Prognostic assessment of 18F-boronophenylalanine positron emission tomography (BPA-PET) in salvage boron neutron capture therapy for malignant brain tumors
Introduction
Boron neutron capture therapy (BNCT) emerges as a promising approach in the domain of anti-cancer methods. Its basis lies in the nuclear capture reaction, wherein non-radioactive boron (10B) strongly absorbs neutrons of thermal energy (E<1 eV), leading to the generation of high-energy alpha particles (4He2+) and lithium (7Li) nuclei. The short range of these high linear-transfer alpha and 7Li particles (5–9 µm) is confined to one cell diameter (<10 µm), facilitating selective tumor eradication while sparing the adjacent normal tissue, thus heralding targeted radiation therapy (1-3). The efficacy of BNCT hinges upon the preferential accumulation of sufficient quantities of 10B in cancerous cells compared to their normal counterparts. This selective uptake of boron is achieved through the utilization of boron carriers, notably boronophenylalanine (BPA) derivatives (3).
BPA represents a tumor-specific probe, attributed to its interaction with L-type amino acid transporter 1 (LAT1). Notably, the LAT1 system facilitates the import of BPA primarily into proliferating cells. Consequently, the expression of LAT1 plays a pivotal role in tumor progression, as BPA actively traverses the tumor cell membrane at an elevated rate in these proliferating cells (4).
BPA, being a boron-containing drug with established clinical use, warrants a thorough examination due to its incorporation into clinical dosimetry and treatment planning through 18F-boronophenylalanine positron emission tomography (BPA-PET) data. This imaging modality enables the estimation of BPA concentration within the tumor, thereby enabling the assessment of treatment feasibility and efficacy. A usually suggestion for targeted radiation therapy is achieving a tumor to normal tissue (T/N) ratio of boron-contained drug concentration greater than a specified threshold, with a patient exhibiting a ratio exceeding 2.5 being deemed an optimal candidate for BNCT (5,6). Nevertheless, quantitative assessment techniques in BPA-PET remain to be established. The T/N ratio and the tumor to blood (T/B) ratio were computed based on the maximum standardized uptake value (SUV) of the brain, tumor, and heart.
Prior investigations have demonstrated favorable treatment outcomes with BNCT, particularly in patients afflicted with brain tumors (6-10). Notably, 18F-BPA injection holds the potential to achieve this goal due to the elevated T/N ratios of BPA uptake observed in cases of newly-diagnosed glioblastoma, recurrent malignant glioma, and brainstem tumors (6-10). Moreover, there have been reports of similar promising results in other tumor types such as head and neck cancers, melanoma, sarcoma, and hepatocellular carcinoma (11-15).
However, no studies have been conducted on patients who underwent BPA-PET evaluation but did not receive BNCT treatment. The present study endeavors to evaluate the relationship between BPA-PET and BNCT treatment in patients and compare the outcomes of those treated with BNCT to those who did not undergo this therapy. We present this article in accordance with the STROBE reporting checklist (available at https://qims.amegroups.com/article/view/10.21037/qims-23-1769/rc).
Methods
Patients
This study was centered around a cohort of patients who underwent BPA-PET for the purpose of proceeding with BNCT for malignant brain tumors at Taipei Veterans General Hospital. A retrospective analysis was conducted (Figure 1). The inclusion criteria were those who underwent BPA-PET between February 2017 and April 2022 (n=460), and who were diagnosed with brain tumors (n=149). Patients were followed up for at least 1 year. The exclusion criteria were those who were loss of follow-up (n=20), or who were found the benign nature of tumors or metastases originating from elsewhere (n=13). Consequently, a total of 116 patients were included in this study, and they were categorized into two cohorts: those who underwent BNCT (Group 1, n=58) and those who did not (Group 2, n=58). The study was conducted in accordance with the Declaration of Helsinki (as revised in 2013). The study was approved by the institutional review board of Taipei Veterans General Hospital (IRB No. 2022-07-0029CC). Due to the retrospective nature of the study, written or verbal informed consent was waived.
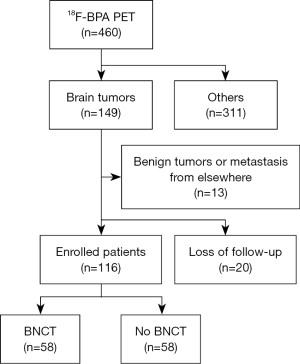
The BNCT protocol used in this study was modified based on the regimen developed by Kyoto University in Japan. The total infused dose was 450 mg/kg body weight. The infusion formula was full infusion for the first two hours (180 mg/kg) and then infusion at half of the initial infusion rate (90 mg/kg) during neutron irradiation to maintain a constant boron concentration in the blood. The epithermal neutron source for BNCT was the Tsing-Hua Open-Pool Reactor (THOR) at National Tsing-Hua University. The blood boron concentration and minimum, maximum, and mean tumor doses required for the gross tumor volume (GTV) were estimated and recorded. In this study, the mean tumor dose required for the GTV was estimated to be 20–40 Gray-Equivalents (Gy-E) (9). Bevacizumab was administered at a dosage of 10 mg/kg promptly following BNCT and at biweekly intervals until disease progression, severe treatment-related toxicity (grade 3), or patient mortality.
BPA-PET imaging and image analysis
To assess the T/N ratio and facilitate treatment planning, BPA-PET imaging was conducted following the Standard Operating Procedure (SOP) at Taipei Veterans General Hospital (6,9-12). 18F-BPA was utilized as the radiotracer with an intravenous injection of 185%±10% MBq dose. The BPA-PET imaging was acquired using integrated PET/MRI or PET/CT scanners 60 minutes post-injection of the radiotracer. PET imaging data was acquired from the head with a 3-minute acquisition period per bed position. The raw PET imaging data was reconstructed into a 128×128 matrix using an iterative reconstruction algorithm. Evaluation and quantification of the SUV were performed using the Xeleris Workstation (GE Healthcare). Regions of interest (ROIs) were drawn on the highest uptake area within the tumor and the normal tissue of the buccal mucosa near the tumor to measure the T/N ratio. The T/N ratio was evaluated from static emission scans acquired 60 minutes after tracer injection, with a 20-minute emission time.
The T/N and T/B ratios of BPA-PET were computed and documented. The contralateral brain lobe was designated as normal tissue for T/N ratio computation, while the heart ventricle served as a blood pool for T/B ratio determination. The mean standard uptake value (SUVmean) was used for the tumor lesion. Herein, Gy-E was used to indicate the biologically equivalent dose administrated to patients during BNCT. Gy-E values were calculated by multiplying the physical dose given by the relative biological effectiveness (RBE). The compound biological effectiveness (CBE) was used to assess the boron dose derived from effects of the 10B(n, alpha)7Li reaction employing 10B-L-BPA as the boron carrier. The following values were used in this study: RBE-thermal neutron =3.2, RBE =1.0, CBE =3.8 for the tumor, CBE =2.5 for normal skin, CBE =1.35 for the nerves, brain max dose <10 Gy-E, and brain mean dose <2 Gy-E.
Response evaluation
Magnetic resonance imaging (MRI) scans were performed before and every 3 months after the BNCT procedure to assess radiologic response according to the Response Assessment in Neuro-Oncology criteria (RANO) (16). Complete response (CR) was defined as the complete disappearance of the tumor within the brain after treatment. Partial response (PR) was defined as a ≥50% reduction in tumor volume after treatment (the largest cross-sectional tumor area) sustained for at least 4 weeks. Progressive disease (PD) was defined as a ≥25% increase in tumor volume after treatment, while stable disease (SD) was defined when the tumor volume after BNCT treatment did not meet the criteria for CR, PR, or PD. Objective response rate (ORR) was defined as the sum of CR and PR, while disease control rate (DCR) was defined as the sum of CR, PR, and SD. All patients were followed up every 3 months with MRI imaging and neurological examination.
Statistical analysis
The clinical outcomes were ascertained from medical records, while potential prognostic factors for survival were assessed. Overall survival (OS) was defined as the time elapsed from the day of intervention to the date of death or the last follow-up, with no restriction on the cause of death. Consequently, the inception of survival was designated as the day of BPA-PET. The T/N ratio derived from BPA-PET was set at 2.5. A cumulative survival curve was generated using the Kaplan-Meier method. Continuous variables were expressed as mean ± standard deviation. Categorical variables were expressed as percentages n (%). Differences between the groups were analyzed using two-sided Student’s t-test, log-rank and the chi-squared test, with statistical significance defined at P<0.05. All calculations were performed using SPSS software, version 20.0 (SPSS, Chicago, IL, USA).
Results
A total of 116 patients with T/N ratios derived from BPA-PET were included in this study. Their demographic information, concerning those who underwent BNCT for malignant brain tumors, was presented in Table 1. The patients were divided into two groups: Group 1 (n=58) comprising those who underwent BNCT and Group 2 (n=58) consisting of those who did not. All of them were recurrent cases. No significant differences were observed in terms of age, gender, or survival rate between the two groups. However, a significant difference was found in T/N ratios derived from BPA-PET (P<0.0001). Specifically, 44 of 58 (75.9%) patients in Group 1 and 26 of 58 (44.8%) patients in Group 2 exhibited T/N ratios greater than 2.5, and this difference was statistically significant (P=0.0007). The tumor types included glioblastoma (n=77), astrocytoma (n=21), oligoastrocytoma (n=7), brainstem glioma (n=7), anaplastic ependymoma (n=2), pineal gland tumor (n=1), and medulloblastoma (n=1). Glioblastoma constituted the major tumor type in both groups, and there was no significant difference in tumor type distribution between the two groups (glioblastoma vs. others, P=0.8458).
Table 1
Total patient (n=116) | BNCT (n=58) | No BNCT (n=58) | P value |
---|---|---|---|
Age (year-old) | 0.8455 | ||
Mean ± SD | 42.1±20.2 | 42.9±23.4 | |
Median (range) | 45 (4–88) | 50 (3–79) | |
Gender, n (%) | 0.7115 | ||
Male | 28 (48.3) | 30 (51.7) | |
Female | 30 (51.7) | 28 (48.3) | |
Survival, n (%) | 0.2835 | ||
Survival | 6 (10.3) | 10 (17.2) | |
Expired | 52 (89.7) | 48 (82.8) | |
Tumor to normal tissue ratio | |||
Mean ± SD | 2.8±0.9 | 2.1±0.7 | <0.0001 |
Median (range) | 2.6 (1.7–5.6) | 2.0 (1.0–4.0) | |
≥2.5, n (%) | 44 (75.9) | 26 (44.8) | 0.0007 |
<2.5, n (%) | 14 (24.1) | 32 (55.2) | |
Tumor type, n (%) | 0.8458 | ||
Glioblastoma | 38 (65.5) | 39 (67.2) | |
Others | 20 (34.5) | 19 (32.8) | |
Astrocytoma | 13 (22.4) | 8 (13.8) | |
Oligoastrocytoma | 3 (5.2) | 4 (6.9) | |
Brainstem glioma | 3 (5.2) | 4 (6.9) | |
Anaplastic ependymoma | 1 (1.7) | 1 (1.7) | |
Pineal gland tumor | 0 (0.0) | 1 (1.7) | |
Medulloblastoma | 0 (0.0) | 1 (1.7) |
BNCT, boron neutron capture therapy; SD, standard deviation.
Mortality was observed in 100 patients from the entire cohort. The median OS for the entire cohort was 7.0 months (range, 0.7–38.7 months, Table 2). Upon comparing the two groups, Group 1 had a median OS of 8.5 months (range, 2.9–36.1 months), while Group 2 had a median OS of 6.0 months (range, 0.7–38.7 months; P=0.3623, Figure 2A). Among the 116 patients, 70 had T/N ratios greater than 2.5, while 46 had T/N ratios lower than 2.5. However, there was no significant difference in patient survival based on T/N ratios greater than 2.5 or not (median OS 7.1 vs. 7.0 months, P=0.8955, Figure 2B), neither in Group 1 (median OS 8.5 vs. 8.5 months, P=0.2630, Figure 2C), nor in Group 2 (median OS 4.3 vs. 6.1 months, P=0.1602, Figure 2D). The cumulative OS of patients with T/N ratios exceeding 2.5 in Group 1 was compared to those in Group 2 (median OS 8.5 vs. 4.3 months, P=0.0783, Figure 2E), albeit no statistically significant distinction was observed.
Table 2
Variables | Patients, n (%) | Mean OS, months | Median OS (range), months | Survival, n (%) | P value |
---|---|---|---|---|---|
Total patients | 116 (100) | 9.4 | 7.0 (0.7–38.7) | 16 (13.8) | |
BNCT | 0.3623 | ||||
Yes | 58 (50.0) | 10.4 | 8.5 (2.9–36.1) | 6 (10.3) | |
No | 58 (50.0) | 8.6 | 6.0 (0.7–38.7) | 10 (17.2) | |
T/N ratio ≥2.5 | |||||
No | 46 (39.7) | 9.5 | 7.0 (0.8–34.5) | 7 (15.2) | 0.8955 |
Yes | 70 (60.3) | 9.3 | 7.1 (0.7–38.7) | 9 (12.9) | |
BNCT | 44 (62.9) | 10.4 | 8.5 (2.9–36.1) | 6 (13.6) | 0.0783 |
No BNCT | 26 (37.1) | 7.4 | 4.3 (0.7–38.7) | 3 (11.5) | |
BNCT | 0.2630 | ||||
T/N ratio ≥2.5 | 44 (75.9) | 10.4 | 8.5 (2.9–36.1) | 6 (13.6) | |
T/N ratio <2.5 | 14 (24.1) | 9.2 | 8.5 (4.3–17.3) | 0 (0.0) | |
No BNCT | 0.1602 | ||||
T/N ratio ≥2.5 | 26 (44.83) | 7.4 | 4.3 (0.7–38.7) | 3 (11.5) | |
T/N ratio <2.5 | 32 (55.17) | 9.6 | 6.1 (0.8–34.5) | 7 (21.9) |
BNCT, boron neutron capture therapy; OS, overall survival; T/N ratio, tumor to normal tissue ratio.
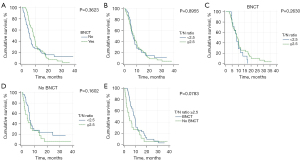
Tumor response in patients was assessed by cranial MRI examination before and 3 months after BNCT (Figure 3). Among Group 1 patients, three patients could not undergo treatment response evaluation due to expiration within 3 months after BNCT. Consequently, 55 patients were included in the response evaluation. The results showed CR in 7 patients, PR in 10 patients, SD in 9 patients, and PD in 29 patients. The proportions of patients who achieved CR, PR, SD, and PD were 12.7%, 18.2%, 16.4%, and 52.7%, respectively. The ORR was 30.9%, and the DCR was 47.3% (Table 3). The T/N ratio, T/B ratio, and absorbed doses were delineated in Table 3. No significant differences were observed among them in terms of T/N ratio (CR vs. PR, P= 0.2060; CR vs. SD, P=0.3497; CR vs. PD, P=0.8359; PR vs. SD, P=0.9743; PR vs. PD, P=0.1127; SD vs. PD, P=0.2676) and absorbed doses (CR vs. PR, P=0.6689; CR vs. SD, P=0.7074; CR vs. PD, P=0.5994; PR vs. SD, P=0.3182; PR vs. PD, P=0.9009; SD vs. PD, P=0.2508; respectively). Regarding the T/B ratio, there was no significant difference among them (CR vs. PR, P=0.2778; CR vs. SD, P=0.3666; PR vs. SD, P=0.8159; PR vs. PD, P=0.5933; SD vs. PD, P=0.3805; respectively), except for CR vs. PD in the T/B ratio (P=0.0323).
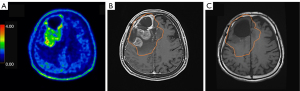
Table 3
Response | BNCT treatment response (n=55) | |||
---|---|---|---|---|
N (%) | T/N ratio | T/B ratio | Doses (Gy-E) | |
CR | 7 (12.73) | 2.71±0.56 | 3.16±0.56* | 24.95±13.48 |
PR | 10 (18.18) | 3.18±0.88 | 2.72±1.03 | 27.56±09.55 |
SD | 9 (16.36) | 3.16±1.22 | 2.82±0.87 | 22.49±11.64 |
PD | 29 (52.73) | 2.66±0.61 | 2.52±0.91 | 28.06±14.06 |
ORR (CR + PR) | 30.91% | |||
DCR (CR + PR + SD) | 47.27% |
Data are N (%) or mean ± standard deviation. *, P=0.0323, CR versus PD. T/N ratio, tumor to normal tissue ratio; T/B ratio, tumor to blood ratio; BNCT, boron neutron capture therapy; Gy-E, Gray-Equivalents; CR, complete response; PR, partial response; SD, stable disease; PD, progressive disease; ORR, objective response rate (ORR = CR + PR); DCR, disease control rate (DCR = CR + PR + SD).
The survival analyses were based on various treatment responses. Mortality was observed in 49 of 55 patients in Group 1. Patient survival analysis, and the association between patient survival and T/N ratios derived from BPA-PET in patients with CR, PR, SD, ORR, and DCR after BNCT were shown in Table 4. The median OS for these patients was 8.5 months (range, 2.9–36.1 months). Among patients with CR, PR, SD, PD, ORR and DCR, the median OS times were 15.7, 11.6, 7.9, 8.4, 13.5 and 10.1 months, respectively. Patients who achieved CR, ORR, and DCR demonstrated significantly higher median OS than those who did not (median OS 15.7 vs. 8.5 months, 13.5 vs. 8.3 months, and 10.1 vs. 8.4 months, P=0.0181, 0.0021, and 0.0133, respectively, Figure 4A-4C). For patients with CR and ORR, T/N ratios greater than 2.5 were associated with significantly higher OS than those with T/N ratios lower than 2.5 (median OS 16.9 vs. 8.5 months and 14.8 vs. 9.0 months, P=0.0253 and 0.0199, respectively, Figure 4D,4E).
Table 4
Variables | Patients, n (%) | Mean OS, months | Median OS (range), months | Survival, n (%) | P value |
---|---|---|---|---|---|
Total patients | 55 (100) | 10.8 | 8.5 (2.9–36.1) | 6 (10.9) | |
T/N ratio ≥2.5 | 42 (76.4) | 10.8 | 6.5 (2.9–36.1) | 6 (14.3) | 0.2705 |
T/N ratio <2.5 | 13 (23.6) | 9.6 | 8.5 (4.3–17.3) | 0 (0.0) | |
CR | |||||
CR | 7 (12.7) | 17.5 | 15.7 (5.8–36.1) | 2 (28.6) | 0.0181 |
PR + SD + PD | 48 (87.3) | 9.5 | 8.5 (2.9–30.6) | 4 (8.3) | |
T/N ratio ≥2.5 | 6 (85.7) | 19.0 | 16.9 (5.8–36.1) | 2 (33.3) | 0.0253 |
T/N ratio <2.5 | 1 (14.3) | 8.5 | 8.5 (8.5–8.5) | 0 (0.0) | |
PR | |||||
PR | 10 (18.2) | 12.0 | 11.6 (4.9–20.1) | 2 (20.0) | 0.2385 |
CR + SD + PD | 45 (81.8) | 10.2 | 8.5 (2.9–36.1) | 4 (8.9) | |
T/N ratio ≥2.5 | 8 (80.0) | 12.6 | 11.8 (7.9–20.1) | 2 (25.0) | 0.1527 |
T/N ratio <2.5 | 2 (20.0) | 9.2 | 9.2 (4.9–13.5) | 0 (0.0) | |
SD | |||||
SD | 9 (16.4) | 8.8 | 7.9 (2.9–16.8) | 1 (11.1) | 0.3724 |
CR + PR + PD | 46 (83.6) | 10.8 | 8.9 (3.8–36.1) | 5 (10.9) | |
T/N ratio ≥2.5 | 7 (77.8) | 7.5 | 5.3 (2.9–16.8) | 1 (14.3) | 0.5329 |
T/N ratio <2.5 | 2 (22.2) | 13.3 | 13.3 (9.7–16.8) | 0 (0.0) | |
PD | |||||
PD | 29 (52.7) | 8.8 | 8.4 (3.8–30.6) | 1 (3.5) | 0.0133 |
CR + PR + SD | 26 (47.3) | 12.3 | 10.1 (2.9–36.1) | 5 (19.2) | |
T/N ratio ≥2.5 | 21 (72.4) | 8.8 | 8.4 (3.8–30.7) | 1 (4.8) | 0.9335 |
T/N ratio <2.5 | 8 (27.6) | 8.9 | 8.1 (4.3–17.3) | 0 (0.0) | |
ORR | |||||
CR + PR | 17 (30.9) | 14.2 | 13.5 (4.9–36.1) | 4 (23.5) | 0.0021 |
SD + PD | 38 (69.1) | 8.8 | 8.3 (2.9–30.7) | 2 (5.3) | |
T/N ratio ≥2.5 | 14 (82.4) | 15.4 | 14.8 (5.8–36.1) | 4 (28.6) | 0.0199 |
T/N ratio <2.5 | 3 (17.6) | 7.3 | 9.0 (4.9–13.5) | 0 (0.0) | |
DCR | |||||
CR + PR + SD | 26 (47.3) | 12.3 | 10.1 (2.9–36.1) | 5 (19.2) | 0.0133 |
PD | 29 (52.7) | 8.8 | 8.4 (3.8–30.6) | 1 (3.5) | |
T/N ratio ≥2.5 | 21 (80.8) | 12.7 | 10.5 (2.9–36.1) | 5 (23.8) | 0.2072 |
T/N ratio <2.5 | 5 (19.2) | 10.7 | 9.8 (4.9–16.8) | 0 (0.0) |
BNCT, boron neutron capture therapy; OS, overall survival; T/N ratio, tumor to normal tissue ratio; CR, complete response; PR, partial response; SD, stable disease; PD, progressive disease; ORR, objective response rate; DCR, disease control rate.
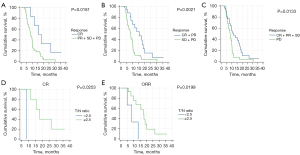
Discussion
BNCT represents a contemporary approach to biochemically targeted radiation treatment, allowing precise delivery of high-dose particle irradiation exclusively to neoplastic cells, thereby sparing adjacent normal tissues. The use of BPA-PET as a reliable tool for patient screening enables noninvasive prediction of tumor uptake and the T/N ratio, aiding physicians in selecting patients most likely to benefit from BNCT. Treatment planning and dosimetry are tailored based on the tumor uptake of the radioisotope adjusted to BPA concentration in normal tissue. A high T/N ratio is preferred to ensure maximum radiation dose to the tumor and minimal background radiation. BNCT is commonly applied if the target lesion demonstrates a T/N ratio on BPA-PET greater than 2.5 (5,6,17). Prior research by Chen et al. evaluated 34 malignant brain tumor patients and revealed improved survival outcomes in patients with a T/N ratio greater than or equal to 4 compared to those with a ratio less than 4 (9). In our current study, there was a significant difference in T/N ratios derived from BPA-PET between patients who received BNCT and those who did not (P<0.0001). When considering a T/N ratio of 2.5, 44 of 58 (75.9%) patients in the BNCT group and 26 of 58 (44.8%) patients in the non-BNCT group exhibited T/N ratios greater than 2.5. This difference reached statistical significance (P=0.0007). Therefore, a T/N ratio greater than 2.5 is commonly regarded as the standard for BNCT among most physicians in our hospital. Within our database, merely 3 out of 55 instances in Group 1 exhibited T/N ratios surpassing 4.0 (4.48, 4.70, and 5.56), corresponding to the PR, PR, and SD categories, respectively. The potential significance might emerge with a larger cohort exhibiting T/N ratios exceeding 4.
The expression of LAT1 may play a significant role in tumor progression, as it acts as a tumor-specific probe of LAT1. Wittig et al. investigated the correlation between the proportion of tumor cells expressing LAT1 and/or Ki67 and BPA uptake in three tumor entities. Their results showed that neither LAT1 nor Ki67 expression predicted BPA uptake (18). Watanabe et al. discovered that the strength of LAT1 expression strongly influenced the BPA uptake ability of tumor cells, with squamous cell carcinomas expressing high levels of LAT1 regardless of the primary tumor site. Higher LAT1 expression in tumors correlated with elevated expression of cell proliferation markers and poorer patient prognosis. Patients with low LAT1 expression in the tumor tissue were more likely to have a better prognosis than those with high LAT1 expression. BPA exhibited higher concentration in tumors with high LAT1 expression, suggesting potential effectiveness of BNCT in tumors with poor prognosis and higher proliferative potential and nutritional requirements (4). However, there was no evident difference in the survival curves of patients with malignant brain tumors based on LAT1 expression (4). In our study, there was no significant difference in patient survival based on T/N ratios greater than 2.5 or not (median OS 7.1 vs. 7.0 months, P=0.8955). In patients who did not receive BNCT, a higher T/N ratio seemed to be associated with a poorer prognosis (median OS 4.3 vs. 6.1 months, P=0.1602). However, this observation did not apply to patients who received BNCT (median OS 8.5 vs. 8.5 months, P=0.2630). The cumulative OS of patients with T/N ratios greater than 2.5 who received BNCT did not show a significant difference compared to those who did not receive BNCT (median OS 8.5 vs. 4.3 months, P=0.0783). Therefore, our findings suggest that the T/N ratio may not strongly correlate with the survival of patients with malignant brain tumors. However, it remains uncertain whether high LAT1 expression correlates with poor prognosis in solid tumor patients (18,19).
Numerous prior studies have presented comprehensive outcomes for patients treated with BNCT (20-24). However, no comparative data have been explored for patients who had tumors evaluated by BPA-PET but did not receive BNCT treatment. Miyatake et al. conducted BNCT in cases of recurrent high-grade gliomas (HGG) (5). Given the absence of an established standard treatment for recurrent HGG, evaluating the survival benefit after BNCT can be challenging. They compared their results with other published series of recurrent malignant gliomas in a combined cohort (n=129) proposed by Carson et al., which revealed a median survival of 4.4 months [95% confidence interval (CI): 3.6–5.4 months] (25). In contrast, in the patients of Miyatake et al. with similar prognostic factors (n=11), the median survival was 9.1 months (95% CI: 4.4–11.0 months), indicating the potential effectiveness of BNCT in managing recurrent HGG even in cases with a poor prognosis (26). In their initial series, more than 50% reduction of the contrast-enhancing lesion volume was demonstrated during follow-up in 8 of 12 patients (27). Upon juxtaposing the two cohorts, the BNCT group exhibited a median OS of 8.5 months (range, 2.9–36.1 months), whereas the non-BNCT group displayed a median OS of 6.0 months (range, 0.7–38.7 months). The cumulative OS of patients with T/N ratios exceeding 2.5 in Group 1 surpassed that of Group 2 (median OS 8.5 vs. 4.3 months, P=0.0783). Regrettably, this disparity failed to attain statistical significance. While we did not observe a difference in the entire cohort of malignant brain tumors, patients with an ORR of 30.9% in our study showed significantly higher median OS than those who did not (median OS 13.5 vs. 8.3 months, P=0.0021). Among the 17 patients with ORR, 14 (82.4%) had a T/N ratio greater than 2.5, indicating significantly higher survival than those without (median OS 14.8 vs. 9.0 months, P=0.0199, respectively). Therefore, ORR appears to be closely associated with the values of T/N ratio derived from BPA-PET. Interestingly, in patients who did not receive BNCT, a higher T/N ratio was associated with a poorer prognosis. However, after effective BNCT, these patients experienced improved survival. Consequently, BNCT with ORR seems necessary to prolong the survival of patients with malignant brain tumors.
Using accelerator-based BNCT combined with BPA as a boron-carrying drug to treat locally recurrent head and neck cancer was approved in Japan in 2020 based on clinic trial results (28-30). In BPA-based BNCT, BPA-PET is crucial to estimate Boron-concentration quantitatively in the target tumors and surrounding normal tissue before BNCT (31-33). Our results could emphasize the use of BPA-PET before BNCT. Moreover, over-expression of LAT-1 in cancer outside the brain, head, and neck now can be quantitatively imaged by BPA-PET (34,35), thereby extending the role of BPA-PET. Using the theranostic combination of BPA and BPA-PET, we not only can assess the aggressiveness of tumor using BPA-PET but also can treat them with BNCT.
Nevertheless, our study possesses certain limitations. Firstly, the dataset was derived from a specific population of critically ill patients with end-stage malignant brain tumors who met the criteria for Emergency and Compassionate Use (Expanded Access Program). Because we are the only medical institute performing BPA-PET and BNCT treatment, the pathologic diagnoses in our patients group became mixed and indetermined. Unfortunately, for patients referred from other institutes, KPS data of some patients were lacking. The histology, especially the tumor type “astrocytoma”, “oligoastrocytoma”, and “brainstem glioma” and the grading, cannot be confirmed. Also, some patients suffered from recurrent brain tumors did not receive re-biopsy for the recurrent lesions, despite high-grade transformation were highly suspected on the surveillant images. BNCT was only utilized as a salvage treatment for severe and life-threatening brain tumors. The exact role of management options in multimodality treatment strategies was not discussed in this study. Secondly, the sample size was relatively small and originated from a single institution, introducing potential biases. Thirdly, Bevacizumab was administered promptly following BNCT and at biweekly intervals until disease progression, severe treatment-related toxicity (grade 3), or patient mortality. The administration of Bevacizumab may influence the MRI and RANO assessments. Additionally, comparative data for treatment response were obtained from critically ill patients who voluntarily bore the high costs of fees or not. Further validation is warranted to establish the predictive efficacy of prognostic factors in treatment response and OS.
Conclusions
In conclusion, patients with higher T/N ratios derived from BPA-PET may initially exhibit a poor prognosis, but this outcome can potentially be reversed after BNCT. While BNCT may not be necessary to prolong the survival of all patients with malignant brain tumors, it becomes crucial when achieving an ORR, which appears to be closely associated with the values of T/N ratio. The use of BPA-PET as a screening tool can aid in patient selection for BNCT, ensuring that those most likely to benefit from the treatment receive it, thereby assisting clinicians in identifying patients with the highest likelihood of benefiting from BNCT. Further research is necessary to establish the quantitative assessment techniques in BPA-PET, explore the relationship between LAT1 expression and patient prognosis in solid tumor cases. Additionally, comparative data between patients who received BNCT and those who did not should be examined to gain further insights into treatment outcomes.
Acknowledgments
We extend our gratitude to Miss Wan-Tien Hsieh for her invaluable statistical assistance and her contributions to patient arrangement and data management throughout the study.
Funding: This work was made possible with the generous support of grant
Footnote
Reporting Checklist: The authors have completed the STROBE reporting checklist. Available at https://qims.amegroups.com/article/view/10.21037/qims-23-1769/rc
Conflicts of Interest: All authors have completed the ICMJE uniform disclosure form (available at https://qims.amegroups.com/article/view/10.21037/qims-23-1769/coif). All authors report that this work was made possible with the generous support of grant V112C-192 from the esteemed Taipei Veterans General Hospital. This study received partial support from grants provided by the Ministry of Science and Technology of Taiwan (MOST110-2314-B-075-030-MY3) and the Melissa Lee Cancer Foundation. The authors have no other conflicts of interest to declare.
Ethical Statement: The authors are accountable for all aspects of the work in ensuring that questions related to the accuracy or integrity of any part of the work are appropriately investigated and resolved. The study was conducted in accordance with the Declaration of Helsinki (as revised in 2013). The study was approved by the institutional review board of Taipei Veterans General Hospital (IRB No. 2022-07-0029CC). Due to the retrospective nature of the study, written or verbal informed consent was waived.
Open Access Statement: This is an Open Access article distributed in accordance with the Creative Commons Attribution-NonCommercial-NoDerivs 4.0 International License (CC BY-NC-ND 4.0), which permits the non-commercial replication and distribution of the article with the strict proviso that no changes or edits are made and the original work is properly cited (including links to both the formal publication through the relevant DOI and the license). See: https://creativecommons.org/licenses/by-nc-nd/4.0/.
References
- Slatkin DN. A history of boron neutron capture therapy of brain tumours. Postulation of a brain radiation dose tolerance limit. Brain 1991;114:1609-29. [Crossref] [PubMed]
- He H, Li J, Jiang P, Tian S, Wang H, Fan R, Liu J, Yang Y, Liu Z, Wang J. The basis and advances in clinical application of boron neutron capture therapy. Radiat Oncol 2021;16:216. [Crossref] [PubMed]
- Malouff TD, Seneviratne DS, Ebner DK, Stross WC, Waddle MR, Trifiletti DM, Krishnan S. Boron Neutron Capture Therapy: A Review of Clinical Applications. Front Oncol 2021;11:601820. [Crossref] [PubMed]
- Watanabe T, Sanada Y, Hattori Y, Suzuki M. Correlation between the expression of LAT1 in cancer cells and the potential efficacy of boron neutron capture therapy. J Radiat Res 2023;64:91-8. [Crossref] [PubMed]
- Miyatake SI, Kawabata S, Hiramatsu R, Kuroiwa T, Suzuki M, Ono K. Boron Neutron Capture Therapy of Malignant Gliomas. Prog Neurol Surg 2018;32:48-56. [Crossref] [PubMed]
- Lan TL, Chou FI, Lin KH, Pan PS, Lee JC, Huang WS, Liu YM, Chao Y, Chen YW. Using salvage Boron Neutron Capture Therapy (BNCT) for recurrent malignant brain tumors in Taiwan. Appl Radiat Isot 2020;160:109105. [Crossref] [PubMed]
- Kageji T, Nagahiro S, Mizobuchi Y, Matsuzaki K, Nakagawa Y, Kumada H. Boron neutron capture therapy (BNCT) for newly-diagnosed glioblastoma: comparison of clinical results obtained with BNCT and conventional treatment. J Med Invest 2014;61:254-63. [Crossref] [PubMed]
- Furuse M, Kawabata S, Wanibuchi M, Shiba H, Takeuchi K, Kondo N, Tanaka H, Sakurai Y, Suzuki M, Ono K, Miyatake SI. Boron neutron capture therapy and add-on bevacizumab in patients with recurrent malignant glioma. Jpn J Clin Oncol 2022;52:433-40. [Crossref] [PubMed]
- Chen YW, Lee YY, Lin CF, Pan PS, Chen JK, Wang CW, et al. Salvage Boron Neutron Capture Therapy for Malignant Brain Tumor Patients in Compliance with Emergency and Compassionate Use: Evaluation of 34 Cases in Taiwan. Biology (Basel) 2021;10:334. [Crossref] [PubMed]
- Chen YW, Lee YY, Lin CF, Huang TY, Ke SH, Mu PF, et al. Compassionate Treatment of Brainstem Tumors with Boron Neutron Capture Therapy: A Case Series. Life (Basel) 2022;12:566. [Crossref] [PubMed]
- Wang LW, Chen YW, Ho CY, Hsueh Liu YW, Chou FI, Liu YH, Liu HM, Peir JJ, Jiang SH, Chang CW, Liu CS, Lin KH, Wang SJ, Chu PY, Lo WL, Kao SY, Yen SH. Fractionated Boron Neutron Capture Therapy in Locally Recurrent Head and Neck Cancer: A Prospective Phase I/II Trial. Int J Radiat Oncol Biol Phys 2016;95:396-403. [Crossref] [PubMed]
- Wang LW, Wang SJ, Chu PY, Ho CY, Jiang SH, Liu YW, Liu YH, Liu HM, Peir JJ, Chou FI, Yen SH, Lee YL, Chang CW, Liu CS, Chen YW, Ono K. BNCT for locally recurrent head and neck cancer: preliminary clinical experience from a phase I/II trial at Tsing Hua Open-Pool Reactor. Appl Radiat Isot 2011;69:1803-6. [Crossref] [PubMed]
- Hiratsuka J, Kamitani N, Tanaka R, Tokiya R, Yoden E, Sakurai Y, Suzuki M. Long-term outcome of cutaneous melanoma patients treated with boron neutron capture therapy (BNCT). J Radiat Res 2020;61:945-51. [Crossref] [PubMed]
- Fujimoto T, Suzuki M, Sudo T, Fujita I, Sakuma T, Sakurai Y, et al. Boron neutron capture therapy for clear cell sarcoma. Appl Radiat Isot 2020;166:109324. [Crossref] [PubMed]
- Lin SY, Lin CJ, Liao JW, Peir JJ, Chen WL, Chi CW, Lin YC, Liu YM, Chou FI. Therapeutic efficacy for hepatocellular carcinoma by boric acid-mediated boron neutron capture therapy in a rat model. Anticancer Res 2013;33:4799-809. [PubMed]
- Wen PY, Macdonald DR, Reardon DA, Cloughesy TF, Sorensen AG, Galanis E, Degroot J, Wick W, Gilbert MR, Lassman AB, Tsien C, Mikkelsen T, Wong ET, Chamberlain MC, Stupp R, Lamborn KR, Vogelbaum MA, van den Bent MJ, Chang SM. Updated response assessment criteria for high-grade gliomas: response assessment in neuro-oncology working group. J Clin Oncol 2010;28:1963-72. [Crossref] [PubMed]
- Chang TY, Chang WY, Chen YW, Ho MC, Chang CW, Lau SO, Peng NJ, Wu CY. Comparison of the synthesis and biological properties of no-carrier-added and carrier-added 4-borono-2-[18F]fluorophenylalanine ([18F]FBPA). Nucl Med Biol 2023;116-117:108313. [Crossref] [PubMed]
- Wittig A, Sheu-Grabellus SY, Collette L, Moss R, Brualla L, Sauerwein W. BPA uptake does not correlate with LAT1 and Ki67 expressions in tumor samples (results of EORTC trial 11001). Appl Radiat Isot 2011;69:1807-12. [Crossref] [PubMed]
- Lu JJ, Li P, Yang Y, Wang L, Zhang Y, Zhu JY, Zhu XR, Chen MB. Prognostic value of LAT-1 status in solid cancer: A systematic review and meta-analysis. PLoS One 2020;15:e0233629. [Crossref] [PubMed]
- Chanana AD, Capala J, Chadha M, Coderre JA, Diaz AZ, Elowitz EH, Iwai J, Joel DD, Liu HB, Ma R, Pendzick N, Peress NS, Shady MS, Slatkin DN, Tyson GW, Wielopolski L. Boron neutron capture therapy for glioblastoma multiforme: interim results from the phase I/II dose-escalation studies. Neurosurgery 1999;44:1182-92; discussion 1192-3. [PubMed]
- Henriksson R, Capala J, Michanek A, Lindahl SA, Salford LG, Franzén L, Blomquist E, Westlin JE, Bergenheim ATSwedish Brain Tumour Study Group. Boron neutron capture therapy (BNCT) for glioblastoma multiforme: a phase II study evaluating a prolonged high-dose of boronophenylalanine (BPA). Radiother Oncol 2008;88:183-91. [Crossref] [PubMed]
- Kawabata S, Miyatake S, Nonoguchi N, Hiramatsu R, Iida K, Miyata S, Yokoyama K, Doi A, Kuroda Y, Kuroiwa T, Michiue H, Kumada H, Kirihata M, Imahori Y, Maruhashi A, Sakurai Y, Suzuki M, Masunaga S, Ono K. Survival benefit from boron neutron capture therapy for the newly diagnosed glioblastoma patients. Appl Radiat Isot 2009;67:S15-8. [Crossref] [PubMed]
- Kageji T, Mizobuchi Y, Nagahiro S, Nakagawa Y, Kumada H. Clinical results of boron neutron capture therapy (BNCT) for glioblastoma. Appl Radiat Isot 2011;69:1823-5. [Crossref] [PubMed]
- Miyatake S, Kawabata S, Hiramatsu R, Kuroiwa T, Suzuki M, Kondo N, Ono K. Boron Neutron Capture Therapy for Malignant Brain Tumors. Neurol Med Chir (Tokyo) 2016;56:361-71. [Crossref] [PubMed]
- Carson KA, Grossman SA, Fisher JD, Shaw EG. Prognostic factors for survival in adult patients with recurrent glioma enrolled onto the new approaches to brain tumor therapy CNS consortium phase I and II clinical trials. J Clin Oncol 2007;25:2601-6. [Crossref] [PubMed]
- Miyatake S, Kawabata S, Yokoyama K, Kuroiwa T, Michiue H, Sakurai Y, Kumada H, Suzuki M, Maruhashi A, Kirihata M, Ono K. Survival benefit of Boron neutron capture therapy for recurrent malignant gliomas. J Neurooncol 2009;91:199-206. [Crossref] [PubMed]
- Miyatake S, Kawabata S, Kajimoto Y, Aoki A, Yokoyama K, Yamada M, Kuroiwa T, Tsuji M, Imahori Y, Kirihata M, Sakurai Y, Masunaga S, Nagata K, Maruhashi A, Ono K. Modified boron neutron capture therapy for malignant gliomas performed using epithermal neutron and two boron compounds with different accumulation mechanisms: an efficacy study based on findings on neuroimages. J Neurosurg 2005;103:1000-9. [Crossref] [PubMed]
- Advances in boron neutron capture therapy. Non-serial publications, IAEA, Vienna, 2023.
- Hirose K, Konno A, Hiratsuka J, Yoshimoto S, Kato T, Ono K, et al. Boron neutron capture therapy using cyclotron-based epithermal neutron source and borofalan ((10)B) for recurrent or locally advanced head and neck cancer (JHN002): An open-label phase II trial. Radiother Oncol 2021;155:182-7. [Crossref] [PubMed]
- Matsumura A, Asano T, Hirose K, Igaki H, Kawabata S, Kumada H. Initiatives Toward Clinical Boron Neutron Capture Therapy in Japan. Cancer Biother Radiopharm 2023;38:201-7. [Crossref] [PubMed]
- Monti Hughes A, Hu N. Optimizing Boron Neutron Capture Therapy (BNCT) to Treat Cancer: An Updated Review on the Latest Developments on Boron Compounds and Strategies. Cancers (Basel) 2023;15:4091. [Crossref] [PubMed]
- Ishiwata K. 4-Borono-2-(18)F-fluoro-L-phenylalanine PET for boron neutron capture therapy-oriented diagnosis: overview of a quarter century of research. Ann Nucl Med 2019;33:223-36. [Crossref] [PubMed]
- Kashihara T, Mori T, Nakaichi T, Nakamura S, Ito K, Kurihara H, Kusumoto M, Itami J, Yoshimoto S, Igaki H. Correlation between L-amino acid transporter 1 expression and 4-borono-2-(18) F-fluoro-phenylalanine accumulation in humans. Cancer Med 2023;12:20564-72. [Crossref] [PubMed]
- Minoji T, Takahashi H, Watabe T, Ishikawa S, Kato H, Ohgaki R, Kanai Y, Sekido Y, Hata T, Hamabe A, Ogino T, Miyoshi N, Uemura M, Yamamoto H, Doki Y, Eguchi H. The Clinical Potential of FBPA-PET/CT Imaging for Colorectal Cancer and its Liver Metastases Expressing LAT1. Anticancer Res 2023;43:3913-21. [Crossref] [PubMed]
- Watabe T, Ose N, Naka S, Fukui E, Kimura T, Kanou T, Funaki S, Sasaki H, Kamiya T, Kurimoto K, Isohashi K, Tatsumi M, Shimosegawa E, Kato H, Ohgaki R, Kanai Y, Shintani Y. Evaluation of LAT1 Expression in Patients With Lung Cancer and Mediastinal Tumors: 18F-FBPA PET Study With Immunohistological Comparison. Clin Nucl Med 2023;48:853-60. [Crossref] [PubMed]