Left atrial and left ventricular strain in feature-tracking cardiac magnetic resonance for predicting patients at high risk of sudden cardiac death in hypertrophic cardiomyopathy
Introduction
Hypertrophic cardiomyopathy (HCM) is an inherited heart disease caused by mutations in the gene encoding myocardial sarcomeric proteins. The main characteristic of this condition is left ventricular (LV) septum and/or LV free wall hypertrophy (1). Due to the accumulation of disturbed myocardial fiber alignment, cardiomyocyte malformations, and myocardial fibrosis, approximately two-thirds of patients with HCM develop ventricular narrowing or LV outflow tract obstruction (2). Ventricular tachyarrhythmias, emanating from regions of disorganized architecture and myocardial fibrosis, are a possible mechanism of sudden cardiac death (SCD) in HCM (3,4). Implantable cardioverter-defibrillator (ICD) implantation is recommended for primary prevention in patients at high risk for SCD, as it has been shown to effectively reduce SCD-related mortality. ICDs are often implanted in young individuals who may need multiple generator changes in their lifetimes. Therefore, the decision to implant an ICD must be carefully considered in light of the potential complications, including infections, thromboembolism, and inappropriate discharges triggered by supraventricular tachycardias (5).
There is ongoing debate regarding the risk stratification of SCD in patients with HCM. In 2014, the European Society of Cardiology (ESC) proposed guidelines to assess the 5-year risk of SCD, and in 2020, the American College of Cardiology/American Heart Association (ACC/AHA) proposed the use of seven biological markers to guide ICD (2,6). Previous studies have identified clinical markers associated with SCD risk stratification in patients with HCM, including family history of SCD, nonsustained ventricular tachycardia (NSVT), left atrium diameter, syncope, LV wall thickness, and LV outflow tract obstruction. Despite this, the limited positive predictive value of these clinical markers and differences in the assessment of SCD risk between the ESC and AHA/ACC guidelines highlight the need for additional indicators to more accurately assess SCD risk and guide ICD implantation while minimizing complications (7,8).
Feature-tracking cardiac magnetic resonance (FT-CMR) demonstrates good consistency and repeatability in assessing myocardial strain, a sensitive and noninvasive measure for evaluating global and segmental left heart function and reflecting changes in myocardial metabolism and contractility (9). Myocardial strain denotes the shortening, thickening, and elongation of the myocardial segment during the cardiac cycle and is a robust and reproducible measure of LV systolic performance. Strain rate (SR) refers to the rate of myocardial deformation per unit time (10). The diastolic SR, less influenced by passive myocardial motion, shows a consistent distribution throughout the left ventricle and accurately reflects diastolic dysfunction of the LV wall (11). Left atrial (LA) remodeling plays a role in determining LA dysfunction, with LA strain being closely associated with LA function. LA function includes the reservoir function during ventricular systole, the conduit function during LV diastole, and the boost function dependent on LA active contraction during the ventricular end-diastolic phase (12). Accurate assessment of LA and LV myocardial deformation using FT-CMR can provide crucial information on left heart function and prognostication in clinical practice. However, the relationship between myocardial strain and the risk factors of SCD in patients with HCM remains unclear.
This study aimed to assess the diagnostic value of myocardial strain in identifying a high risk for SCD among patients with HCM classified according to the 2020 ACC/AHA ICD class of recommendation (ICD-COR) algorithm. Additionally, we investigated the correlation between myocardial strain and the risk factors for assessing SCD risk as outlined in the 2020 ACC/AHA guidelines. The results of this study will contribute to a more comprehensive identification and differentiation of patients at high risk for SCD in clinical practice and contribute to informing the use of ICDs. We present this article in accordance with the STROBE reporting checklist (available at https://qims.amegroups.com/article/view/10.21037/qims-23-1615/rc).
Methods
Study population
Patients with HCM diagnosed via cardiac magnetic resonance (CMR) between February 2021 and July 2023 at the First Hospital of Harbin Medical University were retrospectively included in this study. All patients met the diagnostic criteria for HCM according to the 2020 ACC/AHA, which included a LV wall myocardial thickness of ≥15 mm at end-diastole on CMR or echocardiography, or ≥13 mm in patients with a family history of HCM. The exclusion criteria were the following: (I) age <16 years, (II) CMR images with severe cardiac motion and respiratory artifacts, (III) patients with secondary cardiac hypertrophy due to other causes (e.g., myocardial infarction, cardiac valvular disease, hypertensive cardiomyopathy, and cardiac amyloidosis), and (IV) metabolic diseases such as Fabry disease and congenital syndromes such as Noonan syndrome (Figure 1). This study adhered to the Declaration of Helsinki (as revised in 2013) and was approved by the Human Research and Ethics Committee of the First Affiliated Hospital of Harbin Medical University (No. 2023JS40). Individual consent for this retrospective analysis was waived.
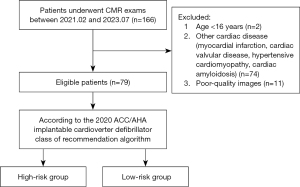
Risk assessment of included patients
The grouping of participants strictly adhered to the ICD-COR algorithm as published in the 2020 ACC/AHA guideline and is applicable to patients aged ≥16 years. Patients classified as class II in the ICD-COR algorithm are at higher risk for SCD and may require of ICD implantation. Class IIa patients are defined as those with at least one of the following: (I) a family history of SCD, (II) maximum LV wall thickness ≥30 mm, (III) unexplained syncope, (IV) LV apical aneurysm, and (V) left ventricular ejection fraction (LVEF) <50%. Class IIb patients are defined as those with (I) late gadolinium enhancement (LGE) ≥15% of the LV mass, and (II) NSVT. Class III patients, defined as patients without any of the above risk factors, have a lower risk of SCD and are not recommended for ICD use. The patients in class II were categorized as the high-risk group, and those in class III were categorized as the low-risk group.
MR protocol
All patients underwent examination using a 3.0 T MR scanner (Elition, Philips Healthcare, Andover, the Netherlands) with a 32-channel abdominal coil. The imaging protocol included chest-lead electrocardiographic gating, respiratory gating, and breath-holding at the end of expiration to acquire images. Two-, three-, and four-chamber views, along with short-axis views, were obtained using the steady-state free precession (SSFP) sequence. The short-axis series was scanned continuously from basal to apical segments under the following scanning parameters: repetition time (TR) =43 ms, echo time (TE) =1 ms, field of view (FOV) =320 mm × 320 mm, and slice thickness =8 mm. LGE imaging was obtained at 10–15 minutes postcontrast using an inversion recovery-prepared T1-weighted gradient echo sequence with an inversion time via the Look-Locker sequence. The scanning parameters for delayed enhancement images were as follows: TR =6.1 ms, TE =3 ms, FOV =320 mm × 320 mm, and slice thickness =8 mm.
MR image analysis
All images were imported into CVI42 software (Circle Cardiovascular Imaging Inc., Calgary, AB, Canada) for analysis. Postprocessing analysis was independently conducted by two cardiac imaging physicians, with one physician repeating the measurement 2 weeks later. The endocardial and epicardial borders were automatically outlined with manual adjustment (Figure 2). LV function parameters include LVEF, LV end-diastolic volume index (LVEDVI), LV end-systolic volume index (LVESVI), and stroke volume index (SVI). LV maximal wall thickness (LVMWT) was obtained from the end-diastolic short-axis cine sequence. LA functional parameters, comprising LA minimal volume index (LAVImin), LA maximal volume index (LAVImax), LA ejection fraction (LAEF), and LA stroke volume index (LASVI), were measured using the multifunctional long-axis module. The LA diameter was measured at the end-systolic phase of the left ventricle in the three-chamber view. The extent of LGE was measured using the tissue characterization module, with a threshold of two standard deviations above the normal area. The strain analysis of the LV was performed in the strain module to obtain LV global longitudinal strain (LVGLS), LV global circumferential strain (LVGCS), and LV global radial strain (LVGRS), which represent the contraction function of the left ventricle. Additionally, peak diastolic-longitudinal strain rate (PD-LSR), peak diastolic-circumferential strain rate (PD-CSR), and peak diastolic-radial strain rate (PD-RSR) were determined to assess the diastolic function of the LV. The LA reservoir strain (LARS), LA conduit strain (LACS), and left atrial booster strain (LABS) were measured by manually outlining the LA endocardium and epicardium at LV end-systole in both two-chamber and four-chamber views. The atrial strain curve was generated by feature tracking (Figure 3).
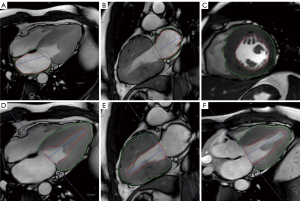
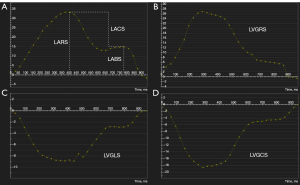
Reproducibility
Intraobserver variability was assessed by comparing selected measurements conducted by the same observer after a 2-week interval. Interobserver variability was measured independently between the two observers.
Statistical analysis
The normality of data was assessed using the Kolmogorov-Smirnov test. Normally distributed data are expressed as the mean ± standard deviation, while data that did not follow a normal distribution are expressed as the median and interquartile range (IQR). Continuous variables between groups were compared using the independent samples t-test and Mann-Whitney test. Spearman correlation analysis was employed to evaluate the correlation between different risk factors and strain parameters. Logistic regression analysis was performed to further explore the factors indicating a high risk of SCD in patients with HCM. Multivariate analysis incorporated variables with a P value <0.05 from the univariate analysis. The receiver operating characteristic (ROC) curve was used to determine the area under the curve (AUC), and the difference in diagnostic value between models was assessed using the DeLong test. Intra- and interobserver reproducibility were evaluated using the intragroup correlation coefficient (ICC). An ICC value of 0.75–0.90 was considered to indicate good reproducibility. P<0.05 was considered statistically significant. All statistical analyses were performed using SPSS software version 25.0 (IBM Corp., Armonk, NY, USA).
Results
Participant characteristics
This study initially included 166 patients with LV hypertrophy, and after unsuitable patients were excluded, 79 patients with HCM were ultimately enrolled in this study, 61% of whom were male. These patients were categorized into two groups, including 34 patients in the high-risk group (mean age 53.38±13.48 years) and 45 patients in the low-risk group (mean age 57±12.49 years). Among the participants, 15 exhibited NSVT, 10 had a history of unexplained syncope, 4 had a family history of HCM, 1 had an LV apical aneurysm, 13 had atrial fibrillation (AF), and 24 had LV outflow tract obstruction. There were no statistically significant differences in age, gender, weight, height, body mass index (BMI), or body surface area (BSA) between patients in the high-risk and low-risk groups (all P values >0.05).
Compared with those in the low-risk group, patients in the high-risk group had lower LVEF (high-risk: median 52.15%, IQR 32.72–59.99%; low-risk: median 61.88, IQR 55.91–67.24; P<0.001), LVSVI (high-risk: mean 31.54±11.33 mL/m2; low-risk: mean 42.10±12.33 mL/m2; P<0.001), and LASVI (high-risk: mean 17.48±6.41 mL/m2; low-risk: 23.94±8.75 mL/m2; P<0.001) but higher LVESVI [high-risk: 33.53 (25.9, 53.16) mL/m2; low-risk: median 27.54 mL/m2, IQR 22.05–30.79 mL/m2; P<0.001], LVMWT (high-risk: median 21 mm, IQR 18–25 mm; low-risk: median 17.25 mm, IQR 16–9.75 mm; P<0.001), LGE (high-risk: median 10.91%, IQR 6.05–18.04%; low-risk: median 3.78%, IQR 2.56–5.13%; P<0.001). and LA diameter (high-risk: median 37.94 mm, IQR 34.33–44.33 mm; low-risk: median 35.35 mm, IQR 32.03–38.27 mm; P=0.02). There were no significant differences in LVEDVI, LVMI, LAVImin, LAVImax, LAEF, cardiac troponin I (cTnI), creatine kinase-MB (CKMB), or N-terminal prohormone of brain natriuretic peptide (NT-pro BNP) between the two groups (all P values >0.05) (Table 1).
Table 1
Variable | High-risk group (n=34) | Low-risk group (n=45) | Test statistic | P value |
---|---|---|---|---|
Age (years) | 53.38±13.48 | 57±12.49 | −1.23 | 0.22 |
Male (n) | 23 | 25 | 0.29 | 0.77 |
Weight (kg) | 75 (62.63, 81.25) | 68.25 (60, 73) | −1.78 | 0.08 |
Height (mm) | 168.03±7.77 | 166.12±8.39 | 1.07 | 0.29 |
BMI (kg/m2) | 25.65 (22.85, 28.47) | 24.22 (23.19, 26.66) | −1.25 | 0.21 |
BSA (m2) | 1.81 (1.66, 1.94) | 1.73 (1.59, 1.82) | −1.84 | 0.07 |
LVEF (%) | 52.15 (32.72, 59.99) | 61.88 (55.91, 67.24) | −4.42 | <0.001** |
LVEDVI (mL/m2) | 67.69 (59.63, 82.04) | 70.24 (59.71, 82.42) | −0.1 | 0.92 |
LVESVI (mL/m2) | 33.53 (25.9, 53.16) | 27.54 (22.05, 30.79) | −3.36 | <0.001** |
LVSVI (mL/m2) | 31.54±11.33 | 42.10±12.33 | −4.05 | <0.001** |
LVMI (g/m2) | 73.69±23.58 | 75.58±25.33 | −0.35 | 0.73 |
LVMWT (mm) | 21 (18, 25) | 17.25 (16, 19.75) | −3.29 | <0.001** |
Obstructive (n) | 8 | 15 | 0.91 | 0.37 |
LGE (% of LVM) | 10.91 (6.05, 18.04) | 3.78 (2.56, 5.13) | −5.68 | <0.001** |
LA diameter (mm) | 37.94 (34.33, 44.33) | 35.35 (32.03, 38.27) | −2.09 | 0.02* |
LAVImin (mL/m2) | 26.5 (19.33, 40.35) | 29.88 (19.07, 39.96) | −0.56 | 0.57 |
LAVImax (mL/m2) | 43.84 (35.04, 57.82) | 54.98 (40.87, 70.34) | −1.87 | 0.06 |
LAEF (%) | 41.71±12.30 | 46.89±11.86 | −1.89 | 0.06 |
LASVI (mL/m2) | 17.48±6.41 | 23.94±8.75 | −3.63 | <0.001** |
AF (n) | 7 | 6 | 0.25 | 0.81 |
cTnI (ng/L) | 20.8 (11.06, 39.2) | 17.08 (10.33, 171.97) | −0.72 | 0.47 |
CKMB (U/L) | 1.15 (0.81, 1.375) | 1.7 (1.09, 2.32) | −1.33 | 0.18 |
NT-pro BNP (pg/mL) | 731.9 (301.15, 819.9) | 723.85 (267.875, 1,936.25) | −0.83 | 0.41 |
LVGCS (%) | −15.5 (−17.63, −10.23) | −18.95 (−21.15, −17.6) | −5.59 | <0.001** |
LVGRS (%) | 16.35±7.07 | 32.98±5.37 | −12.12 | <0.001** |
LVGLS (%) | −8.1 (−11.55, −5.9) | −10.75 (−13.45, −9.8) | 3.75 | <0.001** |
PD-CSR (s–1) | 0.6 (0.4, 0.7) | 0.95 (0.7, 1.18) | −6.13 | <0.001** |
PD-RSR (s–1) | −0.95 (−1.5, −0.6) | −1.9 (−2.3, −1.43) | −5.79 | <0.001** |
PD-LSR (s–1) | 0.4 (0.3, 0.5) | 0.6 (0.5, 0.8) | −5.46 | <0.001** |
LARS (%) | 17.51±6.83 | 21.08±7.68 | −2.14 | 0.04* |
LACS (%) | 9.45 (4.95, 11.6) | 11.65 (8.73, 13.05) | −2.16 | 0.02* |
LABS (%) | 7.04±3.78 | 10.32±4.06 | −3.65 | <0.001** |
Continuous variables are presented as the mean ± standard deviation or as the median (interquartile range). *, P<0.05; **, P<0.001. CMR, cardiac magnetic resonance; HCM, hypertrophic cardiomyopathy; BMI, body mass index; BSA, body surface area; LVEF, left ventricular ejection fraction; LVEDVI, left ventricular end-diastolic volume index; LVESVI, left ventricular end-systolic volume index; LVSVI, left ventricular stroke volume index; LVMI, left ventricular mass index; LVMWT, left ventricular maximum wall thickness; LGE, late gadolinium enhancement; LVM, left ventricular mass; LA, left atrial; LAVImin, left atrial volume minimum; LAVImax, left atrial volume maximum; LAEF, left atrial ejection fraction; LASVI, left atrial stroke volume index; AF, atrial fibrillation; cTnI, cardiac troponin I; CKMB, creatine kinase-MB; NT-pro BNP, N-terminal prohormone of brain natriuretic peptide; LVGCS, left ventricular global circumferential strain; LVGRS, left ventricular global radial strain; LVGLS, left ventricular global longitudinal strain; PD-CSR, peak diastolic-circumferential strain rate; PD-RSR, peak diastolic-radial strain rate; PD-LSR, peak diastolic-longitudinal strain rate; LARS, left atrial reservoir strain; LACS, left atrial conduit strain; LABS, left atrial booster strain.
The high-risk group had worse myocardial strain compared to the low-risk group. Compared with the patients in the low-risk group, those in the high-risk group were associated with higher LVGCS (high-risk: median –15.5%, IQR –17.63 to –10.23%; low-risk: median –18.95%, IQR –21.15 to –17.6%; P<0.001), LVGLS (high-risk: median –8.1%, IQR –11.55 to –5.9%; low-risk: median –10.75%, IQR –13.45 to –9.8%; P<0.001), and PD-RSR (high-risk: median –0.95 s–1, IQR –1.5 to –0.6 s–1; low-risk: median –1.9 s–1, IQR –2.3 to –1.43 s–1; P<0.01) but lower LVGRS (high-risk: mean 16.35%±7.07%; low-risk: mean 32.98%±5.37%; P<0.001), PD-CSR (high-risk: median 0.6 s–1, IQR 0.4–0.7 s–1; low-risk: median 0.95 s–1, IQR 0.7–1.18 s–1; P<0.001), and PD-LSR (high-risk: median 0.4 s–1, IQR 0.3–0.5 s–1; low-risk: median 0.6 s–1, IQR 0.5–0.8 s–1; P<0.001). For LA strain, compared to those in the low-risk group, the patients in the high-risk group exhibited lower LARS (high-risk: mean 17.51%±6.83%; low-risk: mean 21.08%±7.68%; P=0.04), LACS (high-risk: median 9.45%, IQR 4.95–11.6%; low-risk: median 11.65%, IQR 8.73–13.05%; P=0.02), and LABS (high-risk: mean 7.04%±3.78%; low-risk: mean 10.32%±4.06%; P<0.001) (Table 1, Figure 4).
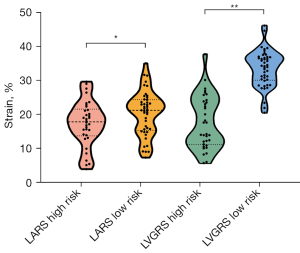
Bivariate analysis regarding the association of myocardial strain with risk factors for SCD
The Spearman correlation test showed that in patients with HCM, LVEF had a strong negative correlation with LVGCS (r=−0.76; P<0.001) (Figure 5); a moderate positive correlation with LVGRS, PD-CSR, and PD-LSR (r=0.63, r=0.49, r=0.48; P<0.001); and a moderate negative correlation with PD-RSR (r=−0.49; P<0.001). Similarly, significant correlations, albeit weak, were found between LGE and left ventricular myocardial strain (LVGCS: r=0.38, P<0.001; LVGRS: r=−0.49, P<0.001; LVGLS: r=0.29, P=0.02; PD-CSR: r=−0.44, P<0.001; PD-RSR: r=0.46, P<0.001; PD-LSR: r=−0.34, P<0.001) (Table 2). Among all indicators of LA strains, LARS showed the most significant correlation with LA diameter (r=−0.48), AF (r=−0.59), and LAEF (r=0.60) (all P values <0.001; Table 3).
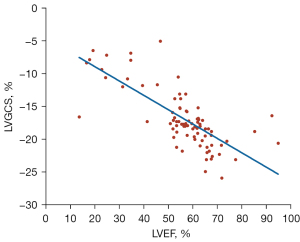
Table 2
Strain | LVEF (%) | LGE (% of LVM) | LVMWT (mm) | |||||
---|---|---|---|---|---|---|---|---|
r value | P value | r value | P value | r value | P value | |||
LVGCS (%) | −0.76 | <0.001** | 0.38 | <0.001** | 0.33 | <0.001** | ||
LVGRS (%) | 0.63 | <0.001** | −0.49 | <0.001** | −0.36 | <0.001** | ||
LVGLS (%) | −0.17 | 0.10 | 0.29 | 0.02* | 0.30 | 0.01* | ||
PD-CSR (s–1) | 0.49 | <0.001** | −0.44 | <0.001** | −0.24 | 0.03* | ||
PD-RSR (s–1) | −0.58 | <0.001** | 0.46 | <0.001** | 0.19 | 0.08 | ||
PD-LSR (s–1) | 0.48 | <0.001** | −0.34 | <0.001** | −0.28 | 0.01* |
*, P<0.05; **, P<0.001. SCD, sudden cardiac death; LVEF, left ventricular ejection fraction; LGE, late gadolinium enhancement; LVM, left ventricular mass; LVMWT, left ventricular maximum wall thickness; LVGCS, left ventricular global circumferential strain; LVGRS, left ventricular global radial strain; LVGLS, left ventricular global longitudinal strain; PD-CSR, peak diastolic-circumferential strain rate; PD-RSR, peak diastolic-radial strain rate; PD-LSR, peak diastolic-longitudinal strain rate.
Table 3
Strain | LA diameter (mm) | AF (n) | LAEF (%) | |||||
---|---|---|---|---|---|---|---|---|
r value | P value | r value | P value | r value | P value | |||
LARS (%) | −0.48 | <0.001** | −0.59 | <0.001** | 0.60 | <0.001** | ||
LACS (%) | −0.32 | <0.001** | −0.58 | <0.001** | 0.47 | <0.001** | ||
LABS (%) | −0.37 | <0.001** | −0.57 | <0.001** | 0.52 | <0.001** |
*, P<0.05; **, P<0.001. SCD, sudden cardiac death; LA, left atrial; AF, atrial fibrillation; LAEF, left atrial ejection fraction; LARS, left atrial reservoir strain; LACS, left atrial conduit strain; LABS, left atrial booster strain.
Analysis of factors influencing the high risk of SCD in HCM
The results of univariate and multivariable binary logistic regression analyses for all patients with HCM in predicting high-risk SCD are shown in Table 4. The multivariate analysis showed that LVGRS and LARS were independent risk factors for classifying patients with HCM into the high-risk SCD group [LVGRS: odds ratio (OR) = 0.69, 95% confidence interval (CI): 0.55–0.87, P<0.001; LARS: OR =1.39, 95% CI: 1.02–1.90, P=0.03]. Patients with HCM and LVGRS <27.15% were more likely to exhibit a high risk of SCD (AUC =0.92; 95% CI: 0.86–0.99; P<0.001). The AUC and cutoff value of strain/SR for predicting high-risk SCD is shown in Table 5. The combined LVGRS-LARS model, established according to the multivariate regression results, was assessed for its diagnostic value in identifying patients at high risk of SCD. The result showed that the diagnostic value of LVGRS was superior to that of LARS (AUC =0.63; 95% CI: 0.51–0.76; P<0.001), while the diagnostic value of the combined LVGRS-LARS model for high risk of SCD was superior to that of LARS (AUC =0.95; 95% CI: 0.90–1.00; P<0.001), although there was no statistical difference compared to the diagnostic performance of LVGRS alone (P=0.47) (Figure 6).
Table 4
Variables | Univariate regression | Multiple regression | |||
---|---|---|---|---|---|
OR (95% CI) | P value | OR (95% CI) | P value | ||
LVEF (%) | 0.90 (0.85–0.95) | <0.001** | – | – | |
LVEDVI (mL/m2) | 1.01 (0.99–1.03) | 0.31 | – | – | |
LVESVI (mL/m2) | 1.08 (1.03–1.12) | <0.001** | – | – | |
LVSVI (mL/m2) | 0.92 (0.88–0.96) | <0.001** | 0.94 (0.83–1.06) | 0.28 | |
LVMI (g/m2) | 0.99 (0.97–1.01) | 0.30 | – | – | |
LVMWT (mm) | 1.21 (1.08–1.36) | 0.01 | – | – | |
LGE (% of LVM) | 1.41 (1.21–1.65) | <0.001** | – | – | |
LVGCS (%) | 1.57 (1.28–1.92) | <0.001** | 0.86 (0.55–1.35) | 0.52 | |
LVGRS (%) | 0.65 (0.53–0.80) | <0.001** | 0.69 (0.55–0.87) | <0.001** | |
LVGLS (%) | 1.12 (1.00–1.26) | <0.001** | 1.15 (0.96–1.37) | 0.14 | |
LA diameter (mm) | 1.10 (1.01–1.19) | 0.03* | 1.18 (0.94–1.47) | 0.15 | |
LAVImin (mL/m2) | 0.99 (0.96–1.02) | 0.61 | – | – | |
LAVImax (mL/m2) | 0.96 (0.95–1.00) | 0.06 | – | – | |
LAEF (%) | 0.97 (0.93–1.00) | 0.06 | – | – | |
LASVI (mL/m2) | 0.89 (0.83–0.96) | <0.001** | 0.97 (0.82–1.14) | 0.68 | |
LARS (%) | 0.93 (0.87–1.00) | 0.04* | 1.39 (1.02–1.90) | 0.03* | |
LACS (%) | 0.88 (0.79–0.99) | 0.03* | 0.93 (0.61–1.42) | 0.74 | |
LABS (%) | 0.80 (0.70–0.92) | <0.001** | 0.68 (0.45–1.04) | 0.07 |
*, P<0.05; **, P<0.001. HCM, hypertrophic cardiomyopathy; SCD, sudden cardiac death; OR, odds ratio; CI, confidence interval; LVEF, left ventricular ejection fraction; LVEDVI, left ventricular end-diastolic volume index; LVESVI, left ventricular end-systolic volume index; LVSVI, left ventricular stroke volume index; LVMI, left ventricular mass index; LVMWT, left ventricular maximum wall thickness; LGE, late gadolinium enhancement; LVM, left ventricular mass; LVGCS, left ventricular global circumferential strain; LVGRS, left ventricular global radial strain; LVGLS, left ventric-ular global longitudinal strain; LAVImin, left atrial volume minimum; LAVImax, left atrial volume maximum; LAEF, left atrial ejection fraction; LASVI, left atrial stroke volume index; LARS, left atrial reservoir strain; LACS, left atrial conduit strain; LABS, left atrial booster strain.
Table 5
Strain | AUC | Sensitivity | Specificity | Cutoff value (%) |
---|---|---|---|---|
LVGRS (%) | 0.92 | 0.86 | 0.92 | 27.15 |
LVGCS (%) | 0.85 | 0.69 | 0.84 | −16.95 |
LVGLS (%) | 0.71 | 0.58 | 0.89 | −8.35 |
PD-CSR (s–1) | 0.90 | 0.92 | 0.69 | 0.75 |
PD-RSR (s–1) | 0.89 | 0.92 | 0.67 | 1.65 |
PD-LSR (s–1) | 0.80 | 0.89 | 0.44 | 0.55 |
LARS (%) | 0.63 | 0.71 | 0.6 | 20.6 |
LACS (%) | 0.65 | 0.79 | 0.49 | 11.65 |
LABS (%) | 0.72 | 0.82 | 0.53 | 9.95 |
ROC, receiver operating characteristic; SCD, sudden cardiac death; AUC, area under the curve; LVGRS, left ventricular global radial strain; LVGCS, left ventricular global circumferential strain; LVGLS, left ventricular global longitudinal strain; PD-CSR, peak diastolic-circumferential strain rate; PD-RSR, peak diastolic-radial strain rate; PD-LSR, peak diastolic-longitudinal strain rate; LARS, left atrial reservoir strain; LACS, left atrial conduit strain; LABS, left atrial booster strain.
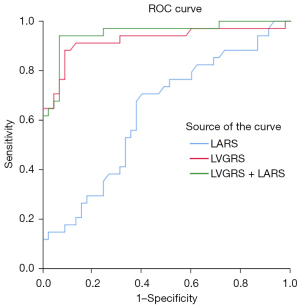
Reproducibility
We found that the intraobserver and interobserver variability of all strain values had good repeatability (all ICCs >0.8). The intraobserver and interobserver ICC values are shown in Table S1.
Discussion
This study focused on assessing the discriminative ability and diagnostic value of LV and LA strain from FT-CMR in individuals at high risk of SCD. The analysis indicated a significant discrepancy in myocardial strain between the high-risk and low-risk SCD groups. Furthermore, there were noteworthy correlations between LVGCS and LVEF and between LVGRS and the SCD risk factors of LVEF, LGE, and LVMWT. Additionally, LARS exhibited associations with AF, LAEF, and LA diameter. Notably, LVGRS and LARS were independently associated with a heightened SCD risk in patients with HCM, and the combined LVGRS-LARS model demonstrated excellent diagnostic value for high-risk SCD.
Myocardial strain analysis is an innovative approach for directly assessing cardiac function by examining myocardial deformation. The results of a recent study also suggest that damaged strain may potentially have predictive value in clinical risk assessment (13). Our study revealed that both LV and LA strain showed significant statistical differences between the high-risk and low-risk groups, which was consistent with the findings of Lee et al. and He et al. (14,15). In early-stage HCM, hyperejection can result in normal or elevated LVEF values, masking underlying myocardial deformation and cardiac dysfunction. Therefore, a threshold of LVEF 50% may not accurately identify risk of SCD. A strong negative correlation between LVGCS and LVEF was also found in our study. LVGCS, which directly measures myofiber deformation in the midmyocardium, is a highly sensitive technique that can detect changes in ventricular function, even in patients with LVEF > 50%, thus facilitating the early detection of cardiac impairment.
LA strain has been shown to be an important prognostic indicator in patients with HCM (16). Our study identified differences in LARS, conduit strain, and boost strain between the high- and low-risk groups for SCD, as well as correlations between LA strain and LA diameter, LAEF, and AF. Patients with HCM are more susceptible to LA remodeling for several reasons. Elevated LV filling pressures impose increased LA afterload, which causes mechanical stress on the LA and results in a reduction in the LA reserve function. On the other hand, chronic increases in LV filling pressures lead to LA dilatation with fibrosis, which may also contribute to the reduction in LA strain (17,18). Therefore, LA strain has gradually become a powerful indicator for assessing LV filling pressure and LV diastolic dysfunction (19).
In this study, LVGRS and LARS showed independent correlations with a high risk of SCD. Recent studies (3) have discovered that diffusely distributed myofibrillar disarray and structural disruptions in HCM serve as the basis for intraventricular conduction dispersion, culminating in refractory arrhythmias and SCD. Additionally, myocardial hypertrophy in HCM can result in increased thickness of the radial ventricular wall and diastolic dysfunction, escalating the risk of AF and SCD due to elevated LV filling pressures, LA afterload caused by mitral regurgitation, and LV outflow tract obstruction. LVGRS, which manifests as myocardial centripetal thickening, is linked with the pathogenesis of myocardial centripetal hypertrophy in HCM, allowing LVGRS to evaluate the risk of SCD in patients with HCM more holistically. Smith et al. observed reduced LVGRS in patients with HCM who experience adverse events (20), which is similar to our results. Moreover, our study also found that the correlation of LVGRS with LVEF, LGE, and LVMWT was also stronger than that of LVGLS and LVGCS. Xu et al. reported that radial strain and LVEF exhibited a positive correlation (21), suggesting a decline in LVEF with radial thickening of the myocardium. As early thickening of the myocardium can lead to a decrease in radial strain, changes in radial strain should be closely monitored.
LARS also showed an independent correlation with SCD. LARS represents the dilatation function of the LA, and chronic secondary changes caused by reduced LV diastolic function in patients with HCM result in decreased LARS. Myocardial thickening, cardiomyocyte disorganization, and increased interstitial fibrosis in patients with HCM lead to decreased passive diastolic elasticity, with early diastolic dysfunction occurring in the form of LA deformity and increased atrial pressure (22,23). Similarly, in a study by Yang et al. (24,25), LA reservoir and conduit dysfunction preceded LA enlargement in patients with nonobstructive HCM, and early changes in LARS were detected in patients with HCM and normal LA size. Lee et al. and Vasquez et al. also demonstrated that patients with HCM and impaired LARS face an increased risk of adverse clinical outcomes (18,26). In a recent meta-analysis, it was shown that LA strain plays a significant role in predicting the occurrence of AF and major adverse cardiovascular events in patients with HCM. These findings suggest that LA strain measurement could serve as a valuable supplement to the risk stratification model for patients with HCM (27).
Some limitations to this study should be mentioned. First, we employed a single-center design with a relatively small sample size and a limited number of adverse events. Further analysis of segmental strain is necessary to assess segmental myocardial deformation. In addition, the predictive value of myocardial strain could not be obtained due to the lack of long-term follow-up and the occurrence of adverse cardiac events.
Conclusions
Myocardial strain analysis can accurately identify patients at high risk of SCD as defined by the 2020 ACC/AHA guidelines. In clinical practice, myocardial strain may be a valuable diagnostic indicator for a high risk of SCD and help to inform the administration of ICDs.
Acknowledgments
Funding: This study was supported by
Footnote
Reporting Checklist: The authors have completed the STROBE reporting checklist. Available at https://qims.amegroups.com/article/view/10.21037/qims-23-1615/rc
Conflicts of Interest: All authors have completed the ICMJE uniform disclosure form (available at https://qims.amegroups.com/article/view/10.21037/qims-23-1615/coif). J.L. serves as a full-time employee of Philips Healthcare during the research period, primarily providing technical support and participating in the revision of the article. The other authors have no conflicts of interest to declare.
Ethical Statement: The authors are accountable for all aspects of the work in ensuring that questions related to the accuracy or integrity of any part of the work are appropriately investigated and resolved. This study was conducted in accordance with the Declaration of Helsinki (as revised in 2013) and approved by the Human Research and Ethics Committee of the First Affiliated Hospital of Harbin Medical University (No. 2023JS40). Individual consent for this retrospective analysis was waived.
Open Access Statement: This is an Open Access article distributed in accordance with the Creative Commons Attribution-NonCommercial-NoDerivs 4.0 International License (CC BY-NC-ND 4.0), which permits the non-commercial replication and distribution of the article with the strict proviso that no changes or edits are made and the original work is properly cited (including links to both the formal publication through the relevant DOI and the license). See: https://creativecommons.org/licenses/by-nc-nd/4.0/.
References
- Elliott P, Andersson B, Arbustini E, Bilinska Z, Cecchi F, Charron P, Dubourg O, Kühl U, Maisch B, McKenna WJ, Monserrat L, Pankuweit S, Rapezzi C, Seferovic P, Tavazzi L, Keren A. Classification of the cardiomyopathies: a position statement from the European Society Of Cardiology Working Group on Myocardial and Pericardial Diseases. Eur Heart J 2008;29:270-6. [Crossref] [PubMed]
- Ommen SR, Mital S, Burke MA, Day SM, Deswal A, Elliott P, Evanovich LL, Hung J, Joglar JA, Kantor P, Kimmelstiel C, Kittleson M, Link MS, Maron MS, Martinez MW, Miyake CY, Schaff HV, Semsarian C, Sorajja P. 2020 AHA/ACC Guideline for the Diagnosis and Treatment of Patients With Hypertrophic Cardiomyopathy: A Report of the American College of Cardiology/American Heart Association Joint Committee on Clinical Practice Guidelines. J Am Coll Cardiol 2020;76:e159-e240. [Crossref] [PubMed]
- Saumarez RC, Pytkowski M, Sterlinski M, Bourke JP, Clague JR, Cobbe SM, Connelly DT, Griffith MJ, McKeown PP, McLeod K, Morgan JM, Sadoul N, Chojnowska L, Huang CL, Grace AA. Paced ventricular electrogram fractionation predicts sudden cardiac death in hypertrophic cardiomyopathy. Eur Heart J 2008;29:1653-61. [Crossref] [PubMed]
- Finocchiaro G, Sheikh N, Leone O, Westaby J, Mazzarotto F, Pantazis A, Ferrantini C, Sacconi L, Papadakis M, Sharma S, Sheppard MN, Olivotto I. Arrhythmogenic potential of myocardial disarray in hypertrophic cardiomyopathy: genetic basis, functional consequences and relation to sudden cardiac death. Europace 2021;23:985-95. [Crossref] [PubMed]
- Maron BJ, Shen WK, Link MS, Epstein AE, Almquist AK, Daubert JP, Bardy GH, Favale S, Rea RF, Boriani G, Estes NA 3rd, Spirito P. Efficacy of implantable cardioverter-defibrillators for the prevention of sudden death in patients with hypertrophic cardiomyopathy. N Engl J Med 2000;342:365-73. [Crossref] [PubMed]
- Elliott PM, Anastasakis A, Borger MA, Borggrefe M, Cecchi F, et al. 2014 ESC Guidelines on diagnosis and management of hypertrophic cardiomyopathy: the Task Force for the Diagnosis and Management of Hypertrophic Cardiomyopathy of the European Society of Cardiology (ESC). Eur Heart J 2014;35:2733-79. [Crossref] [PubMed]
- Maron BJ, Rowin EJ, Maron MS. Evolution of risk stratification and sudden death prevention in hypertrophic cardiomyopathy: Twenty years with the implantable cardioverter-defibrillator. Heart Rhythm 2021;18:1012-23. [Crossref] [PubMed]
- Limongelli G, Monda E. Implantable cardioverter defibrillator in hypertrophic cardiomyopathy: Time to avoid unnecessary procedure. Int J Cardiol 2022;355:30-1. [Crossref] [PubMed]
- Andre F, Steen H, Matheis P, Westkott M, Breuninger K, Sander Y, Kammerer R, Galuschky C, Giannitsis E, Korosoglou G, Katus HA, Buss SJ. Age- and gender-related normal left ventricular deformation assessed by cardiovascular magnetic resonance feature tracking. J Cardiovasc Magn Reson 2015;17:25. [Crossref] [PubMed]
- Scatteia A, Baritussio A, Bucciarelli-Ducci C. Strain imaging using cardiac magnetic resonance. Heart Fail Rev 2017;22:465-76. [Crossref] [PubMed]
- Zhu L, Gu S, Wang Q, Zhou X, Wang S, Fu C, Yang W, Wetzl J, Yan F. Left ventricular myocardial deformation: a study on diastolic function in the Chinese male population and its relationship with fat distribution. Quant Imaging Med Surg 2020;10:634-45. [Crossref] [PubMed]
- Muresan ID, Zlibut A, Orzan RI, Agoston R, Cojan-Minzat BO, Grosu AI, Horvat CCD, Agoston-Coldea L. Characterization of left atrial geometry and function in patients with hypertrophic cardiomyopathy: a cardiac magnetic resonance imaging study. Eur Rev Med Pharmacol Sci 2022;26:4318-30. [PubMed]
- Chen X, Pan J, Shu J, Zhang X, Ye L, Chen L, Hu Y, Yu R. Prognostic value of regional strain by cardiovascular magnetic resonance feature tracking in hypertrophic cardiomyopathy. Quant Imaging Med Surg 2022;12:627-41. [Crossref] [PubMed]
- Lee HJ, Kim HK, Lee SC, Kim J, Park JB, Hwang IC, Choi YJ, Lee SP, Chang SA, Lee W, Park EA, Cho GY, Kim YJ. Supplementary role of left ventricular global longitudinal strain for predicting sudden cardiac death in hypertrophic cardiomyopathy. Eur Heart J Cardiovasc Imaging 2022;23:1108-16. [Crossref] [PubMed]
- He J, Yang W, Wu W, Li S, Yin G, Zhuang B, Xu J, Sun X, Zhou D, Wei B, Sirajuddin A, Teng Z, Zhao S, Kureshi F, Lu M. Early Diastolic Longitudinal Strain Rate at MRI and Outcomes in Heart Failure with Preserved Ejection Fraction. Radiology 2021;301:582-92. [Crossref] [PubMed]
- Leng S, Tan RS, Zhao X, Allen JC, Koh AS, Zhong L. Validation of a rapid semi-automated method to assess left atrial longitudinal phasic strains on cine cardiovascular magnetic resonance imaging. J Cardiovasc Magn Reson 2018;20:71. [Crossref] [PubMed]
- Alfuhied A, Kanagala P, McCann GP, Singh A. Multi-modality assessment and role of left atrial function as an imaging biomarker in cardiovascular disease. Int J Cardiovasc Imaging 2021;37:3355-69. [Crossref] [PubMed]
- Lee HJ, Kim HK, Rhee TM, Choi YJ, Hwang IC, Yoon YE, Park JB, Lee SP, Kim YJ, Cho GY. Left Atrial Reservoir Strain-Based Left Ventricular Diastolic Function Grading and Incident Heart Failure in Hypertrophic Cardiomyopathy. Circ Cardiovasc Imaging 2022;15:e013556. [Crossref] [PubMed]
- Nguyen J, Weber J, Hsu B, Mulyala RR, Wang L, Cao JJ. Comparing left atrial indices by CMR in association with left ventricular diastolic dysfunction and adverse clinical outcomes. Sci Rep 2021;11:21331. [Crossref] [PubMed]
- Smith BM, Dorfman AL, Yu S, Russell MW, Agarwal PP, Ghadimi Mahani M, Lu JC. Relation of strain by feature tracking and clinical outcome in children, adolescents, and young adults with hypertrophic cardiomyopathy. Am J Cardiol 2014;114:1275-80. [Crossref] [PubMed]
- Xu HY, Chen J, Yang ZG, Li R, Shi K, Zhang Q, Liu X, Xie LJ, Jiang L, Guo YK. Early marker of regional left ventricular deformation in patients with hypertrophic cardiomyopathy evaluated by MRI tissue tracking: The effects of myocardial hypertrophy and fibrosis. J Magn Reson Imaging 2017;46:1368-76. [Crossref] [PubMed]
- Bogaert J, Olivotto I. MR Imaging in Hypertrophic Cardiomyopathy: From Magnet to Bedside. Radiology 2014;273:329-48. [Crossref] [PubMed]
- Mandinov L, Eberli FR, Seiler C, Hess OM. Diastolic heart failure. Cardiovasc Res 2000;45:813-25. [Crossref] [PubMed]
- Yang F, Wang L, Wang J, Pu L, Xu Y, Li W, Wan K, Yang D, Sun J, Han Y, Zhu Y, Chen Y. Prognostic value of fast semi-automated left atrial long-axis strain analysis in hypertrophic cardiomyopathy. J Cardiovasc Magn Reson 2021;23:36. [Crossref] [PubMed]
- Yang LT, Yamashita E, Nagata Y, Kado Y, Oshima S, Otsuji Y, Takeuchi M. Prognostic value of biventricular mechanical parameters assessed using cardiac magnetic resonance feature-tracking analysis to predict future cardiac events. J Magn Reson Imaging 2017;45:1034-45. [Crossref] [PubMed]
- Vasquez N, Ostrander BT, Lu DY, Ventoulis I, Haileselassie B, Goyal S, Greenland GV, Vakrou S, Olgin JE, Abraham TP, Abraham MR. Low Left Atrial Strain Is Associated With Adverse Outcomes in Hypertrophic Cardiomyopathy Patients. J Am Soc Echocardiogr 2019;32:593-603.e1. [Crossref] [PubMed]
- Hussain K, Nso N, Tsourdinis G, Haider S, Mian R, Sanagala T, Erwin JP 3rd, Pursnani A. A systematic review and meta-analysis of left atrial strain in hypertrophic cardiomyopathy and its prognostic utility. Curr Probl Cardiol 2024;49:102146. [Crossref] [PubMed]