Preoperative prediction of microvascular invasion: new insights into personalized therapy for early-stage hepatocellular carcinoma
Introduction
Hepatocellular carcinoma (HCC) is the predominant form of primary liver cancer and due to its increasing incidence and mortality, is gradually becoming a global health problem (1-3). In recent decades, with the advance of imaging techniques, an increasing number HCC cases are being detected at the early stage. Although there are several curative treatments for early-stage HCC according to clinical practice guidelines (4,5), such as surgical resection (SR), liver transplant (LT) and radiofrequency ablation (RA), over half of the patients also experience early recurrence, which is defined as disease reoccurring within 2 years after curative treatments (6). Microvascular invasion (MVI) has been demonstrated to be an essential independent predictor for early recurrence and poor prognosis after surgery (6-8). Currently, the gold standard for MVI diagnosis remains postoperative histopathology, but this lagging indicator does not allow clinicians to select the most effective treatment for early-stage HCC at the time of therapy selection. For instance, if it is unknown whether a patient has MVI beforehand, SR or RA is commonly recommended as first-line treatment option for early-stage HCC when LT is not feasible or when both the enlistment and surgery criteria are met according to the 2022 edition of the Barcelona Clinic Liver Cancer (BCLC) guideline. This is because the local regulations for enlistment and priority policies may preclude effective LT for early-stage HCC until recurrence is apparent. However, if MVI can be preoperatively diagnosed, patients can consider LT for a better prognosis (9). Therefore, a noninvasive method for preoperative diagnosis of MVI would be highly desirable and may lead to LT being prioritized in those with early-stage HCC and MVI, thus optimizing the limited availability of donor organs and maximizing survival benefits.
In recent decades, imaging has gradually advanced and emerged as a valuable approach for noninvasively and preoperatively detecting MVI-derived pathological changes. Therefore, numerous studies on different techniques, such as computed tomography (CT), magnetic resonance imaging (MRI), ultrasound (US), and positron emission tomography (PET), have been conducted to explore their ability to predict MVI. These advancements in imaging for MVI diagnosis have allowed for the transition from relying on subjective qualitative radiologic features to the more accurate quantitative radiomics analysis. This has been achieved via the modeling of simple sequences or techniques and the combination of multiple indexes and different techniques with clinical biomarkers, which has greatly improved the diagnostic efficiency of MVI (10-12). However, although the studies in this field have achieved excellent results, there is still no consistent imaging criteria for MVI diagnosis, which greatly impedes its clinical application.
As MVI status is critical to informing the management of patients with HCC, preoperative prediction of MVI status is crucial. In this review, we summarize the underlying imaging mechanisms of MVI-related pathological changes and available imaging features and highlight the key advancements in imaging for predicting MVI. Through this, we hope to provide the reader with a useful reference regarding the role of imaging in the preoperative diagnosis and therapeutic management of patients with HCC and MVI. Importantly, preoperative diagnosis with MVI may revolutionize individualized treatment planning at the time of therapy selection for those with early-stage HCC.
Definition of MVI
Before 2013, when Rodríguez-Perálvarez et al. proposed a definition for MVI in a systematic review (8), there was no uniform definition of MVI in HCC among researchers. Rodríguez-Perálvarez et al.’s review defined MVI as the presence of malignant cell nest in vessels, including branches of the arteries, hepatic vein, and portal vein next to the tumor, lined with endothelial cells visible under microscopy. In addition, based on the existing research at the time, they suggested that MVI should include the invasion of small arteries or lymphatic vessels (8). In 2015, China released their first pathology guideline for diagnosing primary liver cancer (13). This guideline recommended that all types of tumor should be sampled based on the seven-point baseline sample collection protocol to ensure the accuracy of primary liver cancer diagnosis (Figure 1). It also indicated that all tissue sections should be assessed in evaluating MVI status and that that risk should be stratified according to the number and distribution of MVI as follows: no MVI; low-risk (M1), <5 MVI sites and ≤1 cm away from the tumor; and high-risk (M2), >5 MVI sites or >1 cm away from the tumor (Figure 1). The efficacy and accuracy of the sampling protocol and grading scheme was verified by a large-sample study, which recruited 16,144 patients from multiple centers in China (13). However, results from other researchers’ indicated that the number of sampling sites and sampling location would affect the detection rate of MVI and lead to false-negative results. Therefore, they concluded that different sizes of tumor require that number and location of the sampling sites be adjusted (14).
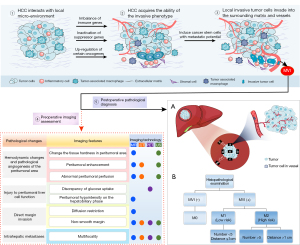
Pathogenesis and pathological changes of MVI
The progression of MVI in HCC involves sequential multistep biological process and a numerous factors, such as the interactions of tumor cells within the local microenvironment and the immune, endocrine, and metabolic status of the patient (15). Studies have identified the following steps in this process: First, a small fraction of cancer stem cells (CSCs) of HCC act as the staple drivers of the aggressive biological progression of HCC through changing multiple regulatory mechanisms, including the upregulation of certain oncogenes, the inactivation of suppressor genes, and the imbalance of immune genes to transform into an invasive phenotype. Second, CSCs respond to contextual signals that induce them to express highly aggressive traits, such as invasiveness and metastatic dissemination, by activating the expression of epithelial-to-mesenchymal transition–inducing transcription factors (16,17). The subsequent epithelial-to-mesenchymal transition leads to a decrease in the amount of cell adhesion proteins and increases the expression of genes involved in cell migration and extracellular matrix degradation. Finally, these cancer cells lose their cell–cell adhesion, break through the tumor-surrounding extracellular matrix, use alternative energy sources and cellular motility to invade into the tumor-surrounding matrix and adjacent vessels, and enter into the hematogenous circulation. Moreover, the cancer cells from the primary tumor metastasize to the microenvironment of a distant tissue via physical translocation and then form metastatic foci through colonization (17). This multistep biological process is accompanied by a series of pathological changes, and these subtle changes can be discerned via biomarkers monitored by imaging and include hemodynamic changes and pathological angiogenesis in the tumor-surrounding area, tumor and peritumoral liver cell’s function change, direct margin invasion, and intrahepatic or extrahepatic metastases (18-23) (Figure 1).
Hemodynamic changes and pathological angiogenesis of the peritumoral area
The pathological manifestation of MVI involves malignant cells nesting in peritumoral vessels (especially the minute portal vein branches) lined with endothelial cells, which cause these vessels to become obstructed. This may reduce portal venous blood flow and lead to the compensatory increase in arterial perfusion in this area (18). A series of studies have confirmed that portal venous arterial shunts is a staple mechanism for the preoperative prediction of MVI (18-20). Moreover, another study also found that MVI is related to the formation of neovasculature in peritumoral tissues (21). Neoplastic tumor cells secrete proangiogenic factors that can recruit various cells in nearby normal liver tissues to the position where angiogenesis is required (22). Meanwhile, increasing expression of placental growth factor and vascular endothelial growth factor receptor can stimulate tumor-surrounding pathologic angiogenesis and induce potential vascular invasion (21). Furthermore, cancer cells invading the microvessels of tumor-surrounding tissues can reduce the number of blood cells and change the local tissue’s hardness; consequently, the hardness discrepancy of the tumor-adjacent tissue and tumor tissue decreases. These manifestation can be quantitatively measured by imaging (23).
Function change of tumor and peritumoral liver cells
Minute portal vein branches around the tumor being blocked by malignant embolus can lead to neoplastic arterial portal shunts, and this alteration in perfusion can result in functional impairment of hepatocytes or Kupffer cells around the tumor. Meanwhile, it can also cause a decrease in the expression of hepatocyte organic anion-transporting polypeptide transporters. Both of these phenomena are related to extracellular liver-specific contrast agent uptake (24), and the related hypointensity on imaging is due to the decrease of contrast agent absorbed by the peritumoral hepatocytes. In addition, HCC with MVI associated with poor tumor grade may have a significantly different level of glucose-6-phosphatase compared with normal hepatocytes and lead a discrepancy in glucose uptake (25).
Direct margin invasion
HCC with aggressive biological tendencies that directly invades the tumor capsule and protrudes into the nontumoral parenchyma can develop a nonsmooth margin, and studies have demonstrated that this can predict MVI-positive status with high accuracy (20,26). Furthermore, when the tumor margin is invaded by the tumor cells, the local microenvironment becomes more complex, with denser cellular structures and local tissue, and is more likely to develop irregular and heterogeneous lesions, thus further limiting the diffusion of water molecules (27).
Metastases
Research suggests that the peritumoral portal vein is an efferent vessel in HCC and is the least resistant channel for tumor cell escape (28). Once cancer cells invade in the efferent vascular cavity and extend beyond the capsule to portal vein branches, they adhere to the portal vein wall and interact with local endothelial cells. This is essential in facilitating tumoral embolism growth and spread, which ultimately disseminate through the portal vein branches to develop multifocal intrahepatic metastatic tumors (28,29). In addition, tumor thrombosis can also be found in hepatic veins or hepatic venous branches, although it has a lower incidence than in the portal system (28). When cancer cells invade into hepatic venous branches, this can lead to the development of metastatic foci in extrahepatic organs.
The clinical importance of MVI
Although significant developments in surgery have improved the outcomes of early-stage HCC, a high risk of early recurrence, even in stage 0 HCC, remains a major challenge in HCC therapy (4). A previous study found that early recurrence, as compared to later recurrence, is more likely to be associated with tumor-related biological aggressiveness, indicated by the presence of MVI and satellitosis, and to poor outcomes (30,31). Other studies have reported that almost one half of tumors ≤2 cm in size also have MVI, suggesting there may be a subtype of HCC with inherently more aggressive biology that can invade the intrahepatic vascular system at an early stage (28,32). Thus, MVI was recognized as a crucial index of prognosis within the T criteria in the eight edition of the American Joint Committee on Cancer staging system (33). The China liver cancer staging system likewise points out that MVI is an important predictor for assessing recurrence risk and therapeutic selections for HCC (34). The 2022 edition of BCLC guideline recommends that BCLC-0 and BCLC-A patients be treated with RA or SR as first-line curative treatment, but if MVI can be diagnosed before treatment, LT should be considered to decrease recurrence risk (9) (Figure 2).
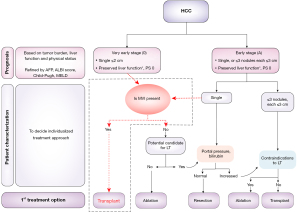
Surgery usually is recommended as curative therapy for early-stage HCC according to current clinical practice guidelines (3,4); however, about 70% of patients treated with SR and 25% of those treated with LT experience recurrence, and the 5-year overall survival (OS) rate is also unsatisfactory, at only 15–20% (35). Numerous studies have demonstrated that MVI is a strong risk factor correlated with these poor outcomes (1,5,9-12). Although it seems that LT could be more beneficial for prognosis than SR, LT requires the high availability of liver grafts, high expense, and highly stringent patient selection. Therefore, various alternative SR methods are used in clinical practice, such as anatomical resection and nonanatomical resection with wide surgical margin, but their effectiveness remains controversial among MVI-positive patients. In addition, in recent years, some studies have evaluated the efficacy of the more aggressive treatment of combining surgical and adjuvant therapies for improving the prognosis of MVI-positive patients (36-40) (Figure 3).
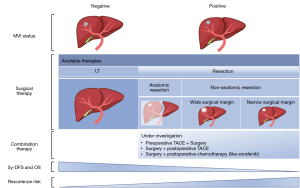
Although MVI is a well-known indicator of early recurrence and poor outcomes after curative therapy, it is a lagging indicator due to the current diagnostic criteria of postoperative histopathology. Meanwhile, preoperative biopsy is not recommended for patients with HCC who meet the clinical diagnostic criteria for undergoing surgery to reduce the risk of tumor rupture, bleeding, and spread. Therefore, a useful noninvasive prediction tool for MVI is urgently needed in the preoperative period for narrowing the gap between clinical therapy and pathology diagnosis. Patients’ expectations need to be guided by the optimal use of the limited availability of donor organs, and so patients should be guided to select the most cost- and outcome-effective therapeutic plan.
Diagnostic imaging features of MVI
Medical imaging has played a crucial role in assessing HCC in recent decades. Clinical practice guidelines (41,42) have recommended imaging modalities, such as US, CT, or MRI, as first-line tools for the screening, diagnosis, staging, and surveillance of HCC. Advanced imaging technology has the advantage of being noninvasive and capable of completely assessing the tumor and host situation, potentially providing insights into the HCC’s biology and heterogeneity before treatment. Therefore, numerous studies have focused on medical imaging as a promising tool for predicting MVI prospectively and have explored the influence of MVI in the prognosis of HCC. A growing body of evidence suggests that several imaging features or combinations of features are correlated with the pathologic and molecular changes derived from MVI (Table S1). These imaging features include morphological features, such as tumor size, nonsmooth tumor margin, and peritumoral enhancement, which can manifest on the conventional enhanced imaging (CT, MRI, or US), and quantitative features based on functional or special scanning sequences, even computer-derived radiomics features (Table 1).
Table 1
MVI-related imaging features | Schematic drawing | Explanation |
---|---|---|
1 Larger tumor | ![]() |
Tumor size >5 cm |
2 Multifocality | ![]() |
Intrahepatic metastases are derived from the main tumor via the portal vein, ≥3 tumors |
3 Nonsmooth tumor margin | ||
3.1 Focal extranodular extension | ![]() |
Focal extranodular extension in the delayed phase |
3.2 Crescent extranodular extension beyond the tumor capsule | ![]() |
Crescent extranodular extension beyond the tumor capsule in the delayed phase |
3.3 Multinodular confluence appearance | ![]() |
Multinodular confluent appearance in the arterial phase |
3.4 Focal infiltrative margin | ![]() |
Tumor with focal infiltrative margin in the delayed phase |
4 Peritumoral enhancement (peritumoral enhancement is considered present when both of the following criteria are satisfied) | ||
4.1 Arterial peritumoral enhancement | ![]() |
A variably shaped hyperintense area outside the tumor in wide contact with the tumor margin on the arterial phase enhancement |
4.2 Isointense with the normal liver tissue on delayed phase or HBP | ![]() |
A variably shaped area outside the tumor in wide contact with the tumor margin that tends to be isointense on the delayed phase or HBP |
5 Peritumoral hypointensity on HBP | ![]() |
An irregular circumferential, wedge-shaped, or flame-like hypointense area of liver parenchyma located outside of the tumor margin |
6 Radiogenomic venous invasion (positive status involves all three of the following conditions) | ||
6.1 Present internal arteries | ![]() |
Persistence of discrete arterial enhancement within the tumor in the venous phase |
6.2 Absent hypodense halo | ![]() |
Lack of a rim of hypoattenuation partially or completely circumscribing the tumor in the portal venous or equilibrium phases of imaging |
6.3 Absent tumor-liver difference | ![]() |
Lack a focal or circumferential sharp transition in attenuation between the tumor and the adjacent liver parenchyma |
MVI, microvascular invasion; HCC, hepatocellular carcinoma; HBP, hepatobiliary phase.
Conventional contrast-enhanced imaging features associated with MVI
Larger tumor and multifocality has also been reported to be able to predict MVI in HCC. A previous study found that larger tumor size had a greater weight for predicting the presence of MVI (20). Similarly, Wei et al. (43) reported that a tumor diameter larger than 5 cm was significantly related to MVI, yielding a sensitivity of 69.2% and a specificity of 61.2% when used as an independent predictor. Other MRI-based research found that when the number of tumors used for predicting MVI increased from ≥3 to ≥4, the specificity could be increased from 88.2% to 91.2% (44). Although there is no uniformity in the criteria of tumor size and number for predicting MVI, it is generally accepted that larger tumor and multifocality are positively related to the presence of MVI.
Nonsmooth tumor margin, a well-known, key feature for predicting MVI (45-49), is an external manifestation related to the aggressive biological behavior of HCC, specifically the invasion of the tumor capsule and tumor-surrounding tissue due to intrahepatic metastasis occurring through the portal venous system. Nonsmooth tumor margin has several forms, including focal extranodular extension, crescent extranodular extension beyond the tumor capsule, multinodular confluence appearance, and focal infiltrative margin. In evaluating the performance of nonsmooth tumor margin for predicting MVI in HCC, the results vary across studies due to the use of different scanning modalities. Renzulli et al. (140 patients) (20) conducted a study based on CT, and their results indicated that nonsmooth tumor margin is highly associated with the presence of MVI (P<0.05). However, Griffin et al. (50), found no correlation between MVI and nonsmooth tumor margin, which was evaluated on enhanced MRI. Currently, nonsmooth tumor margin is widely recognized as an important imaging feature for the clinical prediction of MVI, but the imaging mechanism of different imaging modalities varies, which can lead to differences in evaluation across observers due to the subjective nature of determining whether the tumor margin is smooth.
The tumor capsule, which can be categorized as complete, incomplete, or absent capsule, is defined as a thin, low-signal ring surrounding the tumor on the arterial phase and a high signal on the portal or delayed phase. A plethora of studies have shown that the capsule can act as a protective structure to prevent the tumor from invading adjacent normal liver tissue. Zhao et al. (51) and Chen et al. (52) found that an incomplete tumor capsule was related to MVI (P<0.05). However, other researchers reported that the absence of tumor capsule could not diagnose MVI [diagnostic odds ratio =0.90; 95% confidence interval (CI): 0.64–1.26] (53). In addition, their results also showed that an incomplete capsule had a relatively low sensitivity value of 0.56 (95% CI: 0.42–0.70) and specificity value of 0.68 (95% CI: 0.56–0.79), respectively, in predicting MVI. This may due to the fact that the tumor capsule can prevent tumor invasion to the tumor-surrounding hepatocyte to a degree, but the tumor also may invade the blood vessels in the capsule, thus still resulting in MVI. Consequently, the relationship between an incomplete tumor capsule and MVI is not inconsistent across the different study samples, and so caution is warranted in using tumor capsule to predict MVI in clinical practice.
Peritumoral enhancement is another widely proposed factor for predicting MVI in. MVI involves peritumoral vessels being invaded and obstructed by cancer thrombi from HCC, especially the minute portal vein branches adjacent to the tumor. This can lead to a change of the hemodynamic perfusion of peritumoral liver tissue, which manifests as a loss or reduction of portal blood flow and compensatory arterial hyperperfusion in this area (18). This change may be discerned on conventional enhanced images and is referred to as peritumoral enhancement, which is defined as the presence of a visible tumor-surrounding portion enhanced in the arterial phase and the later reversal of isoattenuation compared with the normal hepatocytes in the portal or delayed phase. Based on this mechanism, many studies based on a variety of imaging modalities have confirmed that peritumoral enhancement is essential for predicting MVI (18-20).
Radiogenomic venous invasion (RVI), first proposed by Banerjee et al. (54), is based on the observation that conventional contrast-enhanced CT can reconstruct global HCC gene expression patterns, in which tumor imaging features are mapped to corresponding gene expression profiles (55,56). Banerjee et al. (54) identified three separate imaging features of RVI: internal arteries, refers to a tumor that exhibits discrete arterial enhancement in the venous phase; hypodense halo, refers to a tumor margin with a partial or complete rim of hypoattenuation; and tumor-liver difference refers to a focal or circumferential sharp transition in attenuation between the tumor and the adjacent nontumor tissue without a hypodense halo. A positive RVI status requires that all the above features be present at the same time. Banerjee et al. found that RVI could predict the presence of MVI in the SR-treated, LT-treated, and overall cohorts with a sensitivity of 84.0%, 66.7%, and 76.1%, respectively, and a specificity of 95.7%, 92.3%, and 93.8%, respectively. Although the research sample on RVI in predicting MVI is still relatively small, it may provide important reference value for the prediction of MVI in the future.
Imaging features associated with MVI based on functional and special scan sequences
Peritumoral hypointensity in the hepatobiliary phase (HBP)
Gadoxetic acid-enhanced MRI is a type of liver-specific intracellular contrast agent imaging and provides a special hepatocellular parenchymal contrast during the late phase. In recent decades, it has been widely used for predicting MVI in HCC. Of note, irregular peritumoral hypointensity in the HBP has been found to be the MRI feature most suggestive of MVI (45,57,58). A hypointense signal is thought to be associated with impairment of organic anion-transporting peptides or the canalicular transporter multidrug resistance-associated protein 2 within hepatocytes or Kupffer cells, which are involved in the uptake of gadoxetic acid (24). A meta-analysis found that the peritumoral hypointensity on HBP was significantly associated with MVI (P<0.05) (59). In addition, Zhou et al. (60) found that peritumoral hypointensity on HBP was an independent risk factor for predicting MVI (P<0.05), yielding an area under the curve (AUC) of 0.883. Currently, although gadoxetic acid-enhanced MRI demonstrates excellent potential in predicting MVI, as a subjective indicator, its efficacy still varies. One study assessed the diagnostic efficacy of gadoxetic acid-enhanced MRI for MVI and found there to be significant interobserver variability, even among more experienced radiologists (61). Nevertheless, the unique peritumoral hypointensity in HBP is still one of the key features for predicting MVI; especially when combined with other features, such as nonsmooth margin and peritumoral enhancement, it can improve the diagnosis of MVI.
Diffusion restriction
Diffusion-weighted imaging (DWI) is a type functional imaging that can quantitatively measure water diffusion and generate different apparent diffusion coefficient (ADC) values. As mentioned above, when MVI in HCC is accompanied by peritumoral tissue and vascular invasion, the local tissue cellularity and integrity of cellular membranes, as well as the hemodynamics, may be altered. In turn, this can lead to the limitation of water diffusion and local microcirculation perfusion changes, and these changes can be reflected by the ADC value. Xu et al. (62) demonstrated that mean ADC (ADCmean) value <1.227×10–3 mm2/s is an independent predictor of MVI. A meta-analysis indicated that both minimum ADC (ADCmin) and true ADC values (D) in MVI-positive tumors were significantly lower than in MVI-negative tumors, and both parameters’ value were under 1.00×10−3 mm2/s in MVI-positive tumors. This may be due to the fact that both ADCmin and D are sensitive to cell count and total nucleic area (63). However, there is still no consistent cutoff value of ADCmin and D for diagnosing MVI (64).
The diffusion kurtosis imaging (DKI) is a special DWI model based on the non-Gaussian distribution model. The mean kurtosis (MK) and mean diffusivity (MD) values are the most widely used parameters in DKI, which can better and more accurately reflect the complexity of microstructure in tumor than can traditional DWI and tend to be associated with aggressive tumor biological behavior (65). One study found that MK had better diagnostic performance than did the conventional ADC value for predicting MVI and that MVI-positive patients had a higher MK value compared to MVI-negative patients (66); however, the effectiveness and reliability of MK and MD remain to be determined in large-sample research.
Intravoxel incoherent motion (IVIM) is another special DWI model, which involves adopting multi-b-value scans and double exponential model fitting. It can simultaneously reflect the diffusion of water molecules in tissues and microvascular blood perfusion, thereby better demonstrating tumor heterogeneity (67). Some studies have shown that intravoxel incoherent motion (IVIM) model-derived D value has a better diagnostic performance for MVI in HCC than does conventional ADC measured with the monoexponential model (66,68-71). Wei et al. (68) found that the D value of the whole tumor with MVI is significantly lower than that without MVI, while the D* and f value of whole tumor had no statistical significance between MVI and non-MVI. Similarly, Zhao et al. (71) reported that among IVIM parameters, only the D value of the tumor was an independent predictor of MVI, with a sensitivity of 66.7% and a specificity of 88.9% when the cutoff value was 1.16×10–3 mm2/s. These results may be explained by the fact that in HCC with MVI, poor differentiation and more densely cell-packed structures are more likely, which can lead to the restriction of molecular diffusion (32); meanwhile, the D value potential may more accurately reflect this feature, as it is related to tissue cellularity (68,71). A recent study proposed using diffusion-derived vessel density (DDVD) to reflect the extent of tissue vessel density of HCC by calculating the signal difference between images when the diffusion gradient is off and images when the diffusion gradient is on (72); this requires only two b-values (with one being b=0 s/mm2), resulting in a significantly shorter scanning time than that of the IVIM protocol. This study found that patients with MVI-positive HCC had a higher DDVD value than did those with MVI-negative HCC due to the fact that those with MVI-positive HCC had a higher blood volume and more differentiated HCC. Although many studies have shown the potential for IVIM to predict MVI in HCC, there is still no consistent scanning protocol. Therefore, multicenter, large-sample studies are needed to confirm the optimal scan protocol of IVIM.
Hardness of tumor and peritumoral liver tissue
Magnetic resonance elastography (MRE) imaging allows for the noninvasive measurement of tissue stiffness and has been widely used to assess liver fibrosis. Recently, a study used a new MRE-based technique, termed slip interface imaging to quantify the degree of tumor-liver tissue adhesion in HCC via shear strain mapping (22). The alterations of the vasculature and extracellular matrix in the tumor-surrounding area caused by MVI leads to a reduction in the difference in shear stiffness of the stiffer tumor and softer normal tumor-surrounding tissue and a change in the sharp boundary of the tumor and normal tumor-surrounding tissue interface (22). Therefore, in MVI-positive patients, MRE-based shear strain at the tumor-liver interface decreases gradually, and the width of the transition in the mechanical properties at the boundary widen, which can be quantified by octahedral shear strain-percentage of low-shear-strain length (OSS-pLSL) derived from MRE. The results of this study indicated that peritumor OSS-pLSL was significantly higher in the MVI-positive group, with the recommended frequency for octahedral shear strain being 30 Hz (22). In addition, another study based on US elastography imaging found that the hardness and strain ratio of tissue near the tumor of an MVI-positive group were obviously lower than those of MVI-negative group in HCC, with peritumoral tissue hardness being identified as a protective feature for MVI (23). In addition, when two tumor features—peritumoral tissue hardness (cutoff 14.150) and maximum cancer diameter (cutoff 43.50 mm)—were used in the prediction model for MVI evaluation, this yielded an accuracy of 75.7% (23). The samples used in the research on elastography to evaluate MVI are still relatively small. In addition, due to the reliance on additional equipment and scanning techniques, its wide application for MVI prediction is also limited.
Iodine-specific maps and iodine concentration
Iodine-specific maps and iodine concentration can quantitatively evaluate abnormal hemodynamic changes of microvascular and provide functional information about the microcirculation of the tumor and tumor-surrounding liver parenchyma. Dual-energy CT (DECT)-based iodine-specific maps can indicate the existence or lack of local iodine in tissues and can be quantified. Quantifying tumor iodine uptake has been used to assess MVI status in patients with HCC, with the result supporting its potential use as a biomarker of MVI (73-75). Miyata et al. (76) reported that those with MVI-positive HCC had a lower arterial iodine concentration value and arterial density value than did those with MVI-negative HCC. Similarly, another study indicated that the spectral CT-specific parameters, including iodine concentration and normalized iodine concentration, in an MVI group were significantly higher than those in a non-MVI group (73). In addition, a lower kiloelectron volt value was found to improve the diagnostic performance from 0.71 (70 keV) to 0.81 (40 keV) (73). The combined analysis of perfusion CT and DECT parameters to evaluate tumor vascularity could provide quantitative data to complement the qualitative visual interpretation, thus increasing the precision of preoperative MVI prediction.
Maximum standardized uptake of tumor
18F-fluorodeoxyglucose (18F-FDG) PET is an important functional molecular imaging that can reflect the metabolic activity and has become an essential technique in cancer imaging (77). Although MVI cannot be directly observed via PET/CT preoperatively, PET/CT can reflect the tumor’s highly invasive and metastatic features and greater metabolic activity in comparison with normal liver tissues. One study reported that the maximum standard uptake value (SUVmax) of tumor of more than 3.2 was an independent predictor of MVI in HCC (78). Likewise, Kornberg et al. (25) found that preoperative PET-positive status, defined as 18F-FDG uptake of the tumor significantly higher than that of in normal tumor-surrounding liver tissues, was related to MVI and had good diagnostic performance in predicting of MVI (sensitivity of 82.3%, specificity of 92%), in patients who underwent LT. They also found that the tumor maximum SUVmax to background liver mean standardized uptake value ratio (TSUVmax/BSUVmean) of 18F-FDG uptake >1 was a reliable preoperative predictor of MVI and tumor recurrence after LT (79). Other researchers also found that TSUVmax/BSUVmean value could preoperatively predict MVI, which at an optimal cutoff value 1.3, yielded a sensitivity of 85.5% and a specificity of 54.9% (80).
However, although numerous studies have demonstrated that the tumor’s SUVmax value is an important predictor of MVI, several studies and a meta-research found low sensitivity and low-to-moderate specificity of 18F-FDG uptake in predicting MVI in HCC, the results suggest that PET/CT should not be used as a reliable maker to exclude MVI (81-83). Ahn et al. indicated that a TSUVmax/BSUVmean >1.2 of 18F-FDG PET may suggest the incidence of MVI in HCC, with a sensitivity of 56% and a specificity of 87% (81). Another study also used the same indicator to predict MVI, but the sensitivity and specificity for predicting MVI before LT or SR were only 55.2% and 69%, respectively (82). Despite the higher 18F-FDG uptake of tumor is a helpful indicator for predicting outcomes of patients with aggressive HCC and an independent predictor of early recurrence in patients with living donor LT. Lee et al. also reported a relatively low diagnostic performance of higher 18F-FDG uptake of tumor for MVI prediction (sensitivity of 45.5%, specificity of 83.9%) (83). Limited resolution and uptake in normal liver tissue is unstable due to susceptibility to hepatitis, cirrhosis, or other factors and can lead to differences in diagnostic performance across various studies. Therefore, the diagnostic efficacy of PET alone remains insufficient.
Radiomics and artificial intelligence
With high-throughput analysis in medical imaging being implemented via a range of data mining algorithms and statistical analysis tools, radiomics has become an emerging, powerful, and noninvasive tool for clinical research. It not only can detect nuanced features from conventional medical images that cannot be seen by the naked eye but also does not rely on radiologists’ potentially biased expertise to determine imaging characteristics. Therefore, the research into quantitative radiomics has grown in recent years and has been increasingly used for MVI prediction in HCC. Radiomics, involves models or nomograms based on various imaging modalities including MRI (83-87), CT (49,88-90), PET (48,91,92), US (93-96). Several meta-analyses found that radiomics has good ability to preoperatively predict MVI status in HCC, with a median AUC ranging from 0.85 to 0.90 across different studies (89,97-99). Zhong et al. (99) also reported that CT and MR-based radiomics models have similar diagnostic efficacy in terms of AUC (0.85 vs. 0.87) and are significantly better than US-based radiomics models (AUC =0.74).
In the era of big data and precision medicine, radiomics modeling is being continually updated to improve the preoperative diagnosis of MVI in HCC. It is widely accepted that single-factor methods for diagnosing MVI often offer relatively poor diagnostic efficacy. An MRI-based radiomics study showed that the efficacy of a multisequence fusion radiomics signature in predicting MVI was better than a single sequence in both the training (AUC: 0.895 vs. 0.754; P=0.002) and validation cohorts (AUC: 0.837 vs. 0.705; P=0.040) (97). Furthermore, Shi et al. used a model based on the radiomics features of tumor derived from PET/CT, whose the diagnostic performance for MVI in HCC was 0.917 and 0.771 in the training and test cohorts, respectively. However, a combination of PET/CT radiomics with contrast-enhanced MRI features (ADC, hypovascular arterial phase enhancement pattern on contrast-enhanced MRI, and nonsmooth tumor margin) yielded a better predictive performance with an AUC of 0.996 and 0.953 in the training and test cohorts, respectively (48). Similarly, other researchers have also explored the role of combining multiple indices in the construction of a model for predicting the preoperative MVI status of patients with HCC. They incorporated several radiographic and clinical characteristics, including higher aspartate aminotransferase (AST) level (>40 U/L), higher alpha-fetoprotein (AFP) level (>400 ng/mL), nonsmooth tumor margin, extrahepatic growth pattern, ill-defined pseudocapsule, peritumoral arterial enhancement, presence of RVI, and higher radiomics score of entire-volumetric interest of the tumor (VOIentire), into the novel RR nomogram. The RR nomogram yielded AUCs of 0.909 in the training cohort and 0.889 in validation cohort for predicting MVI, and its accuracy was higher than that of simple clinical, radiologic, and radiomics features, respectively (49)
Limitations
Although noninvasive imaging methods are playing an increasing important role in predicting MVI of HCC, they still have some limitations. First, conventional contrast-enhanced imaging can only observe some of the morphological features related to MVI, such as nonsmooth tumor margin and peritumoral enhancement, which may be challenging to discern by human vision due to interobserver variability; moreover, they are difficult to compare due to differences in the prediction features and study population (diverse etiologies and sample size) (65). Second, functional and special scanning sequences, such as gadoxetic acid-enhanced MRI, MR, US elastography, and DWI, can improve the preoperative prediction efficiency of MVI to some extent; however, the major disadvantages of these techniques, including the lack of consensus on cutoff points, the use of different scales and techniques by different institutions, and technically challenging acquisitions, have greatly hindered its clinical implementation (22,68). Finally, radiomics and artificial intelligence have achieved some exciting results in predicting MVI of HCC in recent decades; however, it is also difficult for radiomics to traverse the gap between a scientific research and clinically applicable methodologies. For instance there is no consensus regarding image acquisition schemes and segmentation methods, and radiomics models may result in variability in the quantification of radiomic features that are not biologically significant (98,99). Moreover, data-driven radiomics and machine learning are fundamentally incapable of interpreting the biological basis of the observed relationship. This disconnect between the radiomic models and their biological significance inherently limits their use in routine in clinical practice.
Future directions
MVI had been demonstrated to a critical risk indicator for early recurrence and poor prognosis in HCC. Given the shortage of cadaver donors and the challenges in diagnosis MVI preoperatively, many patients with early-stage HCC are MVI are recommended to undergo SR or RA as first-line curative treatment rather than LT, which has been associated with a relatively high recurrence rate and poor OS rate. Therefore, precise identification of MVI status preoperatively in patients with HCC is crucial for clinicians to select the most cost- and outcome-effective individualized therapeutic plan for early-stage HCC. Importantly, HCC with MVI has an aggressive biological behavior. Clinical trials should investigate whether MVI-positive HCC predicted via preoperative imaging modalities should be grade higher in staging guidelines in order to encourage more aggressive treatment regimens and reduce the recurrence rate.
Progress in preoperative diagnosis of MVI by imaging has advanced considerably over the past decades. This has included a shift from morphological and functional features to radiomics and artificial intelligence, from qualitative analysis of traits visible to the naked eyes to quantitative analysis of indiscernible traits (such as tumor size, peritumoral enhancement, and imaging texture), and from single features based on conventional or special scanning methods to fusion radiologic and radiomics signatures using multiple sequences or multiple modalities. These changes have dramatically improved the preoperative efficacy and accuracy in diagnosis MVI in the era of precision medicine. Although there is no consensus regarding this imaging features for diagnosing MVI, it has been widely accepted that combined features are superior to individual features in predicting MVI. On the basis of standardized contrast contrast-enhanced imaging (including Gadoxetic acid-enhanced MRI), combined functional or special scan sequences (such as DWI and perfusion imaging) hold promise for the preoperative prediction of MVI. In the future, large-sample, multicenter, and multinational clinical studies should be conducted to formulate the imaging-based diagnostic criteria of MVI.
Conclusions
MVI is significantly associated with aggressive biological behavior and has been demonstrated to be an independent predictor of early recurrence and poor OS in patients with HCC patients after SR and LT. Advanced imaging technologies hold promise for the reliable, noninvasive, and preoperative prediction of MVI status, which could reduce clinicopathological discrepancies and provide a useful tool for balancing patient desires and the limited pool of donor organs. This review may serve as a valuable reference for clinicians to implement personalized therapy and improve the prognosis of patients with HCC.
Acknowledgments
Funding: This work was supported by
Footnote
Conflicts of Interest: All authors have completed the ICMJE uniform disclosure form (available at https://qims.amegroups.com/article/view/10.21037/qims-24-44/coif). The authors have no conflicts of interest to declare.
Ethical Statement: The authors are accountable for all aspects of the work in ensuring that questions related to the accuracy or integrity of any part of the work are appropriately investigated and resolved.
Open Access Statement: This is an Open Access article distributed in accordance with the Creative Commons Attribution-NonCommercial-NoDerivs 4.0 International License (CC BY-NC-ND 4.0), which permits the non-commercial replication and distribution of the article with the strict proviso that no changes or edits are made and the original work is properly cited (including links to both the formal publication through the relevant DOI and the license). See: https://creativecommons.org/licenses/by-nc-nd/4.0/.
References
- Sung H, Ferlay J, Siegel RL, Laversanne M, Soerjomataram I, Jemal A, Bray F. Global Cancer Statistics 2020: GLOBOCAN Estimates of Incidence and Mortality Worldwide for 36 Cancers in 185 Countries. CA Cancer J Clin 2021;71:209-49. [Crossref] [PubMed]
- Llovet JM, Castet F, Heikenwalder M, Maini MK, Mazzaferro V, Pinato DJ, Pikarsky E, Zhu AX, Finn RS. Immunotherapies for hepatocellular carcinoma. Nat Rev Clin Oncol 2022;19:151-72. [Crossref] [PubMed]
- Yao Y, Civelek AC, Li XF. The application of (18)F-FDG PET/CT imaging for human hepatocellular carcinoma: a narrative review. Quant Imaging Med Surg 2023;13:6268-79. [Crossref] [PubMed]
- EASL Clinical Practice Guidelines. Management of hepatocellular carcinoma. J Hepatol 2018;69:182-236. [Crossref] [PubMed]
- Marrero JA, Kulik LM, Sirlin CB, Zhu AX, Finn RS, Abecassis MM, Roberts LR, Heimbach JK. Diagnosis, Staging, and Management of Hepatocellular Carcinoma: 2018 Practice Guidance by the American Association for the Study of Liver Diseases. Hepatology 2018;68:723-50. [Crossref] [PubMed]
- Dhir M, Melin AA, Douaiher J, Lin C, Zhen WK, Hussain SM, Geschwind JF, Doyle MB, Abou-Alfa GK, Are C. A Review and Update of Treatment Options and Controversies in the Management of Hepatocellular Carcinoma. Ann Surg 2016;263:1112-25. [Crossref] [PubMed]
- Erstad DJ, Tanabe KK. Prognostic and Therapeutic Implications of Microvascular Invasion in Hepatocellular Carcinoma. Ann Surg Oncol 2019;26:1474-93. [Crossref] [PubMed]
- Rodríguez-Perálvarez M, Luong TV, Andreana L, Meyer T, Dhillon AP, Burroughs AK. A systematic review of microvascular invasion in hepatocellular carcinoma: diagnostic and prognostic variability. Ann Surg Oncol 2013;20:325-39. [Crossref] [PubMed]
- Reig M, Forner A, Rimola J, Ferrer-Fàbrega J, Burrel M, Garcia-Criado Á, Kelley RK, Galle PR, Mazzaferro V, Salem R, Sangro B, Singal AG, Vogel A, Fuster J, Ayuso C, Bruix J. BCLC strategy for prognosis prediction and treatment recommendation: The 2022 update. J Hepatol 2022;76:681-93. [Crossref] [PubMed]
- Baheti AD, Dunham GM, Ingraham CR, Moshiri M, Lall C, Park JO, Li D, Katz DS, Madoff DC, Bhargava P. Clinical implications for imaging of vascular invasion in hepatocellular carcinoma. Abdom Radiol (NY) 2016;41:1800-10. [Crossref] [PubMed]
- Reginelli A, Vacca G, Segreto T, Picascia R, Clemente A, Urraro F, Serra N, Vanzulli A, Cappabianca S. Can microvascular invasion in hepatocellular carcinoma be predicted by diagnostic imaging? A critical review. Future Oncol 2018;14:2985-94. [Crossref] [PubMed]
- Lv K, Cao X, Du P, Fu JY, Geng DY, Zhang J. Radiomics for the detection of microvascular invasion in hepatocellular carcinoma. World J Gastroenterol 2022;28:2176-83. [Crossref] [PubMed]
- Sheng X, Ji Y, Ren GP, Lu CL, Yun JP, Chen LH, et al. A standardized pathological proposal for evaluating microvascular invasion of hepatocellular carcinoma: a multicenter study by LCPGC. Hepatol Int 2020;14:1034-47. [Crossref] [PubMed]
- Chen L, Chen S, Zhou Q, Cao Q, Dong Y, Feng S, Xiao H, Wang Y, Liu X, Liao G, Peng Z, Li B, Tan L, Ke Z, Li D, Peng B, Peng S, Zhu L, Liao B, Kuang M. Microvascular Invasion Status and Its Survival Impact in Hepatocellular Carcinoma Depend on Tissue Sampling Protocol. Ann Surg Oncol 2021;28:6747-57. [Crossref] [PubMed]
- Psaila B, Lyden D. The metastatic niche: adapting the foreign soil. Nat Rev Cancer 2009;9:285-93. [Crossref] [PubMed]
- Yang J, Weinberg RA. Epithelial-mesenchymal transition: at the crossroads of development and tumor metastasis. Dev Cell 2008;14:818-29. [Crossref] [PubMed]
- Chaffer CL, Weinberg RA. A perspective on cancer cell metastasis. Science 2011;331:1559-64. [Crossref] [PubMed]
- Matsui O, Kobayashi S, Sanada J, Kouda W, Ryu Y, Kozaka K, Kitao A, Nakamura K, Gabata T. Hepatocelluar nodules in liver cirrhosis: hemodynamic evaluation (angiography-assisted CT) with special reference to multi-step hepatocarcinogenesis. Abdom Imaging 2011;36:264-72. [Crossref] [PubMed]
- Kim H, Park MS, Choi JY, Park YN, Kim MJ, Kim KS, Choi JS, Han KH, Kim E, Kim KW. Can microvessel invasion of hepatocellular carcinoma be predicted by pre-operative MRI? Eur Radiol 2009;19:1744-51. [Crossref] [PubMed]
- Renzulli M, Brocchi S, Cucchetti A, Mazzotti F, Mosconi C, Sportoletti C, Brandi G, Pinna AD, Golfieri R. Can Current Preoperative Imaging Be Used to Detect Microvascular Invasion of Hepatocellular Carcinoma? Radiology 2016;279:432-42. [Crossref] [PubMed]
- Flores A, Asrani SK. Editorial: Magnetic Resonance Elastography and Non-Alcoholic Fatty Liver Disease: Time for an Upgrade? Am J Gastroenterol 2016;111:995-6. [Crossref] [PubMed]
- Li M, Yin Z, Hu B, Guo N, Zhang L, Zhang L, Zhu J, Chen W, Yin M, Chen J, Ehman RL, Wang J MR. Elastography-Based Shear Strain Mapping for Assessment of Microvascular Invasion in Hepatocellular Carcinoma. Eur Radiol 2022;32:5024-32. [Crossref] [PubMed]
- Xu C, Jiang D, Tan B, Shen C, Guo J. Preoperative diagnosis and prediction of microvascular invasion in hepatocellularcarcinoma by ultrasound elastography. BMC Med Imaging 2022;22:88. [Crossref] [PubMed]
- Kim SS, Lee S, Kim MJ. Prognostic factors of gadoxetic acid-enhanced MRI for postsurgical outcomes in multicentric hepatocellular carcinoma. Eur Radiol 2021;31:3405-16. [Crossref] [PubMed]
- Kornberg A, Freesmeyer M, Bärthel E, Jandt K, Katenkamp K, Steenbeck J, Sappler A, Habrecht O, Gottschild D, Settmacher U. 18F-FDG-uptake of hepatocellular carcinoma on PET predicts microvascular tumor invasion in liver transplant patients. Am J Transplant 2009;9:592-600. [Crossref] [PubMed]
- Lee S, Kim SH, Lee JE, Sinn DH, Park CK. Preoperative gadoxetic acid-enhanced MRI for predicting microvascular invasion in patients with single hepatocellular carcinoma. J Hepatol 2017;67:526-34. [Crossref] [PubMed]
- Deng Y, Li J, Xu H, Ren A, Wang Z, Yang D, Yang Z. Diagnostic Accuracy of the Apparent Diffusion Coefficient for Microvascular Invasion in Hepatocellular Carcinoma: A Meta-analysis. J Clin Transl Hepatol 2022;10:642-50. [Crossref] [PubMed]
- Mitsunobu M, Toyosaka A, Oriyama T, Okamoto E, Nakao N. Intrahepatic metastases in hepatocellular carcinoma: the role of the portal vein as an efferent vessel. Clin Exp Metastasis 1996;14:520-9. [Crossref] [PubMed]
- Sugino T, Yamaguchi T, Hoshi N, Kusakabe T, Ogura G, Goodison S, Suzuki T. Sinusoidal tumor angiogenesis is a key component in hepatocellular carcinoma metastasis. Clin Exp Metastasis 2008;25:835-41. [Crossref] [PubMed]
- Jung SM, Kim JM, Choi GS, Kwon CHD, Yi NJ, Lee KW, Suh KS, Joh JW. Characteristics of Early Recurrence After Curative Liver Resection for Solitary Hepatocellular Carcinoma. J Gastrointest Surg 2019;23:304-11. [Crossref] [PubMed]
- Poon RT. Differentiating early and late recurrences after resection of HCC in cirrhotic patients: implications on surveillance, prevention, and treatment strategies. Ann Surg Oncol 2009;16:792-4. [Crossref] [PubMed]
- Pawlik TM, Delman KA, Vauthey JN, Nagorney DM, Ng IO, Ikai I, Yamaoka Y, Belghiti J, Lauwers GY, Poon RT, Abdalla EK. Tumor size predicts vascular invasion and histologic grade: Implications for selection of surgical treatment for hepatocellular carcinoma. Liver Transpl 2005;11:1086-92. [Crossref] [PubMed]
- Chun YS, Pawlik TM, Vauthey JN. 8th Edition of the AJCC Cancer Staging Manual: Pancreas and Hepatobiliary Cancers. Ann Surg Oncol 2018;25:845-7.
- Jin B, Du S, Mao Y, Sang X. Interpretation on the Updated Points of Guidelines for the Diagnosis and Treatment of Primary Liver Cancer (2022 Edition). Medical Journal of Peking Union Medical College hospital 2022;13:785-95.
- He Y, Hu B, Zhu C, Xu W, Ge Y, Hao X, Dong B, Chen X, Dong Q, Zhou X. A Novel Multimodal Radiomics Model for Predicting Prognosis of Resected Hepatocellular Carcinoma. Front Oncol 2022;12:745258. [Crossref] [PubMed]
- Kang I, Jang M, Lee JG, Han DH, Joo DJ, Kim KS, Kim MS, Choi JS, Kim SI, Park YN, Choi GH. Subclassification of Microscopic Vascular Invasion in Hepatocellular Carcinoma. Ann Surg 2021;274:e1170-8. [Crossref] [PubMed]
- Hidaka M, Eguchi S, Okuda K, Beppu T, Shirabe K, Kondo K, Takami Y, Ohta M, Shiraishi M, Ueno S, Nanashima A, Noritomi T, Kitahara K, Fujioka H. Impact of Anatomical Resection for Hepatocellular Carcinoma With Microportal Invasion (vp1): A Multi-institutional Study by the Kyushu Study Group of Liver Surgery. Ann Surg 2020;271:339-46. [Crossref] [PubMed]
- Lim KC, Chow PK, Allen JC, Chia GS, Lim M, Cheow PC, Chung AY, Ooi LL, Tan SB. Microvascular invasion is a better predictor of tumor recurrence and overall survival following surgical resection for hepatocellular carcinoma compared to the Milan criteria. Ann Surg 2011;254:108-13. [Crossref] [PubMed]
- Zhao H, Chen C, Gu S, Yan X, Jia W, Mao L, Qiu Y. Anatomical versus non-anatomical resection for solitary hepatocellular carcinoma without macroscopic vascular invasion: A propensity score matching analysis. J Gastroenterol Hepatol 2017;32:870-8. [Crossref] [PubMed]
- Jung DH, Hwang S, Lee YJ, Kim KH, Song GW, Ahn CS, Moon DB, Lee SG. Small Hepatocellular Carcinoma With Low Tumor Marker Expression Benefits More From Anatomical Resection Than Tumors With Aggressive Biology. Ann Surg 2019;269:511-9. [Crossref] [PubMed]
- Heimbach JK, Kulik LM, Finn RS, Sirlin CB, Abecassis MM, Roberts LR, Zhu AX, Murad MH, Marrero JA. AASLD guidelines for the treatment of hepatocellular carcinoma. Hepatology 2018;67:358-80. [Crossref] [PubMed]
- Zech CJ, Ba-Ssalamah A, Berg T, Chandarana H, Chau GY, Grazioli L, Kim MJ, Lee JM, Merkle EM, Murakami T, Ricke J. B Sirlin C, Song B, Taouli B, Yoshimitsu K, Koh DM. Consensus report from the 8th International Forum for Liver Magnetic Resonance Imaging. Eur Radiol 2020;30:370-82. [Crossref] [PubMed]
- Wei H, Jiang H, Liu X, Qin Y, Zheng T, Liu S, Zhang X, Song B. Can LI-RADS imaging features at gadoxetic acid-enhanced MRI predict aggressive features on pathology of single hepatocellular carcinoma? Eur J Radiol 2020;132:109312. [Crossref] [PubMed]
- Chandarana H, Robinson E, Hajdu CH, Drozhinin L, Babb JS, Taouli B. Microvascular invasion in hepatocellular carcinoma: is it predictable with pretransplant MRI? AJR Am J Roentgenol 2011;196:1083-9. [Crossref] [PubMed]
- Yang H, Han P, Huang M, Yue X, Wu L, Li X, Fan W, Li Q, Ma G, Lei P. The role of gadoxetic acid-enhanced MRI features for predicting microvascular invasion in patients with hepatocellular carcinoma. Abdom Radiol (NY) 2022;47:948-56. [Crossref] [PubMed]
- Chou CT, Chen RC, Lin WC, Ko CJ, Chen CB, Chen YL. Prediction of microvascular invasion of hepatocellular carcinoma: preoperative CT and histopathologic correlation. AJR Am J Roentgenol 2014;203:W253-9. [Crossref] [PubMed]
- Wang P, Nie F, Dong T, Wang G, Wang L, Fan X. Study on correlation between two-dimensional ultrasound, contrast-enhanced ultrasound and microvascular invasion in hepatocellular carcinoma. Clin Hemorheol Microcirc 2022;80:97-106. [Crossref] [PubMed]
- Shi H, Duan Y, Shi J, Zhang W, Liu W, Shen B, Liu F, Mei X, Li X, Yuan Z. Role of preoperative prediction of microvascular invasion in hepatocellular carcinoma based on the texture of FDG PET image: A comparison of quantitative metabolic parameters and MRI. Front Physiol 2022;13:928969. [Crossref] [PubMed]
- Xu X, Zhang HL, Liu QP, Sun SW, Zhang J, Zhu FP, Yang G, Yan X, Zhang YD, Liu XS. Radiomic analysis of contrast-enhanced CT predicts microvascular invasion and outcome in hepatocellular carcinoma. J Hepatol 2019;70:1133-44. [Crossref] [PubMed]
- Griffin N, Addley H, Sala E, Shaw AS, Grant LA, Eldaly H, Davies SE, Prevost T, Alexander GJ, Lomas DJ. Vascular invasion in hepatocellular carcinoma: is there a correlation with MRI? Br J Radiol 2012;85:736-44. [Crossref] [PubMed]
- Zhao H, Hua Y, Dai T, He J, Tang M, Fu X, Mao L, Jin H, Qiu Y. Development and validation of a novel predictive scoring model for microvascular invasion in patients with hepatocellular carcinoma. Eur J Radiol 2017;88:32-40. [Crossref] [PubMed]
- Chen P, Lu J, Zhang T, Zhang X, Liang H, Miao X. The Value of Gadolinium Ethoxybenzyl-Diethylenetriamine Pentaacetic Acid Enhanced MRI in the Prediction of Microvascular Invasion of Hepatocellular Carcinoma. Chinese Journal of Radiology (China) 2019;53:103-8.
- Zhu F, Yang F, Li J, Chen W, Yang W. Incomplete tumor capsule on preoperative imaging reveals microvascular invasion in hepatocellular carcinoma: a systematic review and meta-analysis. Abdom Radiol (NY) 2019;44:3049-57. [Crossref] [PubMed]
- Banerjee S, Wang DS, Kim HJ, Sirlin CB, Chan MG, Korn RL, Rutman AM, Siripongsakun S, Lu D, Imanbayev G, Kuo MD. A computed tomography radiogenomic biomarker predicts microvascular invasion and clinical outcomes in hepatocellular carcinoma. Hepatology 2015;62:792-800. [Crossref] [PubMed]
- Segal E, Sirlin CB, Ooi C, Adler AS, Gollub J, Chen X, Chan BK, Matcuk GR, Barry CT, Chang HY, Kuo MD. Decoding global gene expression programs in liver cancer by noninvasive imaging. Nat Biotechnol 2007;25:675-80. [Crossref] [PubMed]
- Kuo MD, Yamamoto S. Next generation radiologic-pathologic correlation in oncology: Rad-Path 2.0. AJR Am J Roentgenol 2011;197:990-7. [Crossref] [PubMed]
- Kim KA, Kim MJ, Jeon HM, Kim KS, Choi JS, Ahn SH, Cha SJ, Chung YE. Prediction of microvascular invasion of hepatocellular carcinoma: usefulness of peritumoral hypointensity seen on gadoxetate disodium-enhanced hepatobiliary phase images. J Magn Reson Imaging 2012;35:629-34. [Crossref] [PubMed]
- Nishie A, Asayama Y, Ishigami K, Kakihara D, Nakayama T, Ushijima Y, Takayama Y, Shirabe K, Fujita N, Kubo Y, Hirakawa M, Honda H. Clinicopathological significance of the peritumoral decreased uptake area of gadolinium ethoxybenzyl diethylenetriamine pentaacetic acid in hepatocellular carcinoma. J Gastroenterol Hepatol 2014;29:561-7. [Crossref] [PubMed]
- Hu HT, Shen SL, Wang Z, Shan QY, Huang XW, Zheng Q, Xie XY, Lu MD, Wang W, Kuang M. Peritumoral tissue on preoperative imaging reveals microvascular invasion in hepatocellular carcinoma: a systematic review and meta-analysis. Abdom Radiol (NY) 2018;43:3324-30. [Crossref] [PubMed]
- Zhou L, Qu Y, Quan G, Zuo H, Liu M. Nomogram for Predicting Microvascular Invasion in Hepatocellular Carcinoma Using Gadoxetic Acid-Enhanced MRI and Intravoxel Incoherent Motion Imaging. Acad Radiol 2024;31:457-66. [Crossref] [PubMed]
- Min JH, Lee MW, Park HS, Lee DH, Park HJ, Lim S, Choi SY, Lee J, Lee JE, Ha SY, Cha DI, Carriere KC, Ahn JH. Interobserver Variability and Diagnostic Performance of Gadoxetic Acid-enhanced MRI for Predicting Microvascular Invasion in Hepatocellular Carcinoma. Radiology 2020;297:573-81. [Crossref] [PubMed]
- Xu P, Zeng M, Liu K, Shan Y, Xu C, Lin J. Microvascular invasion in small hepatocellular carcinoma: is it predictable with preoperative diffusion-weighted imaging? J Gastroenterol Hepatol 2014;29:330-6. [Crossref] [PubMed]
- Surov A, Caysa H, Wienke A, Spielmann RP, Fiedler E. Correlation Between Different ADC Fractions, Cell Count, Ki-67, Total Nucleic Areas and Average Nucleic Areas in Meningothelial Meningiomas. Anticancer Res 2015;35:6841-6.
- Surov A, Pech M, Omari J, Fischbach F, Damm R, Fischbach K, Powerski M, Relja B, Wienke A. Diffusion-Weighted Imaging Reflects Tumor Grading and Microvascular Invasion in Hepatocellular Carcinoma. Liver Cancer 2021;10:10-24. [Crossref] [PubMed]
- Cao L, Chen J, Duan T, Wang M, Jiang H, Wei Y, Xia C, Zhou X, Yan X, Song B. Diffusion kurtosis imaging (DKI) of hepatocellular carcinoma: correlation with microvascular invasion and histologic grade. Quant Imaging Med Surg 2019;9:590-602. [Crossref] [PubMed]
- Wu B, Jia F, Li X, Zhang M, Han D, Jia Z. Amide Proton Transfer Imaging vs Diffusion Kurtosis Imaging for Predicting Histological Grade of Hepatocellular Carcinoma. J Hepatocell Carcinoma 2020;7:159-68. [Crossref] [PubMed]
- Wang F, Yan CY, Wang CH, Yang Y, Zhang D. The Roles of Diffusion Kurtosis Imaging and Intravoxel Incoherent Motion Diffusion-Weighted Imaging Parameters in Preoperative Evaluation of Pathological Grades and Microvascular Invasion in Hepatocellular Carcinoma. Front Oncol 2022;12:884854. [Crossref] [PubMed]
- Wei Y, Huang Z, Tang H, Deng L, Yuan Y, Li J, Wu D, Wei X, Song B. IVIM improves preoperative assessment of microvascular invasion in HCC. Eur Radiol 2019;29:5403-14. [Crossref] [PubMed]
- Granata V, Fusco R, Catalano O, Guarino B, Granata F, Tatangelo F, Avallone A, Piccirillo M, Palaia R, Izzo F, Petrillo A. Intravoxel incoherent motion (IVIM) in diffusion-weighted imaging (DWI) for Hepatocellular carcinoma: correlation with histologic grade. Oncotarget 2016;7:79357-64. [Crossref] [PubMed]
- Shan Q, Chen J, Zhang T, Yan R, Wu J, Shu Y, Kang Z, He B, Zhang Z, Wang J. Evaluating histologic differentiation of hepatitis B virus-related hepatocellular carcinoma using intravoxel incoherent motion and AFP levels alone and in combination. Abdom Radiol (NY) 2017;42:2079-88. [Crossref] [PubMed]
- Zhao W, Liu W, Liu H, Yi X, Hou J, Pei Y, Liu H, Feng D, Liu L, Li W. Preoperative prediction of microvascular invasion of hepatocellular carcinoma with IVIM diffusion-weighted MR imaging and Gd-EOB-DTPA-enhanced MR imaging. PLoS One 2018;13:e0197488. [Crossref] [PubMed]
- Li XM, Yao DQ, Quan XY, Li M, Chen W, Wáng YXJ. Perfusion of hepatocellular carcinomas measured by diffusion-derived vessel density biomarker: Higher hepatocellular carcinoma perfusion than earlier intravoxel incoherent motion reports. NMR Biomed 2024;37:e5125. [Crossref] [PubMed]
- Yang CB, Zhang S, Jia YJ, Yu Y, Duan HF, Zhang XR, Ma GM, Ren C, Yu N. Dual energy spectral CT imaging for the evaluation of small hepatocellular carcinoma microvascular invasion. Eur J Radiol 2017;95:222-7. [Crossref] [PubMed]
- Lewin M, Laurent-Bellue A, Desterke C, Radu A, Feghali JA, Farah J, Agostini H, Nault JC, Vibert E, Guettier C. Evaluation of perfusion CT and dual-energy CT for predicting microvascular invasion of hepatocellular carcinoma. Abdom Radiol (NY) 2022;47:2115-27. [Crossref] [PubMed]
- Kim TM, Lee JM, Yoon JH, Joo I, Park SJ, Jeon SK, Schmidt B, Martin S. Prediction of microvascular invasion of hepatocellular carcinoma: value of volumetric iodine quantification using preoperative dual-energy computed tomography. Cancer Imaging 2020;20:60. [Crossref] [PubMed]
- Miyata R, Tanimoto A, Wakabayashi G, Shimazu M, Nakatsuka S, Mukai M, Kitajima M. Accuracy of preoperative prediction of microinvasion of portal vein in hepatocellular carcinoma using superparamagnetic iron oxide-enhanced magnetic resonance imaging and computed tomography during hepatic angiography. J Gastroenterol 2006;41:987-95. [Crossref] [PubMed]
- Blodgett TM, Meltzer CC, Townsend DW. PET/CT: form and function. Radiology 2007;242:360-85. [Crossref] [PubMed]
- Kobayashi T, Aikata H, Honda F, Nakano N, Nakamura Y, Hatooka M, Morio K, Morio R, Fukuhara T, Masaki K, Nagaoki Y, Kawaoka T, Tsuge M, Hiramatsu A, Imamura M, Kawakami Y, Ohdan H, Awai K, Chayama K. Preoperative Fluorine 18 Fluorodeoxyglucose Positron Emission Tomography/Computed Tomography for Prediction of Microvascular Invasion in Small Hepatocellular Carcinoma. J Comput Assist Tomogr 2016;40:524-30. [Crossref] [PubMed]
- Kornberg A, Küpper B, Tannapfel A, Büchler P, Krause B, Witt U, Gottschild D, Friess H. Patients with non-[18 F]fludeoxyglucose-avid advanced hepatocellular carcinoma on clinical staging may achieve long-term recurrence-free survival after liver transplantation. Liver Transpl 2012;18:53-61.
- Hyun SH, Eo JS, Song BI, Lee JW, Na SJ, Hong IK, Oh JK, Chung YA, Kim TS, Yun M. Preoperative prediction of microvascular invasion of hepatocellular carcinoma using (18)F-FDG PET/CT: a multicenter retrospective cohort study. Eur J Nucl Med Mol Imaging 2018;45:720-6. [Crossref] [PubMed]
- Ahn SY, Lee JM, Joo I, Lee ES, Lee SJ, Cheon GJ, Han JK, Choi BI. Prediction of microvascular invasion of hepatocellular carcinoma using gadoxetic acid-enhanced MR and (18)F-FDG PET/CT. Abdom Imaging 2015;40:843-51. [Crossref] [PubMed]
- Cheung TT, Chan SC, Ho CL, Chok KS, Chan AC, Sharr WW, Ng KK, Poon RT, Lo CM, Fan ST. Can positron emission tomography with the dual tracers [11 C]acetate and [18 F]fludeoxyglucose predict microvascular invasion in hepatocellular carcinoma? Liver Transpl 2011;17:1218-25.
- Lee SD, Kim SH, Kim YK, Kim C, Kim SK, Han SS, Park SJ. (18)F-FDG-PET/CT predicts early tumor recurrence in living donor liver transplantation for hepatocellular carcinoma. Transpl Int 2013;26:50-60. [Crossref] [PubMed]
- Jiang H, Wei J, Fu F, Wei H, Qin Y, Duan T, Chen W, Xie K, Lee JM, Bashir MR, Wang M, Song B, Tian J. Predicting microvascular invasion in hepatocellular carcinoma: A dual-institution study on gadoxetate disodium-enhanced MRI. Liver Int 2022;42:1158-72. [Crossref] [PubMed]
- Nebbia G, Zhang Q, Arefan D, Zhao X, Wu S. Pre-operative Microvascular Invasion Prediction Using Multi-parametric Liver MRI Radiomics. J Digit Imaging 2020;33:1376-86. [Crossref] [PubMed]
- Chong HH, Yang L, Sheng RF, Yu YL, Wu DJ, Rao SX, Yang C, Zeng MS. Multi-scale and multi-parametric radiomics of gadoxetate disodium-enhanced MRI predicts microvascular invasion and outcome in patients with solitary hepatocellular carcinoma ≤ 5 cm. Eur Radiol 2021;31:4824-38. [Crossref] [PubMed]
- Yang L, Gu D, Wei J, Yang C, Rao S, Wang W, Chen C, Ding Y, Tian J, Zeng M. A Radiomics Nomogram for Preoperative Prediction of Microvascular Invasion in Hepatocellular Carcinoma. Liver Cancer 2019;8:373-86. [Crossref] [PubMed]
- Tong X, Li J. Noninvasively predict the micro-vascular invasion and histopathological grade of hepatocellular carcinoma with CT-derived radiomics. Eur J Radiol Open 2022;9:100424. [Crossref] [PubMed]
- Peng J, Zhang J, Zhang Q, Xu Y, Zhou J, Liu L. A radiomics nomogram for preoperative prediction of microvascular invasion risk in hepatitis B virus-related hepatocellular carcinoma. Diagn Interv Radiol 2018;24:121-7. [Crossref] [PubMed]
- Xu T, Ren L, Liao M, Zhao B, Wei R, Zhou Z, He Y, Zhang H, Chen D, Chen H, Liao W. Preoperative Radiomics Analysis of Contrast-Enhanced CT for Microvascular Invasion and Prognosis Stratification in Hepatocellular Carcinoma. J Hepatocell Carcinoma 2022;9:189-201. [Crossref] [PubMed]
- Kaissis GA, Lohöfer FK, Hörl M, Heid I, Steiger K, Munoz-Alvarez KA, Schwaiger M, Rummeny EJ, Weichert W, Paprottka P, Braren R. Combined DCE-MRI- and FDG-PET enable histopathological grading prediction in a rat model of hepatocellular carcinoma. Eur J Radiol 2020;124:108848. [Crossref] [PubMed]
- Li Y, Zhang Y, Fang Q, Zhang X, Hou P, Wu H, Wang X. Radiomics analysis of [18F]FDG PET/CT for microvascular invasion and prognosis prediction in very-early- and early-stage hepatocellular carcinoma. Eur J Nucl Med Mol Imaging 2021;48:2599-614.
- Hu HT, Wang Z, Huang XW, Chen SL, Zheng X, Ruan SM, Xie XY, Lu MD, Yu J, Tian J, Liang P, Wang W, Kuang M. Ultrasound-based radiomics score: a potential biomarker for the prediction of microvascular invasion in hepatocellular carcinoma. Eur Radiol 2019;29:2890-901. [Crossref] [PubMed]
- Dong Y, Zhou L, Xia W, Zhao XY, Zhang Q, Jian JM, Gao X, Wang WP. Preoperative Prediction of Microvascular Invasion in Hepatocellular Carcinoma: Initial Application of a Radiomic Algorithm Based on Grayscale Ultrasound Images. Front Oncol 2020;10:353. [Crossref] [PubMed]
- Zhong X, Peng J, Xie Y, Shi Y, Long H, Su L, Duan Y, Xie X, Lin M. A nomogram based on multi-modal ultrasound for prediction of microvascular invasion and recurrence of hepatocellular carcinoma. Eur J Radiol 2022;151:110281. [Crossref] [PubMed]
- Yao Z, Dong Y, Wu G, Zhang Q, Yang D, Yu JH, Wang WP. Preoperative diagnosis and prediction of hepatocellular carcinoma: Radiomics analysis based on multi-modal ultrasound images. BMC Cancer 2018;18:1089. [Crossref] [PubMed]
- Li L, Wu C, Huang Y, Chen J, Ye D, Su Z. Radiomics for the Preoperative Evaluation of Microvascular Invasion in Hepatocellular Carcinoma: A Meta-Analysis. Front Oncol 2022;12:831996. [Crossref] [PubMed]
- Huang J, Tian W, Zhang L, Huang Q, Lin S, Ding Y, Liang W, Zheng S. Preoperative Prediction Power of Imaging Methods for Microvascular Invasion in Hepatocellular Carcinoma: A Systemic Review and Meta-Analysis. Front Oncol 2020;10:887. [Crossref] [PubMed]
- Zhong X, Long H, Su L, Zheng R, Wang W, Duan Y, Hu H, Lin M, Xie X. Radiomics models for preoperative prediction of microvascular invasion in hepatocellular carcinoma: a systematic review and meta-analysis. Abdom Radiol (NY) 2022;47:2071-88. [Crossref] [PubMed]