Computed tomography (CT) quantitative assessment of single lobe emphysema correlates with chronic obstructive pulmonary disease (COPD) severity: a cross-sectional study with retrospective data collection
Introduction
Chronic obstructive pulmonary disease (COPD) is a major cause of high mortality worldwide and huge cost drain on healthcare systems (1,2). Computed tomography (CT) is the preferred method of medical imaging in COPD because it can identify key morphological features such as emphysema, bronchial wall thickening, and gas trapping (3-5). Quantitative analysis of CT can reflect histopathologic changes and functional status. For example, the use of −950 Hounsfield units (HU) as a threshold to assess emphysema volume during deep inspiration has been pathologically confirmed (4). Many researchers have studied the quantitative parameters of CT and the correlation between the parameters of CT and those of pulmonary function test (PFT) in the past and have shown good results, yet most of these works have focused on the whole lung level (6-8). Meanwhile Zach et al. (9) reported the difference in volume changes (∆LV) and mean lung density between the upper and lower lobes during 1 respiratory cycle, indicating that analyzing pulmonary functional imaging at the whole lung level is insufficient in describing the status of lungs. This is because emphysema distribution in different lobes requires different treatments (such as lung volume reduction surgery) in clinical practice due to the heterogeneity of emphysematous changes in COPD patients (10-12). Therefore, it is necessary to study the changes in each lobe in depth and analyze each lobe independently to guide clinical treatment measures. In this study, we analyzed the biphasic CT lung volume parameters and the percentage of emphysema in different lobes of the lungs of patients with different grades of COPD and assessed their relationship with different lung function indices. We present this article in accordance with the STROBE reporting checklist (available at https://qims.amegroups.com/article/view/10.21037/qims-23-1496/rc).
Methods
Participants
Patients who underwent PFT at The First Affiliated Hospital of Guangzhou Medical University between 1 July 2019 and 27 January 2020 and underwent biphasic CT scan of chest respiration within 1 week were selected. The inclusion and grading criteria were as follows: according to the grading of Global Initiative for Chronic Obstructive Lung Disease (GOLD), after bronchodilator inhalation, pulmonary function meeting postbronchodilator ratio of the first second forced expiratory volume to forced vital capacity (post-FEV1/FVC) ≥70% were considered as GOLD 0, pulmonary function meeting post-FEV1/FVC <70% and postbronchodilator forced expiratory volume in the first second (measured/predicted values) [post-FEV1 (%Pred)] >80% were considered as GOLD I, pulmonary function meeting post-FEV1/FVC <70% and 80% ≥ post-FEV1 (%Pred) >50% were considered GOLD II, pulmonary function meeting post-FEV1/FVC <70% and 50%≥ post-FEV1 (%Pred) >30% were considered GOLD III, and those with post-FEV1/FVC <70% and post-FEV1 (%Pred) ≤30% were considered GOLD IV. The exclusion criteria were as follows: patients with acute exacerbations, patients with a history of pulmonary surgery, patients with lung diseases that interfere with pulmonary function assessment, such as asthma, lobar pneumonia, and bronchiectasis, and patients with poor quality chest respiratory duplex CT scans. Initially, 452 patients were included. In addition, we excluded 43 patients who failed to complete quantitative analysis of CT images. Finally, a total of 409 patients were enrolled, including 112 patients with GOLD 0, 114 patients with GOLD I, 144 patients with GOLD II, 34 patients with GOLD III, and 5 patients with GOLD IV. Since GOLD I and II are considered the early stages of COPD, and there were very few GOLD IV cases in this group, this study was divided into the following groups according to the severity of pulmonary function when discussing CT lung parenchymal parameters. In addition to including the above 112 GOLD 0 patients as non-COPD controls, we created a mildly to moderately severe group that combined the GOLD I and II patients of 258 cases in total, and severe to extremely severe group that combined the GOLD III and IV patients of 39 cases in total (Table 1). The enrollment of patients is shown in Figure 1. The study was conducted in accordance with the Declaration of Helsinki (as revised in 2013). The study was approved by the Ethics Committee of The First Affiliated Hospital of Guangzhou Medical University (No. 2018-53). Prior to commencement of the study, all participants were given information about radiation exposure of CT and provided written informed consent.
Table 1
Baseline data | Non-COPD control (n=112) |
Mildly to moderately severe group (n=258) |
Severe to extremely severe group (n=39) |
---|---|---|---|
Sex (male/female) | 64/48 | 238/20 | 37/2 |
Age (years) (mean ± SEM) | 59.57±0.75 | 66.23±0.47 | 69.54±1.35 |
Height (cm) (mean ± SEM) | 159.17±0.73 | 161.92±0.42 | 162.51±1.16 |
Weight (kg) (mean ± SEM) | 59.99±0.91 | 59.09±0.61 | 55.17±1.55 |
BMI (kg/m2) (mean ± SEM) | 23.62±0.29 | 22.48±0.19 | 20.86±0.52 |
Smoking history (have/no) | 44/68 | 237/21 | 36/3 |
COPD, chronic obstructive pulmonary disease; SEM, standard error of mean; BMI, body mass index.
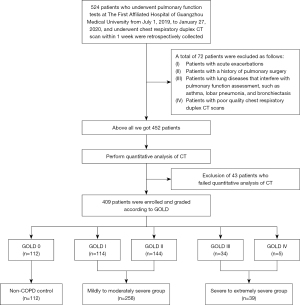
Imaging examination instrument and parameters
The respiratory duplex chest spiral CT scan was performed on Siemens SOMATOM Definition AS+ 128-plane CT (Siemens, Erlangen, Germany), and all patients underwent routine breathing training (deep inhalation followed by breath-hold and deep exhalation followed by breath-hold) prior to the scan. Inspiratory CT refers to the scan taken when the participant inhales to the best of their ability, at which point their lung volume is at total lung capacity (TLC). Expiratory CT refers to the scan taken when the participant exhales to the best of their ability, at which point their lung volume is at residual volume (RV). Scanning parameters were tube voltage: 120 kV, tube current: automatic tube current modulation technique (CARE Dose 4D), field of view (FOV): 400×400 mm, matrix: 512×512, thickness: 1 mm, interval: 1 mm; inspiratory phase scanned from the base to the tip of the lung, expiratory phase scanned from the tip to the base of the lung.
PFT instruments
We used PFT instruments from COSMED Quark PFT series pulmonary function instruments (COSMED, Rome, Italy). All PFT instruments met the instrument quality control standards recommended by the American Thoracic Society (ATS)/European Respiratory Society (ERS). Participants were seated during testing.
Quantitative analysis of CT
The lung quantification software VIDA Apollo Version 2.2 (Apollo, Nashville, TN, USA), was used for quantitative analysis of CT (Figure 2). The process was as follows. First, the lung tissue was divided from the trachea, bronchi, mediastinal vessels, and the soft tissue of the chest wall (Figure 2A). If there was a deviation in the division, it was corrected manually (Figure 2B). Second, we used software to automatically divide the lung lobes and filled them with different colors (Figure 2C). The software automatically saved the parameter values after manual correction for deviations. The biphasic CT lung volume parameters [inspiratory lung volume (LVin), expiratory lung volume (LVex), volume change/inspiratory lung volume (∆LV/LVin)] and the percentage of emphysema volume [low attenuation areas percent below the threshold of −950 in the inspiratory phase (%LAA−950in)] were collected for the left upper lobe (green area), left lower lobe (blue area), right upper lobe (brown area), right middle lobe (purple area), and right lower lobe (yellow area) of the patient’s biphasic breathing (Figure 2D).
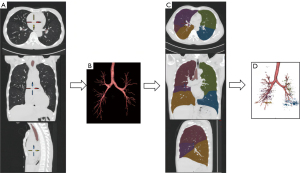
Statistical methods
Measurement data were expressed as mean ± standard deviation, and count data were expressed as frequencies. One-way analysis of variance (ANOVA) was used to compare 3 independent groups of quantitative data if the data of each group followed normal distribution and the variance was equal, otherwise the rank sum test (i.e., Kruskal-Wallis H method) was used. Multiple linear regression was used to assess the correlation between biphasic CT lung volume parameters in different lung lobes as well as emphysema volume ratios and different lung function indices. A two-sided test with of P<0.05 was taken as statistically significant.
Results
Comparison of the CT quantification parameters of different lobes between the groups
Comparison of LVin and LVex in different lobes between groups
The differences in CT lung volume parameters (LVin and LVex) were observed in the deep inspiratory and deep expiratory phases for each lung lobe in the non-COPD control, mildly to moderately severe, and severe to extremely severe groups (Table 2). Each of the 3 groups had statistically significant larger LVin in the left lower lobe (all P<0.05), indicating that LVin in the left lower lobe was greater in the group with poor pulmonary function. The LVin of the remaining lung lobes differed significantly between the non-COPD control and mildly to moderately severe groups, as well as between the non-COPD control and severe to extremely severe groups (all P<0.05), indicating that the LVin of the remaining lung lobes was smaller in the former group than in the latter. Except for the left lower lobe, there was no statistically significant difference between the mildly to moderately severe and severe to extremely severe groups (all P>0.05). The LVex of the right middle lobe was statistically different between the non-COPD control and mildly to moderately severe groups, as well as between the non-COPD control and severe-extremely severe groups (P<0.001 for both), indicating that the LVex of each lung lobe was smaller in the former than in the latter, and the deep expiratory CT lung volume of the remaining lung lobes was statistically different between each of the 3 groups (P<0.05), indicating that the LVex was larger in the group with poorer pulmonary function.
Table 2
LVin and LVex of different lobes |
Non-COPD control (n=112)a |
Mildly to moderately severe group (n=258)b |
Severe to extremely severe group (n=39)c |
P value | ||
---|---|---|---|---|---|---|
a vs. b | a vs. c | b vs. c | ||||
LVin (cm3) (mean ± SEM) | ||||||
LUL | 921.80±23.44 | 1,186.42±17.02 | 1,255.14±45.94 | <0.001 | <0.001 | 0.136 |
LLL | 815.94±25.67 | 994.44±17.65 | 1,124.06±41.01 | <0.001 | <0.001 | 0.007 |
RUL | 780.92±19.74 | 1,029.31±18.09 | 1,098.32±51.59 | <0.001 | <0.001 | 0.415 |
RML | 332.13±10.45 | 394.59±7.95 | 394.09±21.91 | <0.001 | 0.008 | 0.981 |
RLL | 956.91±26.35 | 1,127.28±18.99 | 1,159.95±41.06 | <0.001 | <0.001 | 0.518 |
LVex (cm3) (mean ± SEM) | ||||||
LUL | 453.58±15.20 | 793.02±16.13 | 992.98±46.25 | <0.001 | <0.001 | 0.001 |
LLL | 311.33±11.83 | 595.27±59.52 | 884.23±38.30 | 0.001 | <0.001 | 0.029 |
RUL | 392.98±15.11 | 691.22±15.83 | 895.47±50.76 | <0.001 | <0.001 | 0.001 |
RML | 210.68±9.50 | 291.13±6.32 | 324.83±14.43 | <0.001 | <0.001 | 0.051 |
RLL | 363.03±12.36 | 607.23±15.09 | 883.90±37.98 | <0.001 | <0.001 | <0.001 |
LVin, inspiratory lung volume; LVex, expiratory lung volume; COPD, chronic obstructive pulmonary disease; SEM, standard error of mean; LUL, left upper lobe; LLL, left lower lobe; RUL, right upper lobe; RML, right middle lobe; RLL, right lower lobe.
Comparison of LV/LVin in different lung lobes between groups
∆LV/LVin in each lung lobe was statistically significant between each of the 3 groups (all P<0.05), showing that ∆LV/LVin was smaller in the poorer pulmonary function group (Table 3).
Table 3
∆LV/LVin of different lobes |
Non-COPD control (n=112)a |
Mildly to moderately severe group (n=258)b |
Severe to extremely severe group(n=39)c |
P value | ||
---|---|---|---|---|---|---|
a vs. b | a vs. c | b vs. c | ||||
LUL (mean ± SEM) | 0.49±0.02 | 0.29±0.02 | 0.20±0.03 | <0.001 | <0.001 | 0.002 |
LLL (mean ± SEM) | 0.59±0.02 | 0.36±0.06 | 0.19±0.03 | <0.001 | <0.001 | <0.001 |
RUL (mean ± SEM) | 0.50±0.02 | 0.23±0.05 | 0.19±0.02 | <0.001 | <0.001 | 0.001 |
RML (mean ± SEM) | 0.30±0.05 | 0.15±0.06 | 0.12±0.03 | <0.001 | <0.001 | 0.015 |
RLL (mean ± SEM) | 0.59±0.02 | 0.42±0.02 | 0.21±0.03 | <0.001 | <0.001 | <0.001 |
∆LV/LVin, volume change/inspiratory lung volume; COPD, chronic obstructive pulmonary disease; LUL, left upper lobe; SEM, standard error of mean; LLL, left lower lobe; RUL, right upper lobe; RML, right middle lobe; RLL, right lower lobe.
Comparison of the percentage of CT emphysema volume in different lung lobes between groups and between each lobe
The percentage of emphysema volume (%LAA−950in) in each lobe was statistically different between each of the 3 groups (all P<0.001), with greater %LAA−950in in the group with poorer pulmonary function (Table 4, Figure 3). Comparison of %LAA−950in in each lung lobe showed that there was no significant difference in %LAA−950in between different lung lobes in the non-COPD control and severe to extremely severe group (P>0.05); in the mildly to moderately severe group, %LAA−950in in the upper lobes of both lungs was greater than that in the remaining lobes (P<0.05). The distribution of emphysema in each lobe of the lungs in different groups of patients is shown schematically in Figure 4.
Table 4
%LAA−950in of different lobes |
Non-COPD control (n=112)a |
Mildly to moderately severe group (n=258)b |
Severe to extremely severe group (n=39)c |
P value | ||
---|---|---|---|---|---|---|
a vs. b | a vs. c | b vs. c | ||||
LUL (mean ± SEM) | 0.62±0.07 | 5.67±0.45 | 12.91±1.95 | <0.001 | <0.001 | <0.001 |
LLL (mean ± SEM) | 0.62±0.08 | 4.63±0.35 | 15.78±1.95 | <0.001 | <0.001 | <0.001 |
RUL (mean ± SEM) | 0.89±0.41 | 5.83±0.53 | 13.42±2.13 | <0.001 | <0.001 | <0.001 |
RML (mean ± SEM) | 0.89±0.10 | 4.60±0.36 | 11.89±2.05 | <0.001 | <0.001 | <0.001 |
RLL (mean ± SEM) | 0.40±0.03 | 3.90±0.32 | 10.50±1.53 | <0.001 | <0.001 | <0.001 |
%LAA−950in, low attenuation areas percent below the threshold of –950 in the inspiratory phase; COPD, chronic obstructive pulmonary disease; LUL, left upper lobe; SEM, standard error of mean; LLL, left lower lobe; RUL, right upper lobe; RML, right middle lobe; RLL, right lower lobe.
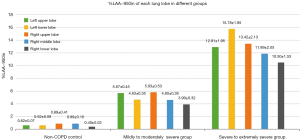
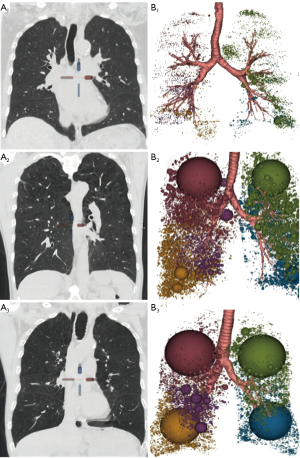
Multiple linear regression analysis of quantitative CT parameters of different lung lobes with different lung function indices
Correlation of LVin in each lung lobe with different lung function indices
The results of the overall significance test and adjusted R-square of the multiple linear regression analysis of LVin in each lung lobe with the 5 lung function indices are shown in Table 5. The multiple linear regression equations of LVin with maximal vital capacity (measured/predicted values) [VC max (%Pred)] were not significant for each lobe (P=0.276), whereas the multiple linear regression equations of LVin with the first second forced expiratory volume (measured/predicted values) [FEV1 (%Pred)], the first second forced expiratory volume/the forced vital capacity (FEV1/FVC), maximal mid-expiratory flow (measured/predicted values) [MMEF (%Pred)], and forced expiratory flow after 50% of the FVC has been exhaled (measured/predicted values) [FEF50% (%Pred)] were significant in the multiple linear regression equations (all P<0.001). In addition, LVin of each lung lobe had the best adjusted R-square to FEV1/FVC, which together explained 19.6% of the variance in FEV1/FVC (adjusted R2=0.196).
Table 5
Lung function indices | LVin | LVex | ∆LV/LVin | %LAA−950in |
---|---|---|---|---|
VC max (%Pred) | ||||
F | 1.269 | 7.951 | 6.073 | 13.29 |
P value | 0.276 | <0.001 | <0.001 | <0.001 |
Adjusted R2 | 0.003 | 0.078 | 0.059 | 0.131 |
FEV1 (%Pred) | ||||
F | 9.262 | 39.315 | 17.385 | 53.736 |
P value | <0.001 | <0.001 | <0.001 | <0.001 |
Adjusted R2 | 0.092 | 0.32 | 0.167 | 0.393 |
FEV1/FVC | ||||
F | 20.918 | 49.466 | 14.616 | 60.794 |
P value | <0.001 | <0.001 | <0.001 | <0.001 |
Adjusted R2 | 0.196 | 0.373 | 0.143 | 0.423 |
MMEF (%Pred) | ||||
F | 10.941 | 43.51 | 20.418 | 25.473 |
P value | <0.001 | <0.001 | <0.001 | <0.001 |
Adjusted R2 | 0.109 | 0.343 | 0.192 | 0.231 |
FEF50% (%Pred) | ||||
F | 11.349 | 46.872 | 21.49 | 30.047 |
P value | <0.001 | <0.001 | <0.001 | <0.001 |
Adjusted R2 | 0.113 | 0.36 | 0.201 | 0.263 |
CT, computed tomography; VC max (%Pred), maximal vital capacity (measured/predicted values); FEV1 (%Pred), the first second forced expiratory volume (measured/predicted values); FEV1/FVC, the first second forced expiratory volume/the forced vital capacity; MMEF (%Pred), maximal mid-expiratory flow (measured/predicted values); FEF50% (%Pred), forced expiratory flow after 50% of the FVC has been exhaled (measured/predicted values); LVin, inspiratory lung volume; LVex, expiratory lung volume; ∆LV/LVin, volume change/inspiratory lung volume; %LAA−950in, low attenuation areas percent below the threshold of −950 in the inspiratory phase.
Multiple linear regression coefficients of LVin with FEV1 (%Pred), FEV1/FVC, MMEF (%Pred), and FEF50% (%Pred) in each lung lobe are shown (Table 6). LVin in the left lower lobe was negatively correlated with FEV1 (%Pred), FEV1/FVC, MMEF (%Pred), and FEF50% (%Pred) and the absolute value of β was greater than that of the rest of the lobes (all β<0, all P<0.05). By comparing the magnitude of the absolute value of β, LVin of the left lower lobe had the most significant effect on FEV1/FVC (B=−0.108, β=−0.326, P<0.001). The LVin in the right middle lobe did not correlate with FEV1 (%Pred), FEV1/FVC, MMEF (%Pred), and FEF50% (%Pred) (all P >0.05). The LVin of the remaining lobes correlated with only some of the lung function indices.
Table 6
Lung function indices | LUL | LLL | RUL | RML | RLL |
---|---|---|---|---|---|
FEV1 (%Pred) | |||||
B | −0.011 | −0.025 | −0.008 | 0.016 | 0.016 |
β | −0.149 | −0.325 | −0.101 | 0.089 | 0.21 |
P value | 0.108 | <0.001 | 0.203 | 0.119 | 0.018 |
FEV1/FVC | |||||
B | −0.011 | −0.108 | −0.009 | 0.002 | 0.01 |
β | −0.207 | −0.326 | −0.168 | 0.018 | 0.195 |
P value | 0.018 | <0.001 | 0.025 | 0.74 | 0.02 |
MMEF (%Pred) | |||||
B | −0.013 | −0.015 | −0.009 | 0.003 | 0.011 |
β | −0.192 | −0.206 | −0.136 | 0.018 | 0.161 |
P value | 0.037 | 0.019 | 0.085 | 0.756 | 0.067 |
FEF50% (%Pred) | |||||
B | −0.015 | −0.019 | −0.011 | 0.004 | 0.014 |
β | −0.185 | −0.239 | −0.133 | 0.023 | 0.175 |
P value | 0.044 | 0.006 | 0.089 | 0.69 | 0.047 |
LVin, inspiratory lung volume; FEV1 (%Pred), the first second forced expiratory volume (measured/predicted values); FEV1/FVC, the first second forced expiratory volume/the forced vital capacity; MMEF (%Pred), maximal mid-expiratory flow (measured/predicted values); FEF50% (%Pred), forced expiratory flow after 50% of the FVC has been exhaled (measured predicted values); LUL, eft upper lobe; LLL, left lower lobe; RUL, right upper lobe; RML, right middle lobe; RLL, right lower lobe.
Correlation of LVex in each lung lobe with different lung function indices
The results of the overall significance test and adjusted R-square of the multiple linear regression analysis of LVex in each lung lobe with the 5 lung function indices are shown in Table 5. The multiple linear regression equations of LVex of each lung lobe with VC max (%Pred), FEV1 (%Pred), FEV1/FVC, MMEF (%Pred), and FEF50% (%Pred) were all significant (all P<0.001), with LVex of each lobe and FEF50% (%Pred) having the best adjusted R-squared and together explaining 36.0% of the variance in FEF50% (%Pred) (adjusted R2=0.360). Multiple linear regression coefficients of LVex in each lobe of the lungs with 5 lung function indices are shown in Table 7. LVex in the right lower lobe was negatively correlated with VC max (%Pred), FEV1 (%Pred), FEV1/FVC, MMEF (%Pred), and FEF50% (%Pred) and the absolute β-value was greater than that of the rest of the lobes (all β<0, all P<0.001). By comparing the magnitude of absolute values of β, LVin of the right lower lobe had the most significant effect on FEV1 (%Pred) (B=−0.031, β=−0.359, P<0.001). LVex in the right middle lobe was negatively correlated with FEV1/FVC, MMEF (%Pred), and FEF50% (%Pred) (all β<0, P<0.05), and LVex in the right middle lobe did not correlate definitively with VC max (%Pred), and FEV1 (%Pred) (P=0.324, P=0.065). No clear correlation existed between LVex of the remaining lobes and the 5 lung function indices (all P>0.05).
Table 7
Lung function indices | LUL | LLL | RUL | RML | RLL |
---|---|---|---|---|---|
VC max (%Pred) | |||||
B | −0.004 | 0.001 | 0.001 | 0.009 | −0.019 |
β | −0.069 | 0.03 | 0.019 | 0.055 | −0.284 |
P value | 0.564 | 0.554 | 0.849 | 0.324 | <0.001 |
FEV1 (%Pred) | |||||
B | −0.008 | −0.001 | −0.009 | −0.018 | −0.031 |
β | −0.105 | −0.018 | −0.110 | −0.088 | −0.359 |
P value | 0.311 | 0.674 | 0.192 | 0.065 | <0.001 |
FEV1/FVC | |||||
B | −0.006 | −0.001 | −0.009 | −0.026 | −0.019 |
β | −0.113 | −0.040 | −0.153 | −0.171 | −0.299 |
P value | 0.255 | 0.338 | 0.059 | <0.001 | <0.001 |
MMEF (%Pred) | |||||
B | −0.011 | −0.001 | −0.006 | −0.025 | −0.024 |
β | −0.163 | −0.041 | −0.089 | −0.129 | −0.307 |
P value | 0.108 | 0.346 | 0.281 | 0.006 | <0.001 |
FEF50% (%Pred) | |||||
B | −0.013 | −0.001 | −0.007 | −0.031 | −0.029 |
β | −0.161 | −0.036 | −0.087 | −0.143 | −0.319 |
P value | 0.108 | 0.397 | 0.287 | 0.002 | <0.001 |
LVex, expiratory lung volume; VC max (%Pred), maximal vital capacity (measured/predicted values); FEV1 (%Pred), the first second forced expiratory volume (measured/predicted values); FEV1/FVC, the first second forced expiratory volume/the forced vital capacity; MMEF (%Pred), maximal mid-expiratory flow (measured/predicted values); FEF50% (%Pred), forced expiratory flow after 50% of the FVC has been exhaled (measured/predicted values); LUL, left upper lobe; LLL, left lower lobe; RUL, right upper lobe; RML, right middle lobe; RLL, right lower lobe.
Correlation of ∆LV/LVin in each lung lobe with different lung function indices
The results of the overall significance test and the adjusted R-square of the multiple linear regression analysis of ∆LV/LVin in each lung lobe with different lung function indices are shown in Table 5. The multiple linear regression equations of ∆LV/LVin of each lung lobe with VC max (%Pred), FEV1 (%Pred), FEV1/FVC, MMEF (%Pred), and FEF50% (%Pred) were all significant (all P<0.001), with ∆LV/LVin of each lobe and FEF50% (%Pred) having the best adjusted R-squared and together explaining 20.1% of the variance in FEF50% (%Pred) (Adjusted R2=0.201). Multiple linear regression coefficients of ∆LV/LVin in each lung lobe with different lung function indices are shown in Table 8. ∆LV/LVin in the right lower lobe was positively correlated with VC max (%Pred), FEV1 (%Pred), FEV1/FVC, MMEF (%Pred), and FEF50% (%Pred) and the absolute value of β was greater than that of the rest of the lobes (all β>0, all P≤0.001). By comparing the magnitude of absolute values of β, ∆LVin of the right lower lobe had the most significant effect on FEF50% (%Pred) (B=27.900, β=0.322, P<0.001). ∆LV/LVin in the upper lobe of the left lung was positively correlated with FEV1 (%Pred), FEV1/FVC, MMEF (%Pred), and FEF50% (%Pred) (all β>0, all P<0.05), and ∆LV/LVin in the upper lobe of the left lung did not correlate unequivocally with VC max (%Pred) (P=0.079). No clear correlation existed between ∆LV/LVin of the remaining lobes and the 5 lung function indices (all P>0.05).
Table 8
Lung function indices | LUL | LLL | RUL | RML | RLL |
---|---|---|---|---|---|
VC max (%Pred) | |||||
B | 7.32 | −0.822 | −0.339 | −1.644 | 13.273 |
β | 0.116 | −0.038 | −0.012 | −0.074 | 0.201 |
P value | 0.079 | 0.453 | 0.83 | 0.14 | 0.001 |
FEV1 (%Pred) | |||||
B | 13.939 | 0.251 | −1.265 | −1.761 | 25.723 |
β | 0.176 | 0.009 | −0.035 | −0.063 | 0.311 |
P value | 0.005 | 0.864 | 0.496 | 0.179 | <0.001 |
FEV1/FVC | |||||
B | 8.759 | 0.686 | −1.026 | −0.717 | 17.185 |
β | 0.153 | 0.035 | −0.039 | −0.035 | 0.287 |
P value | 0.015 | 0.469 | 0.452 | 0.456 | <0.001 |
MMEF (%Pred) | |||||
B | 11.75 | 1.258 | −0.718 | 0.178 | 24.368 |
β | 0.161 | 0.05 | −0.021 | 0.007 | 0.32 |
P value | 0.009 | 0.283 | 0.671 | 0.881 | <0.001 |
FEF50 (%Pred) | |||||
B | 14.492 | 1.261 | −0.627 | −0.098 | 27.9 |
β | 0.174 | 0.044 | −0.016 | −0.003 | 0.322 |
P value | 0.004 | 0.342 | 0.743 | 0.942 | <0.001 |
∆LV/LVin, volume change/inspiratory lung volume; VC max (%Pred), maximal vital capacity (measured/predicted values); FEV1 (%Pred), the first second forced expiratory volume (measured/predicted values); FEV1/FVC, the first second forced expiratory volume/the forced vital capacity; MMEF (%Pred), maximal mid-expiratory flow (measured/predicted values); FEF50% (%Pred), forced expiratory flow after 50% of the FVC has been exhaled (measured/predicted values); LUL, left upper lobe; LLL, left lower lobe; RUL, right upper lobe; RML, right middle lobe; RLL, right lower lobe.
Correlation between the percentage of emphysema volume (%LAA−950in) in each lung lobe and different lung function indices
The results of the overall significance test and adjusted R-square of the multiple linear regression analysis of %LAA−950in in each lung lobe with different lung function indices are shown (Table 5). The multiple linear regression equations of %LAA−950in of each lung lobe with VC max (%Pred), FEV1 (%Pred), FEV1/FVC, MMEF (%Pred), and FEF50% (%Pred) were all significant (all P<0.001). The %LAA−950in of each lung lobe had the best adjusted R-square to FEV1/FVC, which together explained 42.3% of the variance in FEV1/FVC (adjusted R2=0.423).
Multiple linear regression coefficients of %LAA−950in with different lung function indices in each lung lobe are shown in Table 9. In the left upper lobe, %LAA−950in was negatively correlated with VC max (%Pred), FEV1 (%Pred), FEV1/FVC, MMEF (%Pred), and FEF50% (%Pred), and the absolute β values were greater than those of the remaining lobes (all β<0, all P<0.001). By comparing the magnitude of the absolute value of β, %LAA−950in of the left lower lobe had the most significant effect on VC max (%Pred) (B=−0.946, β=−0.375, P<0.001). The %LAA−950in in the right lower lobe was negatively correlated with FEV1 (%Pred), FEV1/FVC, and FEF50% (%Pred) (all β<0, P<0.05), and there was no clear correlation between the %LAA−950in in the upper lobe of the left lung and VC max (%Pred), and MMEF (%Pred) (P=0.848, P=0.073). No clear correlation existed between %LAA−950in in the remaining lobes and the 5 lung function indices (all P>0.05).
Table 9
Lung function indices | LUL | LLL | RUL | RML | RLL |
---|---|---|---|---|---|
VC max (%Pred) | |||||
B | 0.184 | −0.946 | −0.296 | 0.153 | −0.045 |
β | 0.078 | −0.375 | −0.148 | 0.057 | −0.014 |
P value | 0.414 | <0.001 | 0.086 | 0.378 | 0.848 |
FEV1 (%Pred) | |||||
B | −0.137 | −1.178 | −0.332 | −0.085 | −0.653 |
β | −0.046 | −0.372 | −0.132 | −0.025 | −0.167 |
P value | 0.563 | <0.001 | 0.066 | 0.639 | 0.008 |
FEV1/FVC | |||||
B | −0.285 | −0.603 | −0.143 | −0.203 | −0.621 |
β | −0.134 | −0.263 | −0.079 | −0.084 | −0.220 |
P value | 0.088 | <0.001 | 0.261 | 0.113 | <0.001 |
MMEF (%Pred) | |||||
B | −0.298 | −0.679 | −0.143 | −0.134 | −0.454 |
β | −0.110 | −0.239 | −0.062 | −0.044 | −0.126 |
P value | 0.225 | <0.001 | 0.444 | 0.476 | 0.073 |
FEF50% (%Pred) | |||||
B | −0.321 | −0.083 | −0.208 | −0.174 | −0.561 |
β | −0.104 | −0.250 | −0.079 | −0.050 | −0.137 |
P value | 0.24 | <0.001 | 0.318 | 0.406 | 0.047 |
%LAA−950in, low attenuation areas percent below the threshold of −950 in the inspiratory phase; VC max (%Pred), maximal vital capacity (measured/predicted values); FEV1 (%Pred), the first second forced expiratory volume (measured/predicted values); FEV1/FVC, the first second forced expiratory volume/the forced vital capacity; MMEF (%Pred), maximal mid-expiratory flow (measured/predicted values); FEF50% (%Pred), forced expiratory flow after 50% of the FVC has been exhaled (measured/predicted values); LUL, left upper lobe; LLL, left lower lobe; RUL, right upper lobe; RML, right middle lobe; RLL, right lower lobe.
Discussion
Pulmonary function measurement by CT assesses pulmonary tissue function mainly by changes in lung volume or lung density (5). For PFT, RV not directly detectable by spirometry requires indirect conversion by nitrogen flushing or volumetric tracing (13,14), such as RV, functional residual capacity (FRC), and TLC. These indices inevitably include the volume of air within the tracheobronchial tree and thus may overestimate the true ventilatory function of the lung. With paired inspiratory-expiratory chest CT, post-processing software can automatically extract and remove air within the tracheobronchial tree, providing more accurate quantitative values of total lung volume than TLC measured by PFT. Previous studies (15-17) have shown that CT lung volume quantification parameters can effectively discriminate between different grades of COPD and correlate well with pulmonary function volume indexes such as RV, TLC, and FEV1/FVC, and others.
Previously, LVin and LVex were the most commonly used parameters to measure CT lung volumes (17,18). The quantitative CT lung volume analysis of biphasic breathing in this investigation revealed that LVin and LVex were considerably higher in each lobe of the COPD group compared to the non-COPD control, regardless of the inspiratory or expiratory phase. This could be owing to the impaired alveolar retraction of COPD patients, which leads to larger lung volumes due to air trapping. However, there was no statistical difference in lung volume between the mildly to moderately severe and severe to extremely severe groups in the inspiratory or expiratory phase of some lobes. We believe that just comparing LVin and LVex, or directly comparing biphasic volume differences is susceptible to the influence of individual lung volume variances, and that the rate of volume change (∆LV/LVin) may help to balance this influence. Our findings show that ∆LV/LVin is superior to simple LVin and LVex in distinguishing between non-COPD and different degrees of COPD.
Our study found statistically significant differences in %LAA−950in in each lung lobe between all 3 groups. However, in the non-COPD control and severe to extremely severe COPD groups, there was no statistical difference in %LAA−950in. Meanwhile, in the mildly to moderately severe COPD population, the %LAA−950in were higher in the upper lobes of both lungs than in the other lobes. The distribution of percentage of emphysema volume in patients with different degrees of COPD suggests that in mild COPD, lung damage is more pronounced in the upper lung than in the lower lung, whereas differences in percentage of emphysema volume in lobe distribution decrease or disappear as COPD worsens. According to a previous study (19), emphysema begins in the upper lobes of both lungs and subsequently advances to the lower lobes of both lungs, which could be attributed to the following reasons: (I) related to negative intrathoracic pressure: when a person is upright, negative intrathoracic pressure shows a gradient difference in negative pressure values decreasing from top to bottom, and air trapping is more likely to occur in both upper lobes; and (II) related to ischemia-reperfusion: when both the upper and lower lobes are hypoxic, the lower lobes’ reperfusion compensatory ability is greater than that of the upper lobes. Therefore, when decompensation caused significant parenchymal destruction occurred in both lower lobes, the pulmonary function drop was already quite severe. It is also necessary to understand that lung ventilation and perfusion are gravity dependent. When someone stands up, the upper lobes are better ventilated and less perfused, while the lower lobes are better perfused and less ventilated (20). The supine position was employed in this investigation to correct for the effects of gravity and to represent the true status of the disease as accurately as feasible. From another perspective, however, when studying the correlation between PFT and biphasic CT parameters, the difference in the participant’s body position (seated for PFT and supine for CT) may have had some impact on the results.
In addition, we assessed the correlation of LVin, LVex, and ∆LV/LVin with different lung function indices in each lung lobe. There was a clear negative correlation between LVin in the left lower lobe and the remaining four lung function indices, except for VC max (%Pred). This was reflected by the fact that the larger LVin in the left lower lobe, the worse patient’s lung function. Qi et al. (21) employed a computational fluid dynamics approach to predict lung airflow using a whole-inhalation CT image model and discovered that the left lower lung volume distribution was the greatest of the 5 lung lobes. This is consistent with our findings that the left lower lung takes the largest ventilation role among the 5 lobes, so when COPD patients present with emphysematous changes, the inspiratory phase volume change of the left lower lobe will be greater than that of the other lobes, and will be more sensitive to changes in lung function than the other lobes. We also found significant correlations between LVex and ∆LV/LVin in the right lower lobe and 5 lung function indexes. In particular, LVex in the right lower lobe of the lung was negatively correlated with all 5 lung function indices, as demonstrated by the fact that a larger LVex in the right lower lobe of the lung was associated with lower poor lung function. A larger LVex responded to a more pronounced gas trapping condition, which manifested itself as poorer lung function. ∆LV/LVin of the right lower lobe was positively correlated with all five lung function indices, as demonstrated by the fact that ∆LV/LVin in the larger right lower lobe of the lung was associated with better lung function. However, LVin, LVex, and ∆LV/LVin in the upper lobes of both lungs and the right middle lobe correlated with only some of the lung function indices or with none of the 5 lung function indices. Therefore, we concluded that the correlations between LVin, LVex, and ∆LV/LVin and different lung function indices in the upper lobes of both lungs and the right middle lobe were equivocal.
Boueiz et al. (10) investigated the relationship between upper lobe predominant emphysema and lower lobe predominant emphysema in relation to clinical diagnosis and disease progression, and showed that those with lower lobe predominant emphysema had more severe airflow obstruction and a higher incidence of metabolic syndrome compared to cases with upper lobe predominant emphysema. In the present study, we directly analyzed the correlation between %LAA−950in and lung function indices in different lobes. We found that %LAA−950in in the left lower lobe was negatively correlated with different lung function indices, whereas %LAA−950in in the right lower lung was negatively correlated with some lung function indices. However, there was no clear correlation between the upper lobes of both lungs and the right middle lobe with different lung function indices. Therefore, we concluded that %LAA−950in in the left lower lobe better reflected the changes in different lung function indices of the patients, whereas %LAA−950in in the upper lobes of both lungs and the right middle lobe did not have a clear correlation with different lung function indices.
Patients with normal lung function and various COPD grades were selected for this study. As most of the patients with severe COPD were in poor condition, they were often unable to go to the hospital due to dyspnea, and it was even more difficult for them to cooperate with PFT and CT examinations. Therefore, the number of patients with severe and extremely severe COPD included in this study was low, which may have impacted the results of the study. In the present study, we were unable to include lung function data from diffusion capacity of the lung for carbon monoxide (DLCO), a nonradiographic indicator that correlates best with emphysema (22). This indicator would allow a better assessment of the correlation between CT parameters and lung function, which would have made a limitation of our study. Airflow obstruction in patients with COPD can be caused by emphysema or airway thickening associated with chronic bronchitis, which can now be measured automatically by machine software (23), so performing CT to quantify different lung lobe airway conditions should reveal different results. It is hoped that this part of the study can be further explored in the upcoming research. In addition, there is little consensus on how to define subtypes of emphysema with different lobe-predominant emphysema subtypes, and clinical and genetic features associated with these subtypes are currently lacking (24). Therefore, further studies are needed regarding the quantitative analysis of CT at the lobar level in patients with COPD.
Conclusions
The percentage of emphysema did not differ between lobes in the non-COPD control and severe to extremely severe COPD populations, whereas it was higher in the upper lobes than in the remaining lobes in the mildly to moderately severe COPD population, which suggests that differences in percentage of emphysema volume in lobe distribution decrease or disappear as COPD worsens. Using ∆LV/LVin as the lung volume criterion is more effective than LVin and LVex to judge different grades of COPD. LVin and the percentage of emphysema volume in the left upper lobe and LVex and ∆LV/LVin in the right lower lobe better reflect the changes of the patients’ lung function indexes. Therefore, we should pay more attention to the changes of quantitative CT parameters in the lower lobes of both lungs in patients with COPD.
Acknowledgments
Funding: This work was supported by
Footnote
Reporting Checklist: The authors have completed the STROBE reporting checklist. Available at https://qims.amegroups.com/article/view/10.21037/qims-23-1496/rc
Conflicts of Interest: All authors have completed the ICMJE uniform disclosure form (available at https://qims.amegroups.com/article/view/10.21037/qims-23-1496/coif). The authors have no conflicts of interest to declare.
Ethical Statement: The authors are accountable for all aspects of the work in ensuring that questions related to the accuracy or integrity of any part of the work are appropriately investigated and resolved. The study was conducted in accordance with the Declaration of Helsinki (as revised in 2013). The study was approved by the Ethics Committee of The First Affiliated Hospital of Guangzhou Medical University (No. 2018-53). Prior to this study, all participants were given information about radiation exposure of CT and provided written informed consent.
Open Access Statement: This is an Open Access article distributed in accordance with the Creative Commons Attribution-NonCommercial-NoDerivs 4.0 International License (CC BY-NC-ND 4.0), which permits the non-commercial replication and distribution of the article with the strict proviso that no changes or edits are made and the original work is properly cited (including links to both the formal publication through the relevant DOI and the license). See: https://creativecommons.org/licenses/by-nc-nd/4.0/.
References
- Rodríguez García C, Ruano-Ravina A, Pérez Ríos M, Martín Gisbert L, Varela-Lema L, Candal-Pedreira C, Represas-Represas C, Rey-Brandariz J, Valdés-Cuadrado L, Agustí A. Clinical characteristics of chronic obstructive pulmonary disease in never-smokers: A systematic review. Respir Med 2023;214:107284. [Crossref] [PubMed]
- Upadhyay P, Wu CW, Pham A, Zeki AA, Royer CM, Kodavanti UP, Takeuchi M, Bayram H, Pinkerton KE. Animal models and mechanisms of tobacco smoke-induced chronic obstructive pulmonary disease (COPD). J Toxicol Environ Health B Crit Rev 2023;26:275-305. [Crossref] [PubMed]
- Nambu A, Zach J, Schroeder J, Jin G, Kim SS, Kim YI, Schnell C, Bowler R, Lynch DA. Quantitative computed tomography measurements to evaluate airway disease in chronic obstructive pulmonary disease: Relationship to physiological measurements, clinical index and visual assessment of airway disease. Eur J Radiol 2016;85:2144-51. [Crossref] [PubMed]
- Han MK, Kazerooni EA, Lynch DA, Liu LX, Murray S, Curtis JL, Criner GJ, Kim V, Bowler RP, Hanania NA, Anzueto AR, Make BJ, Hokanson JE, Crapo JD, Silverman EK, Martinez FJ, Washko GR. COPDGene Investigators. Chronic obstructive pulmonary disease exacerbations in the COPDGene study: associated radiologic phenotypes. Radiology 2011;261:274-82. [Crossref] [PubMed]
- Bakker JT, Klooster K, Vliegenthart R, Slebos DJ. Measuring pulmonary function in COPD using quantitative chest computed tomography analysis. Eur Respir Rev 2021;30:210031. [Crossref] [PubMed]
- Pistenmaa CL, Nardelli P, Ash SY, Come CE, Diaz AA, Rahaghi FN, Barr RG, Young KA, Kinney GL, Simmons JP, Wade RC, Wells JM, Hokanson JE, Washko GR, San José Estépar R. COPDGene Investigators. Pulmonary Arterial Pruning and Longitudinal Change in Percent Emphysema and Lung Function: The Genetic Epidemiology of COPD Study. Chest 2021;160:470-80. [Crossref] [PubMed]
- Kirby M, Tanabe N, Tan WC, Zhou G, Obeidat M, Hague CJ, Leipsic J, Bourbeau J, Sin DD, Hogg JC, Coxson HO. CanCOLD Collaborative Research Group; Canadian Respiratory Research Network; CanCOLD Collaborative Research Group, the Canadian Respiratory Research Network. Total Airway Count on Computed Tomography and the Risk of Chronic Obstructive Pulmonary Disease Progression. Findings from a Population-based Study. Am J Respir Crit Care Med 2018;197:56-65. [Crossref] [PubMed]
- Nambu A, Zach J, Kim SS, Jin G, Schroeder J, Kim YI, Bowler R, Lynch DA. Significance of Low-Attenuation Cluster Analysis on Quantitative CT in the Evaluation of Chronic Obstructive Pulmonary Disease. Korean J Radiol 2018;19:139-46. [Crossref] [PubMed]
- Zach JA, Newell JD Jr, Schroeder J, Murphy JR, Curran-Everett D, Hoffman EA, Westgate PM, Han MK, Silverman EK, Crapo JD, Lynch DA. COPDGene Investigators. Quantitative computed tomography of the lungs and airways in healthy nonsmoking adults. Invest Radiol 2012;47:596-602. [Crossref] [PubMed]
- Boueiz A, Chang Y, Cho MH, Washko GR, San José Estépar R, Bowler RP, Crapo JD, DeMeo DL, Dy JG, Silverman EK, Castaldi PJ. COPDGene Investigators. Lobar Emphysema Distribution Is Associated With 5-Year Radiological Disease Progression. Chest 2018;153:65-76. [Crossref] [PubMed]
- Winantea J, Darwiche K. Bronchoscopic lung volume reduction. Pneumologie 2023;77:239-49. [PubMed]
- Valdivia D, Langehegermann L, Aigner C. Lobectomy as Lung Volume Reduction Surgery in a Patient With Previous Contralateral Bilobectomy. Ann Thorac Surg 2021;112:e261-4. [Crossref] [PubMed]
- Wanger J, Clausen JL, Coates A, Pedersen OF, Brusasco V, Burgos F, Casaburi R, Crapo R, Enright P, van der Grinten CP, Gustafsson P, Hankinson J, Jensen R, Johnson D, Macintyre N, McKay R, Miller MR, Navajas D, Pellegrino R, Viegi G. Standardisation of the measurement of lung volumes. Eur Respir J 2005;26:511-22. [Crossref] [PubMed]
- Tantucci C, Bottone D, Borghesi A, Guerini M, Quadri F, Pini L. Methods for Measuring Lung Volumes: Is There a Better One? Respiration 2016;91:273-80. [Crossref] [PubMed]
- Chen H, Chen RC, Guan YB, Li W, Liu Q, Zeng QS. Correlation of pulmonary function indexes determined by low-dose MDCT with spirometric pulmonary function tests in patients with chronic obstructive pulmonary disease. AJR Am J Roentgenol 2014;202:711-8. [Crossref] [PubMed]
- Song L, Leppig JA, Hubner RH, Lassen-Schmidt BC, Neumann K, Theilig DC, Feldhaus FW, Fahlenkamp UL, Hamm B, Song W, Jin Z, Doellinger F, Quantitative CT. Analysis in Patients with Pulmonary Emphysema: Do Calculated Differences Between Full Inspiration and Expiration Correlate with Lung Function? Int J Chron Obstruct Pulmon Dis 2020;15:1877-86. [Crossref] [PubMed]
- Gawlitza J, Sturm T, Spohrer K, Henzler T, Akin I, Schönberg S, Borggrefe M, Haubenreisser H, Trinkmann F. Predicting Pulmonary Function Testing from Quantified Computed Tomography Using Machine Learning Algorithms in Patients with COPD. Diagnostics (Basel) 2019;9:33. [Crossref] [PubMed]
- Come CE, Diaz AA, Curran-Everett D, Muralidhar N, Hersh CP, Zach JA, Schroeder J, Lynch DA, Celli B, Washko GR. COPDGene Investigators. Characterizing functional lung heterogeneity in COPD using reference equations for CT scan-measured lobar volumes. Chest 2013;143:1607-17. [Crossref] [PubMed]
- Schroeder JD, McKenzie AS, Zach JA, Wilson CG, Curran-Everett D, Stinson DS, Newell JD Jr, Lynch DA. Relationships between airflow obstruction and quantitative CT measurements of emphysema, air trapping, and airways in subjects with and without chronic obstructive pulmonary disease. AJR Am J Roentgenol 2013;201:W460-70. [Crossref] [PubMed]
- Prisk GK. Gas exchange under altered gravitational stress. Compr Physiol 2011;1:339-55. [Crossref] [PubMed]
- Qi S, Zhang B, Yue Y, Shen J, Teng Y, Qian W, Wu J. Airflow in Tracheobronchial Tree of Subjects with Tracheal Bronchus Simulated Using CT Image Based Models and CFD Method. J Med Syst 2018;42:65. [Crossref] [PubMed]
- de-Torres JP, O'Donnell DE, Marín JM, Cabrera C, Casanova C, Marín M, Ezponda A, Cosio BG, Martinez C, Solanes I, Fuster A, Neder JA, Gonzalez-Gutierrez J, Celli BR. Clinical and Prognostic Impact of Low Diffusing Capacity for Carbon Monoxide Values in Patients With Global Initiative for Obstructive Lung Disease I COPD. Chest 2021;160:872-8. [Crossref] [PubMed]
- Xie X, Dijkstra AE, Vonk JM, Oudkerk M, Vliegenthart R, Groen HJ. Chronic respiratory symptoms associated with airway wall thickening measured by thin-slice low-dose CT. AJR Am J Roentgenol 2014;203:W383-90. [Crossref] [PubMed]
- Castaldi PJ, Xu Z, Young KA, Hokanson JE, Lynch DA, Humphries SM, Ross JC, Cho MH, Hersh CP, Crapo JD, Strand M, Silverman EK. Heterogeneity and Progression of Chronic Obstructive Pulmonary Disease: Emphysema-Predominant and Non-Emphysema-Predominant Disease. Am J Epidemiol 2023;192:1647-58. [Crossref] [PubMed]