for intensive care during hospitalization (Table 2).
Table 2
Clinical characteristics | EAT features | With | Without | P value |
---|---|---|---|---|
Chest pain | EAT-Vol (mL) | 76.0±19.6 | 73.9±27.3 | 0.783 |
EAT-At-mean (HU) | −76.7±3.5 | −74.6±5.2 | 0.149 | |
EAT-VI | 0.11±0.03 | 0.11±0.05 | 0.917 | |
Fever | EAT-Vol (mL) | 79.3±28.3 | 71.8±17.1 | 0.322 |
EAT-At-mean (HU) | −75.4±5.8 | −76.2±2.9 | 0.591 | |
EAT-VI | 0.11±0.04 | 0.10±0.03 | 0.621 | |
ST/T changes | EAT-Vol (mL) | 72.2±21.5 | 82.4±25.2 | 0.217 |
EAT-At-mean (HU) | −75.6±4.5 | −76.4±4.1 | 0.620 | |
EAT-VI | 0.10±0.04 | 0.11±0.04 | 0.746 | |
Impaired systolic function | EAT-Vol (mL) | 75.3±23.4 | 75.1±23.0 | 0.981 |
EAT-At-mean (HU) | −74.7±4.5 | −76.3±4.3 | 0.309 | |
EAT-VI | 0.10±0.04 | 0.11±0.03 | 0.849 | |
Pericardial effusion | EAT-Vol (mL) | 72.2±28.3 | 76.4±20.6 | 0.613 |
EAT-At-mean (HU) | −71.9±4.0 | −77.4±3.5 | <0.001* | |
EAT-VI | 0.10±0.04 | 0.11±0.03 | 0.584 | |
Critical illness | EAT-Vol (mL) | 76.6±21.5 | 73.3±24.9 | 0.660 |
EAT-At-mean (HU) | −75.4±4.6 | −76.2±4.2 | 0.580 | |
EAT-VI | 0.11±0.04 | 0.10±0.03 | 0.369 |
Data are shown as mean ± SD. *, significance of P value (P<0.05). ST/T changes include ST-segment elevation or depression, T-wave inversions. Impaired systolic function includes those impaired systolic left/right ventricle function, with or without increase in wall thickness, with or without dilated left/right ventricle on echocardiography or CMR. EAT, epicardial adipose tissue; EAT-Vol, epicardial adipose tissue volume; HU, Hounsfield units; EAT-VI, epicardial adipose tissue volume index; EAT-At, epicardial adipose tissue attenuation; SD, standard deviation; ST/T, ST-segment and T-wave; CMR, cardiac magnetic resonance.
Comparison of EAT measurements of AM-PE/nonPE patients with control patients
Considering the significant correlation between the presence of PE and the change in EAT-At in AM patients, further comparisons were carried out between the AM subgroups with and without PE and the control group. There was no difference of EAT-Vol among the 3 age groups, even controlling for the difference of heart volume between subjects. Whereas there was significant difference of EAT-At-mean among the three groups (ANOVA P=0.001), and so as to the quantitative histogram parameters, including EAT-At-SD, EAT-At-IQR, EAT-At5%, EAT-At10%, EAT-At25%, EAT-At75%, EAT-At90%, and EAT-At95% (data are shown in Table 3). In the presence of PE, mean EAT-At was significantly higher (−71.9±4.0 HU) when compared to the control group (−77.9±5.0 HU, Bonferroni corrected P=0.001) and AM-nonPE group (−77.4±3.5, Bonferroni corrected P<0.001).
Table 3
Features | AM-PE (N=11) |
AM-nonPE (N=27) |
Control (N=52) |
One-way ANOVA (P value) |
Cp1& (P value) |
Cp2& (P value) |
Cp3& (P value) |
---|---|---|---|---|---|---|---|
Age (years) | 36.2±9.8 | 29.6±9.4 | 31.8±6.8 | 0.079 | 0.075 | 0.312 | 0.760 |
BMI (kg/m2) | 24.0±2.2 | 25.1±3.5 | 24.3±3.6 | 0.540 | >0.99 | >0.99 | 0.991 |
Heart-V (mL) | 748.4±192.5 | 723.6±118.7 | 696.5±122.9 | 0.545 | >0.99 | 0.919 | >0.99 |
EAT-VI | 0.10±0.04 | 0.11±0.03 | 0.09±0.03 | 0.127 | >0.99 | >0.99 | 0.136 |
EAT-Vol (mL) | 72.2±28.3 | 76.4±20.6 | 64.7±26.0 | 0.129 | >0.99 | >0.99 | 0.147 |
EAT-At-mean (HU) | −71.9±4.0 | −77.4±3.5 | −77.9±5.0 | 0.001* | 0.003* | <0.001* | >0.99 |
EAT-At-SD (HU) | 31.2±1.7 | 32.9±2.0 | 33.1±2.4 | 0.042* | 0.100 | 0.038* | >0.99 |
EAT-At-IQR (HU) | 43.9±3.5 | 47.8±3.6 | 47.6±4.6 | 0.025* | 0.036* | 0.029* | >0.99 |
EAT-At 5% (HU) | −131±6.4 | −138.8±7.3 | −139.7±8.7 | 0.006* | 0.025* | 0.005* | >0.99 |
EAT-At 10% (HU) | −114.7±6.3 | −123.1±6.6 | −123.4±8.7 | 0.004* | 0.011* | 0.004* | >0.99 |
EAT-At 25% (HU) | −90.8±6.0 | −98.5±5.3 | −98.8±7.5 | 0.002* | 0.006* | 0.002* | >0.99 |
EAT-At 50% (HU) | −66.3±4.9 | −72.7±3.9 | −73.2±6.2 | 0.001* | 0.004* | 0.001* | >0.99 |
EAT-At 75% (HU) | −46.9±3.0 | −50.7±2.3 | −51.2±3.8 | 0.001* | 0.006* | 0.001* | >0.99 |
EAT-At 90% (HU) | −36.2±1.2 | −37.9±1.1 | −38.2±1.7 | 0.001* | 0.006* | <0.001* | >0.99 |
EAT-At 95% (HU) | −32.8±0.8 | −33.8±0.7 | −33.8±0.8 | 0.001* | 0.002* | 0.001* | >0.99 |
Data are shown as mean ± SD. *, significance of P value; &, Bonferroni correction is applied for post hoc multiple comparisons. Cp1 represent the result of comparison of AM-PE and AM-nonPE; Cp2 represent that of AM-PE and control; Cp3 represent that of control and AM-nonPE. EAT, epicardial adipose tissue; AM-PE, acute myocarditis with pericardial effusion; AM-nonPE, acute myocarditis without pericardial effusion; ANOVA, analysis of variance; BMI, body mass index; EAT-VI, epicardial adipose tissue volume index; EAT-Vol, epicardial adipose tissue volume; EAT-At, epicardial adipose tissue attenuation; HU, Hounsfield units; EAT-At-SD, standard deviation of EAT-At; EAT-At-IQR, interquartile range of EAT-At; SD, standard deviation.
Discussion
In the present study, we found remodeling of the EAT in patients with AM comparing with controls, manifested as changes in volume or CT attenuation of EAT. We report for the first time that AM patients exhibited larger volume and higher mean attenuation of EAT than controls. Notably, changes in attenuation were significantly associated with the presentation of PE in AM patients. When AM was complicated with PE, we observed a significant increase in EAT-At compared to the control. There was a certain degree of reduction in volume which did not significantly differ from both controls and AM patients without PE.
The association of obesity and infection is found not only in nosocomial infections such as sternal wound infections or mediastinitis after cardiac surgery (15) but also in the H1N1 influenza and coronavirus disease 2019 (COVID-19) pandemics (16-18). In these processes, obesity-induced fat tissue remodeling and dysfunction play prominent roles (19). Adipocyte hyperplasia and hypertrophy cause fat tissue expansion, further leading to dysfunctional adipocytes and inflammation. Functionally, EAT secretes adipokines to maintain the equilibrium between protective and detrimental effects on the heart (7). When remodeling of EAT generates a chronic proinflammatory state, the imbalanced production of adipokines could directly or indirectly affect the myocardium. Most commonly, AM is initially caused by viral infection, resulting in distinct lymphocytic infiltration of the myocardium (20). Previous data suggest links of EAT and the secreted adipokines with not only chronic inflammation of myocardium leading to fibrosis and heart failure (21), but also acute inflammation causing myocardial damage (6). The alterations in volume and attenuation observed in this study represent characteristics of remodeled EAT.
The interplay between EAT and the cardiovascular system is bidirectional, therefore, the remodeled EAT identified in this study may be linked to the risk of viral infection in the myocardium and myocardial injury in AM. Unfortunately, due to the lack of follow-up data, our results cannot determine the causal relationship between EAT remodeling and myocarditis. Increased volume of EAT has been found to be negatively correlated with the circulating concentration of adiponectin, which is a widely studied adipokine that can exert anti-inflammatory and immunomodulatory effects (5). Evidence has shown that reduced adiponectin is closely correlated with worse short-term outcomes in patients with fulminant myocarditis (22). Adiponectin can attenuate acute antiviral immune responses by suppressing innate immune responses and reducing the number and activation of NK cells (23). Weight loss and cardiac induction of adiponectin improve the survival and myocardial damage in mice with viral myocarditis (6). Last, increased volume of EAT is associated with the function and structure of the heart, such as abnormal right ventricle geometry, impaired left ventricular strain and diastolic function (10,11,24). Simultaneously, it is noteworthy that some myocarditis patients may exhibit persistent myocardial damage, potentially evolving into inflammatory cardiomyopathy with associated complications such as left ventricular dysfunction, heart failure, or arrhythmias, contributing to an adverse prognosis (25). Consequently, we posit that the remodeling of EAT likely plays a role in the recovery from myocarditis. However, the underlying relationship and mechanisms necessitate further investigation.
Even though the overall AM group showed a significant increment in mean attenuation of EAT, subgroup analysis revealed that this increase was primarily associated with the presence of PE. However, with the increase in attenuation, there was a slight reduction in volume of EAT, and it was no longer significantly larger than control group. Indeed, the histogram analysis also revealed increases in all seven percentiles and the interquartile range. This indicates that the overall attenuation of EAT experienced an increase. Such change is visually represented on the histogram as a rightward shift of the entire curve, meaning it shifted towards higher values (Figure 3). Given the curve’s left-low-right-high morphology, the rightward shift resulted in a reduction in the number of voxels falling within the threshold range of (−190 to −30 HU), ultimately leading to a computed decrease in volume. This explains the inconsistency observed in EAT volume comparisons between the groups with and without PE and the control group. And also, we need to point out that when employing the method of setting attenuation thresholds for quantitative analysis of EAT, the measured EAT volume and mean attenuation are interrelated. Therefore, in studies exploring relationships between EAT and diseases, especially when observing the dynamic changes of EAT, it is essential to consider both EAT volume and mean attenuation values comprehensively. This approach ensures a more comprehensive understanding of the pathophysiological alterations within EAT.
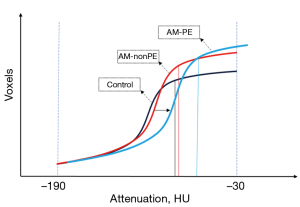
Two mechanisms could potentially explain the increase in attenuation seen here. One mechanism involves local inflammatory activation, a phenomenon observed in postmortem cases of lymphocytic myocarditis, where coexisting inflammation is detected in the left atrial and fat tissue as well (26). The other is transformation of white fat to brown or beige fat tissue with higher activity, which represent a progression to maintain metabolic homeostasis (3,27). In addition, an earlier study on pericarditis reported that lower EAT volume is associated with worse long-term clinical outcome (28), which goes against the common sense that high EAT volume would be associated with an increased risk of future cardiovascular events (12). It is unfortunate that this study did not measure EAT attenuation. However, there is reason to believe that the lower volume may be accompanied by higher attenuation of EAT, which suggests more severe inflammation or dysfunction within the adipose tissue. Future research necessitates long-term follow-up data on the dynamic changes of EAT to explore the prognostic value of EAT imaging features in predicting outcomes for AM.
The present study has some limitations. First, the retrospective and observational cohort design of the study makes it challenging to derive causality. Second, the sample size is small, mainly because CCTA had to be performed on the same scanner because the attenuation is easily affected by the equipment. However, this compromise ensured the reliability of the results. Third, the subgroups based on symptoms are small and overlap with a risk of getting significant P values on coincidence. In the future, with more attention to abnormal EAT in myocarditis, larger observational cohorts may provide more comprehensive evidence. Fourth, none of the AM patients were confirmed by EMB or identified in the etiology. The diagnosis was made by the summation of clinical and imaging findings. Fifth, AM was most frequent in young people and also to minimize confusion with CAD, so their matched controls barely had CCTA data. Even though we carefully selected the control subjects, they might have had some unknown diseases and could bias the true EAT features in young population. Finally, this study did not include patients of older age with a higher prevalence of cardiovascular risk factors and comorbidities. Future research involving such populations should pay closer attention to the heterogeneity of intra- and inter-individual adipose tissue.
In conclusion, the present study found increased EAT volume in AM patients, suggesting that a connection exists between the underlying inflammation of remodeled EAT and the occurrence of AM. And when PE presented, the entire EAT attenuation increased. In addition, we recommend simultaneously evaluating EAT volume and attenuation when quantifying EAT using CT attenuation thresholds.
Acknowledgments
Funding: This research was supported by
Footnote
Reporting Checklist: The authors have completed the STROBE reporting checklist. Available at https://qims.amegroups.com/article/view/10.21037/qims-23-1407/rc
Conflicts of Interest: All authors have completed the ICMJE uniform disclosure form (available at https://qims.amegroups.com/article/view/10.21037/qims-23-1407/coif). S.X. is an employee of Shanghai United Imaging Healthcare Co., Ltd. The other authors have no conflicts of interest to declare.
Ethical Statement:
Open Access Statement: This is an Open Access article distributed in accordance with the Creative Commons Attribution-NonCommercial-NoDerivs 4.0 International License (CC BY-NC-ND 4.0), which permits the non-commercial replication and distribution of the article with the strict proviso that no changes or edits are made and the original work is properly cited (including links to both the formal publication through the relevant DOI and the license). See: https://creativecommons.org/licenses/by-nc-nd/4.0/.
References
- Caforio AL, Pankuweit S, Arbustini E, Basso C, Gimeno-Blanes J, Felix SB, et al. Current state of knowledge on aetiology, diagnosis, management, and therapy of myocarditis: a position statement of the European Society of Cardiology Working Group on Myocardial and Pericardial Diseases. Eur Heart J 2013;34:2636-48, 2648a-2648d.
- Kim JA, Choi KM. Newly Discovered Adipokines: Pathophysiological Link Between Obesity and Cardiometabolic Disorders. Front Physiol 2020;11:568800. [Crossref] [PubMed]
- Morigny P, Boucher J, Arner P, Langin D. Lipid and glucose metabolism in white adipocytes: pathways, dysfunction and therapeutics. Nat Rev Endocrinol 2021;17:276-95. [Crossref] [PubMed]
- Liu J, Li J, Pu H, He W, Zhou X, Tong N, Peng L. Cardiac remodeling and subclinical left ventricular dysfunction in adults with uncomplicated obesity: a cardiovascular magnetic resonance study. Quant Imaging Med Surg 2022;12:2035-50. [Crossref] [PubMed]
- Bobbert P, Scheibenbogen C, Jenke A, Kania G, Wilk S, Krohn S, Stehr J, Kuehl U, Rauch U, Eriksson U, Schultheiss HP, Poller W, Skurk C. Adiponectin expression in patients with inflammatory cardiomyopathy indicates favourable outcome and inflammation control. Eur Heart J 2011;32:1134-47. [Crossref] [PubMed]
- Kanda T, Saegusa S, Takahashi T, Sumino H, Morimoto S, Nakahashi T, Iwai K, Matsumoto M. Reduced-energy diet improves survival of obese KKAy mice with viral myocarditis: induction of cardiac adiponectin expression. Int J Cardiol 2007;119:310-8. [Crossref] [PubMed]
- Iacobellis G. Local and systemic effects of the multifaceted epicardial adipose tissue depot. Nat Rev Endocrinol 2015;11:363-71. [Crossref] [PubMed]
- Banerjee A, Singh J. Remodeling adipose tissue inflammasome for type 2 diabetes mellitus treatment: Current perspective and translational strategies. Bioeng Transl Med 2020;5:e10150. [Crossref] [PubMed]
- Christensen RH, von Scholten BJ, Hansen CS, Jensen MT, Vilsbøll T, Rossing P, Jørgensen PG. Epicardial adipose tissue predicts incident cardiovascular disease and mortality in patients with type 2 diabetes. Cardiovasc Diabetol 2019;18:114. [Crossref] [PubMed]
- de Wit-Verheggen VHW, Altintas S, Spee RJM, Mihl C, van Kuijk SMJ, Wildberger JE, Schrauwen-Hinderling VB, Kietselaer BLJH, van de Weijer T. Pericardial fat and its influence on cardiac diastolic function. Cardiovasc Diabetol 2020;19:129. [Crossref] [PubMed]
- Aliyari Ghasabeh M, Te Riele ASJM, James CA, Chen HSV, Tichnell C, Murray B, Eng J, Kral BG, Tandri H, Calkins H, Kamel IR, Zimmerman SL. Epicardial Fat Distribution Assessed with Cardiac CT in Arrhythmogenic Right Ventricular Dysplasia/Cardiomyopathy. Radiology 2018;289:641-8. [Crossref] [PubMed]
- Eisenberg E, McElhinney PA, Commandeur F, Chen X, Cadet S, Goeller M, Razipour A, Gransar H, Cantu S, Miller RJH, Slomka PJ, Wong ND, Rozanski A, Achenbach S, Tamarappoo BK, Berman DS, Dey D. Deep Learning-Based Quantification of Epicardial Adipose Tissue Volume and Attenuation Predicts Major Adverse Cardiovascular Events in Asymptomatic Subjects. Circ Cardiovasc Imaging 2020;13:e009829. [Crossref] [PubMed]
- Yu W, Chen Y, Zhang F, Liu B, Wang J, Shao X, Yang X, Shi Y, Wang Y. Association of epicardial adipose tissue volume with increased risk of hemodynamically significant coronary artery disease. Quant Imaging Med Surg 2023;13:2582-93. [Crossref] [PubMed]
- Liu Z, Wang S, Wang Y, Zhou N, Shu J, Stamm C, Jiang M, Luo F. Association of epicardial adipose tissue attenuation with coronary atherosclerosis in patients with a high risk of coronary artery disease. Atherosclerosis 2019;284:230-6. [Crossref] [PubMed]
- Ahmed D, Cheema FH, Ahmed YI, Schaefle KJ, Azam SI, Sami SA, Sharif HM. Incidence and predictors of infection in patients undergoing primary isolated coronary artery bypass grafting: a report from a tertiary care hospital in a developing country. J Cardiovasc Surg (Torino) 2011;52:99-104.
- Louie JK, Acosta M, Samuel MC, Schechter R, Vugia DJ, Harriman K, Matyas BTCalifornia Pandemic (H1N1) Working Group. A novel risk factor for a novel virus: obesity and 2009 pandemic influenza A (H1N1). Clin Infect Dis 2011;52:301-12. [Crossref] [PubMed]
- Dalamaga M, Christodoulatos GS, Karampela I, Vallianou N, Apovian CM. Understanding the Co-Epidemic of Obesity and COVID-19: Current Evidence, Comparison with Previous Epidemics, Mechanisms, and Preventive and Therapeutic Perspectives. Curr Obes Rep 2021;10:214-43. [Crossref] [PubMed]
- Parmar C. Bariatric and Metabolic Surgery Can Prevent People with Obesity from COVID-19 Infection. Obes Surg 2021;31:424-5. [Crossref] [PubMed]
- Fuster JJ, Ouchi N, Gokce N, Walsh K. Obesity-Induced Changes in Adipose Tissue Microenvironment and Their Impact on Cardiovascular Disease. Circ Res 2016;118:1786-807. [Crossref] [PubMed]
- Noutsias M, Rohde M, Göldner K, Block A, Blunert K, Hemaidan L, Hummel M, Blohm JH, Lassner D, Kühl U, Schultheiss HP, Volk HD, Kotsch K. Expression of functional T-cell markers and T-cell receptor Vbeta repertoire in endomyocardial biopsies from patients presenting with acute myocarditis and dilated cardiomyopathy. Eur J Heart Fail 2011;13:611-8. [Crossref] [PubMed]
- Packer M. Epicardial Adipose Tissue May Mediate Deleterious Effects of Obesity and Inflammation on the Myocardium. J Am Coll Cardiol 2018;71:2360-72. [Crossref] [PubMed]
- Choi JO, Yun SH, Sung K, Lee YT, Park JI, Ju ES, Lee SC, Park SW, Kim DK, Oh JK, Jeon ES. Thioredoxin, adiponectin and clinical course of acute fulminant myocarditis. Heart 2011;97:1067-73. [Crossref] [PubMed]
- Jenke A, Holzhauser L, Löbel M, Savvatis K, Wilk S, Weithäuser A, Pinkert S, Tschöpe C, Klingel K, Poller W, Scheibenbogen C, Schultheiss HP, Skurk C. Adiponectin promotes coxsackievirus B3 myocarditis by suppression of acute anti-viral immune responses. Basic Res Cardiol 2014;109:408. [Crossref] [PubMed]
- Iacobellis G. Relation of epicardial fat thickness to right ventricular cavity size in obese subjects. Am J Cardiol 2009;104:1601-2. [Crossref] [PubMed]
- Tschöpe C, Ammirati E, Bozkurt B, Caforio ALP, Cooper LT, Felix SB, Hare JM, Heidecker B, Heymans S, Hübner N, Kelle S, Klingel K, Maatz H, Parwani AS, Spillmann F, Starling RC, Tsutsui H, Seferovic P, Van Linthout S. Myocarditis and inflammatory cardiomyopathy: current evidence and future directions. Nat Rev Cardiol 2021;18:169-93. [Crossref] [PubMed]
- Begieneman MP, Emmens RW, Rijvers L, Kubat B, Paulus WJ, Vonk AB, Rozendaal L, Biesbroek PS, Wouters D, Zeerleder S, van Ham M, Heymans S, van Rossum AC, Niessen HW, Krijnen PA. Ventricular myocarditis coincides with atrial myocarditis in patients. Cardiovasc Pathol 2016;25:141-8. [Crossref] [PubMed]
- Gifford A, Towse TF, Walker RC, Avison MJ, Welch EB. Characterizing active and inactive brown adipose tissue in adult humans using PET-CT and MR imaging. Am J Physiol Endocrinol Metab 2016;311:E95-E104. [Crossref] [PubMed]
- Lazaros G, Antonopoulos AS, Oikonomou EK, Vasileiou P, Oikonomou E, Stroumpouli E, Karavidas A, Antoniades C, Tousoulis D. Prognostic implications of epicardial fat volume quantification in acute pericarditis. Eur J Clin Invest 2017;47:129-36. [Crossref] [PubMed]