Retinal thickness and microvascular alterations observed by optical coherence tomography in antineutrophil cytoplasmic antibody-associated vasculitis: a cross-sectional study
Introduction
Antineutrophil cytoplasmic antibody (ANCA)-associated vasculitis (AAV) is a collective term encompassing a set of systemic small vasculitis conditions characterized by the presence of ANCA in serum as their most notable characteristic. Primarily affecting small vessels such as small arteries, small veins, and capillaries, AAV may also involve medium-sized arteries, representing the prevailing form of systemic small vasculitis encountered in clinical settings. The classic manifestations of AAV consist of granulomatosis with polyangiitis (GPA), microscopic polyangiitis (MPA), and eosinophilic granulomatosis with polyangiitis (EGPA) (1). ANCA is the prevailing autoantibody detected in the serum of patients with AAV, serving as a crucial diagnostic criterion for this condition. Based on its fluorescence pattern, ANCA can be categorized into c-ANCA, p-ANCA, and x-ANCA. The pathology of AAV involves the infiltration of various inflammatory cells, including neutrophils, lymphocytes, and macrophages, as well as the presence of celluloid-like necrosis in the vascular wall. These findings suggest that the immune system plays a significant role in the development of AAV. The existing body of evidence provides substantial support for the notion that ANCA plays a direct role in the pathogenesis of small vasculitis (2). As with systemic autoimmune diseases, such as rheumatoid arthritis, Sjögren’s syndrome, seronegative spondyloarthropathy, and so on, AAV can also invade different organs in the body, exhibiting a wide range of symptoms, typically affecting the skin mucosa, respiratory system, peripheral nervous system, and kidneys (3).
In order to promote comprehensive assessment and early diagnosis of AAV, the American College of Rheumatology (ACR)/European League Against Rheumatism (EULAR) 2022 proposed updated classification criteria for vasculitis (4-6). This criterion assigns a certain score to clinical manifestations, laboratory tests, and imaging by means of scoring, and the diagnosis is confirmed when the components are added together and if the score is greater than the diagnostic score. However, ocular signs and symptoms are not adequately represented in this classification criterion.
The eye serves as a unique immune site, which is susceptible to AAV. The association between ocular manifestation and GPA and MPA was initially observed in 1990 (7). About 50% of GPA patients (8-10), a portion of MPA patients, and a few EGPA patients may develop ocular lesions (11). These manifestations can be categorized into conjunctivitis, blepharitis, keratitis, scleritis, iritis, and other localized types, or even epiphora and dry eyes. Additionally, direct sinus infiltration into the orbit can lead to proptosis, nasolacrimal duct obstruction, and optic nerve involvement. The diverse ocular manifestations in individuals with AAV pose a diagnostic challenge for clinicians. Fundus examination often reveals retinal exudation, hemorrhage, vasculitis, and thrombosis (9-11). The presence of diplopia, strabismus, and vision loss in a patient may be attributed to the hindrance of muscle movement and optic nerve impairment caused by the orbital necrotic granuloma. An investigation revealed that strabismus patients had functional abnormalities in multiple regions of the primary visual cortex affecting vision (12). A study conducted by Junek et al. revealed that conjunctivitis and scleritis were the predominant ocular damages observed in AAV. This can be attributed to the vulnerability of conjunctival vessels and the high concentration of scleral collagen to attacks (11,13). Among the complications of AAV, structural injury is highly prevalent (e.g., retroorbital disease, persistent conjunctivitis, and scleritis) and may be difficult to treat (11). It is worth mentioning that ocular lesions may present as the initial symptom in some cases (14). Hence, the significance of discerning ocular signs and symptoms in the prompt identification and evaluation of AAV patients should not be disregarded.
Since AAV is a vascular disease, a vascular examination of the eye is a critical step. Conventional fluorescein fundus angiography (FFA) and indocyanine green angiography (ICGA) remain the most important techniques for the exploration of fundus vascular diseases. However, both methods are invasive and require the injection of a contrast agent, which not only causes some adverse effects to the patient, but also carries the risk of leakage of the contrast agent, affecting the assessment of the fundus vasculature (15). Optical coherence tomography angiography (OCTA), a novel non-invasive imaging technique for retinal and choroidal microvasculature, utilizes laser reflection from the dynamic surface of red blood cells to generate precise, high-resolution images of the vascular structures traversing various regions of the eye (16,17), which produces 3-dimensional microcirculation vascular maps of the retina and choroid without the need for intravascular dye imaging. Simultaneously employing motion contrast imaging and high-resolution volume blood flow information, the integration of the examination of the retinal shallow and deep capillary plexus ultrafine alone, as studied in previous research (18,19), partially mitigates the occurrence of clinical misdiagnosis and enhances the diagnosis and treatment of fundus vascular diseases. Given its good detection sensitivity and specificity, OCTA is currently used in the clinical diagnosis of a variety of retinal diseases, including age-related macular degeneration, diabetic retinopathy, glaucoma, choroidal neovascular disease, centralized plasmacytoid retino-chorioretinopathy, and vascular obstruction (20-25).
Currently, OCTA is not widely used in AAV, and the results of existing reports are controversial. Güven et al. found that the thickness of the retinal nerve fiber layer (RNFL) was decreased by OCTA (26), but Andrada-Elena et al. found that it was increased (27). Therefore, we conducted the present study with 2 objectives: (I) to determine the differences in retinal thickness (RT) and retinal superficial vascular density (SVD) in AAV patients compared to healthy controls (HCs), and (II) to hopefully provide a new method of evaluating and monitoring ocular conditions in the AAV population. We present this article in accordance with the STROBE reporting checklist (available at https://qims.amegroups.com/article/view/10.21037/qims-23-1717/rc).
Methods
Participants
This cross-sectional study was conducted in 2023 at the Department of Ophthalmology and Rheumatology of the First Affiliated Hospital of Nanchang University (Nanchang, China). A total of 16 patients with AAV (32 eyes) were recruited from the Outpatient Department of Rheumatism Immunology (Figure 1), and gender- and age-matched controls were recruited from the Ocular Disease Clinical Research Center. The normal control group consisted of 16 healthy participants (32 eyes), and an ophthalmologist from the medical center evaluated the absence of abnormalities in the eyes of these participants through clinical examination and OCTA imaging. All participants were examined by the same retinal specialist who has nearly 20 years of experience in the field.
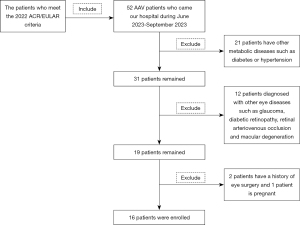
Inclusion and exclusion criteria
All patients consisted of 4 men and 12 women who met the 2022 ACR/EULAR criteria (4-6). The age of the patients ranged from 29 to 76 years, and the duration of the disease ranged from more than 1 month to 10 years. None of the patients showed any other immune system diseases, such as Sjögren’s syndrome or other corneal and ocular diseases. Meanwhile, in order to minimize the influence of non-experimental factor variables, we matched the patients’ age and sex with the corresponding 16 healthy individuals as controls. The basic data for the participants is shown in Table 1.
Table 1
Basic characteristics | Control group | AAV group | t | P value |
---|---|---|---|---|
Age (years) | 52.75±15.02 | 52.69±15.27 | 0.012 | >0.99a |
Gender (male/female) | 4/12 | 4/12 | N/A | >0.99c |
Average vision | 0.98±0.68 | 0.64±0.20 | 6.368 | <0.001b* |
Disease course (years) | N/A | 2.46±3.47 | N/A | N/A |
ESR (mm/h) | N/A | 40.06±33.71 | N/A | N/A |
CRP (mg/L) | N/A | 30.03±38.58 | N/A | N/A |
ANCA+ | 0/16 | 12/16 | N/A | <0.001c* |
Interstitial lung (positive/negative) | N/A | 5/11 | N/A | N/A |
Hematuria (positive/negative) | N/A | 7/9 | N/A | N/A |
Urinary protein (positive/negative) | N/A | 6/10 | N/A | N/A |
Systolic blood pressure (mmHg) | 122.69±4.13 | 123.50±16.09 | −0.196 | 0.85a |
Diastolic blood pressure (mmHg) | 82.69±4.19 | 84.06±10.34 | −0.493 | 0.63a |
Data are presented as mean ± standard deviation or number. a, independent samples t-test; b, Mann-Whitney U test; c, chi-square test. *, statistical difference. AAV, ANCA-associated vasculitis; N/A, not applicable; ESR, erythrocyte sedimentation rate; CRP, C-reactive protein; ANCA, antineutrophil cytoplasmic antibody.
Exclusion criteria for the study included individuals who met any of the following conditions: (I) smokers; (II) diagnosed with other eye diseases such as glaucoma, diabetic retinopathy, retinal arteriovenous occlusion, macular degeneration, ocular trauma, or ocular tumor; (III) experiencing corneal and conjunctival hemorrhage or inflammation in both eyes; (IV) having metabolic diseases such as diabetes or hypertension; (V) currently pregnant or lactating; (VI) having contraindications to pupil dilatation or intolerance to local anesthetic and pupil dilator; (VII) having a history of eye surgery; (VIII) having a refractive error of 6.00 D.
Ethical consideration
The study was approved by the Medical Ethics Committee of the First Affiliated Hospital of Nanchang University (No. 2021039) and followed the principles of the Declaration of Helsinki (as revised in 2013). All 32 participants were notified of the objectives and content of the study and latent risks, and then provided written informed consent to participate.
Clinical examinations
All participants underwent the following clinical and ophthalmic examinations: (I) ANCA testing; (II) erythrocyte sedimentation rate (ESR) and C-reactive protein (CRP) tests as indicators of inflammation; (III) interstitial lung, hematuria, and urinary protein for disease damage complementary assessment; and (IV) vision measurement.
OCTA
We used the RTVue Avanti XR system (Optovue, Fremont, CA, USA) for all participants to present both retinal sections and the microvasculature. The participants were instructed to assume a natural sitting position, with their chin resting on the jaw rest. They were then directed to focus on a blue dot in the eyepiece, which helped minimize blinking and eye movement. The examiner made necessary adjustments based on the refractive state of each participant until an optimal image was achieved. A standardized and specific procedure was followed. First, we employed certain scanning settings, including 70,000 A-scans per second, 840 nm for the central wavelength, 45 nm for the bandwidth, 5 mm for the axial resolution, and 22 µm for the horizontal resolution rate (24). We conducted 5 repeated B-scans (along the x-axis), which located 216 raster points along the y-axis, in a 3×3 mm scan mode for angiography, particularly focused on the fovea with a 3.9-second acquisition duration (24). En-face 3×3 mm OCTA images were obtained through 4 volumetric scans consisting of 2 horizontal and 2 vertical grids (933,120 A-scans). The 270 B-scans (216 y positions × 5 locations) were captured at a rate of 1,080 images per second. Kraus et al. (28) have demonstrated that OCTA is an algorithm that uses orthogonal scan alignment to correct motion artifacts.
After that, we divided the obtained image into 9 regions composed of 3 concentric rounds (0.5, 1.5, and 3 mm in radius) according to the Early Treatment Diabetic Retinopathy Study (ETDRS) (29). including inner superior (IS); outer superior (OS); inner nasal (IN); outer nasal (ON); inner inferior (II); outer inferior (OI); inner temporal (IT); outer temporal (OT); and center (C). We analyzed RT from the full retina—from the internal limiting membrane (ILM) to the retinal pigment epithelium (RPE) and the inner layer—from the ILM to the inner plexiform layer (IPL). The outer layer, reckoned as the difference between the inner layer and the full layer, was calculated indirectly based on the above 2 sets of data. Vascular density was defined as the ratio of the vascular-infused area to the area measured. Using the threshold method, we created 2-dimensional en-face images of the superficial retina (the layer between the vitreous retinal interface and the front boundary of the ganglion cell layer) applied to vessel density measurements. Finally, we allocated a value of 1 (infusion) or 0 (background) to each pixel and scaled the results according to the pixel distance (512 pixels per 3 mm). A brightness gradient image of vascular density observed from the macular center to the 3×3 mm edge was calculated (30). Each participant started with their right (R) eye, and the left (L) eye’s data were reversed to create the R eye’s mirror image. Finally, we averaged and assessed the ocular data from L and R jointly (Figure 2A).
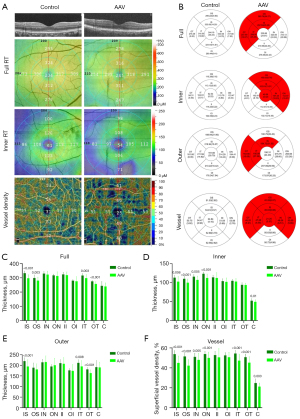
Statistical analysis
This study used the software SPSS 22.0 (IBM Corp., Armonk, NY, USA) to analyze the data collected. Quantitative data were described as mean and standard deviation, whereas qualitative data were expressed as component ratios. In order to explore the differences between the control and AAV groups, different tests were used to test hypotheses on the data. First, we tested the quantitative data for normality. If the data conformed to a normal distribution, we used an independent samples t-test; otherwise, we used a nonparametric test. For qualitative data, we used the chi-square test and Fisher’s exact test for correction. The generalized estimation equation was employed to compare RT and SVD between AAV and controls, and data were adjusted for known confounding variables and to account for the correlation between eyes from the same individual. False discovery rate (FDR) was used to adjust P values. The relationship between RT and systemic and ocular variables was analyzed using univariate and multivariate regression analyses. To analyze the distinction between healthy and AAV participants, receiver operating characteristic (ROC) curves for RT (full, inner, and outer) and SVD were plotted. Spearman’s correlation analysis was performed using GraphPad Prism (version 10.0; GraphPad software, San Diego, CA, USA) to assess the association between disease duration and patient vision as well as IN region of SVD. A P value <0.05 (2-sided) indicated that the difference is statistically significant.
Results
Participants
There were 16 patients with AAV (32 eyes) who underwent selection and were included in this study. The clinical characteristics of control group and AAV group are presented in Table 1. The patients with AAV and control group participants were comparable in the terms of age (the mean age of AAV was 52.69±15.27 years and the mean age of the control was 52.75±15.02, P>0.99) and gender ratio (12 females and 4 male in each group; P>0.99). The mean disease duration in the AAV group was 2.46±3.47 years, mean average vision was 0.64±0.20, ESR was 40.06±33.71 mm/h, CRP was 30.03±38.58 mg/L, systolic blood pressure was 123.50±16.09 mmHg, and diastolic blood pressure was 84.06±10.34 mmHg. Significant differences were observed among the groups in various parameters, including average vision and antiphospholipid antibody, whereas no significant differences were found among other parameters.
Macular RT
The subregional RT in the AAV and control groups is shown in Table 2 and Figure 2B. After adjusting for age, gender, visual acuity, and blood pressure, as well as for P values after multiple correction, the full RT of patients with AAV was found to be significantly reduced in the IS (P<0.001), OS (P=0.003), IT (P=0.003), and OT (P<0.001) regions (Figure 2C), whereas all other regions (IN, ON, II, OI, and C) were not significantly different from HCs (P>0.05).
Table 2
Location | HC (n=16; 32 eyes) | AAV (n=16; 32 eyes) | P value | FDR |
---|---|---|---|---|
Macular full retinal thickness (μm) | ||||
IS | 333.06±14.29 | 297.53±25.25 | <0.001* | <0.001* |
OS | 299.22±20.80 | 280.78±24.27 | 0.001* | 0.003* |
IN | 330.06±14.78 | 323.00±24.24 | 0.17 | 0.24 |
ON | 317.88±7.93 | 313.13±28.98 | 0.13 | 0.21 |
II | 324.44±14.81 | 319.56±22.26 | 0.31 | 0.41 |
OI | 280.44±6.41 | 276.09±32.42 | 0.15 | 0.22 |
IT | 316.50±13.80 | 298.00±25.46 | 0.001* | 0.003* |
OT | 275.38±6.82 | 257.50±15.07 | <0.001* | <0.001* |
C | 244.19±30.23 | 239.88±24.81 | 0.52 | 0.64 |
Macular inner retinal thickness (μm) | ||||
IS | 113.13±5.85 | 102.81±13.21 | 0.003* | 0.006* |
OS | 110.28±6.16 | 100.03±9.76 | <0.001* | <0.001* |
IN | 115.78±5.84 | 107.25±10.23 | 0.002* | 0.005* |
ON | 123.47±4.34 | 112.47±10.37 | <0.001* | <0.001* |
II | 114.22±5.22 | 112.47±13.85 | 0.37 | 0.47 |
OI | 104.09±6.23 | 102.13±10.36 | 0.94 | 0.96 |
IT | 104.91±3.51 | 102.91±8.19 | 0.96 | 0.96 |
OT | 94.31±5.50 | 93.94±4.91 | 0.79 | 0.87 |
C | 52.53±5.22 | 49.56±5.42 | 0.007* | 0.01* |
Macular outer retinal thickness (μm) | ||||
IS | 219.94±15.07 | 194.72±31.55 | <0.001* | <0.001* |
OS | 188.94±21.90 | 180.75±26.40 | 0.08 | 0.14 |
IN | 214.28±14.24 | 215.75±28.60 | 0.80 | 0.87 |
ON | 194.41±9.09 | 200.66±32.26 | 0.88 | 0.93 |
II | 210.22±16.42 | 207.09±29.59 | 0.60 | 0.73 |
OI | 176.34±7.94 | 173.97±30.25 | 0.67 | 0.78 |
IT | 211.59±14.82 | 195.09±27.39 | 0.004* | 0.008* |
OT | 181.06±8.39 | 163.56±15.09 | <0.001* | <0.001* |
C | 191.66±30.79 | 190.31±24.49 | 0.25 | 0.35 |
FDR was used to adjust P values. Data are presented as mean ± standard deviation. Choose independent samples t-test if normal distribution is satisfied, otherwise choose nonparametric test. *, statistical difference. AAV, ANCA-associated vasculitis; HC, healthy control; FDR, false discovery rate; IS, inner superior; OS, outer superior; IN, inner nasal; ON, outer nasal; II, inner inferior; OI, outer inferior; IT, inner temporal; OT, outer temporal; C, center; ANCA, antineutrophil cytoplasmic antibody.
Compared with HCs, the inner RT of AAV patients was lower in the IS (P=0.006), OS (P<0.001), IN (P=0.005), ON (P<0.001), and C (P=0.01) regions than that in HCs (Figure 2D). No significant differences (P>0.05) existed in any of the other regions (IT, OT, II, and OI).
Outer RT of AAV patients showed a reduction in the IS (P<0.001), IT (P=0.008), and OT (P<0.001) regions (Figure 2E). Other regions of the outer layer (OS, IN, ON, II, OI, and C) were similar to the HCs group (P>0.05). In conclusion, IS regions in AAV patients showed significant differences in all layers (full, inner, and outer).
Univariate regression analysis showed a positive correlation between macular RT and gender (β=0.264; P=0.04) and visual acuity (β=0.322; P=0.01), but there was no significant effect on age or blood pressure. Multivariate regression showed significant associations between macular RT and age (β=−0.315; P=0.04), gender (β=0.332; P=0.02), and visual acuity (β=0.352; P=0.005) (Table 3).
Table 3
Parameters | Univariate regression analysis | Multivariate regression analysis | |||
---|---|---|---|---|---|
Regression coefficient (β ± SE) |
P valuea | Regression coefficient (β ± SE) |
P valuea | ||
Age (years) | −0.029±0.163 | 0.82 | −0.315±0.188 | 0.04* | |
Gender (female:male) | 0.264±5.312 | 0.04* | 0.332±6.117 | 0.02* | |
Acuity (log MAR) | 0.322±10.337 | 0.01* | 0.352±10.257 | 0.005* | |
Systolic blood pressure (mmHg) | 0.158±0.207 | 0.21 | 0.132±0.221 | 0.33 | |
Diastolic blood pressure (mmHg) | 0.131±0.308 | 0.30 | −0.002±0.321 | 0.99 |
a, P value was obtained with generalized estimating equation. *, statistical difference. AAV, ANCA-associated vasculitis; SE, standard error; MAR, minimum angle of resolution; ANCA, antineutrophil cytoplasmic antibody.
Macular retinal superficial vessel density
The alteration of macular retinal superficial vessel density (SVD) is shown in Table 4 and Figure 2B. Compared to the healthy group, the SVD of AAV group showed statistically significant differences in IS (P<0.001), OS (P<0.001), IN (P=0.005), ON (P<0.001), IT (P<0.001), OT (P<0.001), and C (P=0.003) (Figure 2F). Only the II and OI regions did not differ significantly from controls.
Table 4
Location (%) | HC (n=16; 32 eyes) | AAV (n=16; 32 eyes) | P value | FDR |
---|---|---|---|---|
IS | 53.81±3.57 | 45.03±6.88 | <0.001* | <0.001* |
OS | 51.53±2.82 | 42.28±6.07 | <0.001* | <0.001* |
IN | 51.09±3.65 | 48.06±6.51 | 0.002* | 0.005* |
ON | 53.88±2.76 | 50.00±5.89 | <0.001* | <0.001* |
II | 52.88±3.70 | 50.63±8.86 | 0.12 | 0.20 |
OI | 52.56±2.82 | 50.72±4.96 | 0.07 | 0.13 |
IT | 54.47±2.90 | 47.28±6.56 | <0.001* | <0.001* |
OT | 50.75±3.02 | 45.25±5.91 | <0.001* | <0.001* |
C | 24.84±4.10 | 21.25±3.57 | 0.001* | 0.003* |
FDR was used to adjust P values. Data are presented as mean ± standard deviation. Choose independent samples t-test if normal distribution is satisfied, otherwise choose nonparametric test. *, statistical difference. AAV, ANCA-associated vasculitis; HC, healthy control; FDR, false discovery rate; IS, inner superior; OS, outer superior; IN, inner nasal; ON, outer nasal; II, inner inferior; OI, outer inferior; IT, inner temporal; OT, outer temporal; C, center; ANCA, antineutrophil cytoplasmic antibody.
ROC curve analysis of RT and SVD
Significant differences between groups were found in IS, full and inner OS, full and outer OT and IT, as well as inner IN, ON, and C regions. We analyzed the ROC curve to find the indicators with the most diagnostic value for AAV. Larger area under the curve (AUC) areas are generally considered to have higher diagnostic efficacy. So, the most sensitive to changes in disease-induced full RT was IS (AUC =0.8892, 95% CI: 0.8041–0.9742, P<0.001); ON (AUC =0.8296, 95% CI: 0.7177–0.9415, P<0.001), which had the most diagnostic value for changes in inner RT in AAV patients; and OT (AUC =0.8413, 95% CI: 0.7412–0.9414, P<0.001) was most sensitive to changes in outer RT. To sum up, the full IS region had the highest diagnostic value for AAV-induced reduction in RT (Figure 3A). The IS (AUC = 0.9121, 95% CI: 0.8322–0.9920, P<0.001) region was also the most sensitive to changes in SVD in patients with AAV (Figure 3B).
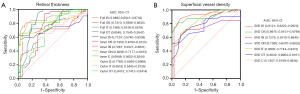
Relationship between disease duration and SVD and visual acuity
We found a negative correlation between the visual acuity (Figure 4A), the SVD of the IN region (Figure 4B), and disease duration in AAV patients. The results were, respectively, r=−0.3922, 95% CI: −0.6579 to −0.0397, P=0.03, and r=−0.4224, 95% CI: −0.6779 to −0.0757, P=0.02.
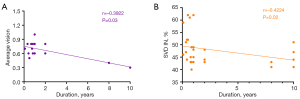
Relationship between RT and SVD
Given that the area of change in inner RT and the area of change in SVD overlap the most (Center, IS, OS, IN, and ON), we boldly speculate that there is a certain correlation between them. However, all the data analysis results were negative, so we have not obtained clear evidence to support our hypothesis. The specific results were as follows: IS (r=0.1397, 95% CI: −0.2299 to 0.4741, P=0.45), OS (r=−0.1748, 95% CI: −0.5015 to 0.1955, P=0.34), IN (r=0.0868, 95% CI: −0.2800 to 0.4315, P=0.64), ON (r=0.2862, 95% CI: −0.0801 to 0.5844, P=0.11), and C (r=0.3180, 95% CI: −0.0453 to 0.6070, P=0.08).
Discussion
AAV, a rare systemic vasculitis, exhibits an insidious onset in certain patients, whereas others experience an acute onset, resulting in multiple organ involvement due to vascular inflammation and necrosis, ultimately leading to fatality (29,30). Despite achieving remission through active treatment, certain patients still require long-term dialysis and potentially kidney transplantation, imposing a substantial burden on both their families and society. Hence, the prompt identification and diagnosis of AAV hold significant importance. The wide-ranging clinical presentations of systemic vasculitis encompass ocular alterations within the range (27,31,32). In light of this, we undertook a study to evaluate RT and microvascular alterations in AAV patients using OCTA measurements. The present study revealed a noteworthy decrease in RT within the full, inner, and outer retinal layers, as well as the SVD, among individuals diagnosed with AAV in comparison to HCs. In order to enhance the elucidation of the regional distribution of RT and SVD reduction, a comprehensive assessment was conducted utilizing ETDRS segmentation. Ultimately, the investigation revealed that the decrease in RT and SVD was most pronounced and had diagnostic value in the IS region.
In the study conducted by Triggianese et al., it was found that there is a positive correlation between higher AAV activity and increased retinal microvascular damage (33). Currently, the Birmingham Vasculitis Disease Activity Score (BVAS) is extensively employed to evaluate the activity of AAV, encompassing various ocular manifestations such as scleritis, conjunctiva, keratitis, and uveitis, as well as retinal alterations including vasculitis, thrombosis, exudation, and bleeding (34,35). AAV, as a systemic disease, can also cause neurological disorders such as peripheral neuropathy, cranial neuropathy, and pituitary involvement, among others. It is important for neurologists to recognize early on that AAV is the fundamental cause of central nervous system diseases (36). The fact that the retina and the brain originate from the same embryonic tissue provides, to some extent, clues for the study of the central nervous system symptoms caused by this disease. Reduced RT may be the earliest sign of neurological disorders (37). However, during the initial phases of the disease, these symptoms have the potential to remain undiagnosed, ultimately progressing to a chronic injury and potentially resulting in a lifelong disability for the patient (29,38).
The retina has 2 blood supply systems: the central retinal artery supplies the inner layer, and the choroidal blood vessels supply the outer layer. Some studies have shown a correlation between RT and retinal blood flow perfusion, which typically involves retinal vessel density (39,40). The initial observation of an AAV patient’s eye revealed histopathological evidence of inflammatory alterations in the choroidal and retinal vessels, along with media thickening and enlargement of choroidal vascular endothelial cells. These changes could potentially contribute to choroidal vascular obstruction, occlusion, and subsequent ischemia (41). Interruption of blood supply leads to loss of nutrition and thinning of the retina. Unfortunately, we have not found a correlation between SVD and inner RT, which may be related to certain limitations and shortcomings in the experiment. Lee et al. found that a decrease in macular thickness leads to a reduction in visual sensitivity (42). As the disease progresses, the degree of visual impairment in patients increases, even leading to blindness, which is consistent with our observed results.
Additionally, it has been postulated that chronic inflammatory stimuli prompt compensatory vasodilation and heightened blood flow density (43). However, our research indicates that the increase in vascular endothelial cell damage and reduction in superficial microvascular density caused by AAV may be more significant compared to the increase in reactive local blood flow. Some assumptions in our research have not been validated, which also reminds us that we should recognize that it is important to acknowledge the limitations of this study. First and foremost, the sample size utilized in this research is relatively small, thus warranting the need for a larger sample size that encompasses stratification by AAV type. This will enable the identification of commonalities across all types and facilitate the determination of optimal parameters for AAV identification, which will contribute to better generalizability of our research. Second, we did not exclude other factors affecting RT and SVD, such as not grouping patients with different treatment regimens, and therefore could not exclude the effect of drug therapy. Third, the size of the eye image we obtained is 3×3 mm, but if we can capture a larger picture, such as 6×6 mm, we will yield more information. A wider field of view will be able to assess more peripheral areas and may reveal a higher degree of involvement under RT and SVD than demonstrated in this study. Although our findings demonstrate the potential of non-invasive and convenient ocular microvascular changes for AAV identification, further analysis is required to elucidate the relationship between AAV and retinal microvessels and thickness, as well as the underlying mechanisms driving fundus vascular changes. Consequently, it is imperative for future studies to involve a greater number of participants in order to explore and enhance these aspects.
Conclusions
RT and SVD were found to be reduced at some specific regions in AAV patients compared to healthy individuals by OCTA. Moreover, with the prolongation of the disease course, the deterioration of the retina’s nourishment caused by the serious destruction of blood vessels, and the serious decline of patients’ visual acuity, early identification and diagnosis of AAV are crucial to the prognosis of patients. OCTA has the potential to be a new approach to diagnose manage and AAV in the future.
Acknowledgments
Funding: This study was supported by
Footnote
Reporting Checklist: The authors have completed the STROBE reporting checklist. Available at https://qims.amegroups.com/article/view/10.21037/qims-23-1717/rc
Conflicts of Interest: All authors have completed the ICMJE uniform disclosure form (available at https://qims.amegroups.com/article/view/10.21037/qims-23-1717/coif). The authors have no conflicts of interest to declare.
Ethical Statement: The authors are accountable for all aspects of the work in ensuring that questions related to the accuracy or integrity of any part of the work are appropriately investigated and resolved. The study was approved by the Medical Ethics Committee of the First Affiliated Hospital of Nanchang University (No. 2021039) and followed the principles of the Declaration of Helsinki (as revised in 2013). All 32 subjects were notified of the objectives and content of the study and latent risks, and then provided written informed consent to participate.
Open Access Statement: This is an Open Access article distributed in accordance with the Creative Commons Attribution-NonCommercial-NoDerivs 4.0 International License (CC BY-NC-ND 4.0), which permits the non-commercial replication and distribution of the article with the strict proviso that no changes or edits are made and the original work is properly cited (including links to both the formal publication through the relevant DOI and the license). See: https://creativecommons.org/licenses/by-nc-nd/4.0/.
References
- Geetha D, Jefferson JA. ANCA-Associated Vasculitis: Core Curriculum 2020. Am J Kidney Dis 2020;75:124-37. [Crossref] [PubMed]
- Pendergraft WF 3rd, Nachman PH. Recent pathogenetic advances in ANCA-associated vasculitis. Presse Med 2015;44:e223-9. [Crossref] [PubMed]
- Nakazawa D, Masuda S, Tomaru U, Ishizu A. Pathogenesis and therapeutic interventions for ANCA-associated vasculitis. Nat Rev Rheumatol 2019;15:91-101. [Crossref] [PubMed]
- Grayson PC, Ponte C, Suppiah R, Robson JC, Craven A, Judge A, Khalid S, Hutchings A, Luqmani RA, Watts RA, Merkel PADCVAS Study Group. 2022 American College of Rheumatology/European Alliance of Associations for Rheumatology Classification Criteria for Eosinophilic Granulomatosis with Polyangiitis. Ann Rheum Dis 2022;81:309-14. [Crossref] [PubMed]
- Robson JC, Grayson PC, Ponte C, Suppiah R, Craven A, Judge A, Khalid S, Hutchings A, Watts RA, Merkel PA, Luqmani RADCVAS Study Group. 2022 American College of Rheumatology/European Alliance of Associations for Rheumatology Classification Criteria for Granulomatosis With Polyangiitis. Arthritis Rheumatol 2022;74:393-9. [Crossref] [PubMed]
- Suppiah R, Robson JC, Grayson PC, Ponte C, Craven A, Khalid S, Judge A, Hutchings A, Merkel PA, Luqmani RA, Watts RA. DCVAS INVESTIGATORS. 2022 American College of Rheumatology/European Alliance of Associations for Rheumatology classification criteria for microscopic polyangiitis. Ann Rheum Dis 2022;81:321-6. [Crossref] [PubMed]
- Pulido JS, Goeken JA, Nerad JA, Sobol WM, Folberg R. Ocular manifestations of patients with circulating antineutrophil cytoplasmic antibodies. Arch Ophthalmol 1990;108:845-50. [Crossref] [PubMed]
- Harper SL, Letko E, Samson CM, Zafirakis P, Sangwan V, Nguyen Q, Uy H, Baltatzis S, Foster CS. Wegener's granulomatosis: the relationship between ocular and systemic disease. J Rheumatol 2001;28:1025-32.
- Macarie SS, Kadar A. Eye involvement in ANCA positive vasculitis. Rom J Ophthalmol 2020;64:3-7.
- Papaliodis GN. Ophthalmologic manifestations of systemic vasculitis. Curr Opin Ophthalmol 2017;28:613-6. [Crossref] [PubMed]
- Junek ML, Zhao L, Garner S, Cuthbertson D, Pagnoux C, Koening CL, Langford CA, McAlear CA, Monach PA, Moreland LW, Rhee RL, Seo P, Specks U, Sreih AG, Warrington K, Wechsler ME, Merkel PA, Khalidi NA. Ocular manifestations of ANCA-associated vasculitis. Rheumatology (Oxford) 2023;62:2517-24. [Crossref] [PubMed]
- Zhu PW, Huang X, Ye L, Jiang N, Zhong YL, Yuan Q, Zhou FQ, Shao Y. Altered intrinsic functional connectivity of the primary visual cortex in youth patients with comitant exotropia: a resting state fMRI study. Int J Ophthalmol 2018;11:668-73. [Crossref] [PubMed]
- Schmidt J, Pulido JS, Matteson EL. Ocular manifestations of systemic disease: antineutrophil cytoplasmic antibody-associated vasculitis. Curr Opin Ophthalmol 2011;22:489-95. [Crossref] [PubMed]
- Hara A, Ohta S, Takata M, Saito K, Torisaki M, Ishida Y, Wada T, Kaneko S. Microscopic polyangiitis with ocular manifestations as the initial presenting sign. Am J Med Sci 2007;334:308-10. [Crossref] [PubMed]
- Do DV, Gower EW, Cassard SD, Boyer D, Bressler NM, Bressler SB, Heier JS, Jefferys JL, Singerman LJ, Solomon SD. Detection of new-onset choroidal neovascularization using optical coherence tomography: the AMD DOC Study. Ophthalmology 2012;119:771-8. [Crossref] [PubMed]
- Al-Sheikh M, Akil H, Pfau M, Sadda SR. Swept-Source OCT Angiography Imaging of the Foveal Avascular Zone and Macular Capillary Network Density in Diabetic Retinopathy. Invest Ophthalmol Vis Sci 2016;57:3907-13. [Crossref] [PubMed]
- Salz DA, de Carlo TE, Adhi M, Moult E, Choi W, Baumal CR, Witkin AJ, Duker JS, Fujimoto JG, Waheed NK. Select Features of Diabetic Retinopathy on Swept-Source Optical Coherence Tomographic Angiography Compared With Fluorescein Angiography and Normal Eyes. JAMA Ophthalmol 2016;134:644-50. [Crossref] [PubMed]
- Spaide RF, Klancnik JM Jr, Cooney MJ. Retinal vascular layers imaged by fluorescein angiography and optical coherence tomography angiography. JAMA Ophthalmol 2015;133:45-50. [Crossref] [PubMed]
- Jia Y, Tan O, Tokayer J, Potsaid B, Wang Y, Liu JJ, Kraus MF, Subhash H, Fujimoto JG, Hornegger J, Huang D. Split-spectrum amplitude-decorrelation angiography with optical coherence tomography. Opt Express 2012;20:4710-25. [Crossref] [PubMed]
- Querques L, Giuffrè C, Corvi F, Zucchiatti I, Carnevali A, De Vitis LA, Querques G, Bandello F. Optical coherence tomography angiography of myopic choroidal neovascularisation. Br J Ophthalmol 2017;101:609-15. [Crossref] [PubMed]
- Sambhav K, Grover S, Chalam KV. The application of optical coherence tomography angiography in retinal diseases. Surv Ophthalmol 2017;62:838-66. [Crossref] [PubMed]
- Durbin MK, An L, Shemonski ND, Soares M, Santos T, Lopes M, Neves C, Cunha-Vaz J. Quantification of Retinal Microvascular Density in Optical Coherence Tomographic Angiography Images in Diabetic Retinopathy. JAMA Ophthalmol 2017;135:370-6. [Crossref] [PubMed]
- Fernández-Vigo JI, Kudsieh B, Shi H, De-Pablo-Gómez-de-Liaño L, Serrano-Garcia I, Ruiz-Moreno JM, Martínez-de-la-Casa JM, García-Feijóo J, Fernández-Vigo JÁ. Normative Database of Peripapillary Vessel Density Measured by Optical Coherence Tomography Angiography and Correlation Study. Curr Eye Res 2020;45:1430-7. [Crossref] [PubMed]
- Kashani AH, Chen CL, Gahm JK, Zheng F, Richter GM, Rosenfeld PJ, Shi Y, Wang RK. Optical coherence tomography angiography: A comprehensive review of current methods and clinical applications. Prog Retin Eye Res 2017;60:66-100. [Crossref] [PubMed]
- Enghelberg M, Gasparian S, Chalam KV. Baseline retinal thickness measurements with a novel integrated imaging system (concurrent optical coherence tomography and fundus photography) positively correlates with spectralis optical coherence tomography. Quant Imaging Med Surg 2022;12:417-24. [Crossref] [PubMed]
- Güven YZ, Akay F, Akmaz B, Solmaz D, Gercik Ö, Akar S. Subclinical Alterations in Retinal Layers and Microvascular Structures with OCTA in ANCA-Associated Vasculitides. Ocul Immunol Inflamm 2023;31:520-5. [Crossref] [PubMed]
- Andrada-Elena M, Ioana TT, Mihaela FM, Irina-Elena C, Andrei TI, Florian B. Wegener's granulomatosis with orbital involvement: case report and literature review. Rom J Ophthalmol 2021;65:93-7. [Crossref] [PubMed]
- Asako K, Takayama M, Kono H, Kikuchi H. Churg-Strauss syndrome complicated by central retinal artery occlusion: case report and a review of the literature. Mod Rheumatol 2011;21:519-23. [Crossref] [PubMed]
- Relhan N, Flynn HW Jr. The Early Treatment Diabetic Retinopathy Study historical review and relevance to today's management of diabetic macular edema. Curr Opin Ophthalmol 2017;28:205-212. [Crossref] [PubMed]
- Mirza S, Raghu Ram AR, Bowling BS, Nessim M. Central retinal artery occlusion and bilateral choroidal infarcts in Wegener's granulomatosis. Eye (Lond) 1999;13:374-6. [Crossref] [PubMed]
- Sfiniadaki E, Tsiara I, Theodossiadis P, Chatziralli I. Ocular Manifestations of Granulomatosis with Polyangiitis: A Review of the Literature. Ophthalmol Ther 2019;8:227-34. [Crossref] [PubMed]
- Huvard MJ, Pecen PE, Palestine AG. The Clinical Characteristics of Noninfectious Occlusive Retinal Vasculitis. Ophthalmol Retina 2022;6:43-8. [Crossref] [PubMed]
- Triggianese P, D'Antonio A, Nesi C, Kroegler B, Di Marino M, Conigliaro P, Modica S, Greco E, Nucci C, Bergamini A, Chimenti MS, Cesareo M. Subclinical microvascular changes in ANCA-vasculitides: the role of optical coherence tomography angiography and nailfold capillaroscopy in the detection of disease-related damage. Orphanet J Rare Dis 2023;18:184. [Crossref] [PubMed]
- Merkel PA, Aydin SZ, Boers M, Direskeneli H, Herlyn K, Seo P, Suppiah R, Tomasson G, Luqmani RA. The OMERACT core set of outcome measures for use in clinical trials of ANCA-associated vasculitis. J Rheumatol 2011;38:1480-6. [Crossref] [PubMed]
- Robson JC, Tomasson G, Milman N, Ashdown S, Boonen A, Casey GC, et al. OMERACT Endorsement of Patient-reported Outcome Instruments in Antineutrophil Cytoplasmic Antibody-associated Vasculitis. J Rheumatol 2017;44:1529-35. [Crossref] [PubMed]
- Lozano-López V, Rodríguez-Lozano B, Losada-Castillo MJ, Delgado-Frías E, Dopazo-Luque D, Serrano-García M. Central retinal artery occlusion in Wegener’s granulomatosis: a diagnostic dilemma. J Ophthalmic Inflamm Infect 2011;1:71-5. [Crossref] [PubMed]
- Chhablani PP, Ambiya V, Nair AG, Bondalapati S, Chhablani J. Retinal Findings on OCT in Systemic Conditions. Semin Ophthalmol 2018;33:525-46. [Crossref] [PubMed]
- Zheng Y, Zhang Y, Cai M, Lai N, Chen Z, Ding M. Central Nervous System Involvement in ANCA-Associated Vasculitis: What Neurologists Need to Know. Front Neurol 2018;9:1166. [Crossref] [PubMed]
- Baker J, Safarzadeh MA, Incognito AV, Jendzjowsky NG, Foster GE, Bird JD, Raj SR, Day TA, Rickards CA, Zubieta-DeUrioste N, Alim U, Wilson RJA. Functional optical coherence tomography at altitude: retinal microvascular perfusion and retinal thickness at 3,800 meters. J Appl Physiol (1985) 2022;133:534-45. [Crossref] [PubMed]
- Salari F, Hatami V, Mohebbi M, Ghassemi F. Assessment of relationship between retinal perfusion and retina thickness in healthy children and adolescents. PLoS One 2022;17:e0273001. [Crossref] [PubMed]
- Cutler WM, Blatt IM. The ocular manifestations of lethal midline granuloma (Wegener's granulomatosis). Am J Ophthalmol 1956;42:21-35. [Crossref] [PubMed]
- Lee SS, Lingham G, Alonso-Caneiro D, Charng J, Chen FK, Yazar S, Mackey DA. Macular Thickness Profile and Its Association With Best-Corrected Visual Acuity in Healthy Young Adults. Transl Vis Sci Technol 2021;10:8. [Crossref] [PubMed]
- McCormick CC, Caballero AR, Balzli CL, Tang A, Weeks A, O'Callaghan RJ. Diverse virulence of Staphylococcus aureus strains for the conjunctiva. Curr Eye Res 2011;36:14-20. [Crossref] [PubMed]