Semi-automatic computed tomography angiography quantification assessment is an alternative method to digital subtraction angiography in intracranial stenosis: a multicenter study
Introduction
Quantifying intracranial atherosclerotic stenosis (ICAS) on digital subtraction angiography (DSA) by the Warfarin-Aspirin Symptomatic Intracranial Disease (WASID) method represents the gold standard (1). In the Stenting and Aggressive Medical Management for Preventing Recurrent Stroke in Intracranial Stenosis (SAMMPRIS) and the China Angioplasty and Stenting for Symptomatic Intracranial Severe Stenosis (CASSISS) trials, focusing on endovascular treatment compared to medical treatment for reducing the recurrent stroke in symptomatic ICAS, DSA has been implemented to measure ICAS for deciding whether the patient fulfilled severe stenosis (70–99%) (2,3). However, in addition to its invasiveness, diagnostic DSA has other pitfalls including excess cost, contrast media use, time-consuming nature, and radiation exposure (4,5). Considering that clinical trials comparing the safety and efficacy of medical treatment and stenting in high-risk patients with ICAS are still needed, looking for a noninvasive diagnostic modality to measure ICAS is imperative to their success.
Noninvasive imaging modalities are frequently utilized for the evaluation of intracranial stenosis diseases (5-7). Computed tomography angiography (CTA) is a fast, cost-effective imaging modality for evaluating the severity of ICAS (diagnosed as mild, moderate, and severe stenosis) and has less contrast media volume and less radiation exposure compared with diagnostic DSA (8,9). However, measuring the absolute degree of ICAS on CTA has not been widely regarded as an alternative to DSA, due to small lumen diameter, vessel tortuosity, and calcification occurrence in intracranial arteries, based on various CTA reconstruction methods, including maximum intensity projection (MIP), multiplanar reconstruction (MPR), and volume rendering (VR) (10-15). With the development of computer-aided analysis, semi-automatic vessel stenosis evaluation methods (for example, Advanced Vessel Analysis software from GE medical systems; GE Healthcare, Chicago, IL, USA) based on multiple reconstructed images (including VR, curved MPR, oblique MPR, and lumen images) have been proposed. The accuracy and reproducibility of such methods have been successfully demonstrated in carotid and peripheral artery stenosis measurement compared with diagnostic DSA (16,17); however, they have not been implemented and validated in the quantification of ICAS compared with DSA.
The ongoing Drug Eluting Stenting and Aggressive Medical Treatment for Preventing Recurrent Stroke in Intracranial Disease (DREAM-PRIDE) trial (18) enrolled 70–99% of ICAS patients using the semi-automatic method on CTA. In the present study, we aimed to evaluate the reliability of measuring absolute stenotic degree of ICAS by a semi-automatic method on CTA compared with the DSA approach, and to determine the optimal protocol for quantifying ICAS on CTA. We present this article in accordance with the STARD reporting checklist (available at https://qims.amegroups.com/article/view/10.21037/qims-23-1343/rc).
Methods
Study design and participants
This study is a prospective, multicenter, and hospital-based study which was registered at www.chictr.org.cn (ChiCTR2100052925). The study was conducted in accordance with the Declaration of Helsinki (as revised in 2013). The study was approved by the Institutional Review Board of Beijing Tiantan Hospital, Capital Medical University (No. KY2019-083-01). All participating hospitals/institutions were informed and agreed with the study. Written informed consent was provided by all participants or their legally authorized guardians. Patients with ischemic stroke ascribed to symptomatic moderate-to-severe ICAS (50–99%) from 6 centers were consecutively and prospectively enrolled between March 2021 and December 2021. All enrolled participants underwent both CTA scan and DSA procedure within 7 days after enrollment. The inclusion criteria were as follows: transient ischemic attack (TIA) or ischemic stroke due to intracranial internal carotid artery (ICA), the M1 segment of middle cerebral artery (MCA), basilar artery (BA), or intracranial vertebral artery (VA) moderate-to-severe stenosis suspected by CTA (≥50% on MIP or VR image) or magnetic resonance imaging (MRA; focal signal loss with the presence of a distal signal). The exclusion criteria were as follows: patients with a stenotic degree <50% confirmed on DSA; non-atherosclerotic disease including Moyamoya disease, intracranial artery dissection; total occlusion of the responsible artery; in-stent restenosis; or images with various artifacts that impact the measurements of ICAS.
Stenosis and reference sites selection
Selection of stenosis and reference sites at the responsible artery was based on the WASID method (1). After reviewing the responsible artery on CTA (VR mode) and DSA, the stenosis and reference sites were consistently identified on CTA and DSA by 2 experienced neurologists (with >10 years of experience) with consensus.
Quantification of ICAS by CTA
The details of CTA scan parameters and contrast agent methods of 6 centers are shown in the Table S1. The axial images were reconstruction by using the standard algorithm. The CTA axial raw data from 6 centers were loaded on a semi-automated vessel analysis software (GE Advantage Workstation, version AW4.7, GE Healthcare) and reformatted (Figure 1A,1B). A 3-dimensional (3D) VR image was created on the workstation (Figure 1C). The operators selected the starting and ending points of the interested vessel lumen by browsing the VR image (the vessel lumen of interest needed to cover at least the stenosis site and the proximal and distal reference site). A central lumen line was then automatically performed by the software (Figure 1D, green line), and the selected vessel lumen was meanwhile displayed to the operators as the curved MPR, axial MPR, oblique MPR (axial vessel view oriented perpendicular to the central lumen line), and lumen MPR (i.e., stretched vessel view). The window width and level were set to 700 and 200 Hounsfield units (HU), but the width/level setting were allowed to be adjusted to best visualize the contrast filled lumen. If the automatically generated central lumen line tracked the lumen poorly, a manual vessel tracking was conducted on the curved MPR, in which the necessary angle adjustment was allowed to best display the lumen center (Figure 1D, red line).
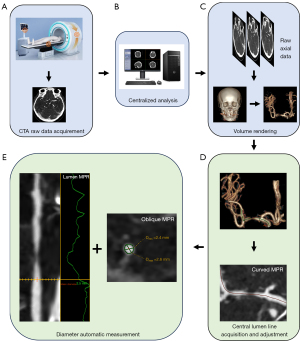
The region of interest (ROI) indicator (Figure 1E, green coil) was then automatically rendered by the software on the oblique MPR at each tracking site, outlining the true cross-sectional area of contrast filled lumen. The minimal diameter and maximal diameter of the generated ROI indicator at the tracking site were automatically measured and displayed on the oblique MPR (Figure 1E), and its mean diameter was automatically measured and displayed on the lumen MPR (Figure 1E). If the ROI indicator of the stenosis site on the oblique MPR was visually estimated to fall outside the range of the actual vessel lumen, manual correction was applied to rectify measurement generated from automatic recognition. All operations on the oblique MRP were performed at the maximum zoom factor that could be achieved.
The diameters of stenosis site and reference site, measured automatically on the semi-automated vessel analysis software, were used to calculate the absolute stenotic degree. The absolute stenotic degree was calculated as (1 − the diameter at the stenosis site/the diameter at the reference site) × 100% (1). Considered minimal, maximal, and mean diameters generated both in stenosis site and reference site (Figure 2), there were 9 permutations of the absolute stenotic degree calculated by CTA.
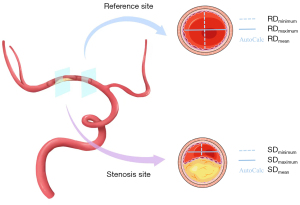
The software identifies calcifications in the arteries within a ROI in every slice as areas with density >130 HU. At least 3 contiguous pixels with HU >130 is registered as calcification (19).
The degree of ICAS was independently semi-automatedly measured by 2 experienced neurologists (with >10 years of experience), using 1 display (MVCD-1619 AW GE) with identical resolution, contrast, color gamut, and colorimetric. Then, the average of their measuring was calculated and recorded. At 1 month after the first measurement, the CTA raw data of the first 30 patients were analyzed and measured by the same neurologist for a second time to verify intra-observer reproducibility.
Quantification of ICAS by DSA
After transfemoral artery puncture under either local or general anesthesia, angiography was performed in all cases. To ensure a comprehensive assessment of the culprit lesion, anteroposterior, lateral, and oblique projections were obtained in all cases, and in some instances, additional rotational DSA was performed. DSA raw data were post-analyzed using RadiAnt Dicom Viewer (Medixant, Poznan, Poland) software. The quantification process of ICAS by DSA was the same as that of the WASID method (1): the absolute stenotic degree was calculated as (1 − Dstenosis/Dnormal) ×100%, and Dstenosis referred to the narrowest diameter at the culprit lesion. The quantitative measurements were performed by the same 2 experienced neurologists independently, and the average of their measurements was recorded.
All measurements with disagreements greater than 10% were reviewed by a third experienced reader (with >20 years of experience), who determined the quantification result from the 2 previous measurements. If the measurements of the stenosis site were unavailable due to a gap sign (i.e., the vessel lumen was not visible at the stenosis site, but contrast media filling was noted in the distal vessel of the stenosis site) on CTA or DSA, a 99% stenosis was defined (Figure 3) (1).
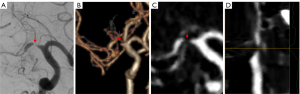
Algorithm of radiation exposure from CTA and DSA
Radiation doses from head CTA and DSA were collected from the first 20 participants in this study. The dose-length product (DLP) from head CTA and dose-area product (DAP) during DSA were recorded. The formula for calculating effective dose (ED) of head CTA and DSA were as follows, respectively: ED (mSv) of CTA = DLP (mGy·cm) × conversion factor (mSv/mGy·cm); ED (mSv) of DSA = median DAP (Gy·cm2) × conversion factor (mSv/Gy·cm2). According to previous studies, the conversion factors of head CTA and DSA were set as 0.0021 mSv/mGy·cm and 0.04 mSv/Gy·cm2, respectively (20,21).
Statistical analysis
The sample size was calculated according to the suggested formula (22). The minimum required sample size was calculated to be 187 participants, with a 95% confidence level, a 10% width of confidence level, and an expected intraclass correlation coefficient (ICC) of 0.810 (23). Categorical data were expressed as frequencies and percentages. Normally distributed continuous data were expressed as the means and standard deviations (SDs), whereas nonnormally distributed continuous data were expressed as the median and interquartile range (IQR). Either Student’s t-test or Mann-Whitney U test was used to compare the degree of stenosis and ED of radiation exposure between CTA and DSA. ICC was used to evaluate the reproducibility of intra-observer and inter-observer quantification of ICAS on CTA through a single measurement, absolute agreement, 2-way mixed effects model. The reliability of quantification of ICAS on CTA, as well as the agreements of quantification of ICAS in various lesion location, calcification or not, and stenosis severity on CTA and DSA were estimated by ICC based on a mean-rating (κ=2), consistency, 2-way randomized effects model (24). An ICC value <0.5, 0.5–0.75, 0.75–0.9, and >0.9 was considered poor, moderate, good, and excellent agreement, respectively (24). A sensitivity analysis to further confirm the reliability for quantifying ICAS on CTA was performed by excluding the lesions with a 99% stenosis. Sensitivity, specificity, positive predictive values, negative predictive values, κ statistic, and receiver operating characteristic (ROC) curve for the identification severe (70–99%) stenosis on CTA with the optimal protocol were calculated using DSA as reference standard. A 2-tailed P<0.05 was considered a statistically significant difference. All statistical analyses were performed using the software SPSS 24.0 (IBM Corp., Armonk, NY, USA).
Results
Participant baseline characteristics
From March 2021 to December 2021, 242 patients met the inclusion criteria. Finally, after excluding 51 patients due to various reasons, 191 participants were consecutively enrolled (flow chart shown in Figure S1). The mean age was 58.8±10.7 years, and among them, 148 of 191 participants (77.5%) were male. The main qualifying events included ischemic stroke in 51.3% (98/191) and TIA in 48.7% (93/191) of the participants.
Among the 191 participants, 11 (5.8%) had intracranial tandem lesions, so a total of 202 lesions were evaluated. These lesions included 22 lesions (10.9%) at the ICA, 88 lesions (43.6%) at the MCA, 43 lesions (21.3%) at the VA, and 49 lesions (24.3%) at the BA (Table 1). None of them had extracranial tandem stenosis. Calcified ICAS was found in 8.4% (17/202) of the lesions, including 9 of 17 at the ICA (52.9%), 6 of 17 at the VA (35.3%), and 2 of 17 at the BA (11.8%). The median of stenotic degree was 78.5% (IQR: 71.2–85.0%) on DSA. On DSA, 80.2% (162/202) lesions were identified as severe stenosis [including 10.9% (22/202) lesions with a 99% stenosis] and 19.8% (40/202) as moderate stenosis. The median time interval from CTA scanning to DSA was 1 day (IQR, 1–1 day).
Table 1
Characteristics | Total (n=191, lesions =202) |
---|---|
Age (years)* | 58.8±10.7 |
Male, n (%) | 148 (77.5) |
Risk factors, n (%) | |
Diabetes mellitus | 65 (34.0) |
Hyperlipidemia | 30 (15.7) |
Hypertension | 123 (64.4) |
Smoking history | 76 (39.8) |
Qualifying events, n (%) | |
Transient ischemic attack | 93 (48.7) |
Ischemic stroke | 98 (51.3) |
Location of target lesions, n (%) | |
Intracranial internal carotid artery | 22 (10.9) |
Middle cerebral artery | 88 (43.6) |
Basilar artery | 49 (24.3) |
Intracranial vertebral artery | 43 (21.3) |
Time interval from CTA to DSA (days)† | 1 (1–1) |
Unless otherwise indicated, data are numbers of participants, and data in parentheses are percentages. *, data are means ± SDs; †, data are reported as the medians, with the IQRs in parentheses. CTA, computed tomography angiography; DSA, digital subtraction angiography; SDs, standard deviations; IQRs, interquartile ranges.
Comparison of ED of CTA and DSA radiation
The median DLP and ED from head CTA were 1,390.85 mGy·cm (IQR, 1,388.30–1,394.41 mGy·cm) and 2.92 mSv (IQR, 2.92–2.93 mSv), respectively. The median DAP and ED during DSA were 219 Gy· cm2 (IQR, 187–271.25 Gy·cm2) and 8.76 mSv (IQR, 7.48–10.85 mSv), and the ED during DSA was higher than that from CTA (P<0.001).
Reproducibility of intra-observer and inter-observer quantification of ICAS on CTA
The reproducibility of intra-observer quantification [ICC, 0.905; 95% confidence interval (CI): 0.731–0.964, P<0.001] and inter-observer quantification (ICC, 0.933; 95% CI: 0.911–0.950, P<0.001) of ICAS on CTA were excellent.
Reliabilities of quantification of ICAS on CTA and DSA
The reliabilities of quantifying ICAS on CTA and DSA were excellent ranging from 0.906 (95% CI: 0.876–0.929, P<0.001) to 0.955 (95% CI: 0.944–0.966, P<0.001) (Table 2). The ICC of the stenotic degree calculated as (1 − Dmin-stenosis/Dmean-normal) × 100% was the highest among the 9 permutations generated from semi-automatic CTA (Table 2). The sensitivity analysis after excluding lesions with 99% stenosis indicated similar results (Table S2). Thus, the (1 − Dmin-stenosis/Dmean-normal) × 100% was used as the standard CTA measurement protocol for quantifying ICAS in the present study. Typical examples of measurement by CTA and DSA are presented in Figures 4,5. In order to assess the impact of different CT devices on image quality, we respectively calculated the agreements of quantification of ICAS on CTA and DSA in the 6 centers. The results are presented in Table S3.
Table 2
Measurement protocols | ICC | 95% CI | P value |
---|---|---|---|
(1 − Dmin-stenosis/Dmax-normal) × 100% | 0.953 | 0.937–0.964 | <0.001 |
(1 − Dmin-stenosis/Dmin-normal) × 100% | 0.943 | 0.925–0.957 | <0.001 |
(1 − Dmin-stenosis/Dmean-normal) × 100% | 0.955 | 0.944–0.966 | <0.001 |
(1 − Dmean-stenosis/Dmax-normal) × 100% | 0.933 | 0.911–0.949 | <0.001 |
(1 − Dmean-stenosis/Dmin-normal) × 100% | 0.906 | 0.876–0.929 | <0.001 |
(1 − Dmean-stenosis/Dmean-normal) × 100% | 0.934 | 0.913–0.950 | <0.001 |
(1 − Dmax-stenosis/Dmax-normal) × 100% | 0.937 | 0.917–0.952 | <0.001 |
(1 − Dmax-stenosis/Dmin-normal) × 100% | 0.913 | 0.885–0.934 | <0.001 |
(1 − Dmax-stenosis/Dmean-normal) × 100% | 0.937 | 0.917–0.952 | <0.001 |
ICAS, intracranial atherosclerotic stenosis; CTA, computed tomography angiography; ICC, intraclass correlation coefficient; CI, confidence interval; D, diameter.
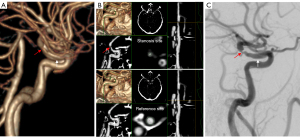
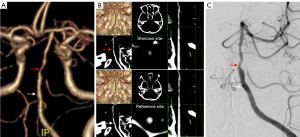
Agreements of quantification of ICAS in various lesion location, calcification or not, and stenosis severity on CTA and DSA
The agreement of CTA and DSA in quantifying BA (ICC, 0.969; 95% CI: 0.945–0.983; P<0.001), MCA (ICC, 0.959; 95% CI: 0.938–0.973; P<0.001), and intracranial VA (ICC, 0.946; 95% CI: 0.901–0.971; P<0.001) was excellent, but was good in quantifying intracranial ICA (ICC, 0.881; 95% CI: 0.714–0.951; P<0.001) (Table 3). The agreement of quantification of non-calcified ICAS on CTA and DSA was excellent (ICC, 0.960; 95% CI: 0.946–0.970; P<0.001), but that of calcified ICAS was good (ICC, 0.849; 95% CI: 0.583–0.945; P<0.001). Similar results were found when excluding lesions with 99% stenosis (Table S4).
Table 3
Characteristics | ICC | 95% CI | P value |
---|---|---|---|
Lesion location | |||
Intracranial internal carotid artery | 0.881 | 0.714–0.951 | <0.001 |
Middle cerebral artery | 0.959 | 0.938–0.973 | <0.001 |
Basilar artery | 0.969 | 0.945–0.983 | <0.001 |
Intracranial vertebral artery | 0.946 | 0.901–0.971 | <0.001 |
Calcified plaque | |||
Non-calcified plaque | 0.960 | 0.946–0.970 | <0.001 |
Calcified plaque | 0.849 | 0.583–0.945 | <0.001 |
Stenosis severity | |||
≥70% stenosis | 0.918 | 0.889–0.940 | <0.001 |
50–69% stenosis | 0.841 | 0.700–0.916 | <0.001 |
ICAS, intracranial atherosclerotic stenosis; CTA, computed tomography angiography; DSA, digital subtraction angiography; ICC, intraclass correlation coefficient; CI, confidence interval.
The agreements of quantifying severe stenosis (ICC, 0.918; 95% CI: 0.889–0.940, P<0.001) on CTA and DSA was higher than that of moderate stenosis (ICC, 0.841; 95% CI: 0.700–0.916, P<0.001). However, when excluding lesions with 99% stenosis, the agreement of severe stenosis (ICC, 0.786; 95% CI: 0.702–0.847, P<0.001) reduced (Table S4).
Accuracy of CTA compared with DSA in detecting severe (70–99%) or 99% stenosis
For the 202 lesions, the median of stenotic degree was 77.8% (IQR: 71.1–83.6%) on CTA. There was no difference in stenotic degree measured on CTA and DSA (P=0.70). On CTA, 77.7% (157/202) of lesions had severe [stenosis including 11.4% (23/202) of lesions with a 99% stenosis], and 22.3% (45/202) had moderate stenosis.
CTA with semi-automatic method detected severe ICAS with a sensitivity of 95.1%, a specificity of 92.5%, a positive predictive value of 98.1%, and a negative predictive value of 82.2% (Table S5). The κ value was 0.836 (95% CI: 0.742–0.930, P<0.001), and the area under the ROC curve (AUC) (Figure 6) was 0.938 (95% CI: 0.887–0.989, P<0.001). CTA detected 99% of ICAS with a sensitivity of 95.5%, specificity of 98.9%, positive predictive value of 91.3%, and a negative predictive value of 99.4%.
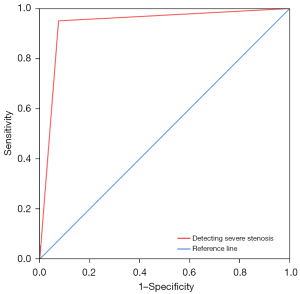
Discussion
The present study found that the semi-automatic quantification of ICAS on CTA has an excellent agreement compared with DSA (ICC, 0.955, P<0.001), and the optimal measurement protocol was calculated as (1 − the minimal diameter of stenosis/the mean diameter of reference) × 100%. After excluding lesions with 99% stenosis, the sensitivity analysis indicated that the results were robust. Besides, the agreements of the semi-automatic CTA with DSA in quantifying non-calcified lesions and severe stenosis were higher than that in calcified lesions (ICC, 0.960 vs. 0.849, P<0.001) and moderate stenosis (ICC, 0.918 vs. 0.841, P<0.001). These findings demonstrated that CTA using the semi-automatic method may be a reliable alternative to DSA for quantifying ICAS.
Previous studies on measuring ICAS with CTA have primarily focused on the classification of stenotic degree, with only a few addressing the comparison of absolute stenotic degree. A large-scale study that compared the accuracy of CTA, using visual inspection on MIP images, to DSA in diagnosing ICAS, demonstrated moderate accuracy in detecting both moderate stenosis (sensitivity =61%, predictive value =84.6%) and severe stenosis (sensitivity =78%, predictive value =81.8%) (25). A following study revealed a high sensitivity (98%) and positive predictive value (93%) of CTA in the classification of ICAS (26). Although previous studies involving absolute stenotic degree reported similar agreement between CTA and DSA, compared with our study (ICC, 0.98, 95% CI: 0.98–0.99; 0.96, 95% CI: 0.95–0.97, respectively) (27,28), it must be noted that both of these studies utilized a digital caliper or ruler respectively for diameter measurement which may be inconvenient for clinical practice. Our study utilized the semi-automated vessel analysis software that can identify the lumen boundary according to intensity discrepancies and ensure consistency by selecting the same point in the halo in both the stenosis site and reference site to measure ICAS, which may decrease reader bias when measuring vessel diameters (16,29). The finding supported that CTA can be an alternative imaging modality for DSA in measuring the absolute stenotic degree of ICAS.
Among 9 permutations from the semi-automatic software in our study, the (1 − Dmin-stenosis/Dmean-normal) × 100% was found to be the optimal combination for the quantifying the stenotic degree. The underlying explanation may be that at the stenosis site, the minimum diameter in the axial reconstruction image on CTA is probably consistent with the diameter measured at the narrowest value on the tangential projection of DSA. When measuring the reference site, the projection of DSA was the same with that at the stenosis site. Considering that the lumen outline at the reference site is quasi-circular shaped, the diameter measured on DSA was more likely to be a value ranging from minimum to maximum diameter of the lumen. Mean diameter generated from CTA is more likely to be consistent with the diameter measured on DSA, but not maximal or minimal diameter form CTA. The previous studies about semi-automated measurement of carotid stenosis used minimum diameter generated from CTA alone (16,17,23). Our study may fill this gap and innovatively provide a practicable and accurate measurement protocol on CTA for ICAS in clinical practice.
Previous studies have demonstrated that evaluating the classification of stenotic degree in the intracranial segment of ICA on CTA had a lower accuracy compared with other lesion locations (25,30). The reasons were as follows: (I) curved course; (II) bone and calcification influence. Similarly, the agreement of CTA and DSA in quantifying intracranial ICA in our study was the lowest among all lesion locations. However, its value (ICC =0.881) still showed a good agreement, which may be attributed to multiple image reconstruction techniques displayed from the semi-automated vessel analysis software, including VR, curved MPR, oblique MPR, and lumen MPR (10-12,14,15). By combining these techniques, it is possible to effectively reconstruct and accurately measure intracranial ICA after careful tracking editing and section editing (10,15).
In the current study, calcified plaque did not demonstrate an excellent agreement compared with non-calcified plaque. The blooming artifacts caused by calcified plaque restricted the delineation of lumen boundary, resulting in reduced measurement accuracy, especially when calcification occurs in small, curved intracranial arteries (30). Not only for intracranial calcified lesions, a similar result was also observed in a study focusing on the measurement of coronary arterial stenosis with high calcium score (31). A study reported that dual-energy CT using a modified algorithm can remove calcified plaque to improve the diagnostic accuracy for calcified stenosis, but iodine in the vessel lumen was concurrently removed (32). Further research is needed to establish the effectiveness of this new method. DSA may be still necessary for severe calcified plaque in the evaluation of ICAS.
In our study, the ICC between CTA and DSA in quantifying severe ICAS was higher than that in moderate stenosis (ICC, 0.918 vs. 0.841), possibly owing to the high sensitivity (95.5%) and specificity (98.9%) between CTA and DSA in assessing the 99% stenosis. Considering that the definition of 99% stenosis (22/202, 10.9%) was a gap sign on CTA or DSA rather than via precise measurement (1), we conducted a sensitivity analysis by excluding lesions with 99% stenosis, and the results presented an attenuated agreement (ICC, 0.786) in quantifying severe stenosis. However, compared with DSA, CTA still has a high sensitivity (95.1%) and positive predictive value (98.1%) in detecting severe stenosis, which suggested the reliability of semi-automatic CTA in diagnose severe ICAS.
The major trials studying ICAS, including SAMMPRIS, WEAVE, and CASSISS, have historically enrolled patients with 70–99% stenosis as evaluated by DSA (2,33,34). Noticeably, the result of our study reveals that the radiation exposure during DSA is higher than that from CTA (median ED, 2.92 vs. 8.76 mSv), which is in line with a previous study (5). These findings supported using CTA as an alternative diagnostic modality to DSA to confirm severe ICAS (70–99%), which can give DSA a pure role focusing on endovascular treatment for the culprit artery. Adverse events related to DSA owing to its invasive features (such as iatrogenic embolization), contrast agent overuse, and X-ray radiation overexposure may decrease (35).
Our study had limitations. Firstly, our study only enrolled patients with moderate or severe ICAS, excluding those with normal vessel and mild stenosis, which may cause a selection bias and restrict the generalizability of our findings to a broader population. Secondly, ICAS located at the anterior cerebral artery, posterior cerebral artery, and so on, was not evaluated in the present study, which may hinder the comprehensiveness of our study findings. Thirdly, as none of the enrolled patients had extracranial tandem stenosis, the potential impacts of stenosis at the initial segment of the ipsilateral internal carotid artery or VA on the measurement of the target intracranial arterial stenosis could not be evaluated.
Conclusions
CTA with semi-automatic analysis demonstrated an excellent agreement with DSA in quantifying ICAS, making it a promising option to replace DSA for the measurement of absolute stenotic degree in clinical trials.
Acknowledgments
We thank all of the participants and healthcare providers who participated in this study.
Funding: This study was supported by
Footnote
Reporting Checklist: The authors have completed the STARD reporting checklist. Available at https://qims.amegroups.com/article/view/10.21037/qims-23-1343/rc
Conflicts of Interest: All authors have completed the ICMJE uniform disclosure form (available at https://qims.amegroups.com/article/view/10.21037/qims-23-1343/coif). The authors have no conflicts of interest to declare.
Ethical Statement: The authors are accountable for all aspects of the work in ensuring that questions related to the accuracy or integrity of any part of the work are appropriately investigated and resolved. The study protocol was approved by the Institutional Review Board of Beijing Tiantan Hospital, Capital Medical University (No. KY2019-083-01) and performed in accordance with the Declaration of Helsinki (as revised in 2013). All participating hospitals/institutions were informed and agreed with the study. Written informed consent was provided by all participants or their legally authorized guardians.
Open Access Statement: This is an Open Access article distributed in accordance with the Creative Commons Attribution-NonCommercial-NoDerivs 4.0 International License (CC BY-NC-ND 4.0), which permits the non-commercial replication and distribution of the article with the strict proviso that no changes or edits are made and the original work is properly cited (including links to both the formal publication through the relevant DOI and the license). See: https://creativecommons.org/licenses/by-nc-nd/4.0/.
References
- Samuels OB, Joseph GJ, Lynn MJ, Smith HA, Chimowitz MI. A standardized method for measuring intracranial arterial stenosis. AJNR Am J Neuroradiol 2000;21:643-6. [PubMed]
- Chimowitz MI, Lynn MJ, Derdeyn CP, Turan TN, Fiorella D, Lane BF, et al. Stenting versus aggressive medical therapy for intracranial arterial stenosis. N Engl J Med 2011;365:993-1003. [Crossref] [PubMed]
- Gao P, Wang T, Wang D, Liebeskind DS, Shi H, Li T, Zhao Z, Cai Y, Wu W, He W, Yu J, Zheng B, Wang H, Wu Y, Dmytriw AA, Krings T, Derdeyn CP, Jiao LCASSISS Trial Investigators. Effect of Stenting Plus Medical Therapy vs Medical Therapy Alone on Risk of Stroke and Death in Patients With Symptomatic Intracranial Stenosis: The CASSISS Randomized Clinical Trial. JAMA 2022;328:534-42. [Crossref] [PubMed]
- Willinsky RA, Taylor SM, TerBrugge K, Farb RI, Tomlinson G, Montanera W. Neurologic complications of cerebral angiography: prospective analysis of 2,899 procedures and review of the literature. Radiology 2003;227:522-8. [Crossref] [PubMed]
- Manninen AL, Isokangas JM, Karttunen A, Siniluoto T, Nieminen MT. A comparison of radiation exposure between diagnostic CTA and DSA examinations of cerebral and cervicocerebral vessels. AJNR Am J Neuroradiol 2012;33:2038-42. [Crossref] [PubMed]
- Chen K, Dou W, Mao H, Wang X, Wang X, Guo Y, Zhang C. Amide proton transfer-weighted magnetic resonance imaging for the diagnosis of symptomatic chronic intracranial artery stenosis: a feasibility study. Quant Imaging Med Surg 2022;12:5184-97. [Crossref] [PubMed]
- Yan L, Song J, Yu Y, Hou Z, Fu W, Cui R, Wan M, Xu X, Lv M, Wang Y, Miao Z, Lou X, Ma N. Predictors of hyperperfusion syndrome after stent implantation in symptomatic intracranial atherosclerotic stenosis. Quant Imaging Med Surg 2023;13:1048-57. [Crossref] [PubMed]
- Safain MG, Rahal JP, Patel S, Lauric A, Feldmann E, Malek AM. Superior performance of cone-beam CT angiography in characterization of intracranial atherosclerosis. J Neurosurg 2014;121:441-9. [Crossref] [PubMed]
- Kim SJ, Schneider DJ, Feldmann E, Liebeskind DS. Intracranial atherosclerosis: Review of imaging features and advances in diagnostics. Int J Stroke 2022;17:599-607. [Crossref] [PubMed]
- van Ooijen PM, Ho KY, Dorgelo J, Oudkerk M. Coronary artery imaging with multidetector CT: visualization issues. Radiographics 2003;23:e16. [Crossref] [PubMed]
- Mallouhi A, Chemelli A, Judmaier W, Giacomuzzi S, Jaschke WR, Waldenberger P. Investigation of cerebrovascular disease with MR angiography: comparison of volume rendering and maximum intensity projection algorithms--initial assessment. Neuroradiology 2002;44:961-7. [Crossref] [PubMed]
- Baskaran V, Pereles FS, Nemcek AA Jr, Carr JC, Miller FH, Ly J, Krupinski E, Finn JP. Gadolinium-enhanced 3D MR angiography of renal artery stenosis: a pilot comparison of maximum intensity projection, multiplanar reformatting, and 3D volume-rendering postprocessing algorithms. Acad Radiol 2002;9:50-9. [Crossref] [PubMed]
- Leclerc X, Godefroy O, Lucas C, Benhaim JF, Michel TS, Leys D, Pruvo JP. Internal carotid arterial stenosis: CT angiography with volume rendering. Radiology 1999;210:673-82. [Crossref] [PubMed]
- Ferencik M, Ropers D, Abbara S, Cury RC, Hoffmann U, Nieman K, Brady TJ, Moselewski F, Daniel WG, Achenbach S. Diagnostic accuracy of image postprocessing methods for the detection of coronary artery stenoses by using multidetector CT. Radiology 2007;243:696-702. [Crossref] [PubMed]
- Addis KA, Hopper KD, Iyriboz TA, Liu Y, Wise SW, Kasales CJ, Blebea JS, Mauger DT. CT angiography: in vitro comparison of five reconstruction methods. AJR Am J Roentgenol 2001;177:1171-6. [Crossref] [PubMed]
- White JH, Bartlett ES, Bharatha A, Aviv RI, Fox AJ, Thompson AL, Bitar R, Symons SP. Reproducibility of semi-automated measurement of carotid stenosis on CTA. Can J Neurol Sci 2010;37:498-503. [Crossref] [PubMed]
- Zhang Z, Berg MH, Ikonen AE, Vanninen RL, Manninen HI. Carotid artery stenosis: reproducibility of automated 3D CT angiography analysis method. Eur Radiol 2004;14:665-72. [Crossref] [PubMed]
- Ma N, Wang Y, Miao Z. Drug Eluting Stenting and Aggressive Medical Treatment for Preventing Recurrent Stroke in Intracranial Atherosclerotic Disease Trial (DREAM-PRIDE). Available online: https://clinicaltrials.gov/study/NCT04948749
- Subedi D, Zishan US, Chappell F, Gregoriades ML, Sudlow C, Sellar R, Wardlaw J. Intracranial Carotid Calcification on Cranial Computed Tomography: Visual Scoring Methods, Semiautomated Scores, and Volume Measurements in Patients With Stroke. Stroke 2015;46:2504-9. [Crossref] [PubMed]
- Le Heron JC. Estimation of effective dose to the patient during medical x-ray examinations from measurements of the dose-area product. Phys Med Biol 1992;37:2117-26. [Crossref] [PubMed]
- Mnyusiwalla A, Aviv RI, Symons SP. Radiation dose from multidetector row CT imaging for acute stroke. Neuroradiology 2009;51:635-40. [Crossref] [PubMed]
- Bonett DG. Sample size requirements for estimating intraclass correlations with desired precision. Stat Med 2002;21:1331-5. [Crossref] [PubMed]
- Silvennoinen HM, Ikonen S, Soinne L, Railo M, Valanne L. CT angiographic analysis of carotid artery stenosis: comparison of manual assessment, semiautomatic vessel analysis, and digital subtraction angiography. AJNR Am J Neuroradiol 2007;28:97-103. [PubMed]
- Koo TK, Li MY. A Guideline of Selecting and Reporting Intraclass Correlation Coefficients for Reliability Research. J Chiropr Med 2016;15:155-63. [Crossref] [PubMed]
- Skutta B, Fürst G, Eilers J, Ferbert A, Kuhn FP. Intracranial stenoocclusive disease: double-detector helical CT angiography versus digital subtraction angiography. AJNR Am J Neuroradiol 1999;20:791-9. [PubMed]
- Bash S, Villablanca JP, Jahan R, Duckwiler G, Tillis M, Kidwell C, Saver J, Sayre J. Intracranial vascular stenosis and occlusive disease: evaluation with CT angiography, MR angiography, and digital subtraction angiography. AJNR Am J Neuroradiol 2005;26:1012-21. [PubMed]
- Nguyen-Huynh MN, Wintermark M, English J, Lam J, Vittinghoff E, Smith WS, Johnston SC. How accurate is CT angiography in evaluating intracranial atherosclerotic disease? Stroke 2008;39:1184-8. [Crossref] [PubMed]
- Duffis EJ, Jethwa P, Gupta G, Bonello K, Gandhi CD, Prestigiacomo CJ. Accuracy of computed tomographic angiography compared to digital subtraction angiography in the diagnosis of intracranial stenosis and its impact on clinical decision-making. J Stroke Cerebrovasc Dis 2013;22:1013-7. [Crossref] [PubMed]
- Liu Y, Hopper KD, Mauger DT, Addis KA. CT angiographic measurement of the carotid artery: optimizing visualization by manipulating window and level settings and contrast material attenuation. Radiology 2000;217:494-500. [Crossref] [PubMed]
- Hirai T, Korogi Y, Ono K, Nagano M, Maruoka K, Uemura S, Takahashi M. Prospective evaluation of suspected stenoocclusive disease of the intracranial artery: combined MR angiography and CT angiography compared with digital subtraction angiography. AJNR Am J Neuroradiol 2002;23:93-101. [PubMed]
- Park MJ, Jung JI, Choi YS, Ann SH, Youn HJ, Jeon GN, Choi HC. Coronary CT angiography in patients with high calcium score: evaluation of plaque characteristics and diagnostic accuracy. Int J Cardiovasc Imaging 2011;27:43-51. [Crossref] [PubMed]
- Qu H, Gao Y, Li M, Zhai S, Zhang M, Lu J. Dual Energy Computed Tomography of Internal Carotid Artery: A Modified Dual-Energy Algorithm for Calcified Plaque Removal, Compared With Digital Subtraction Angiography. Front Neurol 2020;11:621202. [Crossref] [PubMed]
- Alexander MJ, Zauner A, Chaloupka JC, Baxter B, Callison RC, Gupta R, Song SS, Yu WWEAVE Trial Sites and Interventionalists. WEAVE Trial: Final Results in 152 On-Label Patients. Stroke 2019;50:889-94. [Crossref] [PubMed]
- Chimowitz MI, Lynn MJ, Howlett-Smith H, Stern BJ, Hertzberg VS, Frankel MR, Levine SR, Chaturvedi S, Kasner SE, Benesch CG, Sila CA, Jovin TG, Romano JGWarfarin-Aspirin Symptomatic Intracranial Disease Trial Investigators. Comparison of warfarin and aspirin for symptomatic intracranial arterial stenosis. N Engl J Med 2005;352:1305-16. [Crossref] [PubMed]
- Hou Z, Jing J, Yan L, Zhang Z, Fu W, Liu J, Yu Y, Jiang L, Yang J, Wang Y, Miao Z, Lou X, Ma N. New Diffusion Abnormalities Following Endovascular Treatment for Intracranial Atherosclerosis. Radiology 2023;307:e221499. [Crossref] [PubMed]