Neuronavigation-assisted pituitary neuroendocrine tumor resection: a systematic review and meta-analysis
Introduction
Pituitary tumor represents a common intracranial pathology and pituitary neuroendocrine tumor (PitNET) is the most common type of pituitary tumor, in which usually hormones are abnormally secreted and systematic upwards compression of optic structures occurs, resulting in a wide variety of clinical sequelae (1,2). Transsphenoidal surgery (TSS), known for its simplicity and minimal invasiveness, is widely embraced as the primary treatment for most PitNET cases, with both the endoscope and microscope yielding satisfactory clinical outcomes (3,4). For PitNET, gross total resection (GTR) has been found to significantly reduce the chances of recurrence is the surgical ideal target (3,5). In recent years, intraoperative neuronavigation for intracranial surgery, especially intraoperative magnetic resonance imaging (iMRI) system, has become increasingly popular and has significantly facilitated pituitary and other skull base surgeries (6). The combined technique has shown promising results in the enhancement of the therapeutic effects of surgeries and in the preservation of the hypophysis (7). Furthermore, its implementation reduces the associated complications, including cerebrospinal fluid (CSF) leak, intraoperative hemorrhage, hypopituitarism, headache, nasal septal perforation, and postoperative pain and discomfort (8). Nevertheless, controversies exist in the publications on the impact of intraoperative neuronavigation in TSS, regarding the various types of surgical mirrors and imaging systems (8,9).
Currently, a large number of studies have been performed to explore the operative effects of neuronavigation-assisted PitNET resections (9-16), but no comprehensive and consistent evaluation and quantitative analysis of research results have been conducted. Therefore, we conducted a systematic review and meta-analysis to assess the curative effects and complications of PitNET resection with or without the use of an image-based neuronavigation, as well as the clinical values of the image-assisted techniques. We present this article in accordance with the PRISMA reporting checklist (17) (available at https://qims.amegroups.com/article/view/10.21037/qims-23-1570/rc).
Methods
This systematic review and meta-analysis was registered with PROSPERO (CRD42022332705) (https://www.crd.york.ac.uk/PROSPERO/).
Search strategies
We searched publications in the following databases: PubMed, Cochrane Library, EMBASE, Web of Science, and Scopus, which were completed by May 1, 2024. During the literature search phase, there were no restrictions on date and type of publication. Our search strategy included the use of combinations of the following terms: Pituitary Neoplasm; Pituitary Neoplasms; Pituitary Tumor; Pituitary Tumors; Pituitary Adenoma; Pituitary Adenomas; Pituitary Carcinoma; Pituitary Carcinomas; Cancer of the Pituitary; Cancer of Pituitary; Pituitary Cancer; Pituitary Cancers; Frameless Stereotaxy; Stereotaxy, Frameless; Image-guidance; Intraoperative Magnetic; Resonance Imaging; Intraoperative MRI (iMRI); Stereotaxy Neuronavigation (SNN); MR Imaging Neuronavigation (INN); iMRI; SNN; INN; Stereotaxy-guided Operative Neurosurgery; Frame Stereotactic Neurosurgery; Frameless Stereotactic Neurosurgery; MR Image-guided; Neuro-navigation; CT-guided; MRI-guided; Gross Total Resection; GTR; Extent of Resection. We searched for word variations as far as possible. Furthermore, reference lists for previous systematic reviews and other relevant publications were manually searched to complement the database. The last comprehensive search was conducted by May 3, 2024.
Study selection
Only in vivo studies enrolling humans of all age groups in English were considered, regardless of the age and nationality of the subjects. Considering the limited number of the expected randomized controlled trials (RCTs), we also intended to include eligible case-control trials and cohort studies. Conference abstracts and studies with fewer than five subjects were excluded. Studies would be eligible if they fulfilled all of the following criteria: (I) studies reporting pituitary tumor resection without restrictions on the surgical area, surgical personnel, and specific surgical approach; (II) RCTs, case control trials, prospective cohort studies or retrospective cohort studies, including an experimental group with iMRI and a control group without iMRI, or an experimental group with other neuronavigation system, and a control group without any neuronavigation; (III) the enrolled studies included appropriate outcome indicators, such as GTR, operative time, surgery cost, and postoperative complications containing CSF leak, headache, hypopituitarism, diabetes insipidus, vision decreasing, rhinoliquorrhea, epistaxis, and CNS infection; (IV) patients included in the studies underwent either endoscopic or microscopic transsphenoidal resection for PitNET with neuronavigation. In addition, to avoid interference from pituitary tumors of different pathological types on the effectiveness of neuronavigation, cases in the publications reporting Rathke cleft cysts, craniopharyngiomas, gliomas, meningiomas, or other irrelevant lesions without classification, were excluded. Moreover, studies primarily addressing patients for whom the surgical target was solely decompression were excluded. The following consecutive steps of the literature screening process were performed. First, we used Review Manager 5.3 to remove duplicate publications and then manually removed residual duplicates. Second, we analyzed the titles and abstracts, which were performed by two independent investigators (Y.H. and H.L.) based on the pre-set inclusion and exclusion criteria. If disagreements occurred, a third investigator with more than ten-year experience of neurologic radiology (Y.L.) would make the final determination. Third, publications whose titles and abstracts could not provide sufficient information to make the decision on their inclusion or exclusion were subsequently re-analyzed by full-text screening. Finally, to avoid the double counting of data derived from multiple studies from the same group of researchers, we assessed the sample size, recruitment period, and baseline sample characteristics of each article to identify and exclude duplicate data across different publications. While exact cohort duplicates were excluded, we did include updated versions of previously published cohorts with a sample size increase of at least 50%.
Risk of bias assessment
The above-mentioned two investigators also independently evaluated the quality of the included publications. If there was a difference, they would consult with a 3rd reviewer. The quality evaluation and cross-checking were carried out according to the evaluation criteria of the Newcastle-Ottawa Scale (NOS) (5), mainly considering the selection methods of the case groups and the control groups, the comparability of the case groups and the control groups, and the exposure assessment methods.
Data analysis
Review manager 5.3 was employed for meta-analysis. The odds ratio (OR) along with 95% confidence interval (CI) were used as the effect indexes of image-based neuronavigation for the incidence of GTR and postoperative CSF leak. Low, moderate and high have been provisionally assigned to I2 values of 25%, 50% and 75%, respectively (based on the Cochrane Handbook guidelines) (18). I2<50%, indicated that the heterogeneity among studies was acceptable, and a fixed-effect model was used; I2 ≥50% indicated high heterogeneity among the groups, and thus a random-effect model was used (19). After analysis, we found that there was small heterogeneity in GTR-relevant publications, so a fixed effects meta-analysis was decided upon. To explore the source of heterogeneity, the subgroup analysis was performed. The patients were divided into three subgroups based on the MRI field strength: low-field iMRI subgroup (<1.5T), high-field iMRI subgroup (≥1.5T, <3T), and ultra-high-field iMRI subgroup (≥3T) (20). The patients were also divided into a microscopic group and an endoscopic group according to the types of surgical mirrors. However, there was great heterogeneity in postoperative CSF leak-relevant publications, so a random-effect meta-analysis was decided to be implemented. Subgroup analysis could not be performed due to insufficient data. Sensitivity analysis was performed by removing single literature in sequence and then obtaining new statistical results from the remaining articles in Review Manager 5.3. The meta-analysis results were illustrated with forest plots, and the publication bias was analyzed with funnel plots.
Results
Study selection
Through searching electronic databases, we retrieved 2,500 potentially eligible publications (Table S1). After the evaluation of the titles and abstracts, 452 potentially eligible publications were selected for full-text screening. Ultimately, 42 eligible publications were selected for the analysis by synthesis in this study (Figure 1). Moreover, two publications registered in Web of Science that may meet the criteria were not chosen for full-text screen because we did not have access to the full text or the results (the data were not available even after contacting the authors).
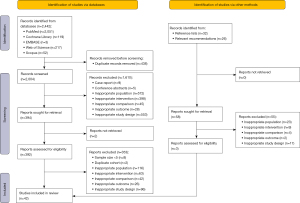
Quality assessment of the included publications
Based on the NOS, the 42 included publications were subjected to a systematic quality evaluation. Eight items were contained in the NOS, categorizing into three dimensions: subject selection containing (I) is the case definition adequate? (II) Representativeness of the cases; (III) selection of controls; (IV) definition of controls, comparability containing (I) comparability of cases and controls on the bases of the design or analysis and exposure containing (I) admission of exposure; (II) same method of admission for cases and controls; (III) non-response rate in case control study. A succession of response options were provided for each item. The star system was used for semi-quantitative assessment of research quality. A maximum of one star was awarded for each item, except for item related to comparability which were awarded two stars. The NOS scores range from zero ★ to nine ★ (21). A higher score indicated a better quality. Generally, studies with at least 4 points were included in the meta-analysis (22). Among all the publications included in this study, 33 publications clearly grouped the patients by “age” and “sex”, indicating that the most important confounding factors were controlled, and the total score was eight points (Tables S2,S3); two publications did not clearly group the patients by “age” or “gender”, which received a total score of seven points (23,24); seven did not clearly group the patients by “age” and “gender”, and received a total score of six points (12,25-30). In addition, the quality of the exposure assessment methods of the 42 publications was high, and thus was given three ★, whereas the quality of the selection assessment methods of the 42 publications was good and awarded two ★.
Characteristics of the included studies
We included a total number of 42 studies, 37 of which assessed intraoperative and postoperative GTR rates and the other five publications were associated with postoperative CSF leak. An overview of the characteristics of the included publications was presented in Tables 1,2. In terms of field strength selection, low-field, high-field, and ultra-high-field iMRI was used in 13, 18, and 6 of the published studies, respectively. Regarding the surgical technique employed, microscopic and endoscopic resection were performed in 17 and 13 of the studies, respectively.
Table 1
Author | Year | Total number of patients | Age (years), mean (± SD) or mean (range) or n [%] | Male, n [%] |
Microscopic/endoscopic, n | Field strength |
NFPT, n [%] |
---|---|---|---|---|---|---|---|
Low-field | |||||||
Ahn et al. (31) | 2008 | 51 | NA | NA | 51/0 | 0.15 T Polestar N20 | NA |
Berkmann et al. (6) | 2011 | 32 | 57 (±17.9) | 23 [72] | NA | 0.15 T PoleStar N20 | 26 [81] |
Berkmann et al. (32) | 2012 | 92 | NA | NA | 92/0 | 0.15 T Polestar N20 | 79 [86] |
Bohinski et al. (8) | 2001 | 29 | 51 (24–74) | 18 [62] | 29/0 | 0.3 T AIRIS II | 22 [76] |
Fahlbusch et al. (33) | 2001 | 44 | 53 (±14.9) | 29 [66] | 44/0 | 0.2 T Magnetom Open | 39 [89] |
García et al. (34) | 2017 | 30 | 55 | 13 [43] | 0/30 | 0.15 T Polestar N30 | 15 [50] |
Hlavica et al. (13) | 2013 | 104 | 59 (22–86) | 57 [55] | 104/0 | 0.15 T Polestar N20 | 104 [100] |
Jiménez et al. (35) | 2016 | 18 | NA | NA | 0/18 | 0.15 T Polestar N20 | 10 [56] |
Martin et al. (36) | 1999 | 5 | 36.2 (28–42) | 2 [40] | 5/0 | 0.5 T | 0 [0] |
Ramm-Pettersen et al. (37) | 2011 | 20 | 54 (23–71) | 13 [65] | 20/0 | 0.5 T Signa SP | 16 [80] |
Schwartz et al. (38) | 2006 | 15 | 49 (29–67) | 9 [60] | 0/15 | 0.12 T Polestar N10 | 11 [73] |
Strange et al. (39) | 2020 | 231 | 55.5 (18–88) | 127 [55] | 0/231 | 0.15 T Polestar N20 | 160 [69] |
Wu et al. (40) | 2009 | 55 | 45.9 (±12.6) | 36 [65] | 55/0 | 0.15 T Polestar N20 | 29 [53] |
High-field | |||||||
Berkmann et al. (41) | 2014 | 85 | 55 (±14) | 57 [67] | 85/0 | 1.5 T Magnetom | 85 [100] |
Chen et al. (23) | 2012 | 13 | NA | NA | 13/0 | 1.5 T Magnetom | NA |
Dort et al. (42) | 2001 | 15 | 50 (15–80) | 8 [53] | 15/0 | 1.5 T | NA |
Gohla et al. (43) | 2020 | 42 | 52 (17–79) | 23 [55] | 42/0 | 1.5 T Espree | 35 [83] |
Hlaváč et al. (44) | 2019 | 111 | 57.3 (22–78) | 75 [68] | 66/45 | 1.5 T Espree | 91 [82] |
Kuge et al. (45) | 2013 | 35 | 54.3 (±15.5) | 18 [51] | 0/35 | 1.5 T | 27 [77] |
Li et al. (46) | 2015 | 30 | 36 (21–65) | 13 [43] | 30/0 | 1.5 T Espree | 9 [30] |
Nimsky et al. (25) | 2004 | 48 | NA | NA | 48/0 | 1.5 T | NA |
Nimsky et al. (12) | 2006 | 85 | NA | NA | 85/0 | 1.5 T Magnetom | 85 [100] |
Pal’a et al. (9) | 2017 | 96 | 54 (7–78) | 71 [74] | 68/28 | 1.5 T Espree | 64 [67] |
Paľa et al. (47) | 2022 | 59 | 57 | 42 [71] | 0/59 | 1.5 T | 42 [71] |
Pala et al. (48) | 2022 | 190 | 55 | 106 [56] | 88/102 | 1.5 T | NA |
Paterno' et al. (29) | 2014 | 49 | NA | NA | 0/49 | 1.5 T Espree | 49 [100] |
Sylvester et al. (30) | 2015 | 156 | NA | NA | 115/41 | 1.5 T Espree | NA |
Szerlip et al. (49) | 2011 | 53 | 49 (1.8 SEM) | 25 [47] | 53/0 | 1.5 T Espree | 39 [74] |
Tanei et al. (50) | 2013 | 14 | 37.4 (±11.8) | 2 [14] | 0/14 | 1.5 T Magnetom | 0 [0] |
Zhang et al. (51) | 2017 | 137 | 7–82 | 73 [53] | 0/137 | 1.5 T Espree | 103 [75] |
Zhang et al. (52) | 2019 | 133 | 50 (±12) | 61 [46] | 0/133 | 1.5 T Espree | 133 [100] |
Ultra-high-field | |||||||
Fomekong et al. (53) | 2014 | 73 | NA | 46 [63] | 73/0 | 3 T Intera | NA |
Netuka et al. (26) | 2011 | 49 | NA | NA | NA | 3 T | NA |
Qiu et al. (27) | 2012 | 49 | NA | NA | NA | 3 T Mangetom | NA |
Serra et al. (11) | 2016 | 51 | 33 [65] | 27 [53] | 0/51 | 3 T Mangetom | 33 [65] |
Staartjes et al. (10) | 2019 | 95 | 65 [68] | 53 [56] | 0/95 | 3 T Mangetom | 65 [68] |
Zaidi et al. (14) | 2016 | 20 | 14 [70] | 9 [45] | 0/20 | 3 T Verio | 14 [70] |
GTR, gross total resection; SD, standard deviation; NFPT, nonfunctioning pituitary tumor; NA, not applicable; SEM, standard error of mean.
Table 2
Author | Year | Sample capacity | Intervening measure | |||
---|---|---|---|---|---|---|
Experimental group | Control group | Experimental group | Control group | |||
Achey et al. (54) | 2019 | 175 | 444 | Stereotactic CT-assisted endoscope | Endoscope | |
Alshareef et al. (55) | 2021 | 34 | 104 | IOUS | Without IOUS | |
Chung et al. (24) | 2015 | 2,996 | 45,446 | CT/MRI-assisted endoscope | Endoscope | |
Eboli et al. (28) | 2011 | 208 | 65 | iCT/EM-assisted TSA | Fluoroscope-assisted TSA | |
Tosaka et al. (56) | 2015 | 30 | 30 | iCT-assisted endoscopic TSA | Conventional endoscopic TSA |
CSF leak, cerebrospinal fluid leak; CT, computed tomography; IOUS, intraoperative ultrasonography; MRI, magnetic resonance imaging; iCT, intraoperative computed tomography; EM, frameless electromagnetic; TSA, transsphenoidal approach.
Statistical analysis of the GTR and postoperative CSF leak results
The fixed-effect model indicated that, in the 37 included publications (2,271 subjects), the OR of image-based neuronavigation was 2.29 (95% CI: 2.02–2.60, P<0.00001) for GTR (Figure 2). The I2 statistic was 24% representing smaller heterogeneity. Therefore, a fixed-effect model was selected for meta-analysis.
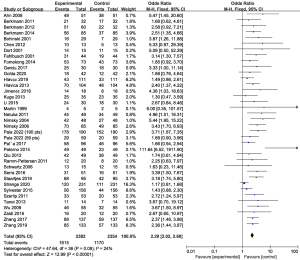
The fixed-effect model indicated that, in the five included publications (49,532 subjects), the OR of image-based neuronavigation was 0.77 (95% CI: 0.67–0.88, P<0.01) for postoperative CSF leak. Heterogeneity, as measured by I2 statistic, reached up to 91%, so a random effect model was selected for meta-analysis.
Factors affecting GTR
To explore the source of heterogeneity, the subgroup analysis was performed in GTR-related publications based on the magnetic field strength and the type of surgical mirror as mentioned before. The statistical analysis results are presented in Figure 3 and Figure 4.
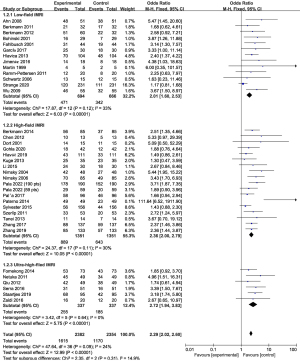
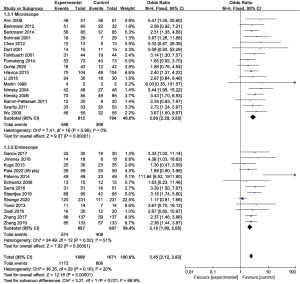
Low-field iMRI publications (n=13), containing a total of 726 objects, indicated that using low-field iMRI was significantly associated with the incidence of GTR (OR: 2.01, 95% CI: 1.60–2.53, P<0.00001, I2=33%) (6,8,13,31-40). High-field iMRI publications (n=18), containing a total number of 1,351 objects, revealed that the high-field iMRI has a higher correlation with the incidence of GTR (OR: 2.36, 95% CI: 2.00–2.79, P<0.00001, I2=30%) (9,12,23,25,29,41-49). In the ultra-high-field iMRI group (n=6) including 337 objects, we found that the ultra-high-field iMRI had the highest correlation with the incidence of GTR (OR: 2.72, 95% CI: 1.94–3.83, P<0.00001, I2=0%) (10,11,14,26,27,53). However, the differences among the three subgroups were not statistically significant (P=0.31, I2=14.9%) (Figure 3).
The microscopic group (n=17), containing a total of 844 objects, indicated that the rate of GTR was 2.85 times higher in patients with iMRI than in patients without iMRI (95% CI: 2.29–3.55, P<0.00001, I2=0%) (8,12,13,23,25,31-33,36,37,40-43,46,53,57). In the endoscopic group (n=13), containing a total of 887 objects, indicated that the rate of GTR was 2.18 times higher in patients with iMRI than in patients without iMRI (95% CI: 1.80–2.65, P<0.00001, I2=51%) (10,11,14,29,34,35,38,39,45,48,50-52). Our results showed that there was no statistically significant difference between the two groups (P=0.07, I2=68.9%) (Figure 4).
However, due to the insufficient literature data, we were unable to perform other subgroup analyses.
Sensitivity analysis
Five publications were subjected to sensitivity analysis. After removing any single literature, a new meta-analysis was carried out respectively. We found that heterogeneity was significantly reduced (91% to 43%) when the literature of Eboli et al. (28) was removed, indicating that the heterogeneity was most likely to be derived from this article. We decided to exclude it and established that the postoperative CSF leak incidence slightly decreased after the utilization of neuronavigation (OR: 0.84, 95% CI: 0.73–0.97, P=0.01, I2=43%).
Evaluation of the publication bias
An assessment of the risk of bias in the publications describing GTR and postoperative CSF leak was performed. The funnel plot analysis showed that the distribution of studies on both sides of the funnel plot was not completely symmetrical, suggesting that publication bias might exist.
Discussion
Our systematic review and meta-analysis summarized the efficacy of the neuronavigation, especially the magnetic resonance imaging neuronavigation, in PitNET resection. To comprehensively evaluate the operative effect, we selected the most commonly used GTR as an evaluation indicator and included postoperative CSF leak as an evaluation indicator for complications. The results of the meta-analysis of previous research achievements showed that the use of neuronavigation systems increased the rate of GTR. In addition, postoperative CSF leak incidence slightly decreased after the utilization of neuronavigation, revealing that intraoperative neuronavigation can improve the surgical outcomes. It is noteworthy that moderate heterogeneity existed among different articles. We speculated that this heterogeneity might be to some extent due to the differences in factors among different centers, such as demographics, surgical techniques, and imaging systems. To explore the specific sources of heterogeneity, we performed subgroup analyses of statistics to address variables such as field strength and surgical instrument. Our results showed that the benefits of iMRI surgical mirror exerted a moderate influence on GTR, whereas the field strength had an insignificant impact on GTR. Considering the huge heterogeneity among the included publications concerning the postoperative CSF leak, these results were to be interpreted with caution. Ultimately, we found that the heterogeneity might have been due mainly to one of the publications (Eboli, 2011). Its exclusion significantly reduced the heterogeneity (I2 from 91% to 43%) (55). Therefore, we assumed that the heterogeneity stemmed from the various quality ratings of the five included articles ranging from six (Eboli, 2011) to eight stars and different imaging equipment, including computed tomography (CT), MRI, and ultrasound. According to the risk of bias analysis in the light of the NOS, all the publications we included were not RCTs, so the "Selection of Controls" item cannot be scored. Among them, nine articles were rated as six and seven points because the confounding factors of age and gender were not adjusted (12,23-30). The statistical results of bias analysis indicated that the funnel plots of GTR-related and postoperative CSF leak-related publications were asymmetric, indicating that potential issues such as publication bias, heterogeneity of studies, and uneven research quality might exist in the studies included. Therefore, caution should be exercised when applying these findings to guide clinical practice.
Previously, few systematic reviews and meta-analyses have evaluated the effects of neuronavigation-assisted PitNET resections (16,58,59). In a systematic review analyzing 85 studies, Soneru et al. (16) reported that the GTR rates in 7,124 PitNET patients were determined: in 62 studies, the pooled proportions of GTR were 68.3% and 70.7% for mTSS + iMRI and eTSS + iMRI, respectively. These research results indicated that the final GTR proportions were similar regardless of whether the surgeons used a microscope supplemented with iMRI or an endoscope with or without iMRI. This study focused on comparing the differences between microscopic- and endoscopic-based approaches, whereas our research was focused mainly on comparing the impacts of PitNET surgery with or without iMRI. In 2021, Staartjes et al. (58) performed a meta-analysis of 34 studies including 2,130 patients. The researchers evaluated the GTR, extent of resection (EOR), and residual volume (RV). Their results revealed that one fifth of patients undergoing PitNET resection converted from non-GTR to GTR after the use of iMRI. However, no data regarding complications were provided in this study. A meta-analysis conducted by Zhang et al. (59) of 33 studies in 2022 and including 2,099 patients analyzed a large number of publications on the evaluation of postoperative CSF leak. Furthermore, these scientists performed subgroup analysis of functioning versus nonfunctioning adenomas, indicating that the use of iMRI significantly increased GTR with comparable benefits for both functioning and nonfunctioning adenomas while decreasing the incidence of postoperative surgical complications. In contrast, more patients were enrolled in our study. According to our evaluation criteria, the included articles in our analysis had a higher level of evidence.
Previous studies have suggested that CT/MRI-assisted neuronavigation could grant surgeons with better anatomical information (60). This combined technique can minimize the risks related to the operative approaches and pituitary pathologic therapies. Our research suggests that neuronavigation can improve the transition from non-GTR to GTR. The preponderance of iMRI to the GTR transition mainly comes from the superior identification of intrasellar remnants, which is conducive to enhancing EOR, reducing RV, and preventing injury of the surrounding tissues, which increases the safety of operations (16,61,62). In the light of some authors’ viewpoints, low-field iMRI cannot assess parasellar anatomy, cavernous sinus invasion, and small lesions as reliably as high-field iMRI (56). Nonetheless, the results reported in other publications have shown that GTR has been somewhat improved in low-field iMRI (13,14,32,39,63). Although high-field iMRI can likely offer a superior quality of contrast and image resolution to confirm GTR in patients with subtotal resection, low-field iMRI also has relatively high sensitivity (39,64). A potential explanation for this performance is that low-field MRI provides sufficient and precise visualization to identify remnants that need to be further resected. However, those remnants that are unresectable even after ultra-high-field iMRI is not suitable for resection. Neuronavigation utilizes imaging data to enhance the surgeon’s orientation, making it a certainly valuable tool to the inexperienced (65). Significantly, the utilization of neuronavigation could not substitute the clinician’s solid basic knowledge of anatomy and surgical experiences (16).
In clinical practice, there are controversies about the effect of neuronavigation-assisted PitNET resection. Most authors consider that neuronavigation facilitate PitNET surgery and reduce the incidence of complications (28,54,55). It was claimed that this technique was beneficial for decreasing complications, such as apoplexy, deep venous thrombosis, meningitis, pulmonary embolism, cough variant asthma, wound infection, and arterial injury (28). On the contrary, a few authors have reported that the use of iMRI in a considerable number of patients for the visualization of adenoma remnants might not be able to facilitate further safe resection and can even lead to increased complications, hypopituitarism, or postoperative CSF leak (5,24,30). One possible explanation for these results is that their studies involved relatively complex cases, including such of recurrent disease, advanced age, large suprasellar adenoma extension, and comorbid medical conditions (8). Visualization of the fine structure of the medial cavernous sinus border using neuronavigation may be crucial for preserving its integrity and function (66). Giant PitNETs, defined as tumors with the largest diameter of ≥4 cm, remain a therapeutic challenge due to high invasiveness, irregular growth, and postoperative complications (67,68). Neuronavigation can help to protect the carotid arteries and other lateral structures during the resection of Giant PitNETs (67). As for the awkwardly shaped adenomas, the location of the residual adenoma may be detected, but intra-operative difficulties exist, which can prevent achieving complete resection (5). The extent of resection was significantly limited by the maximum diameter and Knosp grade of giant pituitary adenomas and the increase in PitNET removal rates may be linked to the likelihood of encountering postoperative CSF leak (67). Alshareef et al. (55) and Tosaka et al. (56) assumed that neuronavigation has no significant effect on postoperative CSF leak. In conclusion, encountering postoperative CSF leaks may be linked to the likelihood of the increase in PitNET removal rates, especially in giant PitNETs, but being irrelevant to whether neuronavigation is applied. In certain scenarios, a concurrent combined approach offers the potential to enhance the removal of adenoma and reduce the risk of residual adenoma swelling and bleeding (4,69). Additionally, some surgeons advocated for a singular transcranial procedure, which is effective in excising suprasellar adenomas and relieving pressure on the optic nerve (70). However, due to the scarcity of literature on PitNET resection based on neuronavigation utilizing either the transcranial or combined approach, these scenarios were not included in our research. Interestingly, we also investigated a small number of articles about CT-based neuronavigation. CT offers advantages such as the provision of more detailed 3D anatomy than that in the conventional setup and the avoidance of repetitive exposure and accumulation of the staff (60). Although the implementation of this technique places patients at an increased risk of radiation exposure, no acute harm has ever been reported (60).
Our results confirm those of previous research and provides reference for the selection of surgical methods for PitNET resection in future clinical practice. Our analysis has shown that the use of iMRI is beneficial for surgery, regardless of the type of mirror and field strength. Additionally, Pojskić et al. (3) considered that the two mirrors were complementary and recommended that both mirrors could be used to optimize the minimally invasive surgical technique. Regarding the selection of field strength, we found little difference among different MRI field strength subgroups. Our study has some limitations. First, only postoperative CSF leak was analyzed as an operative complication in our meta-analysis. Other complications such as hypopituitarism and intraoperative blood loss were not considered, hindering the comprehensive evaluation of the value of intraoperative neuronavigation in the reduction of complications. Actually, we collected data of various indicators of complications, but due to the limited amount of individual literature data, we did not include them in the statistical analysis. The second major limitation is that only case-control trials were included, allowing us to describe the improvements in GTR and postoperative CSF leak, without considering the implicit biases described above. Although RCTs are the “gold standard” in the evaluation of the effectiveness and safety of an intervention, there is no such research in the field of neuronavigation-assisted PitNET resection. Third, because of a lack of granularity in the data collected and assessed in our systematic review, we could not perform a comprehensive analysis of the sources of heterogeneity, such as gender, age, and funding, which might have exerted a certain adverse impact on the statistical results. Fourth it was also difficult to determine whether the effects of iMRI would be influenced by the adenoma function and adenoma size. Finally, there is insufficient evaluation of neuronavigation in long-term prognosis nowadays, and it has certain value in evaluating the effectiveness of surgery. Interestingly, there was a scintilla of evidence indicating that long-term operation outcomes were associated with early postoperative imaging rather than the intraoperative neuronavigation (16). Therefore, relevant research should be performed in the future.
In conclusion, this systematic review and meta-analysis showed that the use of neuronavigation is important as it leads to an increase in the rate of GTR and a decrease of postoperative CSF leak in PitNET resection. Our study provides reference points for the selection of neuronavigation-assisted surgeries for PitNET resection in the clinical settings.
Acknowledgments
Funding: This work was supported by
Footnote
Reporting Checklist: The authors have completed the PRISMA reporting checklist. Available at https://qims.amegroups.com/article/view/10.21037/qims-23-1570/rc
Conflicts of Interest: All authors have completed the ICMJE uniform disclosure form (available at https://qims.amegroups.com/article/view/10.21037/qims-23-1570/coif). The authors have no conflicts of interest to declare.
Ethical Statement: The authors are accountable for all aspects of the work in ensuring that questions related to the accuracy or integrity of any part of the work are appropriately investigated and resolved.
Open Access Statement: This is an Open Access article distributed in accordance with the Creative Commons Attribution-NonCommercial-NoDerivs 4.0 International License (CC BY-NC-ND 4.0), which permits the non-commercial replication and distribution of the article with the strict proviso that no changes or edits are made and the original work is properly cited (including links to both the formal publication through the relevant DOI and the license). See: https://creativecommons.org/licenses/by-nc-nd/4.0/.
References
- Trifiletti DM, Dutta SW, Lee CC, Sheehan JP. Pituitary Tumor Radiosurgery. Prog Neurol Surg 2019;34:149-58. [Crossref] [PubMed]
- Daly AF, Beckers A. The Epidemiology of Pituitary Adenomas. Endocrinol Metab Clin North Am 2020;49:347-55. [Crossref] [PubMed]
- Pojskić M, Arnautovic A, Kovacevic M, Beckford NS, Qureshi MN, Linder J, Arnautović KI. Combined Microsurgical, Endoscopic and Neuronavigation Assisted Transseptal- Transsphenoidal Resection of Pituitary Tumors. Acta Med Acad 2020;49:14-22. [Crossref] [PubMed]
- Han S, Gao W, Jing Z, Wang Y, Wu A. How to deal with giant pituitary adenomas: transsphenoidal or transcranial, simultaneous or two-staged? J Neurooncol 2017;132:313-21. [Crossref] [PubMed]
- Powell M. The value of intra-operative MRI in trans-sphenoidal pituitary surgery. Acta Neurochir (Wien) 2011;153:1375-6. [Crossref] [PubMed]
- Berkmann S, Fandino J, Zosso S, Killer HE, Remonda L, Landolt H. Intraoperative magnetic resonance imaging and early prognosis for vision after transsphenoidal surgery for sellar lesions. J Neurosurg 2011;115:518-27. [Crossref] [PubMed]
- Hlaváč M, Knoll A, Mayer B, Braun M, Karpel-Massler G, Etzrodt-Walter G, Coburger J, Wirtz CR, Paľa A. Ten years' experience with intraoperative MRI-assisted transsphenoidal pituitary surgery. Neurosurg Focus 2020;48:E14. [Crossref] [PubMed]
- Bohinski RJ, Warnick RE, Gaskill-Shipley MF, Zuccarello M, van Loveren HR, Kormos DW, Tew JM Jr. Intraoperative magnetic resonance imaging to determine the extent of resection of pituitary macroadenomas during transsphenoidal microsurgery. Neurosurgery 2001;49:1133-43; discussion 1143-4. [PubMed]
- Pal'a A, Knoll A, Brand C, Etzrodt-Walter G, Coburger J, Wirtz CR, Hlaváč M. The Value of Intraoperative Magnetic Resonance Imaging in Endoscopic and Microsurgical Transsphenoidal Pituitary Adenoma Resection. World Neurosurg 2017;102:144-50. [Crossref] [PubMed]
- Staartjes VE, Serra C, Maldaner N, Muscas G, Tschopp O, Soyka MB, Holzmann D, Regli L. The Zurich Pituitary Score predicts utility of intraoperative high-field magnetic resonance imaging in transsphenoidal pituitary adenoma surgery. Acta Neurochir (Wien) 2019;161:2107-15. [Crossref] [PubMed]
- Serra C, Burkhardt JK, Esposito G, Bozinov O, Pangalu A, Valavanis A, Holzmann D, Schmid C, Regli L. Pituitary surgery and volumetric assessment of extent of resection: a paradigm shift in the use of intraoperative magnetic resonance imaging. Neurosurg Focus 2016;40:E17. [Crossref] [PubMed]
- Nimsky C, von Keller B, Ganslandt O, Fahlbusch R. Intraoperative high-field magnetic resonance imaging in transsphenoidal surgery of hormonally inactive pituitary macroadenomas. Neurosurgery 2006;59:105-14; discussion 105-14. [Crossref]
- Hlavica M, Bellut D, Lemm D, Schmid C, Bernays RL. Impact of ultra-low-field intraoperative magnetic resonance imaging on extent of resection and frequency of tumor recurrence in 104 surgically treated nonfunctioning pituitary adenomas. World Neurosurg 2013;79:99-109. [Crossref] [PubMed]
- Zaidi HA, De Los Reyes K, Barkhoudarian G, Litvack ZN, Bi WL, Rincon-Torroella J, Mukundan S Jr, Dunn IF, Laws ER Jr. The utility of high-resolution intraoperative MRI in endoscopic transsphenoidal surgery for pituitary macroadenomas: early experience in the Advanced Multimodality Image Guided Operating suite. Neurosurg Focus 2016;40:E18. [Crossref] [PubMed]
- Jones PS, Swearingen B. Intraoperative MRI for Pituitary Adenomas. Neurosurg Clin N Am 2019;30:413-20. [Crossref] [PubMed]
- Soneru CP, Riley CA, Hoffman K, Tabaee A, Schwartz TH. Intra-operative MRI vs endoscopy in achieving gross total resection of pituitary adenomas: a systematic review. Acta Neurochir (Wien) 2019;161:1683-98. [Crossref] [PubMed]
- Moher D, Liberati A, Tetzlaff J, Altman DG. PRISMA Group. Preferred reporting items for systematic reviews and meta-analyses: the PRISMA statement. PLoS Med 2009;6:e1000097. [Crossref] [PubMed]
- Higgins JP, Thompson SG, Deeks JJ, Altman DG. Measuring inconsistency in meta-analyses. BMJ 2003;327:557-60. [Crossref] [PubMed]
- Muka T, Glisic M, Milic J, Verhoog S, Bohlius J, Bramer W, Chowdhury R, Franco OH. A 24-step guide on how to design, conduct, and successfully publish a systematic review and meta-analysis in medical research. Eur J Epidemiol 2020;35:49-60. [Crossref] [PubMed]
- Chittiboina P. iMRI During Transsphenoidal Surgery. Neurosurg Clin N Am 2017;28:499-512. [Crossref] [PubMed]
- Stang A. Critical evaluation of the Newcastle-Ottawa scale for the assessment of the quality of nonrandomized studies in meta-analyses. Eur J Epidemiol 2010;25:603-5. [Crossref] [PubMed]
- Fahmy O, Fahmy UA, Alhakamy NA, Khairul-Asri MG. Single-Port versus Multiple-Port Robot-Assisted Radical Prostatectomy: A Systematic Review and Meta-Analysis. J Clin Med 2021;10:5723. [Crossref] [PubMed]
- Chen X, Xu BN, Meng X, Zhang J, Yu X, Zhou D. Dual-room 1.5-T intraoperative magnetic resonance imaging suite with a movable magnet: implementation and preliminary experience. Neurosurg Rev 2012;35:95-109; discussion 109-10. [Crossref] [PubMed]
- Chung TK, Riley KO, Woodworth BA. The use of image-guidance during transsphenoidal pituitary surgery in the United States. Am J Rhinol Allergy 2015;29:215-20. [Crossref] [PubMed]
- Nimsky C, Ganslandt O, Von Keller B, Romstöck J, Fahlbusch R. Intraoperative high-field-strength MR imaging: implementation and experience in 200 patients. Radiology 2004;233:67-78. [Crossref] [PubMed]
- Netuka D, Masopust V, Belšán T, Kramář F, Beneš V. One year experience with 3.0 T intraoperative MRI in pituitary surgery. Acta Neurochir Suppl 2011;109:157-9. [Crossref] [PubMed]
- Qiu TM, Yao CJ, Wu JS, Pan ZG, Zhuang DX, Xu G, Zhu FP, Lu JF, Gong X, Zhang J, Yang Z, Shi JB, Huang FP, Mao Y, Zhou LF. Clinical experience of 3T intraoperative magnetic resonance imaging integrated neurosurgical suite in Shanghai Huashan Hospital. Chin Med J (Engl) 2012;125:4328-33. [PubMed]
- Eboli P, Shafa B, Mayberg M. Intraoperative computed tomography registration and electromagnetic neuronavigation for transsphenoidal pituitary surgery: accuracy and time effectiveness. J Neurosurg 2011;114:329-35. [Crossref] [PubMed]
- Paterno' V, Fahlbusch R. High-Field iMRI in transsphenoidal pituitary adenoma surgery with special respect to typical localization of residual tumor. Acta Neurochir (Wien) 2014;156:463-74; discussion 474. [Crossref] [PubMed]
- Sylvester PT, Evans JA, Zipfel GJ, Chole RA, Uppaluri R, Haughey BH, Getz AE, Silverstein J, Rich KM, Kim AH, Dacey RG, Chicoine MR. Combined high-field intraoperative magnetic resonance imaging and endoscopy increase extent of resection and progression-free survival for pituitary adenomas. Pituitary 2015;18:72-85. [Crossref] [PubMed]
- Ahn JY, Jung JY, Kim J, Lee KS, Kim SH. How to overcome the limitations to determine the resection margin of pituitary tumours with low-field intra-operative MRI during trans-sphenoidal surgery: usefulness of Gadolinium-soaked cotton pledgets. Acta Neurochir (Wien) 2008;150:763-71; discussion 771. [Crossref] [PubMed]
- Berkmann S, Fandino J, Müller B, Remonda L, Landolt H. Intraoperative MRI and endocrinological outcome of transsphenoidal surgery for non-functioning pituitary adenoma. Acta Neurochir (Wien) 2012;154:639-47. [Crossref] [PubMed]
- Fahlbusch R, Ganslandt O, Buchfelder M, Schott W, Nimsky C. Intraoperative magnetic resonance imaging during transsphenoidal surgery. J Neurosurg 2001;95:381-90. [Crossref] [PubMed]
- García S, Reyes L, Roldán P, Torales J, Halperin I, Hanzu F, Langdon C, Alobid I, Enseñat J. Does Low-Field Intraoperative Magnetic Resonance Improve the Results of Endoscopic Pituitary Surgery? Experience of the Implementation of a New Device in a Referral Center. World Neurosurg 2017;102:102-10. [Crossref] [PubMed]
- Jiménez P, Brell M, Sarriá-Echegaray P, Roldán P, Tomás-Barberán M, Ibáñez J. "Intrasellar Balloon Technique" in intraoperative MRI guided transsphenoidal endoscopic surgery for sellar region tumors. Usefulness on image interpretation and extent of resection evaluation. Technical note. Acta Neurochir (Wien) 2016;158:445-9. [Crossref] [PubMed]
- Martin CH, Schwartz R, Jolesz F, Black PM. Transsphenoidal resection of pituitary adenomas in an intraoperative MRI unit. Pituitary 1999;2:155-62. [Crossref] [PubMed]
- Ramm-Pettersen J, Berg-Johnsen J, Hol PK, Roy S, Bollerslev J, Schreiner T, Helseth E. Intra-operative MRI facilitates tumour resection during trans-sphenoidal surgery for pituitary adenomas. Acta Neurochir (Wien) 2011;153:1367-73. [Crossref] [PubMed]
- Schwartz TH, Stieg PE, Anand VK. Endoscopic transsphenoidal pituitary surgery with intraoperative magnetic resonance imaging. Neurosurgery 2006;58:ONS44-51; discussion ONS44-51. [PubMed]
- Strange F, Remonda L, Schütz P, Fandino J, Berkmann S. 10 Years' Experience of Using Low-Field Intraoperative MRI in Transsphenoidal Surgery for Pituitary Adenoma: Results of the Swiss Pituitary Registry (SwissPit). World Neurosurg 2020;136:e284-93. [Crossref] [PubMed]
- Wu JS, Shou XF, Yao CJ, Wang YF, Zhuang DX, Mao Y, Li SQ, Zhou LF. Transsphenoidal pituitary macroadenomas resection guided by PoleStar N20 low-field intraoperative magnetic resonance imaging: comparison with early postoperative high-field magnetic resonance imaging. Neurosurgery 2009;65:63-70; discussion 70-1. [Crossref] [PubMed]
- Berkmann S, Schlaffer S, Nimsky C, Fahlbusch R, Buchfelder M. Follow-up and long-term outcome of nonfunctioning pituitary adenoma operated by transsphenoidal surgery with intraoperative high-field magnetic resonance imaging. Acta Neurochir (Wien) 2014;156:2233-43; discussion 2243. [Crossref] [PubMed]
- Dort JC, Sutherland GR. Intraoperative magnetic resonance imaging for skull base surgery. Laryngoscope 2001;111:1570-5. [Crossref] [PubMed]
- Gohla G, Bender B, Tatagiba M, Honegger J, Ernemann U, Roder C. Identification of tumor residuals in pituitary adenoma surgery with intraoperative MRI: do we need gadolinium? Neurosurg Rev 2020;43:1623-9. [Crossref] [PubMed]
- Hlaváč M, Knoll A, Etzrodt-Walter G, Sommer F, Scheithauer M, Coburger J, Wirtz CR, Pala A. Intraoperative MRI in transsphenoidal resection of invasive pituitary macroadenomas. Neurosurg Rev 2019;42:737-43. [Crossref] [PubMed]
- Kuge A, Kikuchi Z, Sato S, Sakurada K, Takemura S, Kayama T. Practical use of a simple technique, insertion of wet cotton pledgets into the tumor resection cavity in transsphenoidal surgery of pituitary tumors, for a better comparison between pre- and intraoperative high-field magnetic resonance images. J Neurol Surg A Cent Eur Neurosurg 2013;74:366-72. [Crossref] [PubMed]
- Li J, Cong Z, Ji X, Wang X, Hu Z, Jia Y, Wang H. Application of intraoperative magnetic resonance imaging in large invasive pituitary adenoma surgery. Asian J Surg 2015;38:168-73. [Crossref] [PubMed]
- Paľa A, Etzrodt-Walter G, Karpel-Massler G, Pedro MT, Mayer B, Coburger J, Wirtz CR, Hlaváč M. Characterization of tumor remnants in intraoperative MRI-assisted microscopic and endoscopic transsphenoidal resection of less invasive pituitary adenomas. Neurosurg Rev 2022;45:1701-8. [Crossref] [PubMed]
- Pala A, Knoll A, Schneider M, Etzrodt-Walter G, Karpel-Massler G, Wirtz CR, Hlavac M. The Benefit of Intraoperative Magnetic Resonance Imaging in Endoscopic and Microscopic Transsphenoidal Resection of Recurrent Pituitary Adenomas. Curr Oncol 2022;29:392-401. [Crossref] [PubMed]
- Szerlip NJ, Zhang YC, Placantonakis DG, Goldman M, Colevas KB, Rubin DG, Kobylarz EJ, Karimi S, Girotra M, Tabar V. Transsphenoidal resection of sellar tumors using high-field intraoperative magnetic resonance imaging. Skull Base 2011;21:223-32. [Crossref] [PubMed]
- Tanei T, Nagatani T, Nakahara N, Watanabe T, Nishihata T, Nielsen ML, Takebayashi S, Hirano M, Wakabayashi T. Use of high-field intraoperative magnetic resonance imaging during endoscopic transsphenoidal surgery for functioning pituitary microadenomas and small adenomas located in the intrasellar region. Neurol Med Chir (Tokyo) 2013;53:501-10. [Crossref] [PubMed]
- Zhang H, Wang F, Zhou T, Wang P, Chen X, Zhang J, Zhou D. Analysis of 137 Patients Who Underwent Endoscopic Transsphenoidal Pituitary Adenoma Resection Under High-Field Intraoperative Magnetic Resonance Imaging Navigation. World Neurosurg 2017;104:802-15. [Crossref] [PubMed]
- Zhang Z, Yang K, Xia Y, Meng X, Yu X. High-Field Intraoperative Magnetic Resonance Imaging Increases Extent of Resection and Progression-Free Survival for Nonfunctioning Pituitary Adenomas. World Neurosurg 2019;127:e925-31. [Crossref] [PubMed]
- Fomekong E, Duprez T, Docquier MA, Ntsambi G, Maiter D, Raftopoulos C. Intraoperative 3T MRI for pituitary macroadenoma resection: Initial experience in 73 consecutive patients. Clin Neurol Neurosurg 2014;126:143-9. [Crossref] [PubMed]
- Achey RL, Karsy M, Azab MA, Scoville J, Kundu B, Bowers CA, Couldwell WT. Improved Surgical Safety via Intraoperative Navigation for Transnasal Transsphenoidal Resection of Pituitary Adenomas. J Neurol Surg B Skull Base 2019;80:626-31. [Crossref] [PubMed]
- Alshareef M, Lowe S, Park Y, Frankel B. Utility of intraoperative ultrasonography for resection of pituitary adenomas: a comparative retrospective study. Acta Neurochir (Wien) 2021;163:1725-34. [Crossref] [PubMed]
- Tosaka M, Nagaki T, Honda F, Takahashi K, Yoshimoto Y. Multi-slice computed tomography-assisted endoscopic transsphenoidal surgery for pituitary macroadenoma: a comparison with conventional microscopic transsphenoidal surgery. Neurol Res 2015;37:951-8. [Crossref] [PubMed]
- Szerlip NJ, Zhang YC, Placantonakis DG, Goldman M, Colevas KB, Rubin DG, Kobylarz EJ, Karimi S, Girotra M, Tabar V. Transsphenoidal resection of sellar tumors using high-field intraoperative magnetic resonance imaging. Skull Base 2011;21:223-32. [Crossref] [PubMed]
- Staartjes VE, Togni-Pogliorini A, Stumpo V, Serra C, Regli L. Impact of intraoperative magnetic resonance imaging on gross total resection, extent of resection, and residual tumor volume in pituitary surgery: systematic review and meta-analysis. Pituitary 2021;24:644-56. [Crossref] [PubMed]
- Zhang JJY, Lim JX, Lee KS, Kirollos RW, Rao JP. Impact of Intraoperative Magnetic Resonance Imaging on Short-Term and Long-Term Outcomes After Transsphenoidal Resection of Pituitary Adenoma: A Systematic Review and Meta-Analysis. World Neurosurg 2022;167:184-194.e16. [Crossref] [PubMed]
- Ulmer S, Schulz E, Moeller B, Krause UR, Nabavi A, Mehdorn HM, Jansen O. Radiation dose of the lens in trans-sphenoidal pituitary surgery: pros and cons of a conventional setup using fluoroscopic guidance and CT-based neuronavigation. AJNR Am J Neuroradiol 2007;28:1559-64. [Crossref] [PubMed]
- Koc K, Anik I, Ozdamar D, Cabuk B, Keskin G, Ceylan S. The learning curve in endoscopic pituitary surgery and our experience. Neurosurg Rev 2006;29:298-305; discussion 305. [Crossref] [PubMed]
- Lasio G, Ferroli P, Felisati G, Broggi G. Image-guided endoscopic transnasal removal of recurrent pituitary adenomas. Neurosurgery 2002;51:132-6; discussion 136-7. [Crossref] [PubMed]
- Buchfelder M, Schlaffer SM. Intraoperative magnetic resonance imaging during surgery for pituitary adenomas: pros and cons. Endocrine 2012;42:483-95. [Crossref] [PubMed]
- Fahlbusch R. Keller Bv, Ganslandt O, Kreutzer J, Nimsky C. Transsphenoidal surgery in acromegaly investigated by intraoperative high-field magnetic resonance imaging. Eur J Endocrinol 2005;153:239-48. [Crossref] [PubMed]
- Buchfelder M, Schlaffer SM. Novel Techniques in the Surgical Treatment of Acromegaly: Applications and Efficacy. Neuroendocrinology 2016;103:32-41. [Crossref] [PubMed]
- Wolfsberger S, Ba-Ssalamah A, Pinker K, Mlynárik V, Czech T, Knosp E, Trattnig S. Application of three-tesla magnetic resonance imaging for diagnosis and surgery of sellar lesions. J Neurosurg 2004;100:278-86. [Crossref] [PubMed]
- Chen Y, Xu X, Cao J, Jie Y, Wang L, Cai F, Chen S, Yan W, Hong Y, Zhang J, Wu Q. Transsphenoidal Surgery of Giant Pituitary Adenoma: Results and Experience of 239 Cases in A Single Center. Front Endocrinol (Lausanne) 2022;13:879702. [Crossref] [PubMed]
- Raverot G, Jouanneau E, Trouillas J. Management of endocrine disease: clinicopathological classification and molecular markers of pituitary tumours for personalized therapeutic strategies. Eur J Endocrinol 2014;170:R121-32. [Crossref] [PubMed]
- Dehdashti AR, Ganna A, Karabatsou K, Gentili F. Pure endoscopic endonasal approach for pituitary adenomas: early surgical results in 200 patients and comparison with previous microsurgical series. Neurosurgery 2008;62:1006-15; discussion 1015-7. [PubMed]
- Youssef AS, Agazzi S, van Loveren HR. Transcranial surgery for pituitary adenomas. Neurosurgery 2005;57:168-75; discussion 168-75. [PubMed]