Right ventricular longitudinal shortening and right atrial volumes are not associated in healthy adults—detailed analysis from the three-dimensional speckle-tracking echocardiographic MAGYAR-Healthy Study
Introduction
In the past decades, echocardiography has undergone enormous technical development. It is now possible to perform a detailed volumetric analysis of various heart chambers including the right atrium (RA), this latter measurement takes into account the heart cycle as well. Three-dimensional (3D) speckle-tracking echocardiography (3DSTE), which measures volumetric data using virtual 3D models, is ideally suited for this task (1-4). In recent studies, capability of 3DSTE was demonstrated in accurate volumetric assessment of RA with defined normal reference values (5-7). Moreover, the exact relationship of RA volumes, volume-based functional properties and strains was examined in healthy circumstances (7). The RA forms an organic unit with the right ventricle (RV), and the tricuspid annular (TA) (8,9). The TA has a special 3D hyperbolic paraboloid shape with a dynamic 3D motion including longitudinal and sphincter-like movements (10). In a recent study, close relationship between RA volumes and TA dimensions and derived functional sphincter-like features could be detected (5). However, its relation to TA longitudinal motion is not clear, which can even be considered to be a characteristic of RV longitudinal shortening. M-mode echocardiography-derived TA plane systolic excursion (TAPSE) is a long-used parameter, which is suitable for characterising RV longitudinal function with a well-documented strong prognostic impact (8,11-13). Therefore, the aim of this cohort study was to perform a detailed analysis of the relationship of 3DSTE-derived RA volumes and RV longitudinal shortening in healthy individuals. These parameters were examined in the case of average values and larger/smaller values as well. We present this article in accordance with the STROBE reporting checklist (available at https://qims.amegroups.com/article/view/10.21037/qims-24-223/rc).
Methods
Subject population
The present study comprised 93 healthy adults (mean age: 27.7±6.3 years, 46 men) being in sinus rhythm, who participated in a complete medical investigation including physical examination, laboratory test, standard 12-lead electrocardiography and two-dimensional (2D) Doppler echocardiography with results within the normal range. 3DSTE data acquisition was obtained immediately after a routine echocardiographic examination and datasets were analysed at a later date. No healthy participant took any medication or drug regularly, none of them were obese or were smoking regularly, and none of them had any known pathological state or disease. The present retrospective cohort study is part of the ‘Motion Analysis of the heart and Great vessels bY three-dimensionAl speckle-tRacking echocardiography in Healthy subjects’ (MAGYAR-Healthy) Study, which was organized in part to investigate physiological relationships in healthy adults (‘Magyar’ means ‘Hungarian’ in Hungarian language). All individuals who participated in the study signed informed consent. The study was in line with the Helsinki Declaration (as revised in 2013). The study was approved by the Institutional and Regional Human Biomedical Research Committee of University of Szeged, Hungary (registration number: 71/2011, latest approval for prolongation: February 20, 2023).
2D Doppler echocardiography
During 2D Doppler echocardiography, professional guidelines were followed. Measurements were made using a Toshiba ArtidaTM echocardiography machine (Toshiba Medical Systems, Tokyo, Japan), which was attached to a PST-30BT (1–5 MHz) phased-array transducer. All healthy individuals were in the left lateral decubitus position, and after placing the transducer on their chest, examinations were made from the apical and parasternal views. The left atrium (LA) and left ventricle (LV) were quantified according to guidelines, and LV ejection fraction (EF) values were determined using the Simpson’s method (8,14). Continuous Doppler echocardiography was used to exclude valvular stenosis or regurgitation on any valves. Pulsed Doppler echocardiography was used to determine transmitral E and A flow velocities and E/A ratio featuring LV diastolic function. To represent longitudinal shortening of the RV, TAPSE was measured in apical long-axis view by measuring TA lateral edge movement toward the apex in systole in longitudinal direction (Figure 1) (8,14).
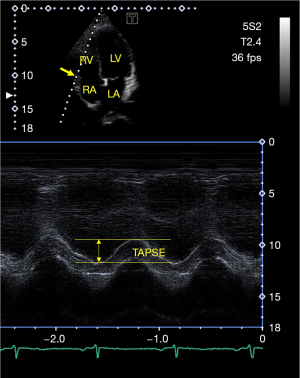
3DSTE-derived data acquisition
In accordance with the established practice, the 3DSTE examination took place in two phases (1-7). For the first time, digital 3D datasets were acquired by the same observer (Á.K.) using the same Toshiba ArtidaTM cardiac ultrasound with a PST-25SX matrix transducer attached. An important condition during data acquisition was that the individual had to be in sinus rhythm, and the volunteer was asked to lie on his left side. During data acquisition, 6 wedge-shaped datasets (subvolumes) were collected within a single breath-hold within 6 heart cycles from the apical window. The automatically merged subvolumes helped to create a complete dataset called full volume. At a second stage, datasets were analysed offline with a 3D Wall Motion Tracking software version 2.7 (Ultra Extend, Toshiba Medical Systems, Tokyo, Japan).
RA volumetric evaluation by 3DSTE
During the analysis of the RA, virtual 3D models were created in the images focused on RA using the methodology already described. Shortly, datasets were presented in apical two- (AP2CH) and four-chamber (AP4CH) views and in short-axis views at basal, midatrial and superior RA levels at end-diastole. After defining the septal and lateral RA-TA edges and the RA apex in AP2CH and AP4CH views at end-diastole, the RA endocardial surface was reconstructed and a sequential analysis was performed. Respecting the cardiac cycle, the following volumes of the RA were measured (Figure 2) (5-7):
- Maximum RA volume, measured at end-systole, just before tricuspid valve opening (Vmax).
- RA volume before atrial contraction, measured at early-diastole at the time of the P wave on the electrocardiogram (VpreA).
- Minimum RA volume measured at end-diastole, just before tricuspid valve closure (Vmin).
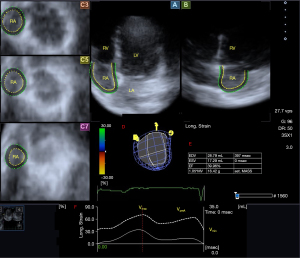
Using RA volumes several RA stroke volumes and emptying fractions were calculated respecting the cardiac cycle as presented in Table 1.
Table 1
Parameters | Systolic reservoir RA function | Early diastolic conduit RA function | Late diastolic booster pump RA function |
---|---|---|---|
RA stroke volumes | TASV = Vmax − Vmin | PASV = Vmax − VpreA | AASV = VpreA − Vmin |
RA emptying fractions | TAEF = TASV/Vmax ×100 | PAEF = PASV/Vmax × 100 | AAEF = AASV/VpreA × 100 |
RA, right atrial; TASV, total atrial stroke volume; Vmax, end-systolic maximum RA volume; Vmin, end-diastolic minimum RA volume; PASV, passive atrial stroke volume; VpreA, early diastolic preatrial contraction RA volume; AASV, active atrial stroke volume; TAEF, total atrial emptying fraction; PAEF, passive atrial emptying fraction; AAEF, active atrial emptying fraction.
Statistical analysis
Depending on the distribution of parameters, for continuous variables, mean ± standard deviation (SD) format and for categorical variables, number/percentage format were used. During the statistical analyses, P less than 0.05 was considered to be significant. Independent sample t-test and analysis of variance (ANOVA) tests were applied for group comparisons. Pearson’s coefficients were determined for featuring correlations between variables. The Bland-Altman method was used for interobserver and intraobserver agreements and intraclass correlation coefficients (ICCs) were also calculated. SPSS software (version 22, SPSS Inc., Chicago, IL, USA) was applied for statistical analyses.
Results
Clinical and two-dimensional Doppler echocardiographic data
All routine two-dimensional echocardiographic data are demonstrated in Table 2. Significant valvular disease were not present in any of subjects.
Table 2
Data | Measurements |
---|---|
Two-dimensional echocardiographic data | |
Left atrial diameter (mm) | 36.9±3.4 |
LV end-diastolic diameter (mm) | 48.1±3.6 |
LV end-systolic diameter (mm) | 32.3±3.2 |
LV end-diastolic volume (mL) | 105.8±24.5 |
LV end-systolic volume (mL) | 38.2±10.1 |
Interventricular septum (mm) | 9.0±1.2 |
LV posterior wall (mm) | 9.3±1.5 |
LV ejection fraction (%) | 64.6±4.1 |
Early diastolic mitral inflow velocity – E (cm/s) | 83.5±15.2 |
Late diastolic mitral inflow velocity – A (cm/s) | 56.9±11.1 |
Tricuspid annular plane systolic excursion (mm) | 23.8±2.9 |
Three-dimensional speckle-tracking echocardiographic data | |
End-systolic maximum RA volume (mL) | 49.5±16.3 |
Early diastolic pre-atrial contraction RA volume (mL) | 35.0±11.2 |
Late diastolic minimum RA volume (mL) | 27.2±9.9 |
Total RA stroke volume (mL) | 22.3±10.2 |
Passive RA stroke volume (mL) | 14.4±8.3 |
Active RA stroke volume (mL) | 7.8±4.9 |
Total RA emptying fraction (%) | 44.3±11.6 |
Passive RA emptying fraction (%) | 28.0±10.6 |
Active RA emptying fraction (%) | 22.5±12.1 |
Data are presented as mean ± standard deviation. LV, left ventricular; RA, right atrial.
Classification of subjects
The study population was divided into several groups of healthy individuals considering their mean ± SD of 3DSTE-derived RA-Vmax, RA-VpreA and RA-Vmin and TAPSE as assessed by M-mode echocardiography, which proved to be 49.5±16.3, 35.0±11.2, 27.2±9.9 mL and 23.8±2.9 mm, respectively. According to these values, the subgroups were classified based on the lower (33.2, 23.8, 17.3 mL and 20.9 mm, respectively) and upper (65.8, 46.2, 37.1 mL and 26.7 mm, respectively) values of these parameters.
Degree of TAPSE and RA properties
RA volumes, stroke volumes and emptying fractions were not related to TAPSE (Table 3).
Table 3
Data | TAPSE ≤20.9 mm (n=11) |
20.9 mm < TAPSE <26.7 mm (n=61) |
26.7 mm ≤ TAPSE (n=21) |
---|---|---|---|
RA-Vmax (mL) | 50.4±22.4 | 49.5±15.5 | 49.0±15.8 |
RA-VpreA (mL) | 36.9±16.8 | 34.5±10.4 | 35.6±10.5 |
RA-Vmin (mL) | 28.7±13.6 | 27.2±9.4 | 26.6±9.3 |
TASV (mL) | 21.7±12.6 | 22.3±9.9 | 22.4±10.5 |
PASV (mL) | 13.5±9.1 | 15.0±8.3 | 13.4±8.4 |
AASV (mL) | 8.2±8.4 | 7.3±4.0 | 9.0±5.0 |
TAEF (%) | 42.3±14.8 | 44.6±11.0 | 44.7±11.9 |
PAEF (%) | 26.7±9.3 | 29.1±10.4 | 25.5±11.6 |
AAEF (%) | 20.7±19.3 | 21.8±10.4 | 25.7±12.0 |
TAPSE (mm) | 19.6±0.5 | 23.1±1.6* | 28.1±1.2*† |
Data are presented as mean ± standard deviation. *, P<0.05 vs. TAPSE ≤20.9 mm; †, P<0.05 vs. 20.9 mm< TAPSE <26.7 mm. TAPSE, tricuspid annular plane systolic excursion; RA, right atrial; Vmax, end-systolic maximum RA volume; VpreA, early diastolic preatrial contraction RA volume; Vmin, end-diastolic minimum RA volume; TASV, total atrial stroke volume; PASV, passive atrial stroke volume; AASV, active atrial stroke volume; TAEF, total atrial emptying fraction; PAEF, passive atrial emptying fraction; AAEF, active atrial emptying fraction.
Degree of RA volumes and TAPSE
Higher RA volumes showed no associations with TAPSE (Table 4).
Table 4
Data | RA-Vmax | RA-VpreA | RA-Vmin | ||||||||
---|---|---|---|---|---|---|---|---|---|---|---|
≤33.2 mL (n=15) | >33.2 to <65.8 mL (n=62) | ≥65.8 mL (n=16) | ≤23.8 mL (n=15) | >23.8 to <46.2 mL (n=64) | ≥46.2 mL (n=14) | ≤17.3 mL (n=13) | >17.3 to <37.1 mL (n=62) | ≥37.1 mL (n=18) | |||
RA-Vmax (mL) | 28.4±3.3 | 47.7±8.9* | 76.1±10.0*† | 28.9±3.8 | 49.3±11.1§ | 72.4±15.0§‡ | 32.5±10.2 | 47.5±11.7# | 68.5±16.1#& | ||
RA-VpreA (mL) | 21.6±3.4 | 34.3±6.9* | 50.6±11.4*† | 21.1±3.0 | 34.1±6.0§ | 54.3±9.1§‡ | 21.5±3.6 | 33.2±6.3# | 51.1±10.3#& | ||
RA-Vmin (mL) | 16.6±2.8 | 26.5±7.6* | 39.9±8.3*† | 15.7±2.5 | 26.2±6.2§ | 44.3±4.9§‡ | 14.8±1.7 | 25.2±5.3# | 43.2±4.8#& | ||
TASV (mL) | 11.8±2.8 | 21.2±7.9* | 36.2±8.1*† | 13.2±4.0 | 23.1±9.3§ | 28.0±13.2§ | 17.8±9.5 | 22.3±9.0 | 25.4±13.7 | ||
PASV (mL) | 6.8±3.4 | 13.4±6.1* | 25.5±8.4*† | 7.7±3.7 | 15.3±7.8§ | 18.0±10.7§ | 11.0±8.6 | 14.3±7.4 | 17.4±10.4 | ||
AASV (mL) | 5.1±2.3 | 7.7±4.8* | 10.7±5.7*† | 5.5±2.7 | 7.9±4.8§ | 10.0±6.3§ | 6.7±2.7 | 8.0±4.5 | 8.0±7.1 | ||
TAEF (%) | 41.6±8.0 | 44.2±12.8 | 47.6±8.5* | 45.2±9.4 | 45.7±11.7 | 36.9±10.8§‡ | 51.7±11.9 | 45.6±9.5# | 34.5±12.4#& | ||
PAEF (%) | 23.5±11.1 | 27.7±10.0 | 33.8±10.6*† | 26.2±11.4 | 29.4±10.3 | 23.5±10.6‡ | 30.6±15.5 | 28.6±9.3 | 24.0±10.2 | ||
AAEF (%) | 23.0±9.3 | 22.9±13.4 | 20.6±8.6 | 25.2±10.9 | 23.0±12.7 | 17.5±8.8§ | 30.2±9.7 | 23.5±11.2# | 13.8±12.0#& | ||
TAPSE (mm) | 23.0±3.0 | 23.9±3.0 | 24.2±2.4 | 23.1±3.0 | 23.9±3.0 | 24.1±2.7 | 23.5±3.1 | 23.9±3.0 | 23.9±2.7 |
Data are presented as mean ± standard deviation. *, P<0.05 vs. RA-Vmax ≤33.2 mL; †, P<0.05 vs. 33.2 mL < RA-Vmax <65.8 mL; §, P<0.05 vs. RA-VpreA ≤23.8 mL; ‡, P<0.05 vs. 23.8 mL < RA-VpreA <46.2 mL; #P<0.05 vs. RA-Vmin ≤17.3 mL; &P<0.05 vs. 17.3 mL< RA-Vmin <37.1 mL. RA, right atrial; Vmax, end-systolic maximum RA volume; VpreA, early diastolic preatrial contraction RA volume; Vmin, end-diastolic minimum RA volume; TASV, total atrial stroke volume; PASV, passive atrial stroke volume; AASV, active atrial stroke volume; TAEF, total atrial emptying fraction; PAEF, passive atrial emptying fraction; AAEF, active atrial emptying fraction; TAPSE, tricuspid annular plane systolic excursion.
Correlations
None of the RA volumes showed correlations with TAPSE.
Interobserver and intraobserver agreement
The mean difference in measured parameters by 2 examiners for RA-Vmax, RA-VpreA, RA-Vmin and TAPSE was 0.9±5.0, −1.3±7.9, 0.9±4.1 mL and 1.2±0.4 mm, respectively. ICC proved to be 0.95 (P<0.001), 0.90 (P<0.001), 0.93 (P<0.001) and 0.98 P<0.001) (interobserver agreement). The mean difference in measured parameters by examiner 1 two times for RA-Vmax, RA-VpreA, RA-Vmin and TAPSE was 1.0±6.1, −1.5±8.9, 0.7±5.1 mL, and 1.2±0.4 mm, respectively. ICC proved to be 0.96 (P<0.001), 0.88 (P<0.001), 0.97 (P<0.001) and 0.98 P<0.001) (intraobserver agreement).
Discussion
In order to get to know the coordinated functioning of the RV, TA and RA, easy-to-perform clinical tests, even on healthy subjects, can help. 3DSTE is suitable for the volumetric examination of the RA by taking into account its changes according to the cardiac cycle. The RA has different phases of function: it behaves like a reservoir during systole, a conduit in early diastole allowing blood flow from the veins to the RV and acting like an actively contracting cavity in late diastole like a booster pump. While the LV looks like a bullet or egg, the RV is fundamentally different from the LV: it is located around the LV and has a triangular shape from the sides, its cross-sectional view it resembles a crescent, widening from the apex of the heart to its base. The deep RV wall muscle fibers are responsible for the base-to-apex longitudinal movement, as a result of which the RV longitudinal axis shortens and the tricuspid valve (TV) moves towards the apex. Superficially located circumferential fibers parallel to the TV are responsible for the movements towards the cavity of the RV (“bellows” effect) (8,15,16). TAPSE characterizes the longitudinal displacement of the TA, which is related to RV contraction with a prognostic power that has long been known and used to characterize RV longitudinal shortening (8,11-13).
According to the presented findings, RA volumes, SVs and EFs were not related to TAPSE, and RA volumes showed no associations and correlations with TAPSE under healthy circumstances. These results suggest independency of RA volumes from RV longitudinal shortening and vice versa. These findings have to be considered in the context of previous results. Lower TAPSE was associated with lower 3DSTE-derived TA fractional area change, which was related to more dilated end-systolic TA area. However, direct correlations between TA longitudinal and sphincter-like features could not be detected (17). Moreover, strong associations between TA dimensions and RA volumes could be demonstrated even under healthy circumstances (5). In summary, these results suggest that although TAPSE-represented RV longitudinal shortening does not correlate with RA volumes, it does associate with TA sphincter-like features, with certain relationship between the two types of TA functions. It is important to emphasize that these associations were found in healthy subjects who did not have functional tricuspid regurgitation (FTR). With other words, while our previous results suggested the role of TA sphincter-like features in the development of FTR, the same cannot be said about RV longitudinal shortening. However, further studies are warranted to confirm our findings, even is certain disorders with concomitant FTR.
Limitation section
Here the most important limitations are listed:
- The lower image quality associated with 3DSTE compared to two-dimensional echocardiography is a well-known fact, which could significantly influence our results (1-4).
- Only limited number of healthy subjects being in sinus rhythm were involved into this study. The findings would have been stronger, if larger number of healthy cases were included.
- Although not only volumetric, but strain data characterizing RA wall contractility can be measured as well using the same virtual RA cast during its assessment, we did not aim to analyze them in this study.
- Moreover, neither volumetric nor strain and other functional features of other cardiac chambers were performed either.
- 3DSTE-derived atrial volumetric assessments and TAPSE measurement are validated procedures, therefore it was not aimed to validate them again (13,18).
- Six wedge-shaped subvolumes during 6 cardiac cycles were acquired during the acquisition phase of 3DSTE analysis, which increases the risk of stitching and motion artifacts. However, considering all benefits of 3DSTE-based RA volumetric analysis, its importance and accuracy are unquestionable.
- STE-derived TA functionality analysis was not purposed either.
- It would have been more convincing, if more advanced methods were used for FTR quantification.
- Although healthy individuals were involved, the possibility that individuals with latent disease were included in the clinical trial cannot be completely excluded. Further special laboratory or imaging tests would have helped with these exclusions.
Conclusions
3DSTE-derived RA volumes and M-mode echocardiography-derived TAPSE representing RV longitudinal shortening are not associated in healthy adults.
Acknowledgments
Funding: None.
Footnote
Reporting Checklist: The authors have completed the STROBE reporting checklist. Available at https://qims.amegroups.com/article/view/10.21037/qims-24-223/rc
Conflicts of Interest: All authors have completed the ICMJE uniform disclosure form (available at https://qims.amegroups.com/article/view/10.21037/qims-24-223/coif). A.N. serves as an unpaid editorial board member of Quantitative Imaging in Medicine and Surgery. The other authors have no conflicts of interest to declare.
Ethical Statement: The authors are accountable for all aspects of the work in ensuring that questions related to the accuracy or integrity of any part of the work are appropriately investigated and resolved. The study was approved by the Institutional and Regional Human Biomedical Research Committee of University of Szeged, Hungary (registration number: 71/2011, latest approval for prolongation: February 20, 2023). All individuals who participated in the study signed informed consent. The study was in line with the Helsinki Declaration (as revised in 2013).
Open Access Statement: This is an Open Access article distributed in accordance with the Creative Commons Attribution-NonCommercial-NoDerivs 4.0 International License (CC BY-NC-ND 4.0), which permits the non-commercial replication and distribution of the article with the strict proviso that no changes or edits are made and the original work is properly cited (including links to both the formal publication through the relevant DOI and the license). See: https://creativecommons.org/licenses/by-nc-nd/4.0/.
References
- Ammar KA, Paterick TE, Khandheria BK, Jan MF, Kramer C, Umland MM, Tercius AJ, Baratta L, Tajik AJ. Myocardial mechanics: understanding and applying three-dimensional speckle tracking echocardiography in clinical practice. Echocardiography 2012;29:861-72. [Crossref] [PubMed]
- Urbano-Moral JA, Patel AR, Maron MS, Arias-Godinez JA, Pandian NG. Three-dimensional speckle-tracking echocardiography: methodological aspects and clinical potential. Echocardiography 2012;29:997-1010. [Crossref] [PubMed]
- Muraru D, Niero A, Rodriguez-Zanella H, Cherata D, Badano L. Three-dimensional speckle-tracking echocardiography: benefits and limitations of integrating myocardial mechanics with three-dimensional imaging. Cardiovasc Diagn Ther 2018;8:101-17. [Crossref] [PubMed]
- Gao L, Lin Y, Ji M, Wu W, Li H, Qian M, Zhang L, Xie M, Li Y. Clinical Utility of Three-Dimensional Speckle-Tracking Echocardiography in Heart Failure. J Clin Med 2022;11:6307. [Crossref] [PubMed]
- Nemes A, Kormányos Á, Rácz G, Ruzsa Z, Achim A, Ambrus N, Lengyel C. Tricuspid annular and right atrial volume changes are associated in healthy adults-insights from the three-dimensional speckle-tracking echocardiographic MAGYAR-Healthy Study. Front Cardiovasc Med 2023;10:1140599. [Crossref] [PubMed]
- Nemes A, Kormányos Á, Domsik P, Kalapos A, Ambrus N, Lengyel C. Normal reference values of three-dimensional speckle-tracking echocardiography-derived right atrial volumes and volume-based functional properties in healthy adults (Insights from the MAGYAR-Healthy Study). J Clin Ultrasound 2020;48:263-8. [Crossref] [PubMed]
- Nemes A, Kormányos Á. Right atrial volumes and strains in healthy adults: is the Frank-Starling mechanism working?-detailed analysis from the three-dimensional speckle-tracking echocardiographic MAGYAR-Healthy Study. Quant Imaging Med Surg 2023;13:825-34. [Crossref] [PubMed]
- Rudski LG, Lai WW, Afilalo J, Hua L, Handschumacher MD, Chandrasekaran K, Solomon SD, Louie EK, Schiller NB. Guidelines for the echocardiographic assessment of the right heart in adults: a report from the American Society of Echocardiography endorsed by the European Association of Echocardiography, a registered branch of the European Society of Cardiology, and the Canadian Society of Echocardiography. J Am Soc Echocardiogr 2010;23:685-713; quiz 786-8. [Crossref] [PubMed]
- Tadic M. The right atrium, a forgotten cardiac chamber: An updated review of multimodality imaging. J Clin Ultrasound 2015;43:335-45. [Crossref] [PubMed]
- Putthapiban P, Amini MR, Abudayyeh I. Anatomy of the Tricuspid Valve and Pathophysiology of Tricuspid Regurgitation. Interv Cardiol Clin 2022;11:1-9. [Crossref] [PubMed]
- Ghio S, Recusani F, Klersy C, Sebastiani R, Laudisa ML, Campana C, Gavazzi A, Tavazzi L. Prognostic usefulness of the tricuspid annular plane systolic excursion in patients with congestive heart failure secondary to idiopathic or ischemic dilated cardiomyopathy. Am J Cardiol 2000;85:837-42. [Crossref] [PubMed]
- Tamborini G, Pepi M, Galli CA, Maltagliati A, Celeste F, Muratori M, Rezvanieh S, Veglia F. Feasibility and accuracy of a routine echocardiographic assessment of right ventricular function. Int J Cardiol 2007;115:86-9. [Crossref] [PubMed]
- Sato T, Tsujino I, Ohira H, Oyama-Manabe N, Yamada A, Ito YM, Goto C, Watanabe T, Sakaue S, Nishimura M. Validation study on the accuracy of echocardiographic measurements of right ventricular systolic function in pulmonary hypertension. J Am Soc Echocardiogr 2012;25:280-6. [Crossref] [PubMed]
- Lang RM, Badano LP, Mor-Avi V, Afilalo J, Armstrong A, Ernande L, Flachskampf FA, Foster E, Goldstein SA, Kuznetsova T, Lancellotti P, Muraru D, Picard MH, Rietzschel ER, Rudski L, Spencer KT, Tsang W, Voigt JU. Recommendations for cardiac chamber quantification by echocardiography in adults: an update from the American Society of Echocardiography and the European Association of Cardiovascular Imaging. Eur Heart J Cardiovasc Imaging 2015;16:233-70. [Crossref] [PubMed]
- Haddad F, Hunt SA, Rosenthal DN, Murphy DJ. Right ventricular function in cardiovascular disease, part I: Anatomy, physiology, aging, and functional assessment of the right ventricle. Circulation 2008;117:1436-48. [Crossref] [PubMed]
- Ho SY, Nihoyannopoulos P. Anatomy, echocardiography, and normal right ventricular dimensions. Heart 2006;92:i2-13. [Crossref] [PubMed]
- Nemes A, Rácz G, Kormányos Á, Ruzsa Z, Achim A, Lengyel C. The Relationship between Tricuspid Annular Longitudinal and Sphincter-like Features of Its Function in Healthy Adults: Insights from the MAGYAR-Healthy Study. Life (Basel) 2023;13:2079. [Crossref] [PubMed]
- Nemes A, Forster T. Echocardiographic evaluation of the right atrium - from M-mode to 3D speckle-tracking imaging. Orv Hetil 2016;157:1698-707. [Crossref] [PubMed]