Diastolic dysfunction in adults with uncomplicated obesity evaluated with left atrial and left ventricular tissue tracking and ventricular volume-time curve: a prospective cardiac magnetic resonance study
Introduction
Obesity increases the risk of heart failure (HF), especially HF with preserved ejection fraction (HFpEF) (1,2). Recently, studies have proposed obesity-related HFpEF as a distinct phenotype of HF, especially in people with obesity-related comorbidities such as diabetes (3,4). However, it is unclear whether obese patients without comorbidities are prone to develop HFpEF.
Diastolic dysfunction plays a critical role in the pathophysiology of HFpEF (5,6). Diastolic dysfunction has traditionally been assessed by invasive cardiac catheter or non-invasive methods using echocardiology (5). Notwithstanding, the presence of excessive fat deposition in obesity causes signal interference, making it difficult for echocardiography to measure cardiac function and structure. Thus, a sensitive, reliable, and non-invasive tool for the early detection of diastolic dysfunction is needed. Currently, the tissue tracking can detect early myocardial dysfunction through measuring cardiac mechanics during myocardial motion (7). A prior study comparing myocardial deformation measurement with cardiac magnetic resonance (CMR) and echocardiography revealed that echocardiographic speckle tracking technique relied heavily on image quality. However, some myocardial segments may be inadequately visualized, and image quality is reduced in the far-end of the ultrasound sector. Meanwhile, the signal-to-noise ratio of echocardiography is lower than that of CMR (8). Additionally, left ventricular (LV) volume-time curve parameters on CMR with high sensitivity and specificity have been used as markers to evaluate diastolic function (9).
Besides, recent studies have also found that left atrial (LA) longitudinal strain is strongly associated with LV end-diastolic pressure and filling pressures, which are invasive gold standards for assessing LV diastolic function (10,11). LA functional parameters include reservoir (LA filling during ventricle systole), conduit (blood from the LA and pulmonary veins to the LV during early diastole), and booster function (atrial contraction during late diastole), which correspond to all stages of LV systole and diastole and may be good indicators of diastolic dysfunction. The effects of uncomplicated obesity on LA strain have not been well clarified.
Previous studies have demonstrated that fat deposition of different regions (such as visceral or subcutaneous adipose tissue) had distinct effects on heart. Dual X-ray absorptiometry (DXA) further divides body fat, such as fat deposits in arms and legs, trunk, android, gynoid, or visceral regions (12). These fat tissues have been linked to cardiovascular risk factors (13-15); however, the effect of fat distribution in these regional areas on left heart function is not clear yet.
Thus, the purpose of this study was to assess the impact of uncomplicated obesity on diastolic function by measuring LA and LV strain and volume-time curve derived from CMR and evaluate the relationship of diastolic function with body fat distribution. We present this article in accordance with the STROBE reporting checklist (available at https://qims.amegroups.com/article/view/10.21037/qims-23-1785/rc).
Methods
Study population
The study was conducted in accordance with the Declaration of Helsinki (as revised in 2013). The study was approved by the Institutional Review Board of West China Hospital of Sichuan University (No. 2019-321) and informed consent was provided by all participants. We prospectively recruited obese patients [body mass index (BMI): 27.5–37.5 kg/m2] and healthy volunteers (BMI: 18.5–23.0 kg/m2) from September 2019 to June 2022 (age ranged from 18 to 60 years). The exclusion criteria were as follows: hypertension [systolic/diastolic blood pressure (SBP/DBP) ≥140/90 mmHg] and diabetes diagnosed based on oral glucose tolerance; history of use of lipid-lowering, hypoglycemic, or antihypertensive drugs; history of cardiovascular diseases or any cardiovascular procedures; major systemic diseases and respiratory diseases that may affect the heart, such as connective tissue disease, endocrine disease, or chronic obstructive pulmonary emphysema; renal failure; and contraindication to magnetic resonance imaging. The power calculation was conducted using “t-tests” of G* power (Version 3.1.9.6). The parameters were set as follows: tails =2, α=0.05, effect size =0.6 (calculated based on mean and standard deviation of LV strain in the 2 groups of samples in the pre-experiment), power (1-β) =0.8, allocation rate N2/N1 =1. Finally, the sample size of group 1 was 45, that of group 2 was 45, constituting a total sample size of 90. In this study, 92 participants (49 uncomplicated obese participants and 43 healthy controls) were enrolled. Due to severe motion artifacts in LA caused by blood flow of pulmonary veins, LA strain data from 9 obese participants had to be excluded. A flow chart of this study is presented in Figure 1.
Baseline characteristic assessment
The participants underwent an extensive baseline characteristic assessment of clinical history, anthropometric measurements of body height and weight, heart rate, SBP, DBP, mean arterial pressure (MAP); laboratory tests including oral glucose tolerance, fasting insulin, lipid profiles such as total cholesterol, triglycerides, high-/low-density lipoprotein (HDL/LDL). MAP was calculated as follows: MAP = DBP + 1/3 (SBP − DBP) (16). The homeostasis model assessment of insulin resistance (HOMA-IR) was calculated according to the following formula: HOMA-IR = [fasting blood glucose (mmol/L) × fasting insulin (mU/L)]/22.5 (17).
Assessment of body fat distribution
Waist circumference (WC) and hip circumference (HC) were measured at the midpoint between the lowest point of the rib and the iliac crest and at the largest diameter of the hip when standing with calculating waist-to-hip ratio. BMI (kg/m2) was obtained by dividing weight (kg) by the square of height (m2). BMI was classified into the 3 groups based on Chinese criteria: normal weight (23.0> BMI ≥18.5 kg/m2), overweight (27.5> BMI ≥23.0 kg/m2), and obese (≥27.5 kg/m2) (18). Body fat distribution was assessed by DXA (Lunar iDXA, GE Medical Systems Lunar, Madison, WI, USA). The specific region of interest of fat deposit regions was displayed in a previous study (12). The percentage of fat mass in visceral, trunk, upper and lower extremities, android, and gynoid areas was calculated by dividing fat mass in the corresponding regions by total fat mass. The percentage of fat mass in peripheral area was calculated as the sum of the percentage of fat mass in the extremities. The percentage of fat mass in visceral (visceral fat%), trunk (trunk fat%), and android (android fat%) regions were taken as indicators of central fat deposits. In contrast, percentage of fat mass in gynoid (gynoid fat%), peripheral (peripheral fat%), upper extremities (upper extremities fat%), and lower extremities (lower extremities fat%) regions were taken as indicators of peripheral fat deposits.
CMR examination and sequences
CMR scans were carried out with an 18-element surface coil on 3.0 T whole-body scanner (MAGNETOM Skyra; Siemens Medical Solutions, Erlangen, Germany) with the patients in the supine position. Data were obtained during end-expiratory breath holds using a standard electrocardiogram (ECG) trigger device. A balanced steady-state free precession (bSSFP) sequence was applied to obtain continuous short-axis cine images, as well as long-axis 2- and 4-chamber cine images. The scan parameters of bSSFP were as follows: temporal resolution of 39.34 ms, repetition time/echo time of 3.3/1.22 ms, slice thickness of 8 mm, flip angle of 41°, field of view of 360×320 mm2, and matrix size of 208×166.
Image analysis
CMR image analysis was performed with commercial software (CVI 42 version 5.11.3; Circle Cardiovascular Imaging Inc., Calgary, Canada). Image measurement and analysis was completed by 2 experienced radiologists (J. Liu with 5 years and L.P. with 8 years of CMR experience) who were unaware of participant status.
LV global geometric and systolic functional parameters and time-volume curve
The borders of LV endocardium and epicardium were drawn at the end-diastole and end-systole in short-axis cine images. The LV global systolic function and geometric parameters, namely, LV ejection fraction (LVEF), end-diastolic/systolic volume (EDV/ESV), cardiac output, and mass at end-diastole, were obtained. LV mass was indexed to height2.7 (g/m2.7) (19). All endocardial contours of the LV short-axis cine images were manually delineated on 25 phases within a cardiac cycle. The LV time-volume curve parameters were automatically calculated, including the peak ejection rate (PER) and peak filling rate (PFR). PER and PFR are often indexed to EDV [peak ejection rate index (PERI) and peak filling rate index (PFRI)] due to strong correlation between PER and PFR and LVEDV (20).
LV and LA strain
The short-axis and long-axis (2-chamber and 4-chamber) cine slices were used for LV strain evaluation. At the LV end-diastole, the borders of LV endocardium and epicardium were manually outlined (excluding the papillary muscles and moderator bands). The LV global myocardial strain rates were automatically acquired, including the radial, circumferential, and longitudinal peak diastolic strain rate (PDSR) and peak systolic strain rates (PSSR). Long-axis 2-chamber and 4-chamber were used for analysis of LA myocardial strain. At ventricular end-diastole phase, endocardial and epicardial borders of LA were manually delineated. LA global longitudinal peak strain parameters were acquired from curve, including reservoir function [total strain (εs) and peak positive strain rate (SRs)], conduit function [passive strain (εe) and peak early negative strain rate (SRe)], and booster pump function [active strain (εa) and peak late negative strain rate (SRa)] (Figure 2).
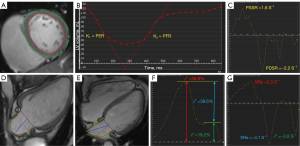
LA volume (LAV)
LAV was assessed at LV end-systole (LAV max), at LV diastole before LA contraction (LAV pre-a), and at LV end-diastole (LAV min) in function biplanar long-axis.
Epicardial adipose tissue (EAT) quantification
EAT represents the region of high signal intensity between the myo-epicardium and pericardium. The specific measurement methods have been elucidated in the previous article (21).
Reproducibility
Intra-/inter-observer variabilities of the LA and LV measurements were assessed in 30 random cases (20 obese cases and 10 healthy controls). To evaluate intra-observer variability, the same images were evaluated by the same observer at a 1-month interval. Besides, in order to assess the inter-observer variability, the other observer, who was blinded to the results from first observer, re-measured and analyzed images.
Statistical analysis
The software SPSS 23.0 (IBM Corp., Armonk, NY, USA) was used for all statistical analysis. Normally distributed continuous data were presented as the means ± standard deviation, whereas non-normally distributed data were presented as the median (25th, 75th percentiles). Continuous variables between the 2 groups were compared using Student’s t-test or Mann-Whitney U test. In addition, categorical data were expressed as numbers (percentages) and compared by the chi-square test. Pearson’s correlation coefficient was applied to analyze the relationship between LA and LV function, and to investigate the association between diastolic function and body fat distribution in obese patients. The strength of the correlation for the absolute value of r was as follows: 0.00–0.10= negligible; 0.10–0.39= weak; 0.40–0.69= moderate; 0.70–0.89= strong; 0.90-1.00= very strong (22). The association between regional fat distribution and diastolic function parameters was analyzed by stepwise multivariable linear regression. Related fat indexes, including WC, waist-to-hip ratio, and waist-to-height ratio, visceral fat%, trunk fat%, android fat%, peripheral fat%, gynoid fat%, and EAT were included in univariable analyses. Variables with a P<0.1 in the univariable analysis were then entered in a multivariable linear regression analysis. Additionally, to investigate the association between obesity and diastolic function while controlling for variations in growth and blood pressure, age, sex, and MAP were added in multivariable analysis. The inter-/intra-observer variability was assessed using intraclass correlation coefficient (ICC). According to the 95% confidence intervals (95% CI) of the ICC estimate, less than 0.5, 0.5–0.75, 0.75–0.9, and greater than 0.90 indicated poor, moderate, good, and excellent confidence, respectively (23). Statistical significance was defined as P<0.05 (2-sided). When the myocardial strain parameters of LA and LV were negative, the absolute values were taken for the statistical analyses.
Results
Baseline characteristics
Of 110 participants assessed for eligibility, according to the screening criteria, 94 adults were recruited for this study. Among them, 2 participants were unable to perform enhanced CMR because of concern for contrast medium side effect. Finally, 49 obese patients (age 32.6±8.8 years, 55.1% males, BMI: 29.9±2.0 kg/m2) and 43 healthy controls (age 29.7±7.3 years, 58.1% males, BMI: 20.2±1.6 kg/m2) were included in the analysis. Due to severe motion artifacts in LA caused by blood flow of pulmonary veins, LA data from 9 obese participants had to be excluded. Baseline assessment of the participants is presented in Table 1. Mean age, sex, body height, and heart rate were not statistically significant between the 2 groups (P>0.05). Although in the normal range, MAP in obesity was increased compared with healthy controls (93.8±6.6 vs. 83.9±9.2 mmHg, P<0.001). For lab tests, compared with the healthy group, the obese group had higher fasting blood glucose, triglycerides, total cholesterol, LDL, fasting insulin, HOMA-IR, and lower HDL (all P<0.001). The obese patients had greater WC, HC, waist-to-hip ratio, and waist-to-height ratio than normal controls (all P<0.001). Furthermore, after analyzing the body fat distribution on DXA, we observed that the obese participants had higher central fat deposits, including visceral fat%, trunk fat%, and android fat% than healthy controls (4.2% vs. 2.2%, 57.7%±4.7% vs. 46.0%±4.9%, 9.8%±1.5% vs. 5.9%±1.1%, respectively; all P<0.001); by contrast, the obese group had lower peripheral fat deposits, containing peripheral fat%, gynoid fat%, and lower extremities fat% than healthy group (38.5%±5.0% vs. 45.7%±5.3%, 14.9%±2.0% vs. 17.6%±2.8%, 28.0%±4.2% vs. 35.1%±4.9%, respectively; all P<0.001). There was a higher EAT volume among the obese patients than among healthy individuals (46.2 vs. 19.7 cm3, P<0.001).
Table 1
Variables | Obese patients (n=49) | Controls (n=43) | P value |
---|---|---|---|
Demographics | |||
Male | 27 (55.1) | 25 (58.1) | 0.769 |
Age (years) | 32.6±8.8 | 29.7±7.3 | 0.090 |
Height (cm) | 167.6±9.4 | 166.6±8.3 | 0.604 |
Weight (kg) | 84±11 | 56.2±8.1 | <0.001 |
BMI (kg/m2) | 29.9±2.0 | 20.2±1.6 | <0.001 |
Hemodynamic variables | |||
Heart rate (bpm) | 73.7±9.6 | 74.2±8.0 | 0.818 |
SBP (mmHg) | 124±10 | 109±12 | <0.001 |
DBP (mmHg) | 78.9±6.5 | 71.6±8.8 | <0.001 |
MAP (mmHg) | 93.8±6.6 | 83.9±9.2 | <0.001 |
Laboratory data | |||
Fasting blood glucose (mmol/L) | 5.4±0.6 | 4.8±0.3 | <0.001 |
Total cholesterol (mmol/L) | 4.9±1.1 | 4.0±0.7 | <0.001 |
Plasma triglycerides (mmol/L) | 1.6 (1.0, 2.7) | 0.5 (0.4, 0.7) | <0.001 |
HDL (mmol/L) | 1.3±0.3 | 1.6±0.4 | <0.001 |
LDL (mmol/L) | 2.7±0.8 | 2.1±0.5 | <0.001 |
Fasting insulin (mmol/L) | 13.8 (10.9, 20.4) | 5.9 (3.7, 7.7) | <0.001 |
HOMA-IR | 3.4 (2.7, 4.8) | 1.2 (0.8, 1.6) | <0.001 |
Adiposity measures | |||
EAT (cm3) | 46.2 (37.7, 56.3) | 19.7 (14.2, 23.1) | <0.001 |
Trunk fat, % | 57.7±4.7 | 46.0±4.9 | <0.001 |
Peripheral fat, % | 38.5±5.0 | 45.7±5.3 | <0.001 |
Upper extremities fat, % | 10.5±1.5 | 10.7±1.1 | 0.557 |
Lower extremities fat, % | 28.0±4.2 | 35.1±4.9 | <0.001 |
Android fat, % | 9.8±1.5 | 5.9±1.1 | <0.001 |
Gynoid fat, % | 14.9±2.0 | 17.6±2.8 | <0.001 |
Visceral fat, % | 4.2 (3.3, 5.9) | 2.2 (0.9, 3.3) | <0.001 |
Waist circumference (cm) | 100±11 | 73.4±5.2 | <0.001 |
Hip circumference (cm) | 107.3±4.1 | 92.6±4.2 | <0.001 |
Waist-to-hip ratio | 0.93±0.09 | 0.79±0.05 | <0.001 |
Waist-to-height ratio | 0.6±0.06 | 0.44±0.04 | <0.001 |
Data are expressed as n (%) or mean ± standard deviation or median (25th, 75th percentiles) appropriately. BMI, body mass index; SBP, systolic blood pressure; DBP, diastolic blood pressure; MAP, mean artery pressure; HDL, high-density lipoprotein; LDL, low-density lipoprotein; HOMA-IR, homeostasis model assessment of insulin resistance; EAT, epicardial adipose tissue.
Comparison of CMR parameters of LA and LV between the obese group and healthy control group
Compared to the controls, obese individuals had greater LA volume in 3 phases. For LA strain analysis, obese participants had lower conduit [εe and SRe (30.8%±8.0% vs. 35.5%±9.8%, P=0.019; −3.1±0.8 vs. −3.5±1.0 s−1, P=0.030, respectively)] and reservoir function [εs and SRs (46.4%±8.4% vs. 51%±12%, P=0.045; 1.9±0.5 vs. 2.3±0.5 s−1, P<0.001, respectively)], and preserved booster pump function [εa and SRa (15.6%±2.8% vs. 15.5%±3.8%, P=0.842; −2.1±0.4 vs. −2.0±0.5 s−1, P=0.697, respectively)] compared with those of controls.
LVEF was within normal range (LVEF >50%) in each of the obese individuals and was not different from that in the control group. In contrast, obese individuals exhibited higher LV geometric parameters (LVEDV, LVESV, LV mass, and LV mass index) compared with the control group (158±28 vs. 128±20 mL, P<0.001; 59±13 vs. 51±10 mL, P=0.001; 91±20 vs. 75±17 g, P<0.001; 22.3±3.5 vs. 19.0±3.7 g/m2.7, P<0.001, respectively). Through the LV strain analysis, the obese cases had reduced global circumferential and longitudinal PDSR as well as preserved radial PDSR compared with the controls (1.3±0.2 vs. 1.5±0.3 s−1, P=0.014; 0.8±0.2 vs. 1.1±0.2 s−1, P<0.001; −2.6±0.6 vs. −2.8±0.7 s−1, P=0.097, respectively). For LV time-volume curve, PFRI declined but PERI preserved in obese participants compared to the healthy controls (3.5±0.6 vs. 3.9±0.7 s−1, P=0.012; 3.7±0.6 vs. 3.8±0.6 s−1, P=0.412, respectively) (Table 2, Figure 3).
Table 2
Variables | Obese patients (n=49) | Controls (n=43) | P value |
---|---|---|---|
LA volume | |||
LAVmax (mL) | 64±12 | 54±12 | <0.001 |
LAVpre-a (mL) | 38.2±8.3 | 30.4±9.3 | <0.001 |
LAVmin (mL) | 21.7±5.5 | 18.7±5.9 | 0.022 |
LA reservoir function | |||
εs (%) | 46.4±8.4 | 51±12 | 0.045 |
SRs (s−1) | 1.9±0.5 | 2.3±0.5 | <0.001 |
LA conduit function | |||
εe (%) | 30.8±8.0 | 35.5±9.8 | 0.019 |
SRe (s−1) | −3.1±0.8 | −3.5±1.0 | 0.030 |
LA booster pump function | |||
εa (%) | 15.6±2.8 | 15.5±3.8 | 0.842 |
SRa (s−1) | −2.1±0.4 | −2.0±0.5 | 0.697 |
LV global geometric and functional parameters | |||
LVEF (%) | 62.7±4.6 | 60.6±5.0 | 0.120 |
LVEDV (mL) | 158±28 | 128±20 | <0.001 |
LVESV (mL) | 59±13 | 51±10 | 0.001 |
LV mass (g) | 91±20 | 75±17 | <0.001 |
LV mass index (g/m2.7) | 22.3±3.5 | 19.0±3.7 | <0.001 |
LV strain rate parameters | |||
Radial PDSR (s−1) | −2.6±0.6 | −2.8±0.7 | 0.097 |
Circumferential PDSR (s−1) | 1.3±0.2 | 1.5±0.3 | 0.014 |
Longitudinal PDSR (s−1) | 0.8±0.2 | 1.1±0.2 | <0.001 |
Radial PSSR (s−1) | 1.9±0.4 | 2.0±0.5 | 0.100 |
Circumferential PSSR (s−1) | −1.0±0.3 | −1.1±0.2 | 0.088 |
Longitudinal PSSR (s−1) | −0.8±0.3 | −0.8±0.2 | 0.072 |
LV volume-time curve parameters | |||
PFRI (s−1) | 3.5±0.6 | 3.9±0.7 | 0.012 |
PERI (s−1) | 3.7±0.6 | 3.8±0.6 | 0.412 |
Data are given as mean ± standard deviation. LA, left atrial; LV, left ventricular; Vmax, maximum volume; Vpre-a, pre-atrial contraction volume; Vmin, minimum volume; εs, total strain; SRs, peak positive strain rate; εe, passive strain; SRe, peak early negative strain rate; εa, active strain; SRa, peak late negative strain rate; EF, ejection fraction; EDV, end diastolic volume; ESV, end systolic volume; PDSR, peak diastolic strain rate; PSSR, peak systolic strain rate; PFRI, peak filling rate index; PERI, peak ejection rate index.
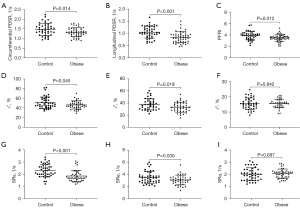
Correlation between LA functional parameters and LV diastolic function parameters in the obese group
LA εs was mild-to-moderately correlated with LV radial and circumferential PDSR and PFRI (r=0.485, 0.359, and 0.392, respectively). LA SRs was mild-to-moderately related with LV radial and longitudinal PDSR (r=0.444 and 0.312, respectively). Additionally, LA εe and SRe were moderately associated with LV radial and circumferential PDSR and PFRI (r=0.557, 0.478, and 0.420 for εe and r=0.516, 0.564, and 0.416 for SRe, respectively). However, LA booster pump function (εa and SRa) had no obvious linear relationships with LV function. Furthermore, LV PFRI was mild-to-moderately associated with LV radial, circumferential, and longitudinal PDSR (r=0.462, 0.476, and 0.379, respectively) (Table 3).
Table 3
Parameters | Radial PDSR | Circumferential PDSR | Longitudinal PDSR | PFRI |
---|---|---|---|---|
Reservoir function | ||||
εs (%) | 0.485** | 0.359* | 0.152 | 0.392* |
SRs (s−1) | 0.444** | 0.261 | 0.312* | 0.306 |
Conduit function | ||||
εe (%) | 0.557** | 0.478** | 0.278 | 0.420** |
SRe (s−1) | 0.516** | 0.564** | 0.258 | 0.416** |
Booster pump function | ||||
εa (%) | −0.128 | −0.279 | −0.232 | −0.018 |
SRa (s−1) | −0.136 | −0.139 | −0.079 | 0.059 |
PFRI | 0.462** | 0.476** | 0.379** | 1** |
*, P<0.05; **, P<0.01. LA, left atrial; LV, left ventricular; PDSR, peak diastolic strain rate; εs, total strain; SRs, peak positive strain rate; εe, passive strain; SRe, peak early negative strain rate; εa, active strain; SRa, peak late negative strain rate; PFRI, peak filling rate index.
Correlation between regional fat distribution and LV diastolic function parameters in the obese group
Univariable analysis indicated that radial PDSR was inversely correlated with trunk fat% (r=−0.289), yet positively correlated with peripheral fat% (r=0.302). LV circumferential and longitudinal PDSR and LA εs and εe were negatively associated with android fat% (r=−0.341, −0.459, −0.312, and −0.453, respectively), trunk fat% (r=−0.418, −0.489, −0.368, and −0.520, respectively), and visceral fat% (r=−0.370, −0.518, −0.270, and −0.403, respectively), yet positively associated with peripheral fat% (r=0.383, 0.496, 0.357, and 0.502, respectively) and gynoid fat% (r=0.336, 0.388, 0.384, and 0.484, respectively). PFRI was positively related with gynoid fat% (r=0.286) (Table 4, Figure 4).
Table 4
Parameters | PDSR | PFRI | εs | εe | ||
---|---|---|---|---|---|---|
Radial | Circumferential | Longitudinal | ||||
Trunk fat% | −0.289* | −0.418** | −0.489** | −0.201 | −0.368* | −0.520** |
Peripheral fat% | 0.302* | 0.383** | 0.496** | 0.219 | 0.357* | 0.502** |
Android fat% | −0.253 | −0.341* | −0.459** | −0.211 | −0.312* | −0.453** |
Gynoid fat% | 0.245 | 0.336* | 0.388** | 0.286* | 0.384* | 0.484** |
Visceral fat% | −0.236 | −0.370** | −0.518** | −0.256 | −0.270 | −0.403** |
EAT | −0.025 | −0.245 | −0.101 | 0.029 | −0.073 | −0.096 |
Waist circumference | 0.001 | −0.031 | −0.144 | −0.095 | −0.124 | −0.163 |
Waist-to-hip ratio | −0.033 | −0.072 | −0.169 | −0.103 | 0.024 | −0.025 |
Waist-to-height ratio | 0.104 | −0.053 | −0.045 | 0.004 | −0.098 | −0.218 |
*, P<0.05; **, P<0.01. PDSR, peak diastolic strain rate; PFRI, peak filling rate index; εs, total strain; εe, passive strain; EAT, epicardial adipose tissue.
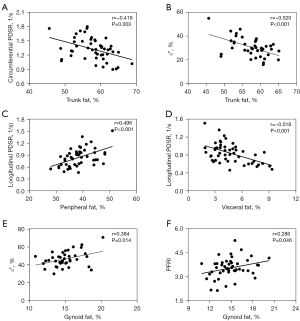
Multivariable analysis indicated that trunk fat% had independent relationships with LV circumferential PDSR (β=−0.418, P=0.003, model R2=0.174) and εe (β=−0.520, P<0.001, model R2=0.270); visceral fat% and peripheral fat% were independently associated with longitudinal PDSR (β=−0.342, P=0.038; β=0.376, P=0.024; model R2=0.389); gynoid fat% was independently associated with PFRI (β=0.286; P=0.046, model R2 = 0.081) and εs (β=0.384, P=0.014, model R2=0.148) (Table 5).
Table 5
Dependent variable | Independent variable | R2 | Adjusted R2 | B | β | P value |
---|---|---|---|---|---|---|
Circumferential PDSR | Trunk fat% | 0.174 | 0.156 | −0.021 | −0.418 | 0.003 |
Longitudinal PDSR | Age | 0.389 | 0.348 | 0.009 | 0.361 | 0.006 |
Peripheral fat% | 0.017 | 0.376 | 0.024 | |||
Visceral fat% | −0.030 | −0.342 | 0.038 | |||
PFRI | Gynoid fat% | 0.081 | 0.062 | 0.086 | 0.286 | 0.046 |
εs | Gynoid fat% | 0.148 | 0.125 | 1.644 | 0.384 | 0.014 |
εe | Trunk fat% | 0.270 | 0.251 | −0.913 | −0.520 | <0.001 |
PDSR, peak diastolic strain rate; PFRI, peak filling rate index; εs, total strain; εe, passive strain.
Association of metabolic-related cardiovascular risk factors with regional fat deposition in obese patients
Triglycerides was negatively associated with peripheral fat% and gynoid fat% (r=−0.542 and −0.439), yet positively associated with trunk fat%, android fat%, and visceral fat% (r=0.576, 0.509, and 0.512, respectively). On the contrary, HDL was negatively associated with trunk fat%, android fat%, and visceral fat% (r=−0.461, −0.427, and −0.484, respectively), yet positively associated with peripheral fat% and gynoid fat% (r=0.478 and 0.457). MAP was negatively associated with peripheral fat% (r=−0.307), yet positively associated with trunk fat% and visceral fat% (r=0.312 and 0.338) (Figure 5).
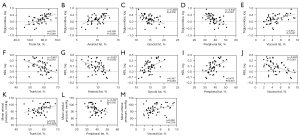
Reproducibility
The intra- and inter-observer agreements for LV strain rates (ICC =0.750–0.912 and 0.769–0.903, respectively), LV volume-time curve (ICC =0.893–0.978 and 0.886–0.949, respectively), and LA function parameters (ICC =0.804–0.936 and 0.812–0.924, respectively).
Discussion
This study compared cardiac diastolic function indices between obese adults with no comorbidities and normal controls based on CMR-derived tissue tracking and volume-time curve; and explored associations between diastolic function with body fat distribution in obese adults. The main results were as follows: (I) obese individuals had decreased LV global circumferential and longitudinal PDSR and PFRI as well as preserved LVEF, LV PSSR, and PERI compared with healthy controls; (II) obese individuals had impaired LA reservoir and conduit functions and preserved booster function compared with healthy controls; (III) LV diastolic indicators (PDSR and PFRI) had linear connections with LA reservoir and conduit function; (IV) central obesity had a negative correlation whereas peripheral obesity had a positive correlation with diastolic function in obesity.
Obesity and diastolic dysfunction
Consistent with previous studies using CMR (24,25), our study showed no difference in LVEF between the obese and control group, which indicates absence of LV global systolic functional impairment in obese individuals without complications. However, myocardial strain parameters can detect early myocardial dysfunction and have been shown to be better predictors of cardiovascular events than LVEF (26). Furthermore, previous studies have also indicated that CMR time-volume curve was a sensitive tool with good repeatability for detecting early dysfunction in diabetic patients (9,27). Thus, we combined these 2 approaches to assess the effects of uncomplicated obesity on cardiac function.
A previous study using 1.5T CMR-tissue tracking revealed subclinical LV diastolic dysfunction in uncomplicated obese adults, whereas indices of subclinical systolic dysfunction were not investigated (25). Therefore, it was unclear whether obese adults had concurrent subclinical systolic dysfunction in that study. Our study found that there was no subclinical systolic dysfunction in obese participants since the differences in LV PSSR and PERI between the 2 groups were not statistically significant. In contrast, impaired subclinical LV diastolic function was found in obese participants, as shown by reduced LV global circumferential and longitudinal PDSR and PFRI. Earlier echocardiographic studies have shown both systolic and diastolic dysfunction in severe obesity. In summary, these findings may suggest that patients with mild-to-moderate uncomplicated obesity initially develop impaired diastolic, rather than systolic, function.
Furthermore, we evaluated LA function to further understand diastolic function impairment in obesity. Compared with controls, obese patients had higher LA volume in our study. LA enlargement has previously been shown to be an indicator for LV diastolic dysfunction (6). However, for obese patients, it might be difficult to differentiate whether LA enlargement was due to compensatory LA remodeling (due to increased total blood volume and cardiac output for obesity) (28) or pathologic remodeling. A study showed that reservoir function was positively associated with cardiac output and negatively associated with LA stiffness (29). In our study, obese participants had reduced LA reservoir function despite higher cardiac output compared with controls, and this result aligned with those of earlier studies that enrolled obese individuals with or without obesity-related complications (30,31). Previous studies revealed that mean LA stiffness index was higher in obese individuals compared to controls (32,33). Additionally, in our study, obese patients had higher HOMA-IR than healthy controls. LA stiffness index was associated with insulin resistance in obesity (32). Thus, we speculate that impaired reservoir function in this study may be mainly due to LA pathologic remodeling. In addition, LA reservoir function is also affected by LV contraction, through the descent of the base during systole (34). In our study, LV systolic function parameters (LVEF, PSSR, and PERI) were not significantly different between the 2 groups, which suggests that LV contraction was not the main underlying factor for reservoir function deterioration in obese patients.
Our study also demonstrated that obese patients had decreased LA conduit function and preserved booster pump function compared with controls. The results are similar to a study that enrolled individuals with obesity and type 2 diabetes using CMR tissue tracking (31). Besides, another echocardiographic study also revealed same results in obese patients (some of them with complications) evaluated by longitudinal strain (30). Collectively, the above findings revealed that obese individuals had impaired LA reservoir and conduit function, regardless of obesity-related complications.
In addition, our study also showed linear correlations between LA reservoir and conduit dysfunction and impaired LV PDSR and PFRI in obese patients, which demonstrated that LA reservoir and conduit function might have the potential to indicate the degree of LV diastolic dysfunction. Previous literature indicated that LA reservoir and conduit function were impaired in all grades of diastolic dysfunction, whereas booster function was increased in mild diastolic dysfunction and reduced as diastolic dysfunction progressed (35,36). Since LV filling in early diastole (corresponding to conduit function) was reduced in mild diastolic dysfunction (35,37), in order to maintain cardiac output, the increase in LV filling in late diastole (caused by the contraction of LA) compensates for the decrease in LV filling in early diastole. The findings of LA function in our study may suggest mild diastolic dysfunction in mild-to-moderate uncomplicated obese patients.
Previous research has shown that impaired myocardial relaxation and stiffness were main factors affecting LV diastolic function, and the 2 factors were partly affected by ventricular hypertrophy and metabolic disorders (38). In our study, the obese group had greater LV mass after even normalized to hight2.7 than the control group. Compared with LV mass indexed to body surface area, LV mass indexed to height2.7 has been verified to be able to avoid underdiagnosis of obesity-related pathological LV hypertrophy (39). Our study also found more EAT in the obese group compared with the control group. An earlier study revealed that EAT might be a better predictor than BMI for diastolic dysfunction in obese men (25). Free fatty acids (FFA) released from EAT, combined with plasma FFA, can cause ventricular dysfunction through myocardial lipotoxicity caused by accumulation of myocardial lipids and fatty acid intermediates, such as ceramide (38,40). Additionally, a recent study suggested that an extrinsic heart compression and/or heart displacement exerted by EAT may result in reduced LV strain parameter (41). Furthermore, insulin resistance had a correlation with LV diastolic dysfunction in a previous study (21).
Regional fat distributions and LA and LV function
This study described the association between fat deposits and LA and LV function in obese individuals, and the results showed that visceral fat% and trunk fat% had inverse impacts, whereas peripheral fat% and gynoid fat% had positive impacts on LA and LV function in obesity.
The findings of this study may partly explain the different effects of fat deposits in different areas on LA and LV function. We found that visceral and trunk fat were positively associated with triglycerides and MAP, but inversely related with HDL in obesity. Differently, peripheral fat and gynoid fat had the opposite effects on these metabolic markers. Hyperlipidemia (increased triglycerides or reduced HDL) is a cardiometabolic risk factor which may promote apoptosis of cardiomyocytes and cardiac dysfunction (42). Central fat deposits were reported to be associated with the increased risk of hypertension, but peripheral fat deposits reduced this risk (13). Hypertension has been shown to be an important risk factor for LV diastolic dysfunction (43). In addition, a study indicated that compressive phenomena caused by abdominal obesity and thoracic adiposity may impair myocardial strain parameters and cardiac relaxation, in absence of any intrinsic myocardial dysfunction (41).
There were some limitations to the current study. First of all, this was a cross-sectional study. We could not establish causal relationships of obesity or body fat distribution with LA and LV strain. It is not known whether damaged myocardial deformation is restored with the reduction of fat deposits. Therefore, longitudinal studies are needed to explore the dynamic cardiac change in obese individuals and their potential factors for reversing these changes. Second, this was a monocentric study with limited sample size. Hence, selection bias may have affected the results. Our results need to be further confirmed in multi-center studies with larger samples. Third, our study reported the impact of regional fat deposits on LA and LV function in obesity, which was partially explained by the associations of fat deposits in different areas with compressive phenomena and metabolism-related cardiovascular risk factors. Nevertheless, earlier studies have indicated that central and peripheral fat deposits have different influences on chronic inflammatory cytokines and adipokines, such as adiponectin, leptin, and C-reactive protein (44,45), which may partly explain various effect of regional fat distribution on heart function. Further studies are needed to evaluate the complex relationships among body fat distribution, inflammatory cytokines and adipokines, and LV function. Finally, although measurements of the LA strain have been shown to have good repeatability and accuracy, the irregular boundary and thin wall of the LV pose a challenge for strain measurement. Measurements need to be taken by experienced experts.
Conclusions
CMR-derived tissue tracking and volume-time curve non-invasively detect subclinical diastolic dysfunction in obese uncomplicated adults with preserved LVEF. Central adipose tissue deposits (trunk fat and visceral fat) may exhibit inverse relationships with LV and LA function in obesity. However, peripheral adipose tissue deposits (peripheral fat and gynoid fat) may show positive relationships with them. The finding suggests that recognizing the role of different areas of fat on the heart may be beneficial for obese patients.
Acknowledgments
Funding: This work was supported by grants from
Footnote
Reporting Checklist: The authors have completed the STROBE reporting checklist. Available at https://qims.amegroups.com/article/view/10.21037/qims-23-1785/rc
Conflicts of Interest: All authors have completed the ICMJE uniform disclosure form (available at https://qims.amegroups.com/article/view/10.21037/qims-23-1785/coif). All authors report that this work was supported by grants from the National Natural Science Foundation of China (No. 81601462), the Key Research & Development Project of Science and Technology of Sichuan Province (No. 2021YFS0142), the Science and Technology Department of Sichuan Province (No. 2019YFS0302), and the 1.3.5. Project for Disciplines of Excellence, West China Hospital, Sichuan University (No. ZYGD18017). The authors have no other conflicts of interest to declare.
Ethical Statement: The authors are accountable for all aspects of the work in ensuring that questions related to the accuracy or integrity of any part of the work are appropriately investigated and resolved. The study was conducted in accordance with the Declaration of Helsinki (as revised in 2013). The study was approved by the Institutional Review Board of the West China Hospital of Sichuan University (No. 2019-321), and informed consent was provided by all participants.
Open Access Statement: This is an Open Access article distributed in accordance with the Creative Commons Attribution-NonCommercial-NoDerivs 4.0 International License (CC BY-NC-ND 4.0), which permits the non-commercial replication and distribution of the article with the strict proviso that no changes or edits are made and the original work is properly cited (including links to both the formal publication through the relevant DOI and the license). See: https://creativecommons.org/licenses/by-nc-nd/4.0/.
References
- Carbone S, Lavie CJ, Elagizi A, Arena R, Ventura HO. The Impact of Obesity in Heart Failure. Heart Fail Clin 2020;16:71-80. [Crossref] [PubMed]
- Pandey A, LaMonte M, Klein L, Ayers C, Psaty BM, Eaton CB, Allen NB, de Lemos JA, Carnethon M, Greenland P, Berry JD. Relationship Between Physical Activity, Body Mass Index, and Risk of Heart Failure. J Am Coll Cardiol 2017;69:1129-42. [Crossref] [PubMed]
- Obokata M, Reddy YNV, Pislaru SV, Melenovsky V, Borlaug BA. Evidence Supporting the Existence of a Distinct Obese Phenotype of Heart Failure With Preserved Ejection Fraction. Circulation 2017;136:6-19. [Crossref] [PubMed]
- Leowattana W. Obesity and heart failure with preserved ejection fraction. Eur J Prev Cardiol 2019;26:1591-3. [Crossref] [PubMed]
- Paulus WJ, Tschöpe C, Sanderson JE, Rusconi C, Flachskampf FA, Rademakers FE, Marino P, Smiseth OA, De Keulenaer G, Leite-Moreira AF, Borbély A, Edes I, Handoko ML, Heymans S, Pezzali N, Pieske B, Dickstein K, Fraser AG, Brutsaert DL. How to diagnose diastolic heart failure: a consensus statement on the diagnosis of heart failure with normal left ventricular ejection fraction by the Heart Failure and Echocardiography Associations of the European Society of Cardiology. Eur Heart J 2007;28:2539-50. [Crossref] [PubMed]
- Nagueh SF, Smiseth OA, Appleton CP, Byrd BF 3rd, Dokainish H, Edvardsen T, Flachskampf FA, Gillebert TC, Klein AL, Lancellotti P, Marino P, Oh JK, Alexandru Popescu B, Waggoner AD. Houston, Texas; Oslo, Norway; Phoenix, Arizona; Nashville, Tennessee; Hamilton, Ontario, Canada; Uppsala, Sweden; Ghent and Liège, Belgium; Cleveland, Ohio; Novara, Italy; Rochester, Minnesota; Bucharest, Romania; and St. Recommendations for the Evaluation of Left Ventricular Diastolic Function by Echocardiography: An Update from the American Society of Echocardiography and the European Association of Cardiovascular Imaging. Eur Heart J Cardiovasc Imaging 2016;17:1321-60. [Crossref] [PubMed]
- Claus P, Omar AMS, Pedrizzetti G, Sengupta PP, Nagel E. Tissue Tracking Technology for Assessing Cardiac Mechanics: Principles, Normal Values, and Clinical Applications. JACC Cardiovasc Imaging 2015;8:1444-60. [Crossref] [PubMed]
- Pedrizzetti G, Claus P, Kilner PJ, Nagel E. Principles of cardiovascular magnetic resonance feature tracking and echocardiographic speckle tracking for informed clinical use. J Cardiovasc Magn Reson 2016;18:51. [Crossref] [PubMed]
- Xu HY, Yang ZG, Guo YK, Shi K, Liu X, Zhang Q, Jiang L, Xie LJ. Volume-time curve of cardiac magnetic resonance assessed left ventricular dysfunction in coronary artery disease patients with type 2 diabetes mellitus. BMC Cardiovasc Disord 2017;17:145. [Crossref] [PubMed]
- Cameli M, Lisi M, Mondillo S, Padeletti M, Ballo P, Tsioulpas C, Bernazzali S, Maccherini M. Left atrial longitudinal strain by speckle tracking echocardiography correlates well with left ventricular filling pressures in patients with heart failure. Cardiovasc Ultrasound 2010;8:14. [Crossref] [PubMed]
- Cameli M, Sparla S, Losito M, Righini FM, Menci D, Lisi M, D'Ascenzi F, Focardi M, Favilli R, Pierli C, Fineschi M, Mondillo S. Correlation of Left Atrial Strain and Doppler Measurements with Invasive Measurement of Left Ventricular End-Diastolic Pressure in Patients Stratified for Different Values of Ejection Fraction. Echocardiography 2016;33:398-405. [Crossref] [PubMed]
- Liu J, Li J, Xia C, He W, Li X, Shen S, Zhou X, Tong N, Peng L. The effect of hyperlipidemia and body fat distribution on subclinical left ventricular function in obesity: a cardiovascular magnetic resonance study. Cardiovasc Diabetol 2024;23:120. [Crossref] [PubMed]
- Vasan SK, Osmond C, Canoy D, Christodoulides C, Neville MJ, Di Gravio C, Fall CHD, Karpe F. Comparison of regional fat measurements by dual-energy X-ray absorptiometry and conventional anthropometry and their association with markers of diabetes and cardiovascular disease risk. Int J Obes (Lond) 2018;42:850-7. [Crossref] [PubMed]
- Peppa M, Koliaki C, Hadjidakis DI, Garoflos E, Papaefstathiou A, Katsilambros N, Raptis SA, Dimitriadis GD. Regional fat distribution and cardiometabolic risk in healthy postmenopausal women. Eur J Intern Med 2013;24:824-31. [Crossref] [PubMed]
- Liu J, Li J, Yu J, Xia C, Pu H, He W, Li X, Zhou X, Tong N, Peng L. Regional Fat Distributions Are Associated With Subclinical Right Ventricular Dysfunction in Adults With Uncomplicated Obesity. Front Cardiovasc Med 2022;9:814505. [Crossref] [PubMed]
- Rehman S, Hashmi MF, Nelson VL. Blood Pressure Measurement. In: StatPearls [Internet]. Treasure Island (FL): StatPearls Publishing, 2022.
- Muniyappa R, Lee S, Chen H, Quon MJ. Current approaches for assessing insulin sensitivity and resistance in vivo: advantages, limitations, and appropriate usage. Am J Physiol Endocrinol Metab 2008;294:E15-26. [Crossref] [PubMed]
- Chen Y, Peng Q, Yang Y, Zheng S, Wang Y, Lu W. The prevalence and increasing trends of overweight, general obesity, and abdominal obesity among Chinese adults: a repeated cross-sectional study. BMC Public Health 2019;19:1293. [Crossref] [PubMed]
- de Simone G, Daniels SR, Devereux RB, Meyer RA, Roman MJ, de Divitiis O, Alderman MH. Left ventricular mass and body size in normotensive children and adults: assessment of allometric relations and impact of overweight. J Am Coll Cardiol 1992;20:1251-60. [Crossref] [PubMed]
- Bojer AS, Soerensen MH, Gaede P, Myerson S, Madsen PL. Left Ventricular Diastolic Function Studied with Magnetic Resonance Imaging: A Systematic Review of Techniques and Relation to Established Measures of Diastolic Function. Diagnostics (Basel) 2021;11:1282. [Crossref] [PubMed]
- Liu J, Li J, Pu H, He W, Zhou X, Tong N, Peng L. Cardiac remodeling and subclinical left ventricular dysfunction in adults with uncomplicated obesity: a cardiovascular magnetic resonance study. Quant Imaging Med Surg 2022;12:2035-50. [Crossref] [PubMed]
- Schober P, Boer C, Schwarte LA. Correlation Coefficients: Appropriate Use and Interpretation. Anesth Analg 2018;126:1763-8. [Crossref] [PubMed]
- Koo TK, Li MY. A Guideline of Selecting and Reporting Intraclass Correlation Coefficients for Reliability Research. J Chiropr Med 2016;15:155-63. [Crossref] [PubMed]
- Homsi R, Yuecel S, Schlesinger-Irsch U, Meier-Schroers M, Kuetting D, Luetkens J, Sprinkart A, Schild HH, Thomas DK. Epicardial fat, left ventricular strain, and T1-relaxation times in obese individuals with a normal ejection fraction. Acta Radiol 2019;60:1251-7. [Crossref] [PubMed]
- Zhu L, Gu S, Wang Q, Zhou X, Wang S, Fu C, Yang W, Wetzl J, Yan F. Left ventricular myocardial deformation: a study on diastolic function in the Chinese male population and its relationship with fat distribution. Quant Imaging Med Surg 2020;10:634-45. [Crossref] [PubMed]
- Kammerlander AA, Donà C, Nitsche C, Koschutnik M, Schönbauer R, Duca F, Zotter-Tufaro C, Binder C, Aschauer S, Beitzke D, Loewe C, Hengstenberg C, Bonderman D, Mascherbauer J. Feature Tracking of Global Longitudinal Strain by Using Cardiovascular MRI Improves Risk Stratification in Heart Failure with Preserved Ejection Fraction. Radiology 2020;296:290-8. [Crossref] [PubMed]
- Yan WF, Gao Y, Zhang Y, Guo YK, Wang J, Jiang L, Li Y, Yang ZG. Impact of type 2 diabetes mellitus on left ventricular diastolic function in patients with essential hypertension: evaluation by volume-time curve of cardiac magnetic resonance. Cardiovasc Diabetol 2021;20:73. [Crossref] [PubMed]
- Alpert MA, Karthikeyan K, Abdullah O, Ghadban R. Obesity and Cardiac Remodeling in Adults: Mechanisms and Clinical Implications. Prog Cardiovasc Dis 2018;61:114-23. [Crossref] [PubMed]
- Barbier P, Solomon SB, Schiller NB, Glantz SA. Left atrial relaxation and left ventricular systolic function determine left atrial reservoir function. Circulation 1999;100:427-36. [Crossref] [PubMed]
- Chirinos JA, Sardana M, Satija V, Gillebert TC, De Buyzere ML, Chahwala J, De Bacquer D, Segers P, Rietzschel ER. Effect of Obesity on Left Atrial Strain in Persons Aged 35-55 Years (The Asklepios Study). Am J Cardiol 2019;123:854-61. [Crossref] [PubMed]
- Evin M, Broadhouse KM, Callaghan FM, McGrath RT, Glastras S, Kozor R, Hocking SL, Lamy J, Redheuil A, Kachenoura N, Fulcher GR, Figtree GA, Grieve SM. Impact of obesity and epicardial fat on early left atrial dysfunction assessed by cardiac MRI strain analysis. Cardiovasc Diabetol 2016;15:164. [Crossref] [PubMed]
- Mahfouz RA, Gomma A, Goda M, Safwat M. Relation of left atrial stiffness to insulin resistance in obese children: Doppler strain imaging study. Echocardiography 2015;32:1157-63. [Crossref] [PubMed]
- Singleton MJ, Nelson MB, Samuel TJ, Kitzman DW, Brubaker P, Haykowsky MJ, Upadhya B, Chen H, Nelson MD. Left Atrial Stiffness Index Independently Predicts Exercise Intolerance and Quality of Life in Older, Obese Patients With Heart Failure With Preserved Ejection Fraction. J Card Fail 2022;28:567-75. [Crossref] [PubMed]
- Vieira MJ, Teixeira R, Gonçalves L, Gersh BJ. Left atrial mechanics: echocardiographic assessment and clinical implications. J Am Soc Echocardiogr 2014;27:463-78. [Crossref] [PubMed]
- Brecht A, Oertelt-Prigione S, Seeland U, Rücke M, Hättasch R, Wagelöhner T, Regitz-Zagrosek V, Baumann G, Knebel F, Stangl V. Left Atrial Function in Preclinical Diastolic Dysfunction: Two-Dimensional Speckle-Tracking Echocardiography-Derived Results from the BEFRI Trial. J Am Soc Echocardiogr 2016;29:750-8. [Crossref] [PubMed]
- Guan Z, Zhang D, Huang R, Zhang F, Wang Q, Guo S. Association of left atrial myocardial function with left ventricular diastolic dysfunction in subjects with preserved systolic function: a strain rate imaging study. Clin Cardiol 2010;33:643-9. [Crossref] [PubMed]
- Webb J, Fovargue L, Tøndel K, Porter B, Sieniewicz B, Gould J, Rinaldi CA, Ismail T, Chiribiri A, Carr-White G. The Emerging Role of Cardiac Magnetic Resonance Imaging in the Evaluation of Patients with HFpEF. Curr Heart Fail Rep 2018;15:1-9. [Crossref] [PubMed]
- von Bibra H, St John Sutton M. Diastolic dysfunction in diabetes and the metabolic syndrome: promising potential for diagnosis and prognosis. Diabetologia 2010;53:1033-45. [Crossref] [PubMed]
- Mancia G, Fagard R, Narkiewicz K, Redon J, Zanchetti A, Böhm M, et al. 2013 ESH/ESC guidelines for the management of arterial hypertension: the Task Force for the Management of Arterial Hypertension of the European Society of Hypertension (ESH) and of the European Society of Cardiology (ESC). Eur Heart J 2013;34:2159-219. [Crossref] [PubMed]
- Iacobellis G, Corradi D, Sharma AM. Epicardial adipose tissue: anatomic, biomolecular and clinical relationships with the heart. Nat Clin Pract Cardiovasc Med 2005;2:536-43. [Crossref] [PubMed]
- Sonaglioni A, Ferrulli A, Nicolosi GL, Lombardo M, Luzi L. The Influence of Anthropometrics on Cardiac Mechanics in Healthy Women With Opposite Obesity Phenotypes (Android vs Gynoid). Cureus 2024;16:e51698. [Crossref] [PubMed]
- Yao YS, Li TD, Zeng ZH. Mechanisms underlying direct actions of hyperlipidemia on myocardium: an updated review. Lipids Health Dis 2020;19:23. [Crossref] [PubMed]
- Nadruz W, Shah AM, Solomon SD. Diastolic Dysfunction and Hypertension. Med Clin North Am 2017;101:7-17. [Crossref] [PubMed]
- Oliveira A, Lopes C, Severo M, Rodríguez-Artalejo F, Barros H. Body fat distribution and C-reactive protein--a principal component analysis. Nutr Metab Cardiovasc Dis 2011;21:347-54. [Crossref] [PubMed]
- Christen T, Trompet S, Noordam R, van Klinken JB, van Dijk KW, Lamb HJ, Cobbaert CM, den Heijer M, Jazet IM, Jukema JW, Rosendaal FR, de Mutsert R. Sex differences in body fat distribution are related to sex differences in serum leptin and adiponectin. Peptides 2018;107:25-31. [Crossref] [PubMed]