Association between the functional connectivity of ventral tegmental area-prefrontal network and pure apathy in Parkinson’s disease: a cross-sectional study
Introduction
Apathy, characterized by lack of motivation in accomplishing goal-directed behaviors (1,2), frequently occurs in patients with Parkinson’s disease (PD), with the prevalence ranging from 23% to 70% (3). As goal-directed behavior is fundamental to survival and well-being, apathy substantially reduces quality of life (4,5), compounding the overall disability substantially and increasing the burden of caregivers (3,4). Apathy is dissociable from cognitive decline and depression, although they do commonly co-occur (4,6-9), and is distinct from depression, as it is not associated with feelings of sadness, worthlessness, hopelessness, guilt, or self-blame (10). Recent studies have focused on pure apathy (i.e., without depression or dementia) to delineate the specific mechanism of apathy. Apathy can also negate efficacious PD treatments, including advanced motor symptom treatments (11,12) and itself lacks established effective treatment (13). There is therefore an urgent need to better understand the mechanism of this behavioral disruption.
According to the framework of normal goal-directed behavior, motivated behavior is characterized by active efforts exerted toward acquiring positive reinforcers (rewards) (14,15), which involves three fundamental processes (13,14). First, a value system computes the subjective value of events; second, a motor system produces behaviors to obtain high-value rewards; and third, a mediating system, based on value information, directs the motor system toward motivationally relevant goals. These processes are ongoing during the initiation and persistence of behaviors and involve a broad network of mesocorticolimbic areas. Among these, dopamine-releasing neurons of the ventral tegmental area (VTA) have been widely accepted to be critically involved in goal-directed behavior (16).
Both animal and human studies indicate that apathy, characterized by reduced goal-directed behaviors, has been closely related to the VTA in PD (17-19). In PD primate models treated with 1-methyl-4-phenyl-1,2,3,6-tetrahydropyridine (MPTP), a toxin that impairs dopaminergic neurons, dopaminergic cell loss in the VTA was found to be significantly predictive of apathy symptoms (17,18). Among patients with PD, those who are apathetic exhibit bilateral dopaminergic disruption within the VTA (18). Moreover, patients with PD and disrupted glucose metabolism in the VTA are predisposed to apathy after undergoing deep brain stimulation operation of the subthalamic nucleus (20). The VTA is not isolated and interacts with other brain regions of the network to play an essential role in goal-directed behaviors. The VTA projects not only to the ventromedial prefrontal cortex (vmPFC), allowing the subjective valuation of events, but also to the limbic cortex and ventral striatum to enable the mediation of value information (15). Moreover, the VTA also participates in reciprocal connectivity with the medial superior frontal gyrus (SFGmed) (21) and has been implicated in reward processing (22). More specifically, VTA activity and dopamine release have been reported to modulate the activity of the SFGmed (23).
However, it remains unknown whether there is variability in the functional relationships of the VTA with other brain regions of the network in patients with PD and pure apathy (PD-PA). Resting-state functional magnetic resonance imaging (rs-fMRI) can probe the neural activity and connections in the human brain. Thought to indicate underlying neural activity, rs-fMRI captures blood oxygen level-dependent (BOLD) signals of brain regions. The correlations of these signals or their temporal patterns can be used to determine functional connectivity (FC) (24). According to Braak staging, degeneration of mesencephalic dopaminergic neurons leads to dopamine depletion in the mesocorticolimbic network (25), and the spread of α-synuclein to dopamine projection brain regions further impairs the functionality of the network (26,27). Accordingly, Alfano et al. found impaired FC within the planum polare and somatosensory network (28). The above-described research highlights the need to ascertain the pure apathy-related circuitry in patients with PD, especially the potential alterations of the VTA-FC network. This study thus aimed to examine the differences in VTA FC patterns among patients with PD-PA, patients with PD but not pure apathy (PD-NPA), and healthy controls (HCs) using rs-fMRI. In addition, as FC might be linked to changes in white matter structural connectivity, we further applied diffusion tensor imaging (DTI) to detect potential white matter tract impairment. We present this article in accordance with the STROBE reporting checklist (available at https://qims.amegroups.com/article/view/10.21037/qims-23-1673/rc).
Methods
Participants
The participants of this cross-sectional retrospective study, including 29 patients with PD-PA and 37 patients with PD-NPA, were consecutively recruited between April 2017 and October 2021 from The First Affiliated Hospital of Nanjing Medical University. All patients with PD met the diagnostic criteria for idiopathic PD of the Parkinson’s UK’s Brain Bank (29) and had at least 3 months of stable dopaminergic treatment. Apathy was diagnosed via semistructured interviews based on the criteria published by Robert et al. (30) and via a 14-item self-report apathy scale (AS) (a score greater than 14 indicated apathy) (9). The exclusion criteria were Parkinson-plus syndromes, secondary parkinsonism, major neurological and psychiatric illness, dementia [Mini Mental State Examination (MMSE) score ≤26 (31)], depression [17-item Hamilton Depression Rating Scale (HAMD) score >7 (32)], anxiety [Hamilton Anxiety Rating Scale (HAMA) >7 (33)], impulse control disorders, the use of antidepressants or other antipsychotic drugs, standard contraindication for MRI examination, or abnormalities on brain MRI, such as tumor, infarction, and white matter lesions. Additionally, 28 HCs, without psychological and neurological disorders or imaging abnormalities, were enrolled from the local community and the Health Examination Center of our hospital. All participants were right-handed and matched for age, gender, education, and affective and cognitive performance. MRI scans and clinical examinations were performed during the off state (at least 12-hour withdrawal of drugs for PD) to reduce potential pharmacological effects on neural activity. Ultimately, the imaging data of 64 patients with PD and 26 HCs were finally analyzed, with 4 participants (2 with PD-PA and 2 HCs) being excluded due to abnormal head motions. The flowchart of the study is shown in Figure 1. This study was conducted in accordance with the Declaration of Helsinki (as revised in 2013) and was approved by the ethics committee of The First Affiliated Hospital of Nanjing Medical University (No. 2016-SRFA-094). Written informed consent was obtained from all participants.
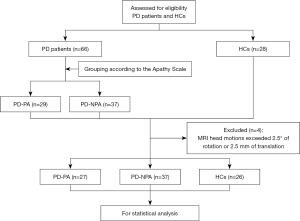
Demographic and clinical assessment
Disease stage and motor symptoms were evaluated with the Hoehn and Yahr (H-Y) stage (34) and Unified Parkinson’s Disease Rating Scale, motor part III (UPDRS-III). Apathy was assessed using AS (range, 0–42; cutoff value 14) (9), a widely used measure of apathy in PD (2). A higher AS, indicating a greater level of apathy, has been used to investigate the presence and severity of apathy in patients with PD (9). The HAMD, HAMA, MMSE, and Frontal Assessment Battery (FAB) (35) were used to assess clinically depressive and anxiety symptoms, global cognitive status, and executive function, respectively. Demographic information including on age, gender, education level, and disease duration was also collected. We also calculated levodopa equivalent daily dose (LEDD) of each patient according to previously described method (36).
MRI data acquisition
MRI scans were performed with a 3.0 T MRI scanner (Siemens Healthineers, Erlangen, Germany) with an eight-channel head coil. Three-dimensional T1-weighted images were first acquired under the following parameters: repetition time (TR) =1,900 ms, echo time (TE) =2.95 ms, flip angle (FA) =9°, slice thickness =1 mm, slices =160, field of view (FOV) =230×230 mm2, and matrix size =256×256. Subsequently, rs-fMRI was obtained with an echo-planar imaging pulse sequence under the following parameters: TR =2,000 ms, TE =21 ms, FA =90°, FOV =256×256 mm2, matrix size =64×64, slices =35, slice thickness =3 mm, no slice gap, and total volumes =240. In addition, DTI images were collected using a spin echo planar imaging sequence under the following parameters: TR =9,800 ms, TE =95 ms, FOV =256×256 mm2, number of excitations =1, matrix =128×128, slice thickness =2 mm, and slice gap =0 mm. Diffusion gradients were applied in 30 noncollinear directions with a b factor of 1,000 s/mm2 after an acquisition without diffusion weighting (b=0 s/mm2) used as the reference.
Preprocessing of rs-fMRI data and VTA-based FC analysis
rs-fMRI data were preprocessed with the Data Processing Assistant for Resting-State fMRI (DPARSF; http://www.restfmri.net/forum/dparsf) (37). The first 10 time-point resting-state images were discarded due to the instability of the initial rs-fMRI signals. The remaining data were corrected for slice timing and then realigned to the middle time-point image using a six-parameter rigid body transformation. Following this, the generated rs-fMRI images were spatially normalized into the Montreal Neurological Institute (MNI) standard anatomic space at a resolution of a 3×3×3 mm3 and smoothed with a Gaussian kernel of 6 mm at full width at half maximum. After removal of the linear trend, the temporal filtering (0.01–0.08 Hz) was conducted to minimize the effects of low- and high-frequency physiological noise. We focused on a low-frequency spectrum (typically around 0.01–0.08 Hz) of BOLD signals due to its neurophysiological properties (38). Moreover, to further reduce the effects of confounding factors, we removed several sources of spurious variance using linear regression. The sources included 24 head motion-related covariates (39) and average signals from the white matter and cerebrospinal fluid. To avoid potential spurious negative correlations, the global signal was not regressed out (40,41). Two patients with PD-PA and two HCs whose head motions exceeded 2.5° of rotation or 2.5 mm of translation were excluded. We also calculated the mean framewise displacement (FD) of each participant.
VTA-based, voxel-wise FC analysis was performed using the DPARSF and SPM12 (http://www.fil.ion.ucl.ac.uk/spm/). The VTA seed region, namely the region of interest (ROI), (with the left and right regions being combined into a whole) was extracted from the probabilistic VTA atlas (Figure 2) based on 7 T MRI data from 27 healthy participants (42). The VTA seed was generated from the probabilistic atlas at a 50% threshold. We conducted a voxel-wise FC analysis by computing temporal cross-correlation between the average time series of the VTA seed region and those of all brain voxels. Fisher Z-transformation was used to improve normality. Analysis of covariance (ANCOVA) was conducted to investigate the brain regions with significant differences in FC among the three groups [voxel-wise P<0.001; cluster-wise family-wise error (FWE)-corrected P (PFWE)<0.05]. P value tests were one-sided. To control for potential confounders, age, gender, education, HAMA score, HAMD score, MMSE score, and mean FD were introduced as covariates. These significant regions were then obtained as an explicit mask. Subsequently, two-sample post hoc t-tests were performed within this mask, with the same covariates as abovementioned, to detect the significant differences between groups (voxel-wise P<0.001; cluster-wise PFWE<0.05).
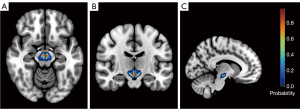
Processing of DTI data and tract-based spatial statistics (TBSS)
DTI data were preprocessed via the Pipeline for Analyzing Brain Diffusion Images (PANDA) toolkit (PANDA; http://www.nitrc.org/projects/panda) based on the Functional Magnetic Resonance Imaging of the Brain (FMRIB) Software Library 5.0 (FSL 5.0; https://www.nitrc.org/projects/fsl). The preprocessing of diffusion images included brain extraction, realignment, eddy current and motion artifact correction, and calculation of fractional anisotropy (FA) and mean diffusivity (MD) maps.
TBSS was performed to analyze the DTI images. This analysis mainly included the following procedures: (I) FA images were realigned to an FMRIB-58 FA map from MNI space using nonlinear registration. (II) The mean FA image was then created and thinned to generate a mean FA skeleton at the 0.2 threshold. (III) FA and MD images of participants were projected onto the skeleton. The voxel-wise statistic analysis of skeletonized FA and MD images was performed using a general linear model. This analysis used threshold-free cluster enhancement (TFCE) for multiple comparison correction (randomized permutation test =5,000 permutations). The nuisance covariates included age, gender, education, MMSE score, HAMD score, and HAMA score. Results were deemed significant at a TFCE-corrected P value of <0.05. P value tests were one-sided.
Statistical analysis
The demographic and clinical data of all participants were analyzed using SPSS 20.0 (IBM Corp., Armonk, NY, USA). Demographic and clinical characteristics were compared with the two-sample t-test, chi-square test, Kruskal-Wallis test, Mann-Whitney test, or one-way analysis of variance (ANOVA), as appropriate. P value tests were two-sided, and Bonferroni correction was used for multiple comparisons. Additionally, we extracted the brain region showing significant differences between the PD-PA and PD-NPA group as the ROI. We employed partial correlation analysis to compute the correlation between the average FC values of the ROI and the AS scores in patients with PD-PA. Age, education level, MMSE score, HAMA score, and HAMD score were introduced as covariates.
Results
Demographic and clinical data
The characteristics of all participants are summarized in Table 1. There were no remarkable differences in age (P=0.76), gender (P=0.93), years of education (P=0.47), MMSE (P=0.29), HAMA (P=0.51), or HAMD (P=0.92) across the three groups. Moreover, no significant differences in PD duration (P=1.00), UPDRS-III (P=0.72), H-Y (P=0.51), or LEDD (P=0.60) between the PD-PA and PD-NPA groups were found. However, the AS scores (P<0.001) were significantly different between the three groups. The PD-PA group exhibited more severe apathy symptoms than did the PD-NPA group (P<0.001). The patients with PD-PA showed a nonsignificant tendency for worse executive function than did the HCs (corrected P value =0.053). No significant differences in mean FD were found among the PD-PA, PD-NPA, and HC groups (PD-PA group: 0.11±0.05; PD-NPA group: 0.12±0.06; HC group: 0.12±0.06; P=0.57) (43).
Table 1
Variable | PD-PA | PD-NPA | HC | P value |
---|---|---|---|---|
N | 27 | 37 | 26 | NA |
Gender (M/F) | 19/8 | 25/12 | 17/9 | 0.93a |
Age (y) | 61.63±10.58 | 63.30±9.40 | 62.73±5.90 | 0.76e |
Education (y) | 11.41±2.95 | 11.14±3.65 | 12.23±3.87 | 0.47b |
Disease duration (y) | 4.81±2.72 | 4.81±3.10 | NA | 1.00c |
H-Y | 1.89±0.68 | 2.00±0.65 | NA | 0.51c |
LEDD (mg/d) | 539.58±226.93 | 503.04±308.34 | NA | 0.60c |
Motor sign | ||||
UPDRS-III score | 25.15±5.96 | 24.22±12.67 | NA | 0.72d |
Nonmotor performance | ||||
AS | 21.63±5.97 | 6.27±3.15 | 4.12±3.19 | <0.001e |
HAMD | 2.41±1.34 | 2.43±1.77 | 2.27±1.54 | 0.92b |
HAMA | 3.33±1.64 | 3.35±1.50 | 2.92±1.55 | 0.51b |
Cognitive performance | ||||
MMSE | 28.19±1.18 | 27.86±4.85 | 29.15±1.12 | 0.29b |
FAB | 15.70±1.71 | 16.24±1.79 | 16.85±1.64 | 0.059b |
FD | 0.11±0.05 | 0.12±0.06 | 0.12±0.06 | 0.57b |
Data are expressed as the mean ± SD. a, Chi-square test; b, one-way analysis of variance; c, two-sample t-test; d, Mann-Whitney test; e, Kruskal-Wallis test. PD-PA, Parkinson’s disease with pure apathy; PD-NPA, Parkinson’s disease without pure apathy; HC, healthy control; M, male; F, female; y, year; NA, not applicable; H-Y, Hoehn and Yahr stage; LEDD, levodopa equivalent daily dose; UPDRS, Unified Parkinson’s Disease Rating Scale; AS, apathy score; HAMD, 17-item Hamilton Depression Rating Scale; HAMA, Hamilton Anxiety Rating Scale; MMSE, Mini Mental State Examination; FAB, Frontal Assessment Battery; FD, framewise displacement; SD, standard deviation.
VTA-based FC data
Compared to the patients with PD-NPA, patients with PD-PA showed reduced VTA FC with the left SFGmed (Figure 3, Table 2), after age, gender, and education, along with mean FD, HAMD, HAMA, and MMSE scores, were controlled for. Relative to HCs, patients with PD-PA demonstrated reduced VTA FC with the left SFGmed, right orbital superior frontal gyrus (SFGorb), and right middle frontal gyrus (MFG) (Figure 3; Table 2). Compared with HCs, patients with PD-NPA exhibited decreased VTA FC with the right MFG (Figure 3, Table 2). Increased FC of the VTA with different brain regions was not found in patients with PD-PA as compared to HCs group or those with PD-NPA.
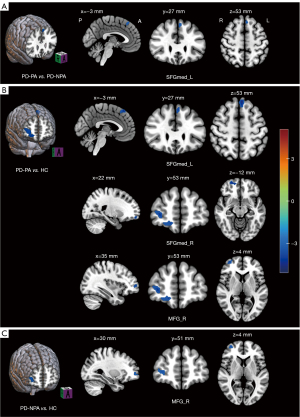
Table 2
Brain region | Side | Cluster size | MNI coordinate | t score | ||
---|---|---|---|---|---|---|
x | y | z | ||||
PD-PA vs. PD-NPA | ||||||
Frontal_Sup_Medial | L | 11 | −3 | 30 | 54 | −3.67 |
PD-PA vs. HC | ||||||
Frontal_Sup_Medial | L | 36 | −6 | 36 | 54 | −4.98 |
Frontal_Mid | R | 64 | 15 | 54 | −12 | −5.08 |
Frontal_Sup_Orb | R | |||||
PD-NPA vs. HC | ||||||
Frontal_Mid | R | 29 | 33 | 54 | 3 | −4.17 |
a, voxel-wise P<0.001, cluster-wise FWE-corrected P<0.05. VTA, ventral tegmental area; FC, functional connectivity; MNI, Montreal Neurological Institute; PD-PA, Parkinson’s disease with pure apathy; PD-NPA, Parkinson’s disease without pure apathy; HC, healthy control; L, left; R, right; FWE, family-wise error; Frontal_Sup_Medial, superior frontal gyrus, medial; Frontal_Mid, middle frontal gyrus; Frontal_Sup_Orb, superior frontal gyrus, orbital part.
TBSS data
We applied TBSS to compare the three groups in terms of the white-matter tract alterations across the whole brain; however, no significant differences between the three groups were found.
Correlation analysis
The brain region (i.e., left SFGmed) that showed significant FC differences between the PD-PA and PD-NPA groups was selected (Figure 4A). Following this, we correlated its FC values with AS scores in patients with PD-PA, aiming to examine whether the observed connectivity alterations were related to apathy symptoms in patients with PD-PA. Correlation analysis revealed a negative relationship between AS scores and mean VTA FC values in the left SFGmed in the PD-PA group (r=−0.600; P=0.003; Figure 4B).
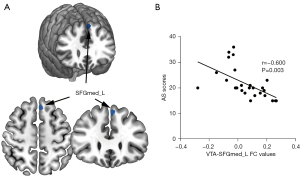
Discussion
Our study provides valuable insights into the neural mechanisms underlying apathy in PD. To the best of our knowledge, this is the first study to examine the relationship between apathy and abnormal VTA FC networks in patients with PD-PDA via rs-fMRI. We found that patients with PD-PA exhibited decreased VTA FC mainly in the prefrontal regions. Specifically, patients with PD-PA showed reduced VTA FC with the left SFGmed as compared to the patients with PD-NPA. Relative to HCs, patients with PD-PA demonstrated reduced VTA FC with the left SFGmed, right SFGorb, and right MFG. Moreover, the VTA FC reductions in left SFGmed were associated with severe apathy symptoms. However, there were no significant differences in fiber tracts between the three groups according to TBSS.
We only found reduced FC in patients with PD-PA, while significant differences were not observed in the DTI analysis. This indicates a disrupted functional organization of the connectome in individuals with PD-PA but a relatively intact structural organization. In fact, this difference between FC and structural connectivity has also been observed in patients with early-stage PD (44) and may partly result from differences in imaging analyses. Moreover, BOLD signals, which were used to measure FC in our study, are thought to be primarily influenced by neurons but also by other nonneuronal elements, such as astrocytes and vascular cells (45). DTI analysis mainly aims to detect potential white-matter tract impairment, and white-matter tract is mainly influenced by neuronal fibers. Therefore, this disparity may also result from the fact BOLD and DTI signals are shaped by different cellular elements and highlights the nuanced relationship between FC and structural brain networks. While rs-fMRI reveals the coordination between brain regions, DTI maps the brain’s physical connections. The absence of correlating structural changes suggests functional reorganization might occur without visible structural alterations.
Our findings are consistent with previous studies. Several studies reported that apathy is significantly more likely to occur if the infarction lesion includes the SFGmed (46) and that the degree of apathy is more severe in patients with bilateral SFGmed lesions than in those with unilateral lesions (47). In line with this, multimodal neuroimaging studies have observed gray-matter atrophy and altered metabolism of the SFGmed in apathetic patients with neurodegenerative diseases (14,48). Taken together, these findings point to the significant role of the SFGmed in the emergence of apathy in patients with PD.
Apathy is characterized by a reduction in goal-directed behavior, which is a complex, multifaceted process (49) that includes series of actions. It involves the integration of cost, reward and information into a value signal in driving behavior toward goals (14,15). There are three dissociable phases involved in goal-directed behavior: the activation of behavior toward a particular goal, the persistence of this behavior over time, and finally, learning from the outcome of this behavior (14). Indeed, the SFGmed, a core node of the mesocorticolimbic network, has been suggested to have roles in motivated behaviors (14) and multifaceted cognitive processes including action selection, inhibitory control, and set shifting. For instance, the SFGmed, together with its closely related region, the anterior cingulate cortex (50), is suggested to be involved in selection of action sets (50) and in guiding decisions about which actions are worth undertaking. These processes are vital to the initiation of goal-directed behavior. More specifically, in an earlier study on the selection of action sets among conflicting choices, the anterior and posterior SFGmed were found to be related to conflict and volition processing, respectively (51). Apart from the action selection role in the start of behavior, inhibitory control is also frequently related to the SFGmed (52-56). After behavioral generation, continued persistence and invigoration are required to attain a goal. Importantly, inhibitory control, a vital part of executive function, exerts a top-down influence in sculpting and sustaining behaviors toward the desired goal in the presence of competing behaviors arising from the changing environment (47,57). The SFGmed is also reported to be involved in set shifting (58,59), a core ingredient of cognitive flexibility. To maintain behaviors toward achieving rewarding goals, the flexible shifting of cognitive processes in response to a changing environment is also important. In line with this, a recent study found that patients with PD-PA exhibit impaired cognitive flexibility and inhibitory control (60). Cumulatively, this evidence suggests that the SFGmed may be a crucial node of the neuronal circuits involved in apathy.
The VTA, one of the largest dopaminergic nuclei, has reciprocal connectivity with the SFGmed (21) and is known to be implicated in reward processing (22). Mastwal et al. reported that VTA activity and dopamine release modulate the SFGmed and have an impact on synaptic plasticity (23). Evidence of the dopaminergic modulation on the SFGmed from experiments in MPTP-treated Parkinson primates has also emerged, with the dopamine concentrations within the SFGmed being significantly decreased (61). It is believed that dopaminergic signaling within the SFGmed supports spatial guidance and temporal structuring (62). Furthermore, another clinical investigation on patients with PD demonstrated that the administration of dopamine agonist drugs can improve frontal cerebral blood flow, particularly in the SFGmed (63). Higher regional blood flow in the SFGmed is associated with improved cognitive processing, including inhibitory control. Moreover, dopamine treatments can improve frontal cortical activity in patients with PD. Collectively, these findings point to the potential modulating role of the VTA on the SFGmed. Therefore, any disruption of the communication between the VTA and the SFGmed may lead to the failure of a multitude of cognitive processes, including action selection, inhibitory control, and set shifting, and subsequently, the reduction of goal-directed behaviors, or apathy. In line with our hypothesis, the correlation analysis of our study showed that the lower FC between the VTA and the SFGmed was correlated with a greater severity of apathy symptoms. This might suggest that with the aggravation of apathy symptoms, functional intercommunication between the VTA and SFGmed worsens.
Clinically, the asymmetrical dopaminergic neurodegeneration and consequent lateralization of motor symptoms are cardinal features in the emergence and progression of PD (64). Moreover, a growing amount of evidence indicates that nonmotor symptoms are also related to lateralized neuropathophysiological changes, such as olfaction, fatigue, cognitive function, impulse control disorder, and apathy (64-67). In our study, we found the reduction in SFGmed connectivity only in the left hemisphere. Considering that interhemispheric coordination is essential for physiological functions, we speculate that the laterality of the observed reduction in SFGmed connectivity may be related to the mechanism of apathy in PD. In line with our assumption, the infarction of the genu of corpus callosum, which connects interhemispheric prefrontal cortex, can lead to sudden emergence apathy (68). Although a causal relationship between human brain laterality and handedness has not been established (69), human brain function shows laterality. In most right-handed individuals, the left hemisphere of the brain is typically dominant in language processing (70), including speech production and comprehension. Additionally, the left hemisphere is often associated with analytical thinking, sequential processing, logical reasoning, verbal memory, and fine motor control (71-73), all of which are vital goal-directed behaviors. In line with this, Zhang et al. found that patients with PD-PA demonstrated brain laterality in the mesocorticolimbic and nigrostriatal systems (65). Thus, the findings in our study might have been influenced by the predominance of right-handed participants in the sample, and the possibility that hemispheric dominance is involved in apathy cannot be completely ruled out. Future research on PD-PA in left-handed individuals will provide further evidence for this hypothesis.
In our study, when the two patient groups were respectively compared with HCs, a difference in FC with the SFGorb was only observed in patients with PD-PA but not in patients with PD-NPA. Therefore, we suggest that reduced FC between VTA and the SFGorb may also have a role in apathy in patients with PD. The SFGorb is mainly located in the vmPFC. The VTA is to project to the vmPFC (21), which allows for the subjective valuation of events (22). Reduced FC between the VTA and SFGorb may underlie the dysfunction of subjective valuation of a given event and poor reward incentivization in patients with PD-PA.
Some limitations to our study to be mentioned. First, the sample size was relatively small, which might have limited the detection of minor white-matter changes. Second, as this was a preliminary study, there were certain biological and technical limitations. Future studies with 7 T MRI could provide higher resolution on the midbrain structures and better help explore the mesocorticolimbic networks. Third, we employed a cross-sectional design, and a longitudinal study is urgently needed to clarify the dynamic alterations of the VTA FC network correlated with the progression of disease in patients with PD-PA. Fourth, we assessed cognitive status using the MMSE and FAB scales. Future research with a more comprehensive battery of cognitive assessments may aid in the detection of specific cognitive subdomain impairment in those with PD-PA. Fifth, we did not include a group of participants with apathy without any neurological disease. In a future study, we will recruit a group of apathy participants without any neurological disease and use FC analysis to ascertain whether there are other brain areas involved in apathy. Sixth, there is no consensus regarding what steps should be included in a pipeline, or in which order they should be performed in fMRI analysis, and modular preprocessing pipelines can reintroduce artifacts into fMRI data. Therefore, the preliminary findings of present study should be interpreted with caution. Finally, resting-state networks are broadband, and our study might have inadvertently removed group-level differences.
Conclusions
We found impaired FC within the mesocortic network in patients with PD-PA. Our findings may provide valuable insights into potential neuromodulation therapies for alleviating the apathy symptoms in patients with PD.
Acknowledgments
We are grateful to all those who participated in this study for their cooperation and patience.
Funding: This work was sponsored by
Footnote
Reporting Checklist: The authors have completed the STROBE reporting checklist. Available at https://qims.amegroups.com/article/view/10.21037/qims-23-1673/rc
Conflicts of Interest: All authors have completed the ICMJE uniform disclosure form (available at https://qims.amegroups.com/article/view/10.21037/qims-23-1673/coif). The authors have no conflicts of interest to declare.
Ethical Statement: The authors are accountable for all aspects of the work in ensuring that questions related to the accuracy or integrity of any part of the work are appropriately investigated and resolved. This study was conducted in accordance with the Declaration of Helsinki (as revised in 2013) and was approved by the Ethics Committee of The First Affiliated Hospital of Nanjing Medical University (No. 2016-SRFA-094). Written informed consent was obtained from all participants.
Open Access Statement: This is an Open Access article distributed in accordance with the Creative Commons Attribution-NonCommercial-NoDerivs 4.0 International License (CC BY-NC-ND 4.0), which permits the non-commercial replication and distribution of the article with the strict proviso that no changes or edits are made and the original work is properly cited (including links to both the formal publication through the relevant DOI and the license). See: https://creativecommons.org/licenses/by-nc-nd/4.0/.
References
- Levy R, Dubois B. Apathy and the functional anatomy of the prefrontal cortex-basal ganglia circuits. Cereb Cortex 2006;16:916-28. [Crossref] [PubMed]
- Leentjens AF, Dujardin K, Marsh L, Martinez-Martin P, Richard IH, Starkstein SE, Weintraub D, Sampaio C, Poewe W, Rascol O, Stebbins GT, Goetz CG. Apathy and anhedonia rating scales in Parkinson's disease: critique and recommendations. Mov Disord 2008;23:2004-14. [Crossref] [PubMed]
- den Brok MG, van Dalen JW, van Gool WA, Moll van Charante EP, de Bie RM, Richard E. Apathy in Parkinson's disease: A systematic review and meta-analysis. Mov Disord 2015;30:759-69. [Crossref] [PubMed]
- Pagonabarraga J, Kulisevsky J, Strafella AP, Krack P. Apathy in Parkinson's disease: clinical features, neural substrates, diagnosis, and treatment. Lancet Neurol 2015;14:518-31. [Crossref] [PubMed]
- Martinez-Martin P, Rodriguez-Blazquez C, Kurtis MM, Chaudhuri KRNMSS Validation Group. The impact of non-motor symptoms on health-related quality of life of patients with Parkinson's disease. Mov Disord 2011;26:399-406. [Crossref] [PubMed]
- Dujardin K, Sockeel P, Delliaux M, Destée A, Defebvre L. Apathy may herald cognitive decline and dementia in Parkinson's disease. Mov Disord 2009;24:2391-7. [Crossref] [PubMed]
- Pluck GC, Brown RG. Apathy in Parkinson's disease. J Neurol Neurosurg Psychiatry 2002;73:636-42. [Crossref] [PubMed]
- Ineichen C, Baumann-Vogel H. Deconstructing Apathy in Parkinson's Disease: Challenges in Isolating Core Components of Apathy From Depression, Anxiety, and Fatigue. Front Neurol 2021;12:720921. [Crossref] [PubMed]
- Starkstein SE, Mayberg HS, Preziosi TJ, Andrezejewski P, Leiguarda R, Robinson RG. Reliability, validity, and clinical correlates of apathy in Parkinson's disease. J Neuropsychiatry Clin Neurosci 1992;4:134-9. [Crossref] [PubMed]
- Béreau M, Van Waes V, Servant M, Magnin E, Tatu L, Anheim M. Apathy in Parkinson's Disease: Clinical Patterns and Neurobiological Basis. Cells 2023;12:1599. [Crossref] [PubMed]
- Aarsland D, Marsh L, Schrag A. Neuropsychiatric symptoms in Parkinson's disease. Mov Disord 2009;24:2175-86. [Crossref] [PubMed]
- Martinez-Fernandez R, Pelissier P, Quesada JL, Klinger H, Lhommée E, Schmitt E, Fraix V, Chabardes S, Mertens P, Castrioto A, Kistner A, Broussolle E, Pollak P, Thobois S, Krack P. Postoperative apathy can neutralise benefits in quality of life after subthalamic stimulation for Parkinson's disease. J Neurol Neurosurg Psychiatry 2016;87:311-8. [Crossref] [PubMed]
- Mele B, Van S, Holroyd-Leduc J, Ismail Z, Pringsheim T, Goodarzi Z. Diagnosis, treatment and management of apathy in Parkinson's disease: a scoping review. BMJ Open 2020;10:e037632. [Crossref] [PubMed]
- Morris LA, O'Callaghan C, Le Heron C. Disordered Decision Making: A Cognitive Framework for Apathy and Impulsivity in Huntington's Disease. Mov Disord 2022;37:1149-63. [Crossref] [PubMed]
- Le Heron C, Holroyd CB, Salamone J, Husain M. Brain mechanisms underlying apathy. J Neurol Neurosurg Psychiatry 2019;90:302-12. [Crossref] [PubMed]
- Morales M, Margolis EB. Ventral tegmental area: cellular heterogeneity, connectivity and behaviour. Nat Rev Neurosci 2017;18:73-85. [Crossref] [PubMed]
- Brown CA, Campbell MC, Karimi M, Tabbal SD, Loftin SK, Tian LL, Moerlein SM, Perlmutter JS. Dopamine pathway loss in nucleus accumbens and ventral tegmental area predicts apathetic behavior in MPTP-lesioned monkeys. Exp Neurol 2012;236:190-7. [Crossref] [PubMed]
- Tian L, Xia Y, Flores HP, Campbell MC, Moerlein SM, Perlmutter JS. Neuroimaging Analysis of the Dopamine Basis for Apathetic Behaviors in an MPTP-Lesioned Primate Model. PLoS One 2015;10:e0132064. [Crossref] [PubMed]
- Maillet A, Krack P, Lhommée E, Météreau E, Klinger H, Favre E, Le Bars D, Schmitt E, Bichon A, Pelissier P, Fraix V, Castrioto A, Sgambato-Faure V, Broussolle E, Tremblay L, Thobois S. The prominent role of serotonergic degeneration in apathy, anxiety and depression in de novo Parkinson's disease. Brain 2016;139:2486-502. [Crossref] [PubMed]
- Gesquière-Dando A, Guedj E, Loundou A, Carron R, Witjas T, Fluchère F, Delfini M, Mundler L, Regis J, Azulay JP, Eusebio A. A preoperative metabolic marker of parkinsonian apathy following subthalamic nucleus stimulation. Mov Disord 2015;30:1767-76. [Crossref] [PubMed]
- Ott T, Nieder A. Dopamine and Cognitive Control in Prefrontal Cortex. Trends Cogn Sci 2019;23:213-34. [Crossref] [PubMed]
- Alberico SL, Cassell MD, Narayanan NS. The Vulnerable Ventral Tegmental Area in Parkinson's Disease. Basal Ganglia 2015;5:51-5. [Crossref] [PubMed]
- Mastwal S, Ye Y, Ren M, Jimenez DV, Martinowich K, Gerfen CR, Wang KH. Phasic dopamine neuron activity elicits unique mesofrontal plasticity in adolescence. J Neurosci 2014;34:9484-96. [Crossref] [PubMed]
- Fox MD, Raichle ME. Spontaneous fluctuations in brain activity observed with functional magnetic resonance imaging. Nat Rev Neurosci 2007;8:700-11. [Crossref] [PubMed]
- Braak H, Del Tredici K, Rüb U, de Vos RA, Jansen Steur EN, Braak E. Staging of brain pathology related to sporadic Parkinson's disease. Neurobiol Aging 2003;24:197-211. [Crossref] [PubMed]
- Goetz CG, Emre M, Dubois B. Parkinson's disease dementia: definitions, guidelines, and research perspectives in diagnosis. Ann Neurol 2008;64:S81-92. [Crossref] [PubMed]
- Luk KC, Kehm V, Carroll J, Zhang B, O'Brien P, Trojanowski JQ, Lee VM. Pathological α-synuclein transmission initiates Parkinson-like neurodegeneration in nontransgenic mice. Science 2012;338:949-53. [Crossref] [PubMed]
- Alfano V, Longarzo M, Mele G, Esposito M, Aiello M, Salvatore M, Grossi D, Cavaliere C. Identifying a Common Functional Framework for Apathy Large-Scale Brain Network. J Pers Med 2021;11:679. [Crossref] [PubMed]
- Hughes AJ, Daniel SE, Kilford L, Lees AJ. Accuracy of clinical diagnosis of idiopathic Parkinson's disease: a clinico-pathological study of 100 cases. J Neurol Neurosurg Psychiatry 1992;55:181-4. [Crossref] [PubMed]
- Robert P, Onyike CU, Leentjens AF, Dujardin K, Aalten P, Starkstein S, Verhey FR, Yessavage J, Clement JP, Drapier D, Bayle F, Benoit M, Boyer P, Lorca PM, Thibaut F, Gauthier S, Grossberg G, Vellas B, Byrne J. Proposed diagnostic criteria for apathy in Alzheimer's disease and other neuropsychiatric disorders. Eur Psychiatry 2009;24:98-104. [Crossref] [PubMed]
- Hoops S, Nazem S, Siderowf AD, Duda JE, Xie SX, Stern MB, Weintraub D. Validity of the MoCA and MMSE in the detection of MCI and dementia in Parkinson disease. Neurology 2009;73:1738-45. [Crossref] [PubMed]
- Hamilton M. A rating scale for depression. J Neurol Neurosurg Psychiatry 1960;23:56-62. [Crossref] [PubMed]
- Hamilton M. The assessment of anxiety states by rating. Br J Med Psychol 1959;32:50-5. [Crossref] [PubMed]
- Hoehn MM, Yahr MD. Parkinsonism: onset, progression and mortality. Neurology 1967;17:427-42. [Crossref] [PubMed]
- Dubois B, Slachevsky A, Litvan I, Pillon B. The FAB: a Frontal Assessment Battery at bedside. Neurology 2000;55:1621-6. [Crossref] [PubMed]
- Tomlinson CL, Stowe R, Patel S, Rick C, Gray R, Clarke CE. Systematic review of levodopa dose equivalency reporting in Parkinson's disease. Mov Disord 2010;25:2649-53. [Crossref] [PubMed]
- Chao-Gan Y, Yu-Feng Z. DPARSF: A MATLAB Toolbox for "Pipeline" Data Analysis of Resting-State fMRI. Front Syst Neurosci 2010;4:13. [PubMed]
- Raichle ME, MacLeod AM, Snyder AZ, Powers WJ, Gusnard DA, Shulman GL. A default mode of brain function. Proc Natl Acad Sci U S A 2001;98:676-82. [Crossref] [PubMed]
- Friston KJ, Williams S, Howard R, Frackowiak RS, Turner R. Movement-related effects in fMRI time-series. Magn Reson Med 1996;35:346-55. [Crossref] [PubMed]
- Murphy K, Birn RM, Handwerker DA, Jones TB, Bandettini PA. The impact of global signal regression on resting state correlations: are anti-correlated networks introduced? Neuroimage 2009;44:893-905. [Crossref] [PubMed]
- Chai XJ, Castañón AN, Ongür D, Whitfield-Gabrieli S. Anticorrelations in resting state networks without global signal regression. Neuroimage 2012;59:1420-8. [Crossref] [PubMed]
- Trutti AC, Fontanesi L, Mulder MJ, Bazin PL, Hommel B, Forstmann BU. A probabilistic atlas of the human ventral tegmental area (VTA) based on 7 Tesla MRI data. Brain Struct Funct 2021;226:1155-67. [Crossref] [PubMed]
- Power JD, Barnes KA, Snyder AZ, Schlaggar BL, Petersen SE. Spurious but systematic correlations in functional connectivity MRI networks arise from subject motion. Neuroimage 2012;59:2142-54. [Crossref] [PubMed]
- Tinaz S, Lauro PM, Ghosh P, Lungu C, Horovitz SG. Changes in functional organization and white matter integrity in the connectome in Parkinson's disease. Neuroimage Clin 2016;13:395-404. [Crossref] [PubMed]
- Howarth C, Mishra A, Hall CN. More than just summed neuronal activity: how multiple cell types shape the BOLD response. Philos Trans R Soc Lond B Biol Sci 2021;376:20190630. [Crossref] [PubMed]
- Kang SY, Kim JS. Anterior cerebral artery infarction: stroke mechanism and clinical-imaging study in 100 patients. Neurology 2008;70:2386-93. [Crossref] [PubMed]
- Jones DT, Graff-Radford J. Executive Dysfunction and the Prefrontal Cortex. Continuum (Minneap Minn) 2021;27:1586-601. [Crossref] [PubMed]
- Rosen HJ, Allison SC, Schauer GF, Gorno-Tempini ML, Weiner MW, Miller BL. Neuroanatomical correlates of behavioural disorders in dementia. Brain 2005;128:2612-25. [Crossref] [PubMed]
- Salamone JD, Correa M, Ferrigno S, Yang JH, Rotolo RA, Presby RE. The Psychopharmacology of Effort-Related Decision Making: Dopamine, Adenosine, and Insights into the Neurochemistry of Motivation. Pharmacol Rev 2018;70:747-62. [Crossref] [PubMed]
- Rushworth MF, Walton ME, Kennerley SW, Bannerman DM. Action sets and decisions in the medial frontal cortex. Trends Cogn Sci 2004;8:410-7. [Crossref] [PubMed]
- Nachev P, Rees G, Parton A, Kennard C, Husain M. Volition and conflict in human medial frontal cortex. Curr Biol 2005;15:122-8. [Crossref] [PubMed]
- Li CS, Huang C, Constable RT, Sinha R. Imaging response inhibition in a stop-signal task: neural correlates independent of signal monitoring and post-response processing. J Neurosci 2006;26:186-92. [Crossref] [PubMed]
- Duann JR, Ide JS, Luo X, Li CS. Functional connectivity delineates distinct roles of the inferior frontal cortex and presupplementary motor area in stop signal inhibition. J Neurosci 2009;29:10171-9. [Crossref] [PubMed]
- Dambacher F, Sack AT, Lobbestael J, Arntz A, Brugmann S, Schuhmann T. The role of right prefrontal and medial cortex in response inhibition: interfering with action restraint and action cancellation using transcranial magnetic brain stimulation. J Cogn Neurosci 2014;26:1775-84. [Crossref] [PubMed]
- Chmielewski WX, Mückschel M, Dippel G, Beste C. Concurrent information affects response inhibition processes via the modulation of theta oscillations in cognitive control networks. Brain Struct Funct 2016;221:3949-61. [Crossref] [PubMed]
- Picton TW, Stuss DT, Alexander MP, Shallice T, Binns MA, Gillingham S. Effects of focal frontal lesions on response inhibition. Cereb Cortex 2007;17:826-38. [Crossref] [PubMed]
- Diamond A. Executive functions. Annu Rev Psychol 2013;64:135-68. [Crossref] [PubMed]
- Klaasen NG, Kos C, Aleman A, Opmeer EM. Apathy is related to reduced activation in cognitive control regions during set-shifting. Hum Brain Mapp 2017;38:2722-33. [Crossref] [PubMed]
- Nagahama Y, Okada T, Katsumi Y, Hayashi T, Yamauchi H, Sawamoto N, Toma K, Nakamura K, Hanakawa T, Konishi J, Fukuyama H, Shibasaki H. Transient neural activity in the medial superior frontal gyrus and precuneus time locked with attention shift between object features. Neuroimage 1999;10:193-9. [Crossref] [PubMed]
- Santangelo G, D'Iorio A, Maggi G, Cuoco S, Pellecchia MT, Amboni M, Barone P, Vitale C. Cognitive correlates of "pure apathy" in Parkinson's disease. Parkinsonism Relat Disord 2018;53:101-4. [Crossref] [PubMed]
- Elsworth JD, Deutch AY, Redmond DE Jr, Sladek JR Jr, Roth RH. MPTP reduces dopamine and norepinephrine concentrations in the supplementary motor area and cingulate cortex of the primate. Neurosci Lett 1990;114:316-22. [Crossref] [PubMed]
- Hosp JA, Coenen VA, Rijntjes M, Egger K, Urbach H, Weiller C, Reisert M. Ventral tegmental area connections to motor and sensory cortical fields in humans. Brain Struct Funct 2019;224:2839-55. [Crossref] [PubMed]
- Trujillo P, van Wouwe NC, Lin YC, Stark AJ, Petersen KJ, Kang H, Zald DH, Donahue MJ, Claassen DO. Dopamine effects on frontal cortical blood flow and motor inhibition in Parkinson's disease. Cortex 2019;115:99-111. [Crossref] [PubMed]
- Riederer P, Jellinger KA, Kolber P, Hipp G, Sian-Hülsmann J, Krüger R. Lateralisation in Parkinson disease. Cell Tissue Res 2018;373:297-312. [Crossref] [PubMed]
- Zhang H, Shan AD, Gan CT, Zhang L, Wang LN, Sun HM, Yuan YS, Zhang KZ. Impaired interhemispheric synchrony in Parkinson's disease patients with apathy. J Affect Disord 2022;318:283-90. [Crossref] [PubMed]
- Yuan YS, Ji M, Gan CT, Sun HM, Wang LN, Zhang KZ. Impaired Interhemispheric Synchrony in Parkinson's Disease with Fatigue. J Pers Med 2022;12:884. [Crossref] [PubMed]
- Gan C, Wang L, Ji M, Ma K, Sun H, Zhang K, Yuan Y. Abnormal interhemispheric resting state functional connectivity in Parkinson's disease patients with impulse control disorders. NPJ Parkinsons Dis 2021;7:60. [Crossref] [PubMed]
- Saito Y, Matsumura K, Shimizu T. Anterograde amnesia associated with infarction of the anterior fornix and genu of the corpus callosum. J Stroke Cerebrovasc Dis 2006;15:176-7. [Crossref] [PubMed]
- Budisavljevic S, Castiello U, Begliomini C. Handedness and White Matter Networks. Neuroscientist 2021;27:88-103. [Crossref] [PubMed]
- Knecht S, Dräger B, Deppe M, Bobe L, Lohmann H, Flöel A, Ringelstein EB, Henningsen H. Handedness and hemispheric language dominance in healthy humans. Brain 2000;123:2512-8. [Crossref] [PubMed]
- Corballis MC. Left brain, right brain: facts and fantasies. PLoS Biol 2014;12:e1001767. [Crossref] [PubMed]
- Henson RN, Burgess N, Frith CD. Recoding, storage, rehearsal and grouping in verbal short-term memory: an fMRI study. Neuropsychologia 2000;38:426-40. [Crossref] [PubMed]
- Prabhakaran V, Narayanan K, Zhao Z, Gabrieli JD. Integration of diverse information in working memory within the frontal lobe. Nat Neurosci 2000;3:85-90. [Crossref] [PubMed]