The effectiveness of magnetic resonance imaging (MRI) iron corrected T1 in monitoring metabolic dysfunction-associated steatohepatitis in obesity following bariatric surgery and lifestyle modification: a prospective cohort study
Introduction
Metabolic dysfunction-associated steatotic liver disease (MASLD), previously non-alcoholic fatty liver disease (NAFLD) is a common cause of chronic liver disease. It affects approximately 32% of adults worldwide (1), and around 75% in people with obesity (2). MASLD can progress from simple steatosis to metabolic dysfunction-associated steatohepatitis (MASH), a condition characterized by hepatocellular ballooning (liver cell damage) and inflammation that can lead to severe liver diseases such as fibrosis, cirrhosis, liver failure, and cancer (3). MASH also increases the risk of cardiovascular disease (3) and is projected to affect 27 million individuals in the USA alone by 2030 (4). Globally, MASH is estimated to affect 33.5% of individuals with overweight and obesity (2), in which about 60% of them require liver biopsy (5).
Early diagnosis, timely intervention, and monitoring response are significantly important in managing MASH before advanced fibrosis sets in. Currently, no approved drugs are available for treating MASH (6), so weight loss strategies are the mainstay of management (7). Common weight loss interventions like bariatric surgery and lifestyle modification have been shown to achieve remission of MASLD and MASH, although with varying magnitudes (8,9). Lassailly et al. (9) found that five years post bariatric surgery, 84% of patients had MASH resolution without fibrosis worsening, 70.2% experienced fibrosis reduction, and it disappeared in 56% of patients, including 45.5% of patients with baseline fibrosis. They also recently reported that 15 years post bariatric surgery, 95% of patients with MASH resolution had fibrosis regression (10).
One of the impediments in managing MASH is the lack of non-invasive biomarkers to track the progression or regression of the disease. Liver biopsy is the current clinical standard for diagnosing MASH, but it has limitations due to its invasive nature, potential for sampling errors, observer variability, rare but potentially fatal complications, and cost (11-13). This highlights the need for reliable, non-invasive alternatives for diagnosing this condition. Quantitative multiparametric magnetic resonance imaging (MRI) offers non-invasive metrics that can objectively assess and monitor liver tissue characteristics. Proton density fat fraction (PDFF) is a reliable and accurate measure for quantifying liver fat and identifying patients with MASLD (14,15). However, PDFF is not an effective biomarker for determining disease severity or identifying MASH patients with significant fibrosis as liver fat diminishes with the increase in fibrosis (16). Iron corrected T1 (cT1) mapping has shown a correlation with fibroinflammatory activity in biopsy samples and has proven to be highly accurate in diagnosing patients with MASLD, MASH, and MASH with advanced stages of fibrosis (16-20). However, very few studies have applied this biomarker following weight loss interventions. Therefore, this study aimed to evaluate differences in liver response to bariatric surgery and lifestyle modification using disease activity cT1 and PDFF in patients with both obesity and MASLD. We present this article in accordance with the STROBE reporting checklist (available at https://qims.amegroups.com/article/view/10.21037/qims-24-148/rc).
Methods
Study participants
This longitudinal study was conducted between March 2019 to February 2022. Forty patients were prospectively recruited after fulfilling the selection criteria. Of these, 34 patients divided into two groups [bariatric surgery- laparoscopic sleeve gastrectomy (LSG) (n=13) and lifestyle modification (n=21)] completed the study (Figure 1). The lifestyle modification program (LMP) group was composed of patients who were not willing to undergo LSG procedure and were invited to participate in the LMP. The study was conducted in accordance with the Declaration of Helsinki (as revised in 2013) and complied with the Hospital Authority Guide on Research Ethics. The study was approved by the joint Chinese University of Hong Kong-New Territories East Cluster Clinical Research Ethics Committee (ethical approval number: 2018.612) and all participants provided written informed consent.
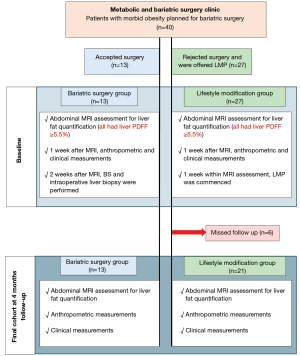
Selection criteria
Inclusion criteria: age 18–65 years, Chinese ethnicity, body mass index (BMI) ≥28 kg/m2 [adjusted criteria for Asian population (21)], with a diagnosis of MASLD based on MRI PDFF ≥5.5% (22) and any one of the following metabolic factors as defined by Rinella et al. (23)]: (I) waist circumference ≥90 cm in Asian men and ≥80 cm in Asian women- ethnically adjusted, (II) fasting serum glucose ≥5.6 mmol/L (100 mg/dL) or 2-h post-load glucose levels ≥7.8 mmol/L (≥140 mg/dL) or glycosylated haemoglobin (HbA1C) ≥5.7% (39 mmol/L) or type 2 diabetes or treatment for type 2 diabetes, (III) blood pressure >130/85 mmHg or specific antihypertensive drug treatment, (IV) plasma triglyceride ≥1.70 mmol/L (150 mg/dL) or lipid lowering treatment and (V) plasma HDL-cholesterol ≤1.0 mmol/L (40 mg/dL) in men and ≤1.3 mmol/L (50 mg/dL) in women or lipid lowering treatment.
Exclusion criteria: any contraindications to MRI, other kind of hepatic diseases or under medications known to affect liver fat accumulation, excessive alcohol consumption (>30 g/d for men and >20 g/d for women), body weight >250 kg and/or waist circumference >150 cm (unable to fit into the MRI scanner).
Clinical assessment
All patients received their anthropometric measurements, biochemical evaluations, dietary assessment based on 3-day diet record and power of food scale at two time points: baseline and four months. The patients’ medical history was recorded.
Clinical and anthropomeric measurements
Anthropometric measurements including body weight, body height, waist circumferences, diastolic and systolic blood pressures were recorded. BMI was calculated as weight in kilograms divided by height in meter squared (m2). Blood tests including liver enzymes, glucose, insulin, and lipids were conducted after 8 hours of fasting, and within 7 days from MRI examination.
Insulin resistance/type 2 diabetes mellitus (T2DM) status
Insulin resistance was estimated using the homeostasis model assessment-insulin resistance (HOMA-IR), calculated as HOMA-IR = fasting plasma glucose (mmol/L) × insulin (mIU/L)/22.5 (24). Insulin resistance was defined as HOMA-IR ≥1.4 in non-diabetic patients and ≥2.0 in diabetic patients (25). The diagnosis of T2DM was based on the criteria set by World health Organization as (26): fasting glucose in whole venous blood ≥6.1 mmol/L or fasting glucose in venous plasma ≥7.0 mmol/L or 2-hour post glucose load in whole venous blood ≥10.0 mmol/L or 2-hour post glucose load in venous plasma ≥11.1 mmol/L.
Metabolic syndrome
Metabolic syndrome was defined using the harmonized criteria (27), i.e., the presence of at least any three of five of the following: (I) central obesity (waist circumference ≥90 cm in Asian men and ≥80 cm in Asian women); (II) triglycerides ≥1.7 mmol/L; (III) reduced high-density lipoprotein-cholesterol (<1.0 mmol/L in men and <1.3 mmol/L in women); (IV) blood pressure ≥130/85 mmHg; and (V) fasting plasma glucose ≥5.6 mmol/L, or receiving treatment for any of the above metabolic abnormalities.
Bariatric surgery
The LSG procedure was performed by two experienced bariatric surgeons (30 and 29 years of experience respectively) following the department’s standard protocols within 2 weeks of MRI liver fat assessment. All patients underwent routine follow-ups in line with the department’s standard treatment guidelines. Written consent was obtained from each subject. During the procedure, an intraoperative liver biopsy was performed using a 16 G × 15 cm Temno bevel tip needle. Each biopsied liver tissue specimen was preserved in a formalin solution, with a median specimen length of 1.25 cm (ranging from 0.3 to 1.9 cm).
Histological assessment
A single expert pathologist with 31 years of experience examined the specimens following the department’s standard protocol. Using the NASH Clinical Research Network (CRN) NAFLD activity score and fibrosis staging (3), the Non-alcoholic steatohepatitis activity score (NAS: 0–8) was obtained as follows: hepatic steatosis (grade 0–3), lobular inflammation (grade 0–3), and ballooning (grade 0–2). MASH was defined as NAS activity score ≥4 (in the presence of ballooning ≥1 and lobular inflammation ≥1). Liver fibrosis staging (stage 0–4) was also conducted using the Brunt’s fibrosis grading scale.
LMP
Patients in this group participated in a 4-month dietitian-led LMP. This intervention was focused on both improving overall health and reduction of weight rather than just weight loss. Thus, it was designed to reduce body weight by 5–10% and achieve a balanced macronutrient distribution to around 45–65% of carbohydrates, 20–35% of total fat and 10–35% of proteins. Generally, the Mediterranean diet was recommended as it has been shown to be associated with improvement in liver steatosis and fibrosis among many other benefits (28,29). Also, patients were encouraged to do aerobic exercises two to three times per week. Patients had weekly individual consultations for the first two months and monthly for the remainder. The dietitian assessed behaviours and eating patterns and provided guidance. Each participant received a personalized menu plan according to the American Dietetic Association standards (30). The diet emphasized on fruits and vegetables, low fat, moderate carbohydrates, and low-glycaemic index foods.
MRI data acquisition and analysis
All patients underwent MRI at baseline and at 4 months. All scanning was performed using a Philips Achieva 3.0T MRI Scanner (Philips Medical System, Best, The Netherlands) equipped with a 16-channel SENSE-XL-Torso array coil. The patients had to fast for at least 8 hours before the examination.
Chemical shift encoded abdominal imaging
Chemical-shift water-fat images were acquired with a three-dimensional (3D) spoiled multi-echo mDIXON sequence to yield co-registered water, fat, fat-fraction, and T2* image series. Three sections of 15 seconds breath-hold acquisition each, of the entire abdomen from the xiphoid process to the pubic symphysis were acquired. Imaging parameters were as follow: repetition time (TR) =5.7–5.9 (ms), echo time (TE)/echo spacing =1.2–1.4 (ms)/1.0–1.2 (ms), number of echoes =6, flip angle =3°, SENSE acceleration =2, reconstructed slice thickness/number of slices =4.0 mm/50, ACQ matrix =128×117.
LiverMultiScan was also performed using an electrocardiogram (ECG) triggered Shortened Modified Look Locker Inversion (shMOLLI) sequence to obtain corrected T1 values from four cross-sectional images at the porta hepatis. The parameters were: TR =2.4 ms, TE =1.05 ms, flip angle =35°, acquisition matrix =192×144, field of view =440×330 mm2, SENSE acceleration =2, slice thickness =8 mm.
Liver cT1/T2* calculation
Liver MR data were post-processed using LiverMultiScan® (Oxford, UK) protocol (31). Briefly, cT1 and PDFF maps of the liver were outlined into comprehensive liver segmentation maps using a semi-automatic method as described by Bachtiar et al. (32). Three circular regions of interest, each 15mm in diameter, were placed on the cross-sectional T2 maps for each slice. These covered a representative sample of the liver and were used to calculate average T2* values for T1 correction. Structures that were not part of the liver parenchyma, such as bile ducts and large blood vessels, as well as image artifacts, were not included in the image analysis. All image analysts were blinded to clinical data.
Subcutaneous (SAT) and visceral adipose (VAT) tissue quantification
SAT and VAT tissue volumes were measured from the dome of the diaphragm to the pubic symphysis from the PDFF image series using an in-house method developed by Hui et al. (33). Briefly, this in-house algorithm detects and removes the narrow connecting regions between SAT and VAT using a spoke-like template constructed by Bresenham’s Line and Midpoint Circle method, which was applied over the adipose tissue to automatically separate SAT and VAT.
Statistical analysis
Given the small sample size in our study, we performed a power analysis to confirm the validity of the study outcomes. We first calculated the Cohen’s d using change in cT1 values between baseline and 4 months, yielding an effect size of 7.19107. This, along with the known sample sizes (LSG =13 and LMP =21) and β/α ratio (0.62), allowed us to achieve a statistical power (1-β err prob) of 0.82. Therefore, with an effect size of 7.2 and a statistical power of 82%, the results presented are supported as statistically and practically significant.
All continuous variables were expressed as median (range) unless stated otherwise. Categorical variables were expressed as number (percentage). Comparisons between two groups were analysed using Mann-Whitney and Fisher’s exact tests accordingly. Wilcoxon’s test and McNemar’s test were used to compare matched data accordingly. Correlations between variables were analysed with Spearman’s correlation coefficient. Area under receiver operating characteristic curve (AUROC) was used to test the diagnostic performance of variables. This study adopted the per protocol analysis, thus, all subjects who were lost at follow-up were excluded in the final analysis. All tests were two sided and P values <0.05 were considered statistically significant. Statistical analyses were performed with SPSS software, version 28.0 (IBM, Chicago, IL, USA).
Results
Baseline
A total of 34 patients completed the study-LSG =13 and LMP =21 as 6 missed the 4 months follow up assessment. From the whole study cohort, 21 (61.8%) were female, median age 45 years; BMI 36 kg/m2. All 34 (100%) had MASLD on MRI, 31 (91.2%) had insulin resistance, 26 (76.5%) had T2DM, and 28 (82.4%) had metabolic syndrome. eighteen (52.9%) were on lipid lowering drugs, 25 (73.5%) on antidiabetics, and 19 (55.9%) were on antihypertensives. Only the number of subjects taking lipid lowering drugs (P=0.010) was significantly different among all measured clinical parameters between interventions. Details are shown in Table 1.
Table 1
Variables, n (%) | All (n=34) | LSG (n=13) | LMP (n=21) | P value |
---|---|---|---|---|
Sex, male/female | 13 (38.2)/21 (61.8) | 5 (38.5)/8 (61.5) | 8 (38.1)/13 (61.9) | >0.99 |
Insulin resistance | 31 (91.2) | 12 (92.3) | 19 (90.5) | 0.406 |
Diabetes | 26 (76.5) | 8 (61.5) | 18 (85.7) | 0.211 |
Antidiabetic drug use | 25 (73.5) | 8 (61.5) | 17 (81) | 0.379 |
Hyperglycaemia | 26 (76.5) | 10 (76.9) | 16 (76.2) | >0.99 |
Hypertension | 26 (76.5) | 8 (61.5) | 17 (81) | 0.254 |
Antihypertensive drug use | 19 (55.9) | 6 (46.2) | 13 (61.9) | 0.473 |
Dyslipidaemia | 10 (29.4) | 4 (30.8) | 6 (28.6) | >0.99 |
Lipid lowering drug use | 18 (52.9) | 3 (23.1) | 15 (71.4) | 0.010 |
Hypoalphalipoproteinemia | 17 (50) | 5 (38.5) | 12 (57.1) | 0.481 |
Metabolic syndrome | 28 (82.4) | 9 (69.2) | 19 (90.5) | 0.173 |
MASLD | 34 (100) | 13 (100) | 21 (100) | >0.99 |
Fisher’s exact test was used. LSG, laparoscopic sleeve gastrectomy; LMP, lifestyle modification program; MASLD, metabolic dysfunction-associated steatotic liver disease.
Effects of LSG and LMP on anthropometric and clinical parameters at 4 months
In the LSG group, patients showed a median percentage weight loss of −22.8% (1st quartile of −25.39 and 3rd quartile of −14.02), P=0.013. This percentage weight loss resulted in significant improvement (reduction) in BMI (P=0.019), waist circumference (P=0.007), triglycerides (P=0.023), glucose (P=0.05), insulin (P=0.034), HOMA-IR (P=0.026), HbA1c (P=0.012), alanine transaminase (ALT) (P=0.003), aspartate transaminase (AST) (P=0.009), and gamma-glutamyl transferase (GGT) (P=0.002) as detailed in Table 2.
Table 2
Characteristics | LSG (n=13) | LMP (n=21) | P value (LSG vs. LMP)† | |||||||
---|---|---|---|---|---|---|---|---|---|---|
Baseline | 4 months | P value‡ | Baseline | 4 months | P value‡ | At baseline | At 4 months | |||
Age (years) | 40 (28–51) | – | – | 48 (35–52) | – | – | 0.004 | – | ||
Weight (kg) | 103.8 (79.7–131.1) | 88.6 (64.9–110.3) | 0.013 | 91.8 (66.4–127.4) | 89.2 (79.9–108.6) | 0.262 | 0.052 | 0.901 | ||
BMI (kg/m2) | 37.29 (28.8–44.6) | 29.32 (23.7–51.8) | 0.019 | 35.1 (28.3–42.1) | 33.4 (28.2–40.3) | 0.203 | 0.485 | 0.038 | ||
Waist circumference (cm) | 115 (97–127) | 105 (94–113) | 0.007 | 112 (95–124) | 107 (92–123) | 0.139 | 0.367 | 0.390 | ||
Systolic blood pressure (mmHg) | 126 (102–140) | 123 (109–172) | 0.834 | 127 (100–143) | 126 (115–165) | 0.263 | 0.681 | 0.549 | ||
Diastolic blood pressure (mmHg) | 73 (57–87) | 76 (60–84) | 0.462 | 83 (67–138) | 80 (71–113) | 0.593 | 0.032 | 0.194 | ||
Total cholesterol (mmol/L) | 4.4 (0.6–6.2) | 4.7 (3.1–6.6) | 0.307 | 3.8 (2.8–6.0) | 4.6 (2.9–7.2) | 0.066 | 0.162 | 0.817 | ||
Triglycerides (mmol/L) | 1.5 (0.7–5.0) | 1.1 (0.5–1.6) | 0.023 | 1.5 (0.7–5.0) | 1.9 (0.8–2.8) | 0.474 | 0.563 | 0.020 | ||
HDL-c (mmol/L) | 1.2 (0.8–1.5) | 1.3 (0.9–1.7) | 0.235 | 1.2 (0.8–1.5) | 1.2 (0.8–1.6) | 0.135 | 0.927 | 0.219 | ||
Fasting blood glucose (mmol/L) | 6.4 (3.9–11) | 4.8 (4.1–7.7) | 0.050 | 6.4 (3.9–11) | 5.7 (4.7–10.8) | 0.610 | 0.903 | 0.060 | ||
Fasting plasma insulin (mIU/L) | 27.4 (5.2–64.5) | 13 (4.2–46.3) | 0.034 | 27.4 (5.2–64.5) | 30.8 (14.9–91) | 0.285 | 0.458 | 0.003 | ||
HbA1C (mmol/L) | 6.5 (5–11.7) | 5.5 (4.6–8.0) | 0.012 | 6.5 (5–11.7) | 6.1 (4.8–7.6) | 0.413 | 0.728 | 0.082 | ||
ALP (IU/L) | 83.5 (40–161) | 81 (42–107) | 0.916 | 55.5 (3.2–102) | 63 (40–78) | 0.422 | 0.005 | 0.014 | ||
ALT (IU/L) | 39 (18–132) | 17 (12–49) | 0.003 | 32 (13–66) | 28 (20–99) | 0.593 | 0.125 | 0.020 | ||
AST (IU/L) | 28 (5.2–96) | 21.5 (12–31) | 0.009 | 31 (18–51) | 24 920–55) | 0.233 | 0.874 | 0.126 | ||
AST/ALT ratio | 0.65 (0.19–1.11) | 1.0 (0.63–1.71) | 0.019 | 0.86 (0.58–1.68) | 0.87 (0.72–1.77) | 0.953 | 0.004 | 0.972 | ||
GGT (IU/L) | 48 (2.8–80) | 21 (10–31) | 0.002 | 32 (2.8–166) | 33 (17–127) | 0.953 | 0.188 | 0.009 | ||
Albumin | 39.5 (5–43) | 38 (8–43) | 0.195 | 39.5 (34–44) | 40 (34–46) | 0.196 | 0.714 | 0.153 | ||
HOMA-IR | 6.03 (2.58–20.53) | 3.29 (0.99–9.05) | 0.026 | 7.89 (1.02–30.75) | 8.41 (3.18–25.08) | 0.721 | 0.167 | 0.002 | ||
MRI-PDFF (%) | 12.8 (5.50–28.40) | 3.0 (1.8–4.5) | 0.012 | 12.7 (5.5–28.40) | 8.9 (5.8–21.9) | 0.012 | 0.386 | <0.001 | ||
MRI-cT1 (ms) | 910 (725–987) | 862 (719–873) | 0.025 | 826 (665–1062) | 907 (770–986) | 0.083 | 0.309 | 0.100 | ||
MRI- T2* (ms) | 20 (12–23.90) | 22.4 (11.8–29.3) | 0.017 | 19.3 (10–29.3) | 21.9 (18.4–24.6) | 0.075 | 0.738 | 0.563 | ||
VAT (litres) | 3.94 (2.77–5.88) | 4.14 (2.75–5.39) | 0.499 | 4.53 (3.04–6.67) | 4.52 (2.74–11.58) | 0.799 | 0.525 | 0.261 | ||
SAT (litres) | 26.06 (16.92–40.04) |
12.9 (23.6) | 0.018 | 21.09 (12.12–29.47) |
22.93 (12.80–30.29) |
0.241 | 0.031 | 0.032 | ||
VAT/SAT ratio | 0.16 (0.10–0.24) | 0.28 (0.17–0.33) | 0.017 | 0.20 (0.13–0.55) | 0.21 (0.11–0.41) | 0.878 | 0.011 | 0.097 | ||
Percentage weight loss* (%) | – | −22.8 (−29.39 to −14.02) |
– | – | −1.31 (−3.22 to 1.10) |
– | – | <0.001 | ||
Percentage PDFF loss* (%) | – | −64.52 (−84.15 to −62.71) |
– | – | −29.16 (−45.68 to −11.31) |
– | – | 0.001 | ||
Percentage cT1 change* (%) | – | −8.04 (−10.37 to −0.81) |
– | – | −3.87 (−8.49 to 1.32) |
– | – | 0.374 | ||
Percentage T2* change* (%) | – | 6.48 (2.15–40.19) |
– | – | 9.05 (1.11–15.71) |
– | – | 0.711 | ||
Percentage VAT loss* (%) | – | 12.71 (−20.41 to 15.48) |
– | – | −0.67 (−6.95 to −0.67) |
– | – | 0.696 | ||
Percentage SAT loss* (%) | – | −39.97 (−61.27 to −24.57) |
– | – | 6.72 (−9.51 to 25.87) |
– | – | <0.001 | ||
Number of subjects with cT1 increase | – | 0 | – | – | 3 (14) | – | – | – | ||
Presence of MASH using cT1 cut off value of 925 ms | 4 (31) | 0 | 0.250§ | 7 (33) | 3 (14) | 0.500§ | 1.000¶ | 0.228¶ |
*, data are presented as median (1st quartile and 3rd quartile). The rest of the data is presented as median (range) or n (%). P value between baseline and 4 months: ‡, Wilcoxon test; §, McNemar’s test. P value between LSG and LMP group at baseline and 4 months follow-up: †, Mann-Whitney U test; ¶, Fisher’s exact test. LSG, laparoscopic sleeve gastrectomy group; LMP, lifestyle modification program; BMI, body mass index; HDL, high density lipoprotein; HbA1c, glycosylated haemoglobin; ALP, alkaline phosphatase; ALT, alanine aminotransferase; AST, aspartate transaminase; GGT, gamma-glutamyl transferase; HOMA-IR, homeostasis model assessment-insulin resistance; MRI, magnetic resonance imaging; PDFF, proton density fat fraction; cT1, iron corrected T1; T2*, effective T2 relaxation time; VAT, visceral adipose; SAT, subcutaneous adipose tissue; MASH, metabolic dysfunction-associated steatohepatitis.
Patients in the LMP group had a median percentage weight loss of −1.31% (1st quartile of −3.22 and 3rd quartile of 1.10), P=0.262. This percentage weight loss was not associated with any significant improvement (reduction) in anthropometric and clinical parameters (Table 2).
Effects of LSG and LMP on liver fibroinflammation, liver fat and abdominal adiposity at 4 months
In the LSG group, there was a significant reduction in the median cT1 (−8.04%, P=0.025), PDFF (−64.52%, P=0.012) and SAT (−77.1%, P=0.018), Table 2. In the LMP group, only liver PDFF (−29.16%, P=0.012) was significantly reduced (Table 2).
The percentage change in cT1 between LSG and LMP groups were not significantly different (−8.04% vs. −3.87%, P=0.374). Post intervention (after 4 months) cT1 increase (indicating worsening of MASH) was not observed in any of the patients (0%) in the LSG group but in 3 (14%) of the LMP group. Figure 2A,2B show the trend of cT1 in both groups between baseline and 4 months follow-up. In other words, 100% and 86% of patients in the LSG and LMP groups, respectively had improvement in liver heath. Figure 3A-3D illustrate sampled image maps of the changes in liver cT1 and liver PDFF in response to either bariatric surgery or lifestyle modification from baseline to 4 months of the interventions.
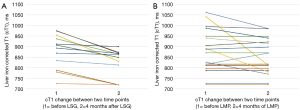
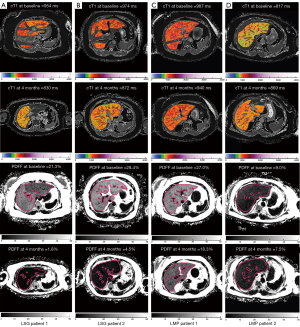
Interestingly, VAT in both groups was not significantly changed at 4 months (LSG: 12.71%, 1st quartile of −20.41 and 3rd quartile of 15.48, P=0.499 and LMP: −0.67%, 1st quartile of −6.95 and 3rd quartile of −0.67, P=0.261), and there were no significant differences in the percentage change in VAT between interventions (P=0.696).
Significant absolute differences at 4 months between interventions were only observed in BMI (P=0.038), triglycerides (P=0.020), insulin (P=0.003), alkaline phosphatase (ALP) (P=0.014), ALT (P=0.020), GGT (P=0.009), liver PDFF (P<0.001), SAT (P=0.032), and HOMA-IR (P=0.002) (Table 2).
Sub analysis-liver histology and imaging
Histology analysis showed that all 13 patients in the LSG group had MASLD. MASH was present in 4 (31%) patients, spotty necrosis was present in 12 (92%), hepatocyte ballooning was present in 4 (31%) and fibrosis was present in 7 (54%). Details of the liver histology outcomes are shown in Table 3.
Table 3
Variable | Liver histology |
---|---|
MASH | 4 (31) |
NAS activity score | |
0 | 0 (0) |
1 | 1 (8) |
2 | 3 (23) |
3 | 5 (39) |
4 | 2 (15) |
5 | 0 (0) |
6 | 2 (15) |
7 | 0 (0) |
8 | 0 (0) |
Steatosis grade | |
0 | 0 (0) |
1 | 5 (39) |
2 | 6 (46) |
3 | 2 (15) |
Spotty necrosis | |
0 | 1 (8) |
1 | 9 (69) |
2 | 3 (23) |
Lobular inflammation grade | |
0 | 1 (8) |
1 | 9 (69) |
2 | 3 (23) |
3 | 0 (0) |
Ballooning grade | |
0 | 9 (69) |
1 | 4 (31) |
2 | 0 (0) |
Fibrosis stage | |
0 | 6 (46) |
1 | 6 (46) |
2 | 1 (8) |
3 | 0 (0) |
4 | 0 (0) |
Data are presented as number (%). MASH, metabolic dysfunction-associated steatohepatitis; NAS activity score, non-alcoholic steatohepatitis activity score.
Liver cT1 correlated with histological degree of steatosis [r=0.788, 95% confidence interval (CI): 0.405 to 0.936, P=0.001] and with NAS (r=0.701, 95% CI: 0.228 to 0.907, P=0.008) but as expected not with fibrosis stage (r=−0.040, 95% CI: −0.590 to 0.536, P=0.897) due to the low stage of fibrosis in this population. Liver PDFF correlated with histological degree of steatosis (r=0.821, 95% CI: 0.480 to 0.947, P<0.001) and with NAS (r=0.810, 95% CI: 0.453 to 0.943, P<0.001) but not with fibrosis stage (r=0.128, 95% CI: −0.469 to 0.645, P=0.676). T2* inversely correlated with histological degree of steatosis (r=−0.595, 95% CI: −0.868 to −0.126, P=0.032) but not with NAS and fibrosis stage (r=−0.517, 95% CI: −0.868 to −0.047, P=0.071 and r=−0.153, 95% CI: −0.660 to 0.449, P=0.618, respectively). Between the imaging biomarkers, liver cT1 correlated with liver PDFF (r=0.835, 95% CI: 0.513 to 0.951, P<0.001).
MASH determination using cT1
In terms of the diagnostic performance between cT1 and PDFF in determining MASH, it was shown that the AUROC of cT1 was 0.89 (95% CI: 0.67 to 1.00) with a cut point of 925 ms, 75% (95% CI: 19.4% to 99.4) sensitivity and 89% (95% CI: 66.4% to 100%) specificity. PDFF had an AUROC of 0.83 (95% CI: 0.57 to 1.00) with a cut point of 20.75%, 75% (95% CI: 19.4% to 99.4%) sensitivity and 89% (95% CI: 51.8% to 99.7%) specificity.
Using the cT1 cut off value of 925 ms to rule in MASH as above, it was shown that 4 (31%) patients in the LSG group had MASH at baseline [of these 4, 3 (75%) were confirmed on histology while 1 (25%) patient was a false negative] and zero (none) at 4 months. In the lifestyle group, at this cT1 cut off point to rule in MASH, 7 (33%) had MASH at baseline and 3 (14%) had MASH at 4 months of the intervention. Figure 4 and Table 4 summarise the above data.
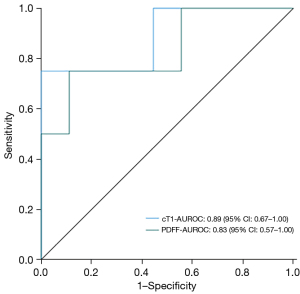
Table 4
MRI biomarker | Cut-off | AUROC | Sensitivity (%) | Specificity (%) | P value |
---|---|---|---|---|---|
cT1 (ms) | 925 | 0.89 (0.67–1.00) | 75 (19.4–99.4) | 89 (66.4–100) | <0.001 |
PDFF (%) | 20.75 | 0.83 (0.57–1.00) | 75 (19.4–99.4) | 89 (51.8–99.7) | 0.013 |
AUROC, sensitivity and specificity data are presented as (95% confidence interval). MRI, magnetic resonance imaging; cT1, iron corrected T1; PDFF, proton density fat fraction; AUROC, area under receiver operating characteristic.
Discussion
This study examined how liver fat and hepatic fibroinflammation are affected by either bariatric surgery and lifestyle modification, in patients with both obesity and MASLD, using liver disease activity cT1 and liver PDFF. The results showed that bariatric surgery (LSG) led to a significant weight loss, which was associated with a significant reduction in both liver cT1 and liver PDFF. In contrast, LMP did not result in significant weight loss, but there was a significant reduction in liver PDFF. In a sub-analysis of patients who underwent intraoperative liver biopsy during the LSG procedure, it was found that liver cT1 and liver PDFF correlated with each other, with hepatic steatosis grade, NAS activity score, and both metrics had a good AUROC in identifying MASH with that of cT1 being superior.
Our study showed that liver cT1 could discriminate between patients with and without histologically proven MASH with a good AUROC (0.89), and a cut-off cT1 value of 925 ms ruled in MASH. cT1 also correlated with the NAS activity score. These findings align with the findings of a systematic review and meta-analysis study by Andersson et al. (34), which showed that the pooled AUROC of liver cT1 for NASH is 0.78 (95% CI: 0.74 to 0.82) and a cT1 cut off value of 925 ms ruled in NASH. At this AUROC, even patients with high-risk NASH could also be identified. With regards to liver PDFF, it correlated with steatosis grade/NAS activity score and had a good AUROC (0.83) but inferior to that of cT1. The apparent correlation of liver PDFF with both NAS activity score and steatosis is due to its sensitivity to fat, an element that is central in both MASLD and MASH. These outcomes agree with previous studies as summarised in a systematic review and meta-analysis study by Andersson et al. (34) that showed a pooled liver PDFF AUROC of 0.69 (95% CI: 0.64 to 0.74). They further showed that liver cT1 AUROC (0.78) was significantly superior to that of liver PDFF (AUROC =0.69). The diagnostic accuracy of liver PDFF to identify NASH has been shown to be poor in the presence of significant fibrosis (16) and this could be the reason why liver PDFF even in our study was not significantly different between patients with and without MASH as 54% of patients in the LSG cohort had fibrosis.
Similar to other findings in literature, these outcomes confirm that liver cT1 could be a useful tool in identifying MASH. This is particularly important as early diagnosis and the differentiation of simple steatosis, MASH and MASH with fibrosis is one of the primary goals in managing MASH, before advanced fibrosis develops. Moreover, it is important to identify patients likely to progress from simple steatosis to MASH, as such a progression is further compounded by hepatic related and non-hepatic related clinical events (35). Indeed, these results gain more significance as they add to the existing non-invasive techniques for early MASH detection, of course with room for improvement in their accuracy, sensitivity, and specificity in determining MASH.
This study showed that bariatric surgery led to a significant weight loss of 22.8%. This was associated with significant reduction in liver cT1, liver PDFF, SAT, anthropometric indexes, HOMA-IR, HbA1c and liver enzymes. In contrast, lifestyle modification led to a weight loss of 1.31%, which only significantly reduced liver PDFF. In agreement with this outcome, previous studies indicate that low fat and low carbohydrate diets can decrease liver fat, even with minimal or absent weight loss (36-38). However, this reduction of liver PDFF in the LMP group did not reach the remission levels for MASLD (liver PDFF <5.5%), unlike in the bariatric surgery group. With this outcome, it is worth noting that inflammation could be resolved (improving MASH) while some steatosis remains, or fat accumulation could be reduced without fully resolving inflammation. This, currently, can only be definitively confirmed through biopsy due to the lack of highly sensitive non-invasive biomarkers for MASH- a gap this study aims to address.
With regards to liver cT1, the bariatric surgery group had a median decrease of 8.04%, while the lifestyle modification group had a median decrease of 3.87%. There was no patient in the bariatric surgery group who experienced an increase in liver cT1 (worsening liver health) as was the case in the lifestyle modification group where 3 patients had increased cT1. However, despite these three patients with elevated liver cT1 in this group, it is reassuring that 43% of patients who had high cT1 values (possible MASH) at baseline had reduced cT1 values indicating improvement in liver health notwithstanding the negligeable weight loss achieved. In agreement with our results, studies suggest that a weight loss of 5–7% is necessary for significant resolution of MASH (8,9). This could explain the absence of patients deemed to have MASH at 4 months (patients had a significant reduction in both liver PDFF and liver cT1 as well as liver enzymes) in the bariatric surgery group, given their overall weight loss of 22.8%. These results suggest that bariatric surgery may be more effective in reversing MASH and/or steatosis than lifestyle modification, at least in the short term (and possibly in the long term) in patients with obesity and MASLD. In fact, this assertion is consolidated by a recent study of Verrastro et al. (39) on patients with histologically confirmed NASH which showed that after one-year, bariatric surgery (sleeve gastrectomy) was 3.43 times more effective than lifestyle modification in achieving NASH resolution without progressing to fibrosis. Thus, our study supports the idea that bariatric surgery could be a primary treatment option for patients with obesity and MASLD, who may be at high risk of MASH, especially considering that there are currently no approved drugs for treating MASH. Therefore, these outcomes are clinically important because fibrosis is a primary indicator of liver complications, as well as cardiovascular mortality and morbidity in MASH.
In the bariatric surgery group, both liver PDFF (64.52%) and liver cT1 (8.04%) were significantly reduced at 4 months, similar to Tan et al. (40) findings that showed liver PDFF loss of 65.25% and 7.07% of liver cT1 at six months post bariatric surgery (LSG). In the lifestyle modification group, our study showed a significant reduction in liver PDFF (29.16%) and not cT1 (3.87%). In like manner, Koutoukidis et al. (41) in a 24-week study in patients subjected to low energy total diet replacement showed a reduction in liver PDFF of 20.71% and 15% in liver cT1. Moreover, previous studies have found that a 21.1% decrease in liver fat (42) and a 7.62% to 8.88% decrease in cT1 (43) are associated with improved NAS activity score and liver fibrosis, without worsening steatohepatitis. Altogether, these results suggest the utility of these metrics to non-invasively monitor liver fat and inflammation status following treatment. This is particularly important and encouraging as one of the current challenges in managing MASH is the lack of highly sensitive non-invasive biomarkers to track the disease’s progression or regression. This is because the current available non-invasive methods are mostly highly sensitive in ruling out advanced fibrosis.
It is interesting that this study shows that four months after bariatric surgery, there was a significant decrease in SAT, but not in VAT. This aligns with a study by Sun et al. (44), which found a greater reduction in SAT compared to VAT three months after LSG. The possible explanations for this difference could be the short time between surgery and follow-up. Also, it could be that since the body generally has more SAT than VAT, so there is simply more SAT to lose. Additionally, the process of reducing VAT might be more complex and slower due to its unique characteristics. VAT has a richer blood supply and more receptors for catecholamines, hormones that trigger fat mobilisation (45). However, VAT also has more insulin receptors, which promote fat storage and inhibit fat breakdown (45). This dual nature could make it more resistant to the effects of weight loss compared to SAT.
This study is not without limitations. Firstly, our sample size is small, so these findings should be validated in larger, multi-centre, and multi-ethnic studies. However, our results are consistent with previous studies. The highest fibrosis stage in our study (bariatric surgery group) was F2 thus, lacked patients with advanced liver fibrosis, while this was unknown in the lifestyle modification group as liver biopsy was not performed. Future studies are needed to confirm the impact of bariatric surgery and lifestyle modification on liver cT1 and liver PDFF in patients with advanced fibrosis, however, our results are consistent with those from populations with more variation. Only LSG was utilised in our study, and it remains uncertain whether other bariatric surgery methods would have similar outcomes as in our study. Although our follow-up time was relatively short (4-month) and it can be argued that longer duration could yield better understanding of the longitudinal changes in imaging markers, our findings highlight the ability of cT1 and PDFF to measure early changes in liver health following intervention. The lack of biopsy at 4 months in the LSG group, and both at baseline and follow-up in the LMP group to relate with the cT1 outcomes in our study is another limitation. However, doing serial biopsies could raise ethical issues. Although our cohort was Chinese and ethnicity has been shown to have an effect on liver fat accumulation in some studies (46), our findings are comparable with those from other populations. Finally, given the small sample size and our study cohort, caution must be exercised in the interpretation and generalisation of these results.
Conclusions
This study is indicative of the potential MRI biomarker-cT1 may have in monitoring MASH following intervention in patients with both obesity and MASLD, allowing objective comparison between intervention strategies. Compared to lifestyle modification, bariatric surgery was more effective in improving liver health.
Acknowledgments
The authors thank all the participants in the study.
Funding: The study was supported by
Footnote
Reporting Checklist: The authors have completed the STROBE reporting checklist. Available at https://qims.amegroups.com/article/view/10.21037/qims-24-148/rc
Conflicts of Interest: All authors have completed the ICMJE uniform disclosure form (available at https://qims.amegroups.com/article/view/10.21037/qims-24-148/coif). E.S. is an employee of Perspectum Ltd. Perspectum Ltd. is a privately funded commercial enterprise that develops medical devices to address unmet clinical needs, including LiverMultiScan®. V.W.S.W. has served as a consultant for AbbVie, Boehringer Ingelheim, Echosens, Gilead Sciences, Intercept, Inventiva, Novo Nordisk, Pfizer, Sagimet Biosciences, TARGET PharmaSolutions, and Visirna; and a speaker for Abbott, AbbVie, Echosens, Gilead Sciences, Novo Nordisk, and Unilab. He has received a research grant from Gilead Sciences, and is a co-founder of Illuminatio Medical Technology. The other authors have no conflicts of interest to declare.
Ethical Statement: The authors are accountable for all aspects of the work in ensuring that questions related to the accuracy or integrity of any part of the work are appropriately investigated and resolved. The study was conducted in accordance with the Declaration of Helsinki (as revised in 2013) and complied with the Hospital Authority Guide on Research Ethics. The study was approved by the joint Chinese University of Hong Kong-New Territories East Cluster Clinical Research Ethics Committee (ethical approval number: 2018.612) and all participants provided written informed consent.
Open Access Statement: This is an Open Access article distributed in accordance with the Creative Commons Attribution-NonCommercial-NoDerivs 4.0 International License (CC BY-NC-ND 4.0), which permits the non-commercial replication and distribution of the article with the strict proviso that no changes or edits are made and the original work is properly cited (including links to both the formal publication through the relevant DOI and the license). See: https://creativecommons.org/licenses/by-nc-nd/4.0/.
References
- Riazi K, Azhari H, Charette JH, Underwood FE, King JA, Afshar EE, Swain MG, Congly SE, Kaplan GG, Shaheen AA. The prevalence and incidence of NAFLD worldwide: a systematic review and meta-analysis. Lancet Gastroenterol Hepatol 2022;7:851-61. [Crossref] [PubMed]
- Quek J, Chan KE, Wong ZY, Tan C, Tan B, Lim WH, et al. Global prevalence of non-alcoholic fatty liver disease and non-alcoholic steatohepatitis in the overweight and obese population: a systematic review and meta-analysis. Lancet Gastroenterol Hepatol 2023;8:20-30. [Crossref] [PubMed]
- Kleiner DE, Brunt EM, Van Natta M, Behling C, Contos MJ, Cummings OW, Ferrell LD, Liu YC, Torbenson MS, Unalp-Arida A, Yeh M, McCullough AJ, Sanyal AJNonalcoholic Steatohepatitis Clinical Research Network. Design and validation of a histological scoring system for nonalcoholic fatty liver disease. Hepatology 2005;41:1313-21. [Crossref] [PubMed]
- Estes C, Razavi H, Loomba R, Younossi Z, Sanyal AJ. Modeling the epidemic of nonalcoholic fatty liver disease demonstrates an exponential increase in burden of disease. Hepatology 2018;67:123-33. [Crossref] [PubMed]
- Younossi ZM, Koenig AB, Abdelatif D, Fazel Y, Henry L, Wymer M. Global epidemiology of nonalcoholic fatty liver disease-Meta-analytic assessment of prevalence, incidence, and outcomes. Hepatology 2016;64:73-84. [Crossref] [PubMed]
- Sangro P, de la Torre Aláez M, Sangro B, D'Avola D. Metabolic dysfunction-associated fatty liver disease (MAFLD): an update of the recent advances in pharmacological treatment. J Physiol Biochem 2023;79:869-79. [Crossref] [PubMed]
- Slomski A. Bariatric Surgery Approaches Reduce Fatty Liver Disease. JAMA 2022;327:710. [Crossref] [PubMed]
- Vilar-Gomez E, Martinez-Perez Y, Calzadilla-Bertot L, Torres-Gonzalez A, Gra-Oramas B, Gonzalez-Fabian L, Friedman SL, Diago M, Romero-Gomez M. Weight Loss Through Lifestyle Modification Significantly Reduces Features of Nonalcoholic Steatohepatitis. Gastroenterology 2015;149:367-78.e5; quiz e14-5. [Crossref] [PubMed]
- Lassailly G, Caiazzo R, Ntandja-Wandji LC, Gnemmi V, Baud G, Verkindt H, Ningarhari M, Louvet A, Leteurtre E, Raverdy V, Dharancy S, Pattou F, Mathurin P. Bariatric Surgery Provides Long-term Resolution of Nonalcoholic Steatohepatitis and Regression of Fibrosis. Gastroenterology 2020;159:1290-1301.e5. [Crossref] [PubMed]
- Lassailly G, Caiazzo R, Gnemmi V, Verkindt H, Ntandja-Wandji LC, Ningarhari M, Leteurtre E, Raverdy V, Dharancy S, Louvet A, Pattou F. MASH resolution without fibrosis worsening after bariatric surgery improves long-term survival. The Liver Meeting 2023: AASLD, 2023.
- Angulo P. Nonalcoholic fatty liver disease. N Engl J Med 2002;346:1221-31. [Crossref] [PubMed]
- Bedossa P, Dargère D, Paradis V. Sampling variability of liver fibrosis in chronic hepatitis C. Hepatology 2003;38:1449-57. [Crossref] [PubMed]
- Intraobserver and interobserver variations in liver biopsy interpretation in patients with chronic hepatitis C. The French METAVIR Cooperative Study Group. Hepatology 1994;20:15-20.
- Middleton MS, Heba ER, Hooker CA, Bashir MR, Fowler KJ, Sandrasegaran K, Brunt EM, Kleiner DE, Doo E, Van Natta ML, Lavine JE, Neuschwander-Tetri BA, Sanyal A, Loomba R, Sirlin CBNASH Clinical Research Network. Agreement Between Magnetic Resonance Imaging Proton Density Fat Fraction Measurements and Pathologist-Assigned Steatosis Grades of Liver Biopsies From Adults With Nonalcoholic Steatohepatitis. Gastroenterology 2017;153:753-61. [Crossref] [PubMed]
- Yokoo T, Shiehmorteza M, Hamilton G, Wolfson T, Schroeder ME, Middleton MS, Bydder M, Gamst AC, Kono Y, Kuo A, Patton HM, Horgan S, Lavine JE, Schwimmer JB, Sirlin CB. Estimation of hepatic proton-density fat fraction by using MR imaging at 3.0 T. Radiology 2011;258:749-59. [Crossref] [PubMed]
- Dennis A, Mouchti S, Kelly M, Fallowfield JA, Hirschfield G, Pavlides M, Banerjee R. A composite biomarker using multiparametric magnetic resonance imaging and blood analytes accurately identifies patients with non-alcoholic steatohepatitis and significant fibrosis. Sci Rep 2020;10:15308. [Crossref] [PubMed]
- Thomaides-Brears HB, Lepe R, Banerjee R, Duncker C. Multiparametric MR mapping in clinical decision-making for diffuse liver disease. Abdom Radiol (NY) 2020;45:3507-22. [Crossref] [PubMed]
- Pavlides M, Banerjee R, Sellwood J, Kelly CJ, Robson MD, Booth JC, Collier J, Neubauer S, Barnes E. Multiparametric magnetic resonance imaging predicts clinical outcomes in patients with chronic liver disease. J Hepatol 2016;64:308-15. [Crossref] [PubMed]
- Pavlides M, Banerjee R, Tunnicliffe EM, Kelly C, Collier J, Wang LM, Fleming KA, Cobbold JF, Robson MD, Neubauer S, Barnes E. Multiparametric magnetic resonance imaging for the assessment of non-alcoholic fatty liver disease severity. Liver Int 2017;37:1065-73. [Crossref] [PubMed]
- Banerjee R, Pavlides M, Tunnicliffe EM, Piechnik SK, Sarania N, Philips R, Collier JD, Booth JC, Schneider JE, Wang LM, Delaney DW, Fleming KA, Robson MD, Barnes E, Neubauer S. Multiparametric magnetic resonance for the non-invasive diagnosis of liver disease. J Hepatol 2014;60:69-77. [Crossref] [PubMed]
- Appropriate body-mass index for Asian populations and its implications for policy and intervention strategies. Lancet 2004;363:157-63. [Crossref] [PubMed]
- Wong VW, Adams LA, de Lédinghen V, Wong GL, Sookoian S. Noninvasive biomarkers in NAFLD and NASH - current progress and future promise. Nat Rev Gastroenterol Hepatol 2018;15:461-78. [Crossref] [PubMed]
- Rinella ME, Lazarus JV, Ratziu V, Francque SM, Sanyal AJ, Kanwal F, et al. A multisociety Delphi consensus statement on new fatty liver disease nomenclature. Hepatology 2023;78:1966-86. [Crossref] [PubMed]
- Matthews DR, Hosker JP, Rudenski AS, Naylor BA, Treacher DF, Turner RC. Homeostasis model assessment: insulin resistance and beta-cell function from fasting plasma glucose and insulin concentrations in man. Diabetologia 1985;28:412-9. [Crossref] [PubMed]
- Lee CH, Shih AZ, Woo YC, Fong CH, Leung OY, Janus E, Cheung BM, Lam KS. Optimal Cut-Offs of Homeostasis Model Assessment of Insulin Resistance (HOMA-IR) to Identify Dysglycemia and Type 2 Diabetes Mellitus: A 15-Year Prospective Study in Chinese. PLoS One 2016;11:e0163424. [Crossref] [PubMed]
- World Health Organization. Definition and diagnosis of diabetes mellitus and intermediate hyperglycaemia: report of a WHO/IDF consultation. 2006.
- Alberti KG, Eckel RH, Grundy SM, Zimmet PZ, Cleeman JI, Donato KA, Fruchart JC, James WP, Loria CM, Smith SC JrInternational Diabetes Federation Task Force on Epidemiology and Prevention. Hational Heart, Lung, and Blood Institute; American Heart Association; World Heart Federation; International Atherosclerosis Society; International Association for the Study of Obesity. Harmonizing the metabolic syndrome: a joint interim statement of the International Diabetes Federation Task Force on Epidemiology and Prevention; National Heart, Lung, and Blood Institute; American Heart Association; World Heart Federation; International Atherosclerosis Society; and International Association for the Study of Obesity. Circulation 2009;120:1640-5. [Crossref] [PubMed]
- Kouvari M, Boutari C, Chrysohoou C, Fragkopoulou E, Antonopoulou S, Tousoulis D, Pitsavos C, Panagiotakos DB, Mantzoros CSATTICA study Investigators. Mediterranean diet is inversely associated with steatosis and fibrosis and decreases ten-year diabetes and cardiovascular risk in NAFLD subjects: Results from the ATTICA prospective cohort study. Clin Nutr 2021;40:3314-24. [Crossref] [PubMed]
- Becerra-Tomás N, Blanco Mejía S, Viguiliouk E, Khan T, Kendall CWC, Kahleova H, Rahelić D, Sievenpiper JL, Salas-Salvadó J. Mediterranean diet, cardiovascular disease and mortality in diabetes: A systematic review and meta-analysis of prospective cohort studies and randomized clinical trials. Crit Rev Food Sci Nutr 2020;60:1207-27. [Crossref] [PubMed]
- Friedewald WT, Levy RI, Fredrickson DS. Estimation of the concentration of low-density lipoprotein cholesterol in plasma, without use of the preparative ultracentrifuge. Clin Chem 1972;18:499-502.
- Wilman HR, Kelly M, Garratt S, Matthews PM, Milanesi M, Herlihy A, Gyngell M, Neubauer S, Bell JD, Banerjee R, Thomas EL. Characterisation of liver fat in the UK Biobank cohort. PLoS One 2017;12:e0172921. [Crossref] [PubMed]
- Bachtiar V, Kelly MD, Wilman HR, Jacobs J, Newbould R, Kelly CJ, Gyngell ML, Groves KE, McKay A, Herlihy AH, Fernandes CC, Halberstadt M, Maguire M, Jayaratne N, Linden S, Neubauer S, Banerjee R. Repeatability and reproducibility of multiparametric magnetic resonance imaging of the liver. PLoS One 2019;14:e0214921. [Crossref] [PubMed]
- Hui SCN, Zhang T, Shi L, Wang D, Ip CB, Chu WCW. Automated segmentation of abdominal subcutaneous adipose tissue and visceral adipose tissue in obese adolescent in MRI. Magn Reson Imaging 2018;45:97-104. [Crossref] [PubMed]
- Andersson A, Kelly M, Imajo K, Nakajima A, Fallowfield JA, Hirschfield G, Pavlides M, Sanyal AJ, Noureddin M, Banerjee R, Dennis A, Harrison S. Clinical Utility of Magnetic Resonance Imaging Biomarkers for Identifying Nonalcoholic Steatohepatitis Patients at High Risk of Progression: A Multicenter Pooled Data and Meta-Analysis. Clin Gastroenterol Hepatol 2022;20:2451-2461.e3. [Crossref] [PubMed]
- Simon TG, Roelstraete B, Khalili H, Hagström H, Ludvigsson JF. Mortality in biopsy-confirmed nonalcoholic fatty liver disease: results from a nationwide cohort. Gut 2021;70:1375-82. [Crossref] [PubMed]
- Westerbacka J, Lammi K, Häkkinen AM, Rissanen A, Salminen I, Aro A, Yki-Järvinen H. Dietary fat content modifies liver fat in overweight nondiabetic subjects. J Clin Endocrinol Metab 2005;90:2804-9. [Crossref] [PubMed]
- Ryan MC, Abbasi F, Lamendola C, Carter S, McLaughlin TL. Serum alanine aminotransferase levels decrease further with carbohydrate than fat restriction in insulin-resistant adults. Diabetes Care 2007;30:1075-80. [Crossref] [PubMed]
- Browning JD, Baker JA, Rogers T, Davis J, Satapati S, Burgess SC. Short-term weight loss and hepatic triglyceride reduction: evidence of a metabolic advantage with dietary carbohydrate restriction. Am J Clin Nutr 2011;93:1048-52. [Crossref] [PubMed]
- Verrastro O, Panunzi S, Castagneto-Gissey L, De Gaetano A, Lembo E, Capristo E, et al. Bariatric-metabolic surgery versus lifestyle intervention plus best medical care in non-alcoholic steatohepatitis (BRAVES): a multicentre, open-label, randomised trial. Lancet 2023;401:1786-97. [Crossref] [PubMed]
- Tan HC, Shumbayawonda E, Beyer C, Cheng LT, Low A, Lim CH, Eng A, Chan WH, Lee PC, Tay MF, Kin S, Chang JPE, Bee YM, Goh GBB. Multiparametric Magnetic Resonance Imaging and Magnetic Resonance Elastography to Evaluate the Early Effects of Bariatric Surgery on Nonalcoholic Fatty Liver Disease. Int J Biomed Imaging 2023;2023:4228321. [Crossref] [PubMed]
- Koutoukidis DA, Mozes FE, Jebb SA, Tomlinson JW, Pavlides M, Saffioti F, Huntriss R, Aveyard P, Cobbold JF. A low-energy total diet replacement program demonstrates a favorable safety profile and improves liver disease severity in nonalcoholic steatohepatitis. Obesity (Silver Spring) 2023;31:1767-78. [Crossref] [PubMed]
- Dennis A, Kelly MD, Fernandes C, Mouchti S, Fallowfield JA, Hirschfield G, Pavlides M, Harrison S, Chakravarthy MV, Banerjee R, Sanyal A. Correlations Between MRI Biomarkers PDFF and cT1 With Histopathological Features of Non-Alcoholic Steatohepatitis. Front Endocrinol (Lausanne) 2020;11:575843. [Crossref] [PubMed]
- Harrison SA, Rossi SJ, Paredes AH, Trotter JF, Bashir MR, Guy CD, Banerjee R, Jaros MJ, Owers S, Baxter BA, Ling L, DePaoli AM. NGM282 Improves Liver Fibrosis and Histology in 12 Weeks in Patients With Nonalcoholic Steatohepatitis. Hepatology 2020;71:1198-212. [Crossref] [PubMed]
- Sun J, Lv H, Li M, Zhao L, Liu Y, Zeng N, Wei X, Chen Q, Ren P, Liu Y, Zhang P, Yang Z, Zhang Z, Wang Z. How much abdominal fat do obese patients lose short term after laparoscopic sleeve gastrectomy? A quantitative study evaluated with MRI. Quant Imaging Med Surg 2021;11:4569-82. [Crossref] [PubMed]
- Lass A, Zimmermann R, Oberer M, Zechner R. Lipolysis - a highly regulated multi-enzyme complex mediates the catabolism of cellular fat stores. Prog Lipid Res 2011;50:14-27. [Crossref] [PubMed]
- de Groot JM, Geurtsen ML, Santos S, Jaddoe VWV. Ethnic disparities in liver fat accumulation in school-aged children. Obesity (Silver Spring) 2022;30:1472-82. [Crossref] [PubMed]