Hypoxia predicts favorable response to carbon ion radiotherapy in non-small cell lung cancer (NSCLC) defined by 18F-FMISO positron emission tomography/computed tomography (PET/CT) imaging
Introduction
Lung cancer is the leading cause of cancer-related deaths worldwide. Approximately 80–85% of lung cancer patients are classified as non-small cell lung cancer (NSCLC) (1). Oxygen deprivation (hypoxia) is related to poor prognosis and indicates a poor response to tumor treatments in NSCLC, including photon radiotherapy (2). Thus, hypoxia is a promising treatment target in the setting of photon radiotherapy, which is delivered to over 50% of patients with NSCLC (3). However, trials of hypoxia-targeted therapies for NSCLC have not yet translated to benefits for patients. A variety of approaches have been undertaken to overcome or reverse tumor hypoxia but failed due to restricted efficacy and/or unwanted side effects (4).
Carbon ion radiotherapy (CIRT) acts independently of the oxygen concentration and triggers complex and clustered DNA double strand breaks (DSBs) difficult to repair (5). With low-linear energy transfer (LET) radiation such as X-ray radiation, the radiation resistance of tumor cells under hypoxic conditions is 2 to 3 times higher than that under normoxic conditions (6). As for high-LET radiation such as carbon ion, the oxygen enhancement ratio (OER) can reach 1 (7). Thus, CIRT is considered more efficient than X-ray and proton therapy for hypoxic tumors and could eradicate radioresistant tumors in clinic (8,9).
The most direct way used to detect tumor hypoxia is the Eppendorf O2 polarographic needle electrode. However, it has never been adopted into a clinical routine because of its invasive nature and lack of spatial and temporal resolution (10). Instead, hypoxia positron emission tomography (PET) tracers such as 18F-fluoromisonidazole (18F-FMISO) have been extensively studied to monitor tumor hypoxia non-invasively (11). 18F-FMISO PET/computed tomography (CT) has also been reported suitable for monitoring therapeutic efficiency in NSCLC patients (12), thereby providing information for the design of response-adapted therapy strategies.
Hence, we used 18F-FMISO as a PET probe to monitor the changes of hypoxia before and after CIRT in NSCLC patients in this study. Moreover, the capability of 18F-FMISO PET/CT to predict the response to CIRT in NSCLC patients was also analyzed. We present this article in accordance with the STROBE reporting checklist (available at https://qims.amegroups.com/article/view/10.21037/qims-23-1685/rc).
Methods
Patient characteristics and treatment
We retrospectively analyzed stage II–III NSCLC patients treated with CIRT who were previously enrolled in a prospective trial comparing photon radiation therapy with CIRT. The study was conducted in accordance with the Declaration of Helsinki (as revised in 2013). The study was approved by the institutional review board of the Shanghai Proton and Heavy Ion Center (SPHIC) (ethical code: 1707-16-03-1804A) and the requirement for individual consent for this retrospective analysis was waived. A total of 29 patients with NSCLC who were treated with CIRT in SPHIC from April 2018 to November 2021 were retrospectively enrolled, including stage T1-4N0-3M0 (7 cases in stage II and 22 cases in stage III). All of the 29 patients met the following criteria: (I) primary lesions were confirmed by pathology; (II) inoperable or refusal of surgery; (III) the target lung lesions can be measured; (IV) 18F-FMISO PET/CT scans were performed within 1 week before and after radiotherapy; (V) informed consent was provided by patients who underwent CIRT. The exclusion criteria included the following: (I) inability to cooperate with 18F-FMISO PET/CT examination; (II) poor PET/CT image quality or small lung lesions (diameter <1.0 cm) affecting tumor segmentation; (III) interruption of radiotherapy by various factors.
All patients were treated with CIRT alone, with no concurrent chemotherapy. Systemic therapies were administered sequentially in most of the patients (26/29) at the physicians’ discretion in or out of SPHIC. The carbon ion dose was a relative biological effectiveness-weighted dose (DRBE) in units of Gray (Gy). We delivered 72.0–83.6 Gy/20–22 Fx in nearly all of the patients, except for 3 of them, who received 60 Gy/10 Fx, 63 Gy/18 Fx, and 64 Gy/16 Fx, respectively. We delivered carbon ion beams for NSCLC with different dose fractionation based on the position of the tumor. Furthermore, the biological effective doses were all close to or more than 100 Gy, which has been shown to produce excellent clinical outcomes for NSCLC (13). The pencil beam scanning (PBS) technology was used for CIRT with the Siemens IONTRIS system (Siemens, Erlangen, Germany), and treatment planning was conducted using the Siemens Syngo treatment planning system (versions VC 11 & 13). The PBS plan was implemented by multi-field optimization with 2–3 fields. The respiratory gating system (AZ-773V; Anzai Medical, Tokyo, Japan) was utilized to detect the external respiratory motion in real-time during CT scan and beam delivery. After radiotherapy, the immediate radiotherapy response and early response (ER) were evaluated using enhanced chest CT examination with the response evaluation criteria in solid tumors 1.1 (RECIST 1.1) (14). Immediate response (IR) refers to the evaluation within 1 week after the completion of all radiotherapy. ER refers to the evaluation at the first follow-up visit after radiotherapy (3–4 months after the completion of CIRT). Patients with complete response (CR) or partial response (PR) were ascribed to responders, and those with stable disease (SD) or progressive disease (PD) were ascribed to non-responders.
18F-FMISO PET/CT scan
18F-FMISO was produced by a cyclotron (RDS Eclips ST; Siemens CTI) and GN (Siemens) module using 1-(2'-nitro-1'-imidazolyl)-2-O-tetrahydropyranyl-3-O-tosyl-propanediol as a labeling precursor. Radio-high-performance liquid chromatography (HPLC) test showed good stability of the 18F-FMISO injection with radiochemical purity exceeding 99% in 3 half-lives. All PET/CT scans were acquired on a Biograph 16 HR PET/CT (Siemens) operating in 3-dimensional, high-resolution mode. No fasting was required before imaging. All patients were injected intravenously with 370 MBq (4.81 mSv) of 18F-FMISO. At 4 hours after injection, static emission scans were obtained. The data acquisition procedure was as follows: The respiratory-gated CT scans were performed first (a slice thickness of 3.0 mm) with 120 kVp, 150 mAs, and 0.33 s per rotation and reconstructed to a 512×512 matrix (voxel size: 0.98×0.98×3.0 mm3). Immediately after CT, a 10-minute PET scan in 2-bed position was performed. A TrueX algorithm (2 iterations, 24 subsets, and 2 mm full width at half maximum) with time-of-flight information was used to reconstruct the PET images. For all PET reconstructions, the anisotropic voxels were 4.07×4.07×3.0 mm3 because the matrix size was 200×200. The PET images were converted into standard uptake value (SUV) units by normalizing the activity concentration to the dosage of injected 18F-FMISO and patient body weight.
18F-FMISO PET/CT image analysis
The region of interest (ROI) of the primary tumors was delineated on PET/CT images by gradient method (MIM software, PET-Edge tool; MIM Software Inc., Cleveland, OH, USA) (15). The PET/CT parameters involved in this study include tumor volume (TV), tumor-to-muscle ratio (TMR), hypoxia volume (HV), ΔX, and maximum standard uptake value (SUVmax). TV and SUVmax were automatically calculated by MIM software. TMR was calculated as follows: TMR=T/M. An elliptic ROI was drawn manually around the primary lesion to measure SUVmax for 18F-FMISO uptake which was noted as T. Additionally, 6 small spheres of 0.5×0.5×0.5 cm (background) were located at the bilateral triceps brachii muscles, scapula muscles, and latissimus dorsi muscles. The average value of SUVmax of the six background ROI was noted as M. TMR ≥1.4 was used as the cut-off value of hypoxia because NSCLC is less hypoxic than other types of tumors, which may be due to the unique lung parenchyma structure, namely double blood supply and abundant oxygen-containing cavities (16,17). The patients were divided into a hypoxia group and a non-hypoxia group according to the hypoxia threshold. HV was defined as the TV with TMR ≥1.4. The change of HV and TMR after radiation was designated as ΔX, and ΔX = (X0−X1)/X0 × 100%, where X0 was the parameter just before radiation, X1 was the parameter after radiation.
Statistical analysis
The software SPSS 22.0 (IBM Corp., Armonk, NY, USA) was used for the statistical analysis. A 2-sided P<0.05 was considered statistically significant. The recorded quantitative data that did not conform to normal distribution were represented by M (Q1, Q3), and the classified data were expressed as frequency and percentage. Wilcoxon signed rank test was used to compare the changes of TV, HV and TMR at baseline and after CIRT. The difference of radiotherapy response between 2 groups was compared by Fisher’s exact test. Mann-Whitney U test was used to compare the difference of parameters between responders and non-responders. A binary logistic regression analysis was performed to evaluate the impact of PET hypoxic parameters on the prediction of radiotherapy response. The sensitivity and specificity in the predicting responses were calculated using areas under the receiver operating characteristic (ROC) curves (AUC).
Results
Demographic data in 29 stage II–III NSCLC patients treated with CIRT
Initially, 37 patients with NSCLC who were scheduled to receive CIRT were retrospectively enrolled in the study, 8 were excluded, and 29 patients were eventually included. The study flow chart and the reasons for negligibility are presented in Figure 1. The final 29 NSCLC patients (25 males and 4 females, mean age 63.8±11.0 years) included 17 squamous cell carcinomas (SCC), 10 adenocarcinoma (AC), 1 adenosquamous carcinoma (ASC), and 1 sarcomatoid carcinoma (SarC). The patient characteristics are listed in Table 1. All patients completed CIRT treatment as planned. At the first follow-up visit, 15 patients (51.7%) were early responders and the other 14 (48.3%) were non-responders. The differences of some important clinical factors such as patient age, gender, tumor location (left vs. right), pathology (SCC vs. non-SCC), clinic stage, and history of chemotherapy between the responder and non-responder group were analyzed, but no significant between-group difference was detected.
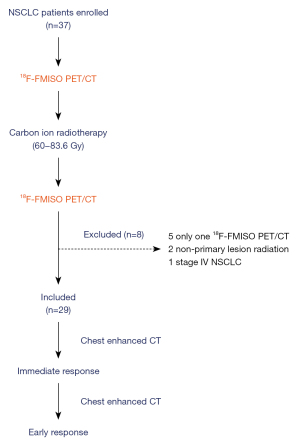
Table 1
Characteristics | Total (n=29) | Early responders (n=15) | Non-responders (n=14) | P value* |
---|---|---|---|---|
Age (years), mean ± SD | 63.8±11.0 | 63.3±10.4 | 64.4±12.1 | 0.68 |
Sex, n (%) | 0.32 | |||
Male | 25 (86.2) | 12 (80.0) | 13 (92.9) | |
Female | 4 (13.8) | 3 (20.0) | 1 (7.1) | |
Smoking history, n (%) | 0.36 | |||
Yes | 23 (79.3) | 11 (73.3) | 12 (85.7) | |
No | 6 (20.7) | 4 (26.7) | 2 (14.3) | |
BMI (kg/m2), n (%) | 0.16 | |||
>24.0 | 12 (41.4) | 8 (53.3) | 4 (28.6) | |
≤24.0 | 17 (58.6) | 7 (46.7) | 10 (71.4) | |
Tumor location, n (%) | 0.57 | |||
Left | 11 (27.9) | 7 (46.7) | 4 (28.6) | |
Right | 18 (62.1) | 8 (53.3) | 10 (71.4) | |
Histology, n (%) | 0.29 | |||
SCC | 17 (58.6) | 10 (66.7) | 7 (50.0) | |
Non-SCC | 12 (41.4) | 5 (33.3) | 7 (50.0) | |
Clinical stage, n (%) | 0.11 | |||
II | 7 (24.1) | 6 (40.0) | 1 (7.1) | |
III | 22 (75.9) | 9 (60.0) | 13 (92.9) | |
Clinical T status, n (%) | 0.60 | |||
1 | 1 (3.4) | 1 (6.7) | 0 (0) | |
2 | 6 (20.7) | 4 (26.7) | 2 (14.3) | |
3 | 15 (51.7) | 7 (46.7) | 8 (57.1) | |
4 | 7 (24.1) | 3 (20.0) | 4 (28.6) | |
Clinical N status, n (%) | 0.44 | |||
0 | 5 (17.2) | 4 (26.7) | 1 (7.1) | |
1 | 7 (24.1) | 4 (26.7) | 3 (21.4) | |
2 | 11 (37.9) | 5 (33.3) | 6 (42.9) | |
3 | 6 (20.7) | 2 (13.3) | 4 (28.6) | |
Clinical M status, n (%) | N/A | |||
0 | 29 (100.0) | 15 (100.0) | 14 (100.0) | |
1 | 0 (0) | 0 (0) | 0 (0) | |
History of chemotherapy, n (%) | 0.52 | |||
Yes | 26 (89.7) | 13 (86.7) | 13 (92.9) | |
No | 3 (10.3) | 2 (13.3) | 1 (7.1) |
*, comparison between responders and non-responders. SD, standard deviation; BMI, body mass index; SCC, squamous cell carcinoma; N/A, not applicable.
CIRT could alleviate hypoxia of NSCLC tumors
Taking the baseline TMR value of tumors ≥1.4 shown by the 18F-FMISO PET/CT as the hypoxia threshold, 72.4% lesions (21 of the 29 patients) showed hypoxia, and 27.6% (8 of the 29 patients) showed non-hypoxia, suggesting that hypoxia is a frequent feature of NSCLC tumors. In this study, it was observed that the TV in non-hypoxia group decreased significantly after radiotherapy [9.16 (3.67, 33.02) vs. 5.61 (1.83, 18.85) mL, Z=−2.100, P=0.03]. Meanwhile, a significant reduction in TV in the hypoxia group was also found after radiotherapy [55.95 (25.90, 82.47) vs. 29.94 (15.34, 48.14) mL, Z=−2.833, P=0.005] (Table S1). Both non-hypoxic and hypoxic NSCLC tumors responded to CIRT. We also observed that the HV of 52.4% (11/21) patients with hypoxic lesions decreased to 0 mL after treatment. Therefore, we compared TMR and HV changes in 21 NSCLC patients with hypoxia before and after CIRT to demonstrate the control of tumor hypoxia. The results revealed that after CIRT treatment, TMR and HV of the hypoxia group decreased significantly (Z=−3.354, P=0.001; Z=−3.389, P=0.001) (Table 2). The finding provides clinical evidence that CIRT could alleviate tumor hypoxia in NSCLC. Based on this result, we further focused on the relationship between the hypoxic PET image parameters and radiotherapy response.
Table 2
Groups | Hypoxia group (n=21) | |
---|---|---|
HV (mL) | TMR | |
Before treatment | 9.02 (2.92, 16.29) | 2.13 (1.81, 2.51) |
After treatment | 0.00 (0.00, 2.51) | 1.39 (1.25, 1.69) |
Z value | −3.389 | −3.354 |
P value | 0.001 | 0.001 |
Data are presented as median (interquartile range). CIRT, carbon ion radiation therapy; HV, hypoxia volume; TMR, tumor-to-muscle ratio.
Baseline TMR could predict the IR of CIRT in 21 NSCLC patients with hypoxic tumors
The IR was evaluated in the 21 patients with hypoxic tumors after radiotherapy, of which 5 were immediate responders and 16 were non-responders. 18F-FMISO PET/CT parameters were compared between immediate responders and non-responders in the 21 patients using Mann-Whitney U test (Table 3). The differences of HV0, TMR0, TMR1, and ΔTMR% between immediate responders and non-responders were P<0.1 in statistics [14.73 (9.12, 45.05) vs. 5.83 (2.38, 14.20) mL, P=0.07; 3.06 (2.18, 3.51) vs. 2.05 (1.63, 2.19), P=0.01; 1.26 (1.09, 1.39) vs. 1.44 (1.28, 1.85), P=0.07; 58.8 (39.1, 65.2) vs. 12.3 (5.1, 39.3), P=0.008]. Then, all the parameters with P<0.1 in Mann-Whitney U test were included in the binary logistic regression model to analyze the independent parameters associated with IR prediction. Finally, the data indicated that baseline TMR was the only significant predictor of the IR to CIRT. With ROC curve analysis, the optimal cut-off value of baseline TMR was 2.35 with a sensitivity of 80%, a specificity of 87.5%, an accuracy of 85.7%, and an AUC of 0.85 [95% confidence interval (CI): 0.62–1.00] (Figure S1A).
Table 3
Parameters | All patients (n =21) | Responders (n =5) (immediate) | Non-responders (n=16) (immediate) | P value* |
---|---|---|---|---|
HV0 (mL) | 9.02 (2.92, 16.29) | 14.73 (9.12, 45.05) | 5.83 (2.38, 14.20) | 0.07 |
HV1 (mL) | 0.00 (0.00, 2.51) | 0.00 (0.00, 0.43) | 0.15 (0.00, 5.47) | 0.20 |
ΔHV%† | 100.0 (42.7, 100.0) | 100.0 (95.5, 100.0) | 99.3 (14.9, 100.0) | 0.20 |
TMR0 | 2.13 (1.81, 2.51) | 3.06 (2.18, 3.51) | 2.05 (1.63, 2.19) | 0.02 |
TMR1 | 1.39 (1.25, 1.69) | 1.26 (1.09, 1.39) | 1.44 (1.28, 1.85) | 0.07 |
ΔTMR%† | 33.3 (8.6, 52.7) | 58.8 (39.1, 65.2) | 12.3 (5.1, 39.3) | 0.008 |
Data are presented as median (interquartile range). *, comparisons between responders and non-responders; †, the change of HV and TMR after radiation was designated as ΔX, and ΔX = (X0−X1)/X0 × 100%, where the X0 was the parameter just before radiation, X1 was the parameter after radiation. FMISO, fluoromisonidazole; RECIST, Response Evaluation Criteria in Solid Tumors; NSCLC, non-small cell lung cancer; CIRT, carbon ion radiation therapy; HV, hypoxia volume; TMR, tumor-to-muscle ratio.
There is a positive correlation between tumor hypoxia and response to CIRT
Taking the baseline TMR =2.35 as the cut-off value, 21 hypoxic patients were divided into a high-hypoxia group (n=6) and a low-hypoxia group (n=15). We found that the decrease of hypoxia degree in the high-hypoxia group after treatment was more obvious than that in the low-hypoxia group (Figure 2A). Thus, we analyzed the difference of IR between the 2 groups. The analysis found that there were 6 cases in the high-hypoxia group, 4 cases were immediate responders, 2 cases were non-responders, and the IR rate was 66.7% (4/6). Among the 15 cases in the low-hypoxia group, 1 case was an immediate responder, 14 cases were non-responders, and the IR rate was only 6.7% (1/15). The immediate radiotherapy responses of the 2 groups were completely different (P=0.01) (Figure 2B). The higher the baseline degree of tumor hypoxia, the better the IR to CIRT.
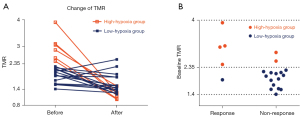
ΔTMR could predict the ER of CIRT in 21 NSCLC patients with hypoxic tumors
Since baseline TMR was able to predict IR, the ability of these parameters to predict the subsequent response was also analyzed. All patients were followed up with chest CT after treatment to evaluate the ER to CIRT. The initial follow-up of the 21 patients with hypoxic tumors showed that 11 were early responders and 10 were early non-responders. A comparison of 18F-FMISO PET/CT parameters between early responders and non-responders is shown in Table 4. We found that ΔTMR of 11 early responders was 44.0% (13.1%, 59.1%), and that of 10 early non-responders was 11.2% (−3.7%, 34.1%). The difference between the 2 groups was statistically significant (P=0.02). The data was then analyzed in the same way as for the IR to define the effective factors for predicting ER. The following analysis of binary logistic regression found that ΔTMR could predict ER at initial follow-up after CIRT. The optimal cut-off value for identifying early responders was 36.6% [sensitivity 72.7%, specificity 90%, accuracy 71.4%, AUC =0.80 (95% CI: 0.61–1.00)] (Figure S1B). Tumors with a higher ΔTMR% value, especially when ΔTMR% above 36.6%, exhibited a better ER to CIRT.
Table 4
Parameters | All patients (n =21) | Responders (n =11) (early) | Non-responders (n=10) (early) | P value* |
---|---|---|---|---|
HV0 (mL) | 9.02 (2.92, 16.29) | 13.13 (3.33, 21.04) | 5.83 (1.80, 11.63) | 0.17 |
HV1 (mL) | 0.00 (0.00, 2.51) | 0.00 (0.00, 0.20) | 0.88 (0.00, 5.94) | 0.17 |
ΔHV%† | 100.0 (42.7, 100.0) | 100.0 (99.2, 100.0) | 65.1 (−46.3, 100.0) | 0.09 |
TMR0 | 2.13 (1.81, 2.51) | 2.25 (2.13, 2.87) | 1.93 (1.69, 2.10) | 0.06 |
TMR1 | 1.39 (1.25, 1.69) | 1.27 (1.16, 1.52) | 1.50 (1.33, 2.03) | 0.04 |
ΔTMR%† | 33.3 (8.6, 52.7) | 44.0 (13.1, 59.1) | 11.2 (−3.7, 34.1) | 0.02 |
Data are presented as median (interquartile range). *, comparisons between responders and non-responders; †, the change of HV and TMR after radiation was designated as ΔX, and ΔX = (X0−X1)/X0×100%, where the X0 was the parameter just before radiation, X1 was the parameter after radiation. FMISO, fluoromisonidazole; RECIST, Response Evaluation Criteria in Solid Tumors; NSCLC, non-small cell lung cancer; CIRT, carbon ion radiation therapy; HV, hypoxia volume; TMR, tumor-to-muscle ratio.
ΔTMR is positively correlated with continued tumor shrinkage
By analyzing differences of TMR changes between early responders and non-responders, we found that hypoxia changed more obviously in the early responders [ΔTMR% =44.0 (13.1, 59.1)] than it did in the non-responders [ΔTMR% =11.2 (−3.7, 34.1)] (Figure 3A). Afterwards, we analyzed differences of ER according to a cut-off value of ΔTMR =36.6%. The monitored ER demonstrated that among the 9 patients with ΔTMR above 36.6%, 88.9% (8/9) had a response at the initial follow-up, and 11.1% (1/9) had no response. Of the 12 patients with ΔTMR lower than 36.6%, only 25.0% (3/12) had a response at the initial follow-up, and 75.0% (9/12) had no response (Figure 3B). The more pronounced the improvement of the degree of hypoxia, the better the effect of successive tumor regression.
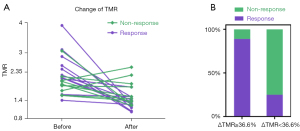
Typical responses of hypoxic tumors to CIRT
Two typical cases are shown in Figure 4. A 64-year-old male with clinical stage IIIc and SCC of the left lung showed a primary lesion with low-hypoxia. The patient received a total carbon ion radiation dose of 77 Gy in 22 fractions. The patient was assessed as a non-responder in IR and remained non-responder at the initial follow-up. 18F-FMISO PET/CT displayed the primary lesion with HV of 4.89 mL and TMR of 1.90 before CIRT. After CIRT, neither HV nor TMR changed significantly (4.89 vs. 5.99 mL, 1.90 vs. 1.85) (Figure 4A). For patients with high-hypoxic tumors, a typical case is shown in Figure 4B. A 62-year-old male who presented with stage IIIc and SCC of the left lung received a total dose of 72.6 Gy in 22 fractions showed a primary lesion with high-hypoxia. The patient was evaluated as a non-responder in IR, but responded at the initial follow-up. 18F-FMISO PET/CT demonstrated a primary lesion with HV of 21.04 mL and TMR of 2.57 before CIRT. After CIRT, the HV and TMR both decreased significantly to non-hypoxia in comparison with that before treatment (21.04 vs. 0 mL, 2.57 vs. 1.05).
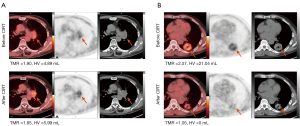
Discussion
In this study, we enrolled 29 NSCLC patients, most of whom showed remarkable remission of tumor hypoxia after CIRT. This is quite different from photon radiotherapy (18). CIRT is a novel radiotherapy tool with superior dose distribution and higher LET than conventional photon radiation, thus carbon ion acts as a direct ionization beam does not depend on tumor oxygen concentration to inhibit tumor (19). Therefore, in previous studies, carbon ion has been generally considered a powerful tool to inhibit tumor hypoxia in preclinical and clinical studies (20,21). Klein et al. found that the hypoxic NSCLC tumor cells were also sensitive to CIRT compared with cells under normoxic conditions (22). In 2006, a clinical study reported that a needle-type polarographic oxygen electrode was used to measure oxygen partial pressure, and the results showed that the local control rate of CIRT on hypoxic cervical cancer was similar to that of oxygenated cervical tumors (21).
Surprisingly, high-hypoxic tumors responded significantly better to CIRT than low-hypoxic tumors after CIRT. Our results provide strong clinical evidence of the unique effectiveness of carbon ion in hypoxic NSCLC tumors. This result also confirms the finding of our previous in vivo study that 18F-FMISO PET/CT imaging-guided irradiation on the hypoxic area of the tumor could induce a similar effect compared with the whole tumor carbon ion radiation (23). Since over 84.6% of NSCLC tumors were hypoxic (24), this result also partly explained why carbon ion-treated NSCLC patients showed better response. In SPHIC, we have treated 478 patients with lung cancer from May 2015 to June 2021. From August 2014 to March 2018, 31 non-selected NSCLC patients (T1–2a, N0–1, M0) treated with particle (proton or/and carbon) therapy were retrospectively analyzed. The 2-year progression-free survival (PFS) rate, local control rate, cause-specific survival rate, and overall survival rate were 85.5%, 95.2%, 95.0%, and 90.7%, respectively (25).
To date, 18F-FMISO is the most extensively investigated hypoxia PET tracer clinically. The observed high reproducibility of 18F-FMISO intratumor distribution in NSCLC patients facilitates its use in guiding hypoxic tumors’ delineation, individualized hypoxia-targeted therapies, and monitoring treatment response (26). Fortunately, we found that ΔTMR could predict early treatment response after CIRT. Similarly, the changes of 18F-FMISO uptake were reported to evaluate the ER to chemoradiotherapy in NSCLC (27). Likewise, fractional hypoxic volume was reported to be a valuable factor for predicting short-term response and PFS of chemoradiotherapy in NSCLC (12). It can also be seen in Figure 4 that the tumor with marked improvement of hypoxia during treatment will show sustained remission over time, even if the IR is not satisfied at first. It has been suggested that this follow-up effect of radiotherapy may originate from the fundamental reduction of the tumor burden and hypoxia by CIRT, thereby causing a sustained shrinkage of the tumor.
ΔTMR may be an appropriate and representative hypoxic parameter to evaluate the CIRT response in the 18F-FMISO PET/CT imaging; it is worth increasing the sample size for further prospective clinical studies. More details will be investigated in our center. Although the results are encouraging, the present study has several limitations. The main limitations are the limited sample size, single-center design, and lack of long-term follow-up and overall survival data. Although the representative hypoxia parameters displayed by 18F-FMISO PET/CT imaging could serve to predict CIRT response in NSCLC patients, larger and multi-center studies are still needed to verify the results and to optimize the hypoxia thresholds. In addition, the effects of different irradiation dose designs, inclusion of metastatic lymph nodes, and combination with other adjuvant therapies need to be explored in future studies, as these factors may have an impact on the hypoxia status and effectiveness of multidisciplinary treatment. Last but not least, each PET voxel size (approximately 4×4×4 mm3) is much larger than the hypoxic tumor microenvironment region, which has dimensions of ≤100 µm. Therefore, the intensity of 18F-FMISO within PET image voxels may reflect the combined uptake of normoxic and hypoxic regions, which may result in temporal instability in PET/CT imaging. It is obvious that choosing any single threshold value to determine hypoxic regions has limitations. Besides, all the uncertainties including statistical noise, differences in imaging start time, and variation in anatomic location between scans may contribute to the limitation of 18F-FMISO.
Conclusions
CIRT could relieve tumor hypoxia. TMR 2.35 is a valuable cut-off value; the higher the value, the better the response to CIRT, which is different from photon radiation. ΔTMR could predict the ER of CIRT with a cut-off value of 36.6%. Functional PET/CT imaging based on 18F-FMISO presents its potential in precise and individual CIRT, which could further improve tumor control.
Acknowledgments
A part of the manuscript has been presented in 2023 Academic annual meeting of Chinese Society of Nuclear Medicine and the 61st Annual Conference of the Particle Therapy.
Funding: This work was supported by
Footnote
Reporting Checklist: The authors have completed the STROBE reporting checklist. Available at https://qims.amegroups.com/article/view/10.21037/qims-23-1685/rc
Conflicts of Interest: All authors have completed the ICMJE uniform disclosure form (available at https://qims.amegroups.com/article/view/10.21037/qims-23-1685/coif). All authors report that this work was supported by Pudong New Area Science and Technology Development Foundation (grant Nos. PKJ 2018-Y49, PKJ 2020-Y56, PKJ 2023-Y44); Natural Science Foundation of Shanghai (grant Nos. 21ZR1460300 and 20ZR1453300); Shanghai Municipal Health Commission (grant Nos. 202040279 and 201940334); Shanghai Sailing Program (grant No. 21YF1444300). The authors have no other conflicts of interest to declare.
Ethical Statement: The authors are accountable for all aspects of the work in ensuring that questions related to the accuracy or integrity of any part of the work are appropriately investigated and resolved. The study was conducted in accordance with the Declaration of Helsinki (as revised in 2013). The study was approved by the institutional review board of SPHIC (ethical code: 1707-16-03-1804A) and the requirement for individual consent for this retrospective analysis was waived.
Open Access Statement: This is an Open Access article distributed in accordance with the Creative Commons Attribution-NonCommercial-NoDerivs 4.0 International License (CC BY-NC-ND 4.0), which permits the non-commercial replication and distribution of the article with the strict proviso that no changes or edits are made and the original work is properly cited (including links to both the formal publication through the relevant DOI and the license). See: https://creativecommons.org/licenses/by-nc-nd/4.0/.
References
- Duma N, Santana-Davila R, Molina JR. Non-Small Cell Lung Cancer: Epidemiology, Screening, Diagnosis, and Treatment. Mayo Clin Proc 2019;94:1623-40. [Crossref] [PubMed]
- Salem A, Little RA, Latif A, Featherstone AK, Babur M, Peset I, Cheung S, Watson Y, Tessyman V, Mistry H, Ashton G, Behan C, Matthews JC, Asselin MC, Bristow RG, Jackson A, Parker GJM, Faivre-Finn C, Williams KJ, O'Connor JPB. Oxygen-enhanced MRI Is Feasible, Repeatable, and Detects Radiotherapy-induced Change in Hypoxia in Xenograft Models and in Patients with Non-small Cell Lung Cancer. Clin Cancer Res 2019;25:3818-29. [Crossref] [PubMed]
- Salem A, Asselin MC, Reymen B, Jackson A, Lambin P, West CML, O'Connor JPB, Faivre-Finn C. Targeting Hypoxia to Improve Non-Small Cell Lung Cancer Outcome. J Natl Cancer Inst 2018; [Crossref]
- Graham K, Unger E. Overcoming tumor hypoxia as a barrier to radiotherapy, chemotherapy and immunotherapy in cancer treatment. Int J Nanomedicine 2018;13:6049-58. [Crossref] [PubMed]
- Wozny AS, Alphonse G, Cassard A, Malésys C, Louati S, Beuve M, Lalle P, Ardail D, Nakajima T, Rodriguez-Lafrasse C. Impact of hypoxia on the double-strand break repair after photon and carbon ion irradiation of radioresistant HNSCC cells. Sci Rep 2020;10:21357. [Crossref] [PubMed]
- Rockwell S, Dobrucki IT, Kim EY, Marrison ST, Vu VT. Hypoxia and radiation therapy: past history, ongoing research, and future promise. Curr Mol Med 2009;9:442-58. [Crossref] [PubMed]
- Valable S, Gérault AN, Lambert G, Leblond MM, Anfray C, Toutain J, Bordji K, Petit E, Bernaudin M, Pérès EA. Impact of Hypoxia on Carbon Ion Therapy in Glioblastoma Cells: Modulation by LET and Hypoxia-Dependent Genes. Cancers (Basel) 2020;12:2019. [Crossref] [PubMed]
- Miyasaka Y, Komatsu S, Abe T, Kubo N, Okano N, Shibuya K, Shirai K, Kawamura H, Saitoh JI, Ebara T, Ohno T. Comparison of Oncologic Outcomes between Carbon Ion Radiotherapy and Stereotactic Body Radiotherapy for Early-Stage Non-Small Cell Lung Cancer. Cancers (Basel) 2021;13:176. [Crossref] [PubMed]
- Kamada T, Tsujii H, Blakely EA, Debus J, De Neve W, Durante M, Jäkel O, Mayer R, Orecchia R, Pötter R, Vatnitsky S, Chu WT. Carbon ion radiotherapy in Japan: an assessment of 20 years of clinical experience. Lancet Oncol 2015;16:e93-e100. [Crossref] [PubMed]
- Rajendran JG, Krohn KA. F-18 fluoromisonidazole for imaging tumor hypoxia: imaging the microenvironment for personalized cancer therapy. Semin Nucl Med 2015;45:151-62. [Crossref] [PubMed]
- Stieb S, Eleftheriou A, Warnock G, Guckenberger M, Riesterer O. Longitudinal PET imaging of tumor hypoxia during the course of radiotherapy. Eur J Nucl Med Mol Imaging 2018;45:2201-17. [Crossref] [PubMed]
- Li L, Wei Y, Huang Y, Yu Q, Liu W, Zhao S, Zheng J, Lu H, Yu J, Yuan S. To Explore a Representative Hypoxic Parameter to Predict the Treatment Response and Prognosis Obtained by [18F]FMISO-PET in Patients with Non-small Cell Lung Cancer. Mol Imaging Biol 2018;20:1061-7.
- Ma L, Men Y, Feng L, Kang J, Sun X, Yuan M, Jiang W, Hui Z. A current review of dose-escalated radiotherapy in locally advanced non-small cell lung cancer. Radiol Oncol 2019;53:6-14. [Crossref] [PubMed]
- Eisenhauer EA, Therasse P, Bogaerts J, Schwartz LH, Sargent D, Ford R, Dancey J, Arbuck S, Gwyther S, Mooney M, Rubinstein L, Shankar L, Dodd L, Kaplan R, Lacombe D, Verweij J. New response evaluation criteria in solid tumours: revised RECIST guideline (version 1.1). Eur J Cancer 2009;45:228-47. [Crossref] [PubMed]
- Liao S, Penney BC, Wroblewski K, Zhang H, Simon CA, Kampalath R, Shih MC, Shimada N, Chen S, Salgia R, Appelbaum DE, Suzuki K, Chen CT, Pu Y. Prognostic value of metabolic tumor burden on 18F-FDG PET in nonsurgical patients with non-small cell lung cancer. Eur J Nucl Med Mol Imaging 2012;39:27-38. [Crossref] [PubMed]
- Thureau S, Chaumet-Riffaud P, Modzelewski R, Fernandez P, Tessonnier L, Vervueren L, et al. Interobserver agreement of qualitative analysis and tumor delineation of 18F-fluoromisonidazole and 3'-deoxy-3'-18F-fluorothymidine PET images in lung cancer. J Nucl Med 2013;54:1543-50. [Crossref] [PubMed]
- Cheng J, Lei L, Xu J, Sun Y, Zhang Y, Wang X, Pan L, Shao Z, Zhang Y, Liu G. 18F-fluoromisonidazole PET/CT: a potential tool for predicting primary endocrine therapy resistance in breast cancer. J Nucl Med 2013;54:333-40. [Crossref] [PubMed]
- Vera P, Mihailescu SD, Lequesne J, Modzelewski R, Bohn P, Hapdey S, Pépin LF, Dubray B, Chaumet-Riffaud P, Decazes P, Thureau S. all investigators of RTEP5 study (list in annexe). Radiotherapy boost in patients with hypoxic lesions identified by 18F-FMISO PET/CT in non-small-cell lung carcinoma: can we expect a better survival outcome without toxicity? Eur J Nucl Med Mol Imaging 2019;46:1448-56. [RTEP5 long-term follow-up].
- Mohamad O, Sishc BJ, Saha J, Pompos A, Rahimi A, Story MD, Davis AJ, Kim DWN. Carbon Ion Radiotherapy: A Review of Clinical Experiences and Preclinical Research, with an Emphasis on DNA Damage/Repair. Cancers (Basel) 2017;9:66. [Crossref] [PubMed]
- Masunaga S, Hirayama R, Uzawa A, Kashino G, Suzuki M, Kinashi Y, Liu Y, Koike S, Ando K, Ono K. The effect of post-irradiation tumor oxygenation status on recovery from radiation-induced damage in vivo: with reference to that in quiescent cell populations. J Cancer Res Clin Oncol 2009;135:1109-16. [Crossref] [PubMed]
- Nakano T, Suzuki Y, Ohno T, Kato S, Suzuki M, Morita S, Sato S, Oka K, Tsujii H. Carbon beam therapy overcomes the radiation resistance of uterine cervical cancer originating from hypoxia. Clin Cancer Res 2006;12:2185-90. [Crossref] [PubMed]
- Klein C, Dokic I, Mairani A, Mein S, Brons S, Häring P, Haberer T, Jäkel O, Zimmermann A, Zenke F, Blaukat A, Debus J, Abdollahi A. Overcoming hypoxia-induced tumor radioresistance in non-small cell lung cancer by targeting DNA-dependent protein kinase in combination with carbon ion irradiation. Radiat Oncol 2017;12:208. [Crossref] [PubMed]
- Huang Q, Sun Y, Wang W, Lin LC, Huang Y, Yang J, Wu X, Kong L, Lu JJ. Biological Guided Carbon-Ion Microporous Radiation to Tumor Hypoxia Area Triggers Robust Abscopal Effects as Open Field Radiation. Front Oncol 2020;10:597702. [Crossref] [PubMed]
- Li L, Hu M, Zhu H, Zhao W, Yang G, Yu J. Comparison of 18F-Fluoroerythronitroimidazole and 18F-fluorodeoxyglucose positron emission tomography and prognostic value in locally advanced non-small-cell lung cancer. Clin Lung Cancer 2010;11:335-40. [Crossref] [PubMed]
- Chen J, Lu JJ, Ma N, Zhao J, Chen C, Fan M, Jiang G, Mao J. Early stage non-small cell lung cancer treated with pencil beam scanning particle therapy: retrospective analysis of early results on safety and efficacy. Radiat Oncol 2019;14:16. [Crossref] [PubMed]
- Grkovski M, Schwartz J, Rimner A, Schöder H, Carlin SD, Zanzonico PB, Humm JL, Nehmeh SA. Reproducibility of (18)F-fluoromisonidazole intratumour distribution in non-small cell lung cancer. EJNMMI Res 2016;6:79. [Crossref] [PubMed]
- Gagel B, Reinartz P, Demirel C, Kaiser HJ, Zimny M, Piroth M, Pinkawa M, Stanzel S, Asadpour B, Hamacher K, Coenen HH, Buell U, Eble MJ. [18F] fluoromisonidazole and [18F] fluorodeoxyglucose positron emission tomography in response evaluation after chemo-/radiotherapy of non-small-cell lung cancer: a feasibility study. BMC Cancer 2006;6:51.