Susceptibility vessel sign in patients with subacute ischemic stroke
Introduction
More than one-third of acute ischemic stroke (AIS) cases are caused by intracranial large vessel occlusion (LVO), which is associated with a higher disability risk and mortality (1). Cardioembolism (CE) and large artery arteriosclerosis (LAA) are the two main causes of LVO stroke (2). Due to the distinct responses to early reperfusion therapy, including mechanical thrombectomy and intravenous thrombolysis, as well as to inform the selection of antithrombotic regimens for secondary prevention between the LAA subtype and CE subtype, it is essential to differentiate between these two subtypes of LVO stroke (3).
Susceptibility vessel sign (SVS) appears as a dark, blooming, visible artifact on the susceptibility-weighted imaging (SWI) sequence due to the magnetic susceptibility effect of deoxygenated hemoglobin in the red blood cells (RBCs) trapped in occluded vessels (4). In addition to being a specific marker for acute clot in large intracranial vessels—with a positive predictive value of nearly 95%—SVS has been reported to play a role in the etiology diagnosis of LVO stroke (5). The CE subtype has been found to have a higher incidence of SVS than the LAA subtype in the acute stage (6-8). The majority of studies examining this phenomenon have focused on SVS within the first 24 hours after stroke onset. In reality, due to equipment availability and patient tolerance, many patients with LVO stroke undergo MRI examinations at a later time point. Nevertheless, there is limited information on SVS found beyond 24 hours in stroke survivors.
In this study, we aimed to compare the presence and quantitative measurement of SVS between the LAA subtype and CE subtype between day 3 and day 14 after stroke onset and to assess whether SVS found at the subacute stage can aid in determining the stroke subtype in LVO stroke survivors. We present this article in accordance with the STROBE reporting checklist (available at https://qims.amegroups.com/article/view/10.21037/qims-23-1797/rc).
Methods
Study population
We retrospectively reviewed consecutive patients survivors admitted to the Department of Neurology, Peking University First Hospital, between December 2017 and January 2022. We enrolled patients who met the following criteria: (I) a diagnosis of ischemic stroke confirmed by diffusion-weighted imaging (DWI); (II) SWI performed between day 3 and day 14 after stroke onset; (III) an occlusion or severe stenosis of >70% of the responsible intracranial large artery as confirmed by magnetic resonance angiography (MRA), computed tomography angiography (CTA), or digital subtraction angiography (DSA); and (IV) stroke subtype classified as LAA or CE according to the Trial of OG 10172 in Acute Stroke Treatment (TOAST) classification (9). We excluded those patients who had received intravenous thrombolysis or mechanical thrombectomy after stroke onset or had poor SWI image quality. This study was conducted in accordance with the Declaration of Helsinki (as revised in 2013) and was approved by the Ethics Committee of Peking University First Hospital (No. 2024-020). Individual consent for this retrospective analysis was waived.
Clinical information
We abstracted the following patient demographic and clinical information from medical records: age; sex; medical histories such as hypertension, diabetes mellitus, hyperlipidemia, atrial fibrillation, coronary artery disease, history of previous stroke, smoking, and drinking; National Institute of Health Stroke Scale (NIHSS) score (10); levels of D-dimer and fibrinogen degradation product (FDP) at admission; and the subtype of stroke classified according to the TOAST classification (9).
Neuroimaging
All patients received magnetic resonance imaging (MRI) with a 3-tesla unit (GE HealthCare, Chicago, IL, USA), including T1, SWI, DWI, and fluid-attenuated inversion recovery (FLAIR). Axial SWI was obtained under the following parameters: repetition time (TR)/echo time (TE), 2,000/30 ms; field of view (FOV), 240 mm2; and slice thickness, 2 mm.
Two neuroradiologists (Zhenying Zhan and J.L.), who were blinded to the patient’s clinical and laboratory data, assessed the presence and location of SVS and measured its length and diameter. SVS was defined as the presence of hypointense signs in the corresponding symptomatic vessels with a blooming artifact on SWI (Figure 1) (6). The location of SVS was categorized as occurring in the anterior cerebral artery (ACA), middle cerebral artery (MCA), posterior cerebral artery (PCA), or vertebral and basilar artery (BA). The in-plane length of the SVS (M1 and P1 segments) corresponded to the distance between the proximal and distal ends of the SVS. The length of the SVS perpendicular to the axial acquisition plane (BA, A1, A2, M2) was calculated by multiplying the number of cross-sectional locations where the SVS was visible by the slice thickness (6,11). Discrepancies regarding the presence and the location of SVS were resolved via consensus. The quantitative measurements (length and diameter) of the SVS obtained from both observers were averaged.
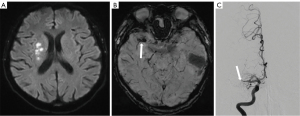
Statistical analysis
Continuous variables are expressed as the mean and standard deviation (SD) or as the median with interquartile range (IQR), and categorical variables are expressed as percentages. Demographic, clinical, and imaging characteristics were compared between the LAA group and CE group using the Mann-Whitney test, Student t-test, χ2 test, or Fisher exact test, as appropriate. The association of the presence of SVS with stroke subtype was estimated with univariate and multivariable binary logistic regression analyses and is presented as the odds ratio (OR) with the 95% confidence interval (CI). In the multivariable analysis, the confounders included age, sex, and the time interval between stroke onset and SWI imaging and NIHSS at admission. Additionally, in patients with SVS, we compared the length and diameter of SVS between the LAA and CE groups. Receiver operating characteristic (ROC) analysis was used to evaluate the discriminative power of SVS length for the LAA and CE subtypes, and the area under the curve (AUC) was used to determine the optimal cutoff and to quantify the performance.
We performed several sensitivity analyses to validate the relationship between SVS and the stroke subtypes. First, most patients did not undergo both MRI examination and vascular imaging on the same day. Among patients who had vascular imaging prior to the MRI test, some individuals might have experienced recanalization of the occluded artery prior to SWI imaging. Considering the potential influence of recanalization on SVS assessment, we performed a subgroup analysis by excluding patients who underwent vascular imaging prior to the MRI examination. Second, we explored the association of SVS and stroke subtype among individuals who received SWI 7 days after stroke onset. Third, we analyzed the association of SVS and atrial fibrillation. A two-tailed P value <0.05 was considered to be statistically significant. All analyses were conducted using SPSS 25.0 software (IBM Corp., Armonk, NY, USA).
Results
Patient population
There were 991 patients with suspected ischemic stroke admitted in our department between December 2017 and January 2022. Of these patients, 223 met the inclusion criteria of our study. After exclusion of 36 patients who had undergone intravenous thrombolysis or mechanical thrombectomy after stroke and 14 patients who had a poor quality of imaging, 173 patients were ultimately included in our analysis. Their mean age was 64.0±12.3 years, and 123 (71.1%) were male. There were 133 patients (76.9%) with the LAA subtype and 40 patients (23.1%) with the CE subtype. Compared to the CE group, the LAA group was younger and had a lower proportion of atrial fibrillation (Table 1). The were no significant differences in sex, medical history excluding atrial fibrillation, NIHSS score at admission, or levels of D-dimer and FDP between the two groups (Table 1).
Table 1
Characteristics | Total (n=173) | LAA group (n=133) | CE group (n=40) | P value |
---|---|---|---|---|
Age (years) | 64.0±12.3 | 62.8±11.2 | 68.1±15.0 | 0.044 |
Males | 123 (71.1) | 93 (69.9) | 30 (75.0) | 0.535 |
Hypertension | 134 (77.5) | 100 (75.2) | 34 (85.0) | 0.193 |
Diabetes mellitus | 82 (47.4) | 64 (48.1) | 18 (45.0) | 0.729 |
Hyperlipidemia | 92 (53.2) | 70 (52.6) | 22 (55.0) | 0.792 |
Atrial fibrillation | 27 (15.6) | 0 | 27 (67.5) | <0.001 |
Coronary artery disease | 24 (13.9) | 18 (13.5) | 6 (15.0) | 0.814 |
Previous stroke | 51 (29.5) | 12 (9.0) | 39 (97.5) | 0.862 |
Ever smoking | 91 (52.6) | 70 (52.6) | 21 (52.5) | 0.988 |
Ever drinking | 75 (43.4) | 60 (45.1) | 15 (37.5) | 0.394 |
NIHSS score at admission | 3 [2–7] | 3 [1–6] | 4 [2–10] | 0.213 |
D-dimer (mg/L) | 0.12 [0.70–0.27] | 0.11 [0.07–0.22] | 0.15 [0.06–0.49] | 0.107 |
FDP (mg/L) | 1.0 [0.5–2.2] | 0.9 [0.5–1.8] | 1.0 [0.6–3.5] | 0.205 |
Variables are displayed as the absolute number (percentage), the mean ± SD, or the median [25th–75th percentiles] as appropriate. LAA, large artery arteriosclerosis; CE, cardioembolism; NIHSS, National Institutes of Health Stroke Score; FDP, fibrinogen degradation product; SD, standard deviation.
Table 2 presents the imaging characteristics of the patients included in the study. An occlusion of the intracranial large artery responsible for the index stroke was found in 97 (56.1%) patients, and severe stenosis was observed in 76 (43.9%) patients. SVS was present in 95 patients (54.9%). The average time interval between stroke onset and MRI examination was similar between the two groups (LAA subtype: 7.4±3.5 days; CE subtype: 6.7±3.7 days; P=0.15). Additionally, there were no significant differences in the distribution of the responsible artery or in the proportion of occlusive lesions between the two groups. The frequency of SVS was significantly higher in the LAA group compared to the CE group (59.4% vs. 40.0%; P=0.031). After adjustments were made for age, sex, the time interval between stroke onset and MRI examination, and NIHSS score at admission, the association between LAA subtype and SVS remained (OR =2.199; 95% CI: 1.019–4.745; P=0.045), as presented in Table 3.
Table 2
Characteristics | Total (n=173) | LAA group (n=133) | CE group (n=40) | P value |
---|---|---|---|---|
Time from stroke onset to MRI examination (days) | 7.3±3.5 | 7.4±3.5 | 6.7±3.7 | 0.15 |
Distribution of responsible artery | 0.974 | |||
ACA | 11 (6.4) | 9 (6.8) | 2 (5.0) | |
MCA | 91 (52.6) | 69 (51.9) | 22 (55.0) | |
PCA | 26 (15.0) | 20 (15.0) | 6 (15.0) | |
Vertebral and BA | 45 (26.0) | 35 (26.3) | 10 (25.0) | |
Occlusion of responsible artery | 97 (56.1) | 75 (56.4) | 22 (55.0) | 0.876 |
SVS | 95 (54.9) | 79 (59.4) | 16 (40.0) | 0.031 |
Variables are displayed as the absolute number (percentage) or the mean ± SD as appropriate. LAA, large artery arteriosclerosis; CE, cardioembolism; MRI, magnetic resonance imaging; ACA, anterior cerebral artery; MCA, middle cerebral artery; PCA, posterior cerebral artery; BA, basilar artery; SVS, susceptibility vessel sign; SD, standard deviation.
Table 3
Variable | OR | 95% CI | P value |
---|---|---|---|
Age | 0.965 | 0.935–0.995 | 0.022 |
Male gender | 1.416 | 0.597–3.356 | 0.430 |
Time interval between stroke onset and MRI examination | 1.028 | 0.918–1.150 | 0.636 |
NHISS at admission | 0.942 | 0.870–1.021 | 0.145 |
Presence of SVS | 2.199 | 1.019–4.745 | 0.045 |
A multivariable logistic regression model was fitted, and the confounders included age, gender, time interval between stroke onset and MRI examination, and NHISS at admission. SVS, susceptibility vessel sign; OR, odds ratio; CI, confidence interval; MRI, magnetic resonance imaging; NIHSS, National Institutes of Health Stroke Score.
In the 95 patients with SVS, the average SVS length and diameter were 19.6±10.2 and 3.9±1.5 mm, respectively (Table 4). The most frequent location of SVS was the MCA (50.5%), followed by the vertebral artery and BA (23.2%). There was a significant difference in the length of SVS between the LAA group and the CE group (20.7±10.6 vs. 13.8±5.1 mm; P<0.001). Based on the ROC curve analysis, a cutoff length of 17.2 mm was suggested for distinguishing between the LAA subtype and the CE subtype, with a sensitivity of 54.4% and a specificity of 87.5%. The AUC value for the ROC curve was 0.71 (95% CI: 0.593–0.832), as shown in Figure 2. The diameter or the location distribution of SVS did not differ significantly between the two groups.
Table 4
Characteristics | Total (n=95) | LAA group (n=79) | CE group (n=16) | P value |
---|---|---|---|---|
SVS length (mm) | 19.6±10.2 | 20.7±10.6 | 13.8±5.1 | <0.001 |
SVS diameter (mm) | 3.9±1.5 | 3.9±1.6 | 3.6±1.1 | 0.50 |
Location of SVS | 0.551 | |||
ACA | 8 (8.4) | 6 (7.6) | 2 (12.5) | |
MCA | 48 (50.5) | 42 (53.2) | 6 (37.5) | |
PCA | 17 (17.9) | 13 (16.5) | 4 (25.0) | |
Vertebral and BA | 22 (23.2) | 18 (22.8) | 4 (25.0) |
Variables are displayed as the absolute number (percentage) or the mean ± SD as appropriate. SVS, susceptibility vessel sign; LAA, large artery arteriosclerosis; CE, cardioembolism; ACA, anterior cerebral artery; MCA, middle cerebral artery; PCA, posterior cerebral artery; BA, basilar artery; SD, standard deviation.
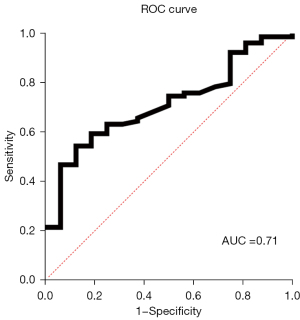
Our sensitivity analyses yielded similar results to our principal analysis. First, after 80 patients who had vascular imaging prior to MRI were excluded, the proportion of SVS remained higher in the LAA group than in the CE group (57.1% vs. 43.5%; P=0.25). Second, among patients who had SWI examinations between 7 and 14 days after stroke onset, the result did not change substantially. The LAA group exhibited a higher proportion of patients with SVS presence than did the CE group (70.1% vs. 40.9%; P=0.02). Third, there were 27 patients with atrial fibrillation in our study, and SVS was more common in the non-atrial fibrillation (AF) group than in the AF group (59.6% vs. 29.6%; P=0.04).
Discussion
The findings of this study indicated that in patients with subacute ischemic stroke caused by large intracranial vessel occlusion or severe stenosis, SVS was more prevalent in patients of the LAA subtype compared to the CE subtype. Moreover, of those patients with SVS, those in LAA group had a longer SVS than did those in the CE group.
Several studies have demonstrated a difference in SVS between the LAA subtype and CE subtype in LVO stroke survivors (6,7,12). In a study of 80 acute stroke survivors with intracranial arterial occlusion, Horie et al. (12) reported the presence of SVS found within 24 h after stroke onset to be significantly associated with the CE subtype (OR =21.6; 95% CI: 2.66–176.95; P=0.004). In another study, SVS was found in 86% of the patients with the CE subtype and 66% of the patients with LAA subtype patients (6), which represented a statistically significant difference (P=0.03) (6). Bourcier et al. (7) also reported that SVS within 6 hours of stroke onset was more prevalent in the CE subtype than in the LAA subtype (OR =2.14; 95% CI: 1.02–4.45; P=0.04). However, these studies were limited by their small sample size and a primary focus on acute stroke.
Compared to those of previous studies, our findings suggest that stroke patients with LAA subtype exhibit a higher incidence of SVS than do those with the CE subtype during the subacute stage. This discrepancy can be attributed to the different time points of the SWI examination. In our study, the patients underwent SWI imaging between 3 and 14 days following stroke onset, while other studies assessed SVS at an earlier time point, within 24 hours after stroke onset (6,7).
Some studies have demonstrated the evolution of intracranial blood clots with time (13). The conversion of oxyhemoglobin to deoxyhemoglobin occurs within a few hours. Therefore, during the hyperacute stage of stroke, the primary component may remain as oxyhemoglobin, which does not produce a magnetic susceptibility effect (14). In parallel, the presence of SVS in stroke survivors increases progressively from symptom onset: approximately 60% at 1 hour, 70% at 3 hours, 80% at 6 hours, and 90% at 8 hours (14). This implied time may be a critical determinant of the presence of SVS after stroke, and therefore, the observations of SVS during the acute stage after stroke cannot be extrapolated to the subacute stage without reservation.
Across a prolonged timeframe, Sato et al. (15) observed a significant difference in the composition of intracranial thrombus compared to the acute stage via autopsy. Recent pathological research suggests that atherosclerosis thrombus has a higher content of RBCs than does cardioembolic thrombus (16,17). The presence of more RBCs and deoxyhemoglobin in intracranial thrombus reportedly leads to a higher incidence of SVS in the LAA subtype, which is consistent with our findings. It has been proposed that in situ atherosclerotic thrombus expansion resulting in an increase of RBC-rich “red tails” could account for the differences in evolution patterns of thrombus components between the LAA and CE subtypes (18). In one study involving 120 stroke survivors who received T2* MRI upon admission and again 24 hours later, 34% of patients experienced elongation of SVS, thus supporting the abovementioned hypothesis (11).
Furthermore, the activation of the fibrinolytic system and spontaneous recanalization of occluded intracranial arteries poststroke could also impact the presence of SVS during the subacute stage. Compared to other stroke subtypes, CE has the highest spontaneous recanalization rate, which ranges from 18.8% to 72% in the literature (19-21). This phenomenon may contribute to the lower presence of SVS in the CE subtype during the subacute stage. However, our study only included patients with severe stenosis or occlusion of the responsible artery confirmed through vascular imaging. Therefore, the reduced incidence of SVS in the CE group cannot be attributed to spontaneous thrombolysis.
We also found that the incidence of SVS was lower in the CE subtype than in the LAA subtype. This suggests a distinct evolution pattern of SVS for the two subtypes after stroke. Therefore, we should be cautious when considering the influence of the assessment timepoint on the significance of SVS.
Another prominent finding of our study was that patients with the LAA subtype had a longer SVS compared to those with CE subtype, aligning with the recent findings reported by Alhazmi et al. (19). Moreover, a CT study found non-cardioembolic stroke to be associated with longer thrombus duration compared to stroke caused by CE (22). Furthermore, a histological study focusing on retrieved clot composition quantified clot fragments and confirmed that the LAA subtype had larger clots and higher RBC content than did the CE subtype (23).
Our study involves several limitations that should be addressed. First, due to the single-center design and small sample size, the study’s generalizability is limited. Although the sensitivity analysis, which included patients whose SWI examination was performed between 7 and 14 days after stroke onset, produced results consistent with our principal analysis, we cannot fully rule out the influence of random error. Second, some patients did not undergo MRI and vascular imaging on the same day. The possibility of recanalization of the occluded artery prior to SWI imaging may affect the evaluation of the significance of SVS. However, even after exclusion of patients who underwent vascular imaging prior to MRI examination, the sensitivity analysis still showed a tendency for higher rates of SVS in the LAA group, supporting our main results. Third, we used the TOAST classification to divide patients into the CE and LAA groups. The relevant literature suggests that the TOAST classification may overestimate the incidence of cardiogenic embolism and be overly strict in diagnosing the LAA subtype (24). In the sensitivity analysis, we used atrial fibrillation as an alternative marker for CE subtype and found that the results still supported our primary findings. Fourth, because severely ill patients often cannot cooperate with MRI examination, our sample had relatively low NIHSS scores, thus limiting the generalizability of our results. Furthermore, our study revealed different patterns of SVS evolution between the LAA and CE subtypes. Given the cross-sectional design employed in this study, further longitudinal observation of SVS is necessary to validate our results and to evaluate the potential of SVS in clinical practice.
Conclusions
Our study found that in patients with subacute ischemic stroke due to intracranial LVO or severe stenosis, those with the LAA subtype had a higher incidence of SVS and a longer SVS compared to those with the CE subtype. This suggests that SVS may serve as a potential biomarker for determining the cause of stroke. Additional studies with dynamic observation are needed to confirm our findings and further elucidate the progression of SVS after stroke.
Acknowledgments
Funding: This work was supported by
Footnote
Reporting Checklist: The authors have completed the STROBE reporting checklist. Available at https://qims.amegroups.com/article/view/10.21037/qims-23-1797/rc
Conflicts of Interest: All authors have completed the ICMJE uniform disclosure form (available at https://qims.amegroups.com/article/view/10.21037/qims-23-1797/coif). The authors have no conflicts of interest to declare.
Ethical Statement: The authors are accountable for all aspects of the work in ensuring that questions related to the accuracy or integrity of any part of the work are appropriately investigated and resolved. This study was conducted in accordance with the Declaration of Helsinki (as revised in 2013) and was approved by the Ethics Committee of Peking University First Hospital (No. 2024-020). Individual consent for this retrospective analysis was waived.
Open Access Statement: This is an Open Access article distributed in accordance with the Creative Commons Attribution-NonCommercial-NoDerivs 4.0 International License (CC BY-NC-ND 4.0), which permits the non-commercial replication and distribution of the article with the strict proviso that no changes or edits are made and the original work is properly cited (including links to both the formal publication through the relevant DOI and the license). See: https://creativecommons.org/licenses/by-nc-nd/4.0/.
References
- Qin C, Zhao XL, Ma XT, Zhou LQ, Wu LJ, Shang K, Wang W, Tian DS. Proteomic profiling of plasma biomarkers in acute ischemic stroke due to large vessel occlusion. J Transl Med 2019;17:214. [Crossref] [PubMed]
- Ornello R, Degan D, Tiseo C, Di Carmine C, Perciballi L, Pistoia F, Carolei A, Sacco S. Distribution and Temporal Trends From 1993 to 2015 of Ischemic Stroke Subtypes: A Systematic Review and Meta-Analysis. Stroke 2018;49:814-9. [Crossref] [PubMed]
- Campbell BCV, De Silva DA, Macleod MR, Coutts SB, Schwamm LH, Davis SM, Donnan GA. Ischaemic stroke. Nat Rev Dis Primers 2019;5:70. [Crossref] [PubMed]
- Cho KH, Kim JS, Kwon SU, Cho AH, Kang DW. Significance of susceptibility vessel sign on T2*-weighted gradient echo imaging for identification of stroke subtypes. Stroke 2005;36:2379-83. [Crossref] [PubMed]
- Ritzenthaler T, Lacalm A, Cho TH, Maucort-Boulch D, Klaerke Mikkelsen I, Ribe L, Østergaard L, Hjort N, Fiehler J, Pedraza S, Louis Tisserand G, Baron JC, Berthezene Y, Nighoghossian N, Sequential MR. Assessment of the Susceptibility Vessel Sign and Arterial Occlusion in Acute Stroke. J Neuroimaging 2016;26:355-9. [Crossref] [PubMed]
- Kang DW, Jeong HG, Kim DY, Yang W, Lee SH. Prediction of Stroke Subtype and Recanalization Using Susceptibility Vessel Sign on Susceptibility-Weighted Magnetic Resonance Imaging. Stroke 2017;48:1554-9. [Crossref] [PubMed]
- Bourcier R, Derraz I, Delasalle B, Beaumont M, Soize S, Legrand L, Desal H, Bracard S, Naggara O, Oppenheim CTHRACE investigators. Susceptibility Vessel Sign and Cardioembolic Etiology in the THRACE Trial. Clin Neuroradiol 2019;29:685-92. [Crossref] [PubMed]
- Chen J, Zhang Z, Nie X, Xu Y, Liu C, Zhao X, Wang Y. Basilar artery thrombus magnetic susceptibility for cardioembolic stroke identification. Quant Imaging Med Surg 2022;12:1579-84. [Crossref] [PubMed]
- Goldstein LB, Jones MR, Matchar DB, Edwards LJ, Hoff J, Chilukuri V, Armstrong SB, Horner RD. Improving the reliability of stroke subgroup classification using the Trial of ORG 10172 in Acute Stroke Treatment (TOAST) criteria. Stroke 2001;32:1091-8. [Crossref] [PubMed]
- Lyden P, Lu M, Jackson C, Marler J, Kothari R, Brott T, Zivin J. Underlying structure of the National Institutes of Health Stroke Scale: results of a factor analysis. NINDS tPA Stroke Trial Investigators. Stroke 1999;30:2347-54. [Crossref] [PubMed]
- Seners P, Hurford R, Tisserand M, Turc G, Legrand L, Naggara O, Mas JL, Oppenheim C, Baron JC. Is Unexplained Early Neurological Deterioration After Intravenous Thrombolysis Associated With Thrombus Extension? Stroke 2017;48:348-52. [Crossref] [PubMed]
- Horie N, Tateishi Y, Morikawa M, Morofuji Y, Hayashi K, Izumo T, Tsujino A, Nagata I, Matsuo T. Acute stroke with major intracranial vessel occlusion: Characteristics of cardioembolism and atherosclerosis-related in situ stenosis/occlusion. J Clin Neurosci 2016;32:24-9. [Crossref] [PubMed]
- Kim YD, Nam HS, Kim SH, Kim EY, Song D, Kwon I, Yang SH, Lee K, Yoo J, Lee HS, Heo JH. Time-Dependent Thrombus Resolution After Tissue-Type Plasminogen Activator in Patients With Stroke and Mice. Stroke 2015;46:1877-82. [Crossref] [PubMed]
- Soize S, Manceau PF, Gauberti M, Herbin T, Zuber M, Pierot L, Touzé E. Susceptibility Vessel Sign in Relation With Time From Onset to Magnetic Resonance Imaging. Stroke 2021;52:1839-42. [Crossref] [PubMed]
- Sato Y, Ishibashi-Ueda H, Iwakiri T, Ikeda Y, Matsuyama T, Hatakeyama K, Asada Y. Thrombus components in cardioembolic and atherothrombotic strokes. Thromb Res 2012;130:278-80. [Crossref] [PubMed]
- Boeckh-Behrens T, Kleine JF, Zimmer C, Neff F, Scheipl F, Pelisek J, Schirmer L, Nguyen K, Karatas D, Poppert H. Thrombus Histology Suggests Cardioembolic Cause in Cryptogenic Stroke. Stroke 2016;47:1864-71. [Crossref] [PubMed]
- Brinjikji W, Nogueira RG, Kvamme P, Layton KF, Delgado Almandoz JE, Hanel RA, et al. Association between clot composition and stroke origin in mechanical thrombectomy patients: analysis of the Stroke Thromboembolism Registry of Imaging and Pathology. J Neurointerv Surg 2021;13:594-8. [Crossref] [PubMed]
- Qazi EM, Sohn SI, Mishra S, Almekhlafi MA, Eesa M, d'Esterre CD, Qazi AA, Puig J, Goyal M, Demchuk AM, Menon BK. Thrombus Characteristics Are Related to Collaterals and Angioarchitecture in Acute Stroke. Can J Neurol Sci 2015;42:381-8. [Crossref] [PubMed]
- Alhazmi H, Bani-Sadr A, Bochaton T, Paccalet A, Da Silva CC, Buisson M, Amaz C, Ameli R, Berthezene Y, Eker OF, Ovize M, Cho TH, Nighoghossian N, Mechtouff L. Large vessel cardioembolic stroke and embolic stroke of undetermined source share a common profile of matrix metalloproteinase-9 level and susceptibility vessel sign length. Eur J Neurol 2021;28:1977-83. [Crossref] [PubMed]
- Molina CA, Montaner J, Abilleira S, Ibarra B, Romero F, Arenillas JF, Alvarez-Sabín J. Timing of spontaneous recanalization and risk of hemorrhagic transformation in acute cardioembolic stroke. Stroke 2001;32:1079-84. [Crossref] [PubMed]
- Vanacker P, Lambrou D, Eskandari A, Ntaios G, Cras P, Maeder P, Meuli R, Michel P. Improving the Prediction of Spontaneous and Post-thrombolytic Recanalization in Ischemic Stroke Patients. J Stroke Cerebrovasc Dis 2015;24:1781-6. [Crossref] [PubMed]
- Boodt N, Compagne KCJ, Dutra BG, Samuels N, Tolhuisen ML, Alves HCBR, Kappelhof M, Lycklama À, Nijeholt GJ, Marquering HA. Majoie CBLM, Lingsma HF, Dippel DWJ, van der Lugt A; Coinvestigators MR CLEAN Registry. Stroke Etiology and Thrombus Computed Tomography Characteristics in Patients With Acute Ischemic Stroke: A MR CLEAN Registry Substudy. Stroke 2020;51:1727-35. [Crossref] [PubMed]
- Fitzgerald S, Rossi R, Mereuta OM, Jabrah D, Okolo A, Douglas A, et al. Per-pass analysis of acute ischemic stroke clots: impact of stroke etiology on extracted clot area and histological composition. J Neurointerv Surg 2021;13:1111-6. [Crossref] [PubMed]
- Zhang H, Li Z, Dai Y, Guo E, Zhang C, Wang Y. Ischaemic stroke etiological classification system: the agreement analysis of CISS, SPARKLE and TOAST. Stroke Vasc Neurol 2019;4:123-8. [Crossref] [PubMed]