Effect of contrast agent on T2-weighted fat-suppressed imaging and diffusion-weighted imaging in the diagnosis of breast tumors
Introduction
In recent years, breast cancer has surpassed lung cancer as the cancer with the highest diagnosis rate in the world and the leading cause of cancer death among women worldwide (1,2). Early and accurate diagnosis and timely treatment can improve breast cancer staging, reduce mortality, and increase cure rates (3).
About 90% of breast cancers are diagnosed by magnetic resonance images (MRI), compared with 38% for both mammography and ultrasound (4). According to the American Society of Radiology (ACR) and breast imaging association guidelines (5,6), T1-weighted imaging (T1WI) sequence, T2-weighted fat-suppressed (T2 FS) sequence, T1WI-enhanced scan sequence, and diffusion weighted imaging (DWI) sequence are the recommended sequences for diagnosing breast cancer. Multiparametric evaluation of these sequences can well distinguish benign and malignant breast lesions, which can achieve a high-precision diagnosis of breast cancer, which is of great significance for the diagnosis of breast cancer (7-12). At present, there are many simplified protocols available, but there is no complete standardization process. Their design is usually based on a single specific clinical problem. Traditional breast MRI scans typically place T2 FS sequences and DWI sequences before contrast-enhanced scans. Some centers, including our institution, prefer to perform T2 FS and DWI sequence scans after the administration of contrast agents, because in certain special circumstances, patients may not tolerate subsequent dynamic contrast-enhanced (DCE) scans, potentially compromising the image quality (13-17). Furthermore, previous studies have failed to provide systematic quantitative metrics to assess the potential impact of contrast agent injection on T2 FS and DWI sequence images. Our study aims to investigate whether the injection of contrast agents affects the images of T2 FS sequences and DWI sequences, including signal-to-noise ratio (SNR) and the contrast-to-noise ratio (CNR) for the T2 sequence and apparent diffusion coefficient (ADC) values for the DWI sequence. This series of studies will provide valuable insights and quantitative data for clinical practice to help physicians better select the appropriate scanning protocol, thereby improving diagnostic accuracy and treatment outcomes for patients with breast disease.
Studies (18-20) have shown a different effect on the ADC of 3T magnetic resonance after gadolinium contrast injection, which we suspect may be related to the scanning time point after contrast injection. Overall, there are few studies on the effect of contrast injection on image quality, and there are no quantitative studies on T2 FS sequence image quality and changes between pre- and post-contrast ADC.
Therefore, we redesigned the scanning protocol with the aim to comprehensively investigate the image quality of T2 FS sequence after contrast injection, the changes in pre- and post-contrast ADC, the impact of these changes on the identification of different types of masses, and the effect on the ability to differentiate between benign and malignant breast lesions. We present this article in accordance with the STROBE reporting checklist (available at https://qims.amegroups.com/article/view/10.21037/qims-23-1700/rc).
Methods
Patients
A total of 178 patients from the Second People’s Hospital of Yibin, who underwent breast cancer MRI from October 2021 to April 2022, were consecutively included in this study. This prospective cohort study was conducted in accordance with the Declaration of Helsinki (as revised in 2013) and was approved by the Institutional Review Board of the Second People’s Hospital of Yibin (No. 2023-104-01). After providing a complete description of the study, informed written consent was obtained from each participant before the study.
MRI
All patients were scanned with bilateral 10-channel coils on a 3.0T MRI system (uMR790; China Shanghai United Imaging Medical Co., Ltd., Shanghai, China). During the whole examination, all patients were carefully instructed to breathe normally and not to move. Breast MRI sequences included axial fast spin-echo Dixon sequences (including T2 FS), T1W fast spin-echo sequences, DWI sequences (a spin-echo sequence immediately followed by an epi acquisition module and a diffusion module), ultra-fast DCE sequences, and conventional DCE sequences (Figure 1). In all sequences, the patient was imaged in the prone position with free breathing. In the beginning of the third phase of the 30-phase ultra-fast contrast-enhanced sequence (P30), 0.1 mmol/kg of MR contrast agent (gadopentetate dimeglumine; Guangzhou Chenkang Pharmaceutical Co., Ltd., Guangzhou, China) was injected at a flow rate of 3 mL/s. The remaining parameters are detailed in Table 1.
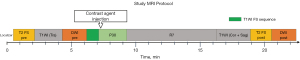
Table 1
MR parameters | Ultra-fast DCE-MRI | T2 FS | DWI |
---|---|---|---|
TR/TE (ms) | 4.66/1.96 | 3,700/75.6 | 3,800/72.4 |
Thickness (mm) | 1.1 | 5 | 5 |
Number of slices | 138 | 24 | 24 |
Flip angle | 10° | 90° | 90° |
FOV (mm) | 340×340 | 360×360 | 360×360 |
Matrix | 336×336 | 336×336 | 240×240 |
b value (s/mm2) | – | – | 50, 800 |
DCE-MRI, dynamic contrast enhanced-magnetic resonance imaging; T2 FS, T2-weighted fat-suppressed; DWI, diffusion weighted imaging; TR/TE, repetition time/echo time; FOV, field of view.
After the localization sequence (scan time: 18 seconds), the first images acquired were a pre-T2 FS sequence (scan time: 1 min 36 s) and a T1W axial sequence (scan time: 2 min 10 s). Subsequently, a pre-DWI sequence (scan time: 1 min 47 s) was performed, followed by a DCE-MRI examination after an ultra-rapid T1W fat suppression sequence (scan time: 1 min). This consisted of a 30-phase ultra-fast contrast-enhanced sequence (P30, scan time: 2 min 10 s) and a 7-phase conventional sequence (R7, scan time: 7 min). Contrast was injected at the beginning of the third stage of P30. After the contrast-enhanced scan was completed, T1W coronal and sagittal scans were performed (scan time: 3 min 50 s). Subsequently, a post-T2 FS sequence (scan time: 1 min 36 s) and a post-DWI sequence (scan time: 1 min 47 s) were performed. The total scan time was approximately 23 minutes.
Image analysis
All images were transferred to a dedicated post-processing station for analysis. A radiologist (Mulan) conducted an independent review using the uMR790 workstation, excluding the following data: (I) 12 patients with incomplete pathological information and diagnoses, including medullary carcinoma, breast carcinoma, encapsulated papillary carcinoma, and Paget’s disease; (II) 25 cases with lesion diameters smaller than 1 cm, making it difficult to delineate regions of interest (ROI); (III) 78 patients exhibited image artifacts, with 56 cases of artifacts in any T2 FS image and 22 cases of artifacts in any DWI image. In the end, data from 90 patients were collected, constituting a T2 FS dataset of 140 lesions, including 99 invasive carcinoma, 21 carcinoma in situ, and 20 benign tumors. A total of 124 patients contributed to an ADC dataset of 142 lesions, including 104 invasive carcinoma, 23 carcinoma in situ, and 15 benign tumors.
Referring to the enhanced images from the 20th phase of ultra-fast contrast-enhanced imaging post-injection, we positioned the lesion ROI (ROI tumor) and the healthy glandular tissue ROI (ROI normal) on the pre-contrast T2 FS images. We measured and recorded the signal intensity (SI) for these ROIs. Additionally, we placed ROIs (ROI noise) in the upper left of the image and in background (air) anterior to patients’ chest wall to capture and average the standard deviation (SD) of the measured SI. Care was taken to avoid tissues such as fat, vessels, and air that could affect the measurements, ensuring the selected lesion area displayed the utmost uniformity in imaging. These ROI areas were then replicated onto the post-contrast T2 FS sequence images, as shown in Figure 2. Subsequently, the SNR and the CNR were calculated using the following formula:

The ROIs were manually plotted in the darkest portion of the ADC image prior to contrast injection, avoiding necrotic, noisy, or non-enhancing lesion voxels, with reference to the enhanced image of ultra-fast contrast-enhanced imaging phase 20 after injection of the contrast agent. The ROIs described above were then copied into the ADC sequence after injection of the contrast agent. The position of the ROIs was further manually adjusted in case of slight misalignment due to patient movement.
Statistical analysis
Differences in SNR and CNR in T2 FS sequences before and after contrast injection were assessed using the Wilcoxon signed-rank test, and furthermore, differences in ADC values between different tumor types (invasive carcinoma, carcinoma in situ, and benign tumors) were compared before and after contrast injection, Discriminatory power between pre- and post-contrast ADC in identifying different tumor types were compared using the Kruskal-Wallis H-test and the paired comparison test. The accuracy of ADC values between pre- and post-contrast ADC in distinguishing benign and malignant breast masses was assessed using receiver operating characteristic (ROC) curves.
Results
Comparison of pre- and post-contrast SNR and CNR of T2 FS sequence of different breast lesions
The SNR and CNR of T2 FS of breast lesions and different types of tumors before and after contrast injection and the corresponding P values are listed in Table 2. The SNR of T2 FS images of benign tumors increased significantly from 82.22±27.96 to 127.86±85.34 (P=0.006) and the CNR from 71.83±26.97 to 114.06±81.47 (P=0.005) after injection of contrast agent. The SNR of T2 FS images of invasive carcinoma increased significantly from 69.72±34.326 to 112.99±57.81 (P<0.001), and the CNR increased significantly from 60.96±31.80 to 100.14±53.74 (P<0.001). The SNR of T2 FS images of carcinoma in situ increased significantly from 55.72±32.04 to 96.94±53.13 (P<0.001), and the CNR increased significantly from 50.04±29.57 to 87.15±47.72 (P<0.001).
Table 2
Wilcoxon signed-rank test | Benign tumor (N=20) | Invasive carcinoma (N=99) | Carcinoma in situ (N=21) | |||||
---|---|---|---|---|---|---|---|---|
SNR | CNR | SNR | CNR | SNR | CNR | |||
Pre-contrast | 82.22±27.96 | 71.83±26.97 | 69.72±34.32 | 60.96±31.80 | 55.72±32.04 | 50.04±29.57 | ||
Post-contrast | 127.86±85.34 | 114.06±81.47 | 112.99±57.81 | 100.14±53.74 | 96.94±53.13 | 87.15±47.72 | ||
P value | 0.006 | 0.005 | <0.001 | <0.001 | <0.001 | <0.001 |
The value is presented as mean ± SD. SNR, signal-to-noise ratio; CNR, contrast-to-noise ratio; T2 FS, T2-weighted fat-suppressed; SD, standard deviation.
Comparison of pre- and post-contrast ADC values and its differentiation in different breast lesions
Considering all lesions collectively, the pre-contrast ADC values exhibited a slight reduction from (1.159±0.410)×10−3 to (1.156±0.406)×10−3 mm2/s at post-contrast. In benign tumors, the pre-contrast ADC values experienced a marginal decrease from (1.860±0.551)×10−3 to (1.841±0.555)×10−3 mm2/s at post-contrast. Among invasive carcinomas, the pre-contrast ADC values minimally dropped from (1.005±0.166)×10−3 to (1.004±0.166)×10−3 mm2/s at post-contrast. In the case of in situ carcinomas, the pre-contrast ADC values displayed a minor increase from (1.153±0.186)×10−3 to (1.158±0.174)×10−3 mm2/s. However, it is important to emphasize that the observed changes did not reach statistical significance, as depicted in Figure 3.
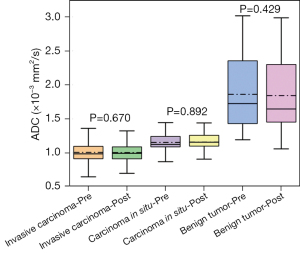
Differentiation of pre- and post-contrast ADC in different breast lesions is shown in Table 3. The results showed that the difference in ADC between invasive carcinoma and carcinoma in situ before and after contrast was significant (P=0.02, P=0.006). The difference in ADC between carcinoma in situ and benign tumor before and after comparison was significant (P=0.009, P=0.03), whereas the difference in ADC between benign tumor and invasive carcinoma was highly significant (P<0.001). Figure 4 shows representative images of DWI and ADC sequences before and after contrast injection for invasive carcinoma.
Table 3
Kruskal-Wallis H-test | Pre ADC (P) | Post ADC (P) |
---|---|---|
Invasive carcinoma vs. carcinoma in situ | 0.02 | 0.006 |
Carcinoma in situ vs. benign tumor | 0.009 | 0.03 |
Benign tumor vs. invasive carcinoma | <0.001 | <0.001 |
ADC, apparent diffusion coefficient.
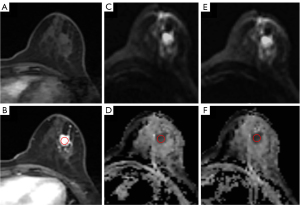
Comparison of the accuracy in distinguishing benign and malignant lesions based on pre- and post-contrast ADC values
The accuracy of pre- and post-contrast ADC values for benign and malignant breast lesions was 0.894 (0.832–0.940) and 0.900 (0.838–0.944), as shown in Table 4. Delong test showed that there was no significant difference among pre- and post-contrast ADC values (P=0.61). The area under the curve (AUC) for diagnosis of benign and malignant tumors comparing pre- and post-contrast ADC values is shown in Figure 5.
Table 4
ADC measurement | Pre ADC | Post ADC |
---|---|---|
AUC | 0.894 | 0.900 |
95% CI | 0.832–0.940 | 0.838–0.944 |
Accuracy | 0.817 | 0.845 |
Sensitivity | 0.789 | 0.837 |
Specificity | 0.895 | 0.868 |
Youden index | 0.683 | 0.705 |
Cut-off | 1.104 | 1.120 |
ADC, apparent diffusion coefficient; AUC, area under the curve; CI, confidence interval.
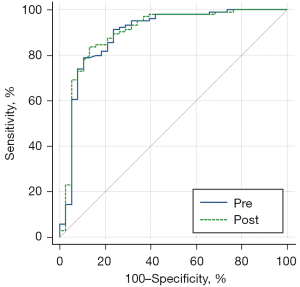
Discussion
In this study, SNR and CNR were calculated for all breast lesions on T2 FS sequence images before and after contrast injection. These lesions were further classified into 3 typical lesion types (invasive carcinoma, carcinoma in situ, and benign tumor) in order to investigate whether there was a significant difference in the ADC values of these lesions before and after contrast injection and whether this difference would affect the interpretation of breast disease results.
T2 FS may improve lesion classification by demonstrating peri- or anterior breast edema, a known marker of poor prognosis in patients with breast cancer. Several studies have reported that T2WI (including T2 FS) improves the specificity of differentiating benign and malignant lesions, and others have suggested that improving the quality of T2WI images by combining T2WI and DWI sequences can help in the diagnosis of breast cancer (11,20-23). Therefore, improving the quality of T2WI images is very important for breast cancer diagnosis. As it happens, the experimental results showed that the SNR and CNR of T2 FS sequences of all breast lesions before and after contrast injection were improved by 62.38% and 64.55%, respectively, which indicates that contrast injection is beneficial to improve the imaging quality of T2 FS.
According to previous studies, when the concentration of gadolinium-based contrast agents is low, the effect on T1 relaxation time is more pronounced due to the longer T1 relaxation time of bodily tissues. This is manifested in T1WI as an increase in SI in tissues containing contrast agents. As the concentration increases, the T2-shortening effect gradually becomes more evident. When the concentration is significantly higher than the clinical dose (0.1–0.2 mmol/kg), T2 shortening is pronounced, and the T2 enhancement effect surpasses the T1 shortening effect. In this case, if T2-weighted imaging is used, tissues containing contrast agents will exhibit a reduction in signal. This phenomenon is also known as negative contrast enhancement (24-26). This indicates that T2 relaxation time can also be shortened, but a certain high concentration is required to visually display it on the image. In our study, the magnetic resonance contrast agent was administered at a low concentration of 0.1 millimoles per kilogram, which is the standard clinical dose. The acquisition time was approximately 12 minutes after the injection of the contrast agentand the absolute effects of 0.1 mmol/kg dose on 1/T1 and 1/T2 are very similar. The Relaxivity of magnetic resonance contrast agent is a function of the relaxation rate of tissue with the change of contrast agent concentration. which is larger than 1/T1, but smaller than 1/T2. Therefore, the signal attenuation in T2 image is far less obvious than that in T1 signal enhancement, and it is even possible that T1 enhancement effect compensates the signal attenuation caused by T2 shortening, which leads to the increase of T2 SI and the improvement of image signal after contrast agent application.
DWI is a special functional imaging sequence in MRI sequences, which is the only examination method to date that can noninvasively detect the diffusive motion of water molecules in living tissues, and ADC value is the main quantitative parameter. This study demonstrated that for invasive carcinoma, carcinoma in situ, and benign neoplastic lesions, there was a significant difference in ADC values prior to contrast injection. Invasive carcinoma exhibited lower ADC values, which was attributed to the tissue destruction and abnormal cell proliferation typical of these lesions. The overpopulation of malignant cells disrupts the tissue structure, increases cell density, and alters the microstructure, leading to changes in ADC values. These factors limited the spread of water within the tumor. Carcinoma in situ is an early-stage cancer in which the abnormal cell proliferation remains confined to the original tissue and does not invade surrounding tissues. Carcinoma in situ is defined as a noninvasive breast cancer in which the abnormal cells are confined to the ducts or lobules without invading the surrounding tissue. Carcinoma in situ typically exhibits lower cell density and less structural tissue destruction than invasive carcinoma. As a result, carcinoma in situ tends to have higher baseline ADC values than invasive carcinoma. Benign breast tumors are noncancerous growths and lack the invasive characteristics of malignant tumors. These tumors typically have lower cell densities and more preserved tissue structure than invasive carcinomas and carcinomas in situ, resulting in higher ADC values.
The experimental results showed that the ADC values of breast lesions (including benign tumors and invasive carcinoma) at 13 minutes after the injection of the contrast agent were generally lower than those before the contrast injection, and this result was considered to originate from the principle of the T2 shortening effect, which means that the gadolinium contrast agent reduces the SI of the intravascular fluid, leading to the inhibition of the perfusion effect, which results in the decrease of the ADC values. At the same time, the gadolinium contrast agent is a paramagnetic substance, which increases the magnetic field distribution around the vessel and decreases the T1 and T2 thresholds, thus further affecting the ADC values. This result has now been confirmed by several studies (27,28). However, this trend was not shown in carcinoma in situ, considering that it may be related to the biological characteristics of carcinoma in situ and warrants further study in future data with large samples. In this study, these differences were not statistically significant, and the reason for this might be related to the gradual decrease of the contrast agent in the lesion microcirculation over time (29).
Nguyen et al. (18) demonstrated that there was no difference in ADC values before and after contrast agent injection when the interval time between pre and post was 9 minutes. This current study, offering a larger-scale prospective sample with a greater number of cases and a broader spectrum of malignant lesion subtypes, reinforces previous research findings. It can provide new perspectives for the development of related fields and could be beneficial for future investigations into simplified sequences for ultrafast MRI. Additionally, studies have suggested that the possible cause of ADC decrease may be the T2* effect (29), which is attributed to signal attenuation caused by inhomogeneous main magnetic field. However, the DWI sequence used in the present study is spin-echo sequence, which corrects the signal attenuation of inhomogeneous main magnetic field and excludes the effect of T2* effect.
According to ROC analysis, the ADC values of pre-contrast and post-contrast were 0.978 and 0.967, respectively, which are capable of distinguishing benign and malignant tumors (30). The range of ADC thresholds reported in the literature for identifying benign and malignant breast lesions is (1.1–1.6)×10−3 mm2/s, and the results of the present study showed that the diagnostic thresholds for pre- and post-enhancement ADC values were 1.222×10−3 and 1.301×10−3 mm2/s, respectively, which is in agreement with the previous result.
This study has several limitations. Firstly, there is a scarcity of data concerning specific types of breast cancer (e.g., medullary carcinoma, mucinous carcinoma, encapsulated papillary carcinoma, Paget’s disease, etc.). The lack of comparative experiments involving ADC values for DWI and SNR/CNR values for T2 FS before and after contrast agent injection for these specific types is notable. Secondly, we conducted experiments using only 1 specification of contrast agent (gadopentetate dimeglumine, 15 mL, 7.04 g). Further research is needed to investigate the effects of different contrast agents on SNR/CNR values of T2 FS and ADC values. Thirdly, the b values used in this study were 50 and 800, and it is acknowledged that different b values may impact the ADC value. Future exploration of the relationship between a broader range of b values and ADC values would be beneficial. Fourthly, the study involved a limited number of cases, with an uneven distribution among the 3 tumor types, possibly introducing some degree of randomness. Further experimental analysis could be performed with an expanded dataset to address these limitations. Lastly, although we did not control for the parallel imaging techniques in DWI, which might affect the measurement of background noise, it is important to note that the same imaging acquisition method was used both before and after the injection of the contrast agent. Considering that the focus of this study is on the changes following the injection of the contrast agent, we did not take specific measures regarding this issue. Future research will further explore this matter.
Conclusions
Contrast agent injection can improve the SNR and CNR of T2 FS, thus providing higher quality images for breast lesions diagnosis. Furthermore, injection of contrast agent had little effect on the ability of ADC values to identify different types of lesions and both ADC values before and after the contrast agent were able to distinguish between benign and malignant tumors with almost the same accuracy.
Acknowledgments
Funding: The study was partially supported by
Footnote
Reporting Checklist: The authors have completed the STROBE reporting checklist. Available at https://qims.amegroups.com/article/view/10.21037/qims-23-1700/rc
Conflicts of Interest: All authors have completed the ICMJE uniform disclosure form (available at https://qims.amegroups.com/article/view/10.21037/qims-23-1700/coif). S.Z. and S.Y. are employees of Shanghai United Imaging Healthcare. Na Zhang is an employee of the United Imaging Research Institute of Innovative Medical Equipment. The other authors have no conflicts of interest to declare.
Ethical Statement: The authors are accountable for all aspects of the work in ensuring that questions related to the accuracy or integrity of any part of the work are appropriately investigated and resolved. This prospective cohort study was conducted in accordance with the Declaration of Helsinki (as revised in 2013) and was approved by the Institutional Review Board of the Second People’s Hospital of Yibin (No. 2023-104-01). After providing a complete description of the study, informed written consent was obtained from each participant before the study.
Open Access Statement: This is an Open Access article distributed in accordance with the Creative Commons Attribution-NonCommercial-NoDerivs 4.0 International License (CC BY-NC-ND 4.0), which permits the non-commercial replication and distribution of the article with the strict proviso that no changes or edits are made and the original work is properly cited (including links to both the formal publication through the relevant DOI and the license). See: https://creativecommons.org/licenses/by-nc-nd/4.0/.
References
- Siegel RL, Miller KD, Wagle NS, Jemal A. Cancer statistics, 2023. CA Cancer J Clin 2023;73:17-48. [Crossref] [PubMed]
- Fan L, Strasser-Weippl K, Li JJ, St Louis J, Finkelstein DM, Yu KD, Chen WQ, Shao ZM, Goss PE. Breast cancer in China. Lancet Oncol 2014;15:e279-89. [Crossref] [PubMed]
- Zhang M, Bao H, Zhang X, Huang Z, Zhao Z, Li C, Zhou M, Wu J, Wang L, Wang L. Breast Cancer Screening Coverage - China, 2018-2019. China CDC Wkly 2023;5:321-6. [Crossref] [PubMed]
- Montemezzi S, Cardano G, Storer S, Cardobi N, Cavedon C, Camera L. MRI-guided breast biopsy based on diffusion-weighted imaging: a feasibility study. Eur Radiol 2021;31:2645-56. [Crossref] [PubMed]
- Monticciolo DL, Newell MS, Hendrick RE, Helvie MA, Moy L, Monsees B, Kopans DB, Eby PR, Sickles EA. Breast Cancer Screening for Average-Risk Women: Recommendations From the ACR Commission on Breast Imaging. J Am Coll Radiol 2017;14:1137-43. [Crossref] [PubMed]
- Bhushan A, Gonsalves A, Menon JU. Current State of Breast Cancer Diagnosis, Treatment, and Theranostics. Pharmaceutics 2021;13:723. [Crossref] [PubMed]
- Zhang M, Horvat JV, Bernard-Davila B, Marino MA, Leithner D, Ochoa-Albiztegui RE, Helbich TH, Morris EA, Thakur S, Pinker K. Multiparametric MRI model with dynamic contrast-enhanced and diffusion-weighted imaging enables breast cancer diagnosis with high accuracy. J Magn Reson Imaging 2019;49:864-74. [Crossref] [PubMed]
- Li Z, Li J, Lu X, Qu M, Tian J, Lei J. The diagnostic performance of diffusion-weighted imaging and dynamic contrast-enhanced magnetic resonance imaging in evaluating the pathological response of breast cancer to neoadjuvant chemotherapy: A meta-analysis. Eur J Radiol 2021;143:109931. [Crossref] [PubMed]
- Sharma U, Agarwal K, Hari S, Mathur SR, Seenu V, Parshad R, Jagannathan NR. Role of diffusion weighted imaging and magnetic resonance spectroscopy in breast cancer patients with indeterminate dynamic contrast enhanced magnetic resonance imaging findings. Magn Reson Imaging 2019;61:66-72. [Crossref] [PubMed]
- Yuen S, Monzawa S, Yanai S, Matsumoto H, Yata Y, Ichinose Y, Deai T, Hashimoto T, Tashiro T, Yamagami K. The association between MRI findings and breast cancer subtypes: focused on the combination patterns on diffusion-weighted and T2-weighted images. Breast Cancer 2020;27:1029-37. [Crossref] [PubMed]
- Kim KW, Kuzmiak CM, Kim YJ, Seo JY, Jung HK, Lee MS. Diagnostic Usefulness of Combination of Diffusion-weighted Imaging and T2WI, Including Apparent Diffusion Coefficient in Breast Lesions: Assessment of Histologic Grade. Acad Radiol 2018;25:643-52. [Crossref] [PubMed]
- Partridge SC, McDonald ES. Diffusion weighted magnetic resonance imaging of the breast: protocol optimization, interpretation, and clinical applications. Magn Reson Imaging Clin N Am 2013;21:601-24. [Crossref] [PubMed]
- Rubesova E, Grell AS, De Maertelaer V, Metens T, Chao SL, Lemort M. Quantitative diffusion imaging in breast cancer: a clinical prospective study. J Magn Reson Imaging 2006;24:319-24. [Crossref] [PubMed]
- Le TT, McDonald ES, Isaac G, Rosen MA, Dougherty L. Effect of contrast dose on diagnostic performance in DCE-MR breast imaging. J Appl Clin Med Phys 2020;21:188-94. [Crossref] [PubMed]
- Al Ewaidat H, Ayasrah M. A Concise Review on the Utilization of Abbreviated Protocol Breast MRI over Full Diagnostic Protocol in Breast Cancer Detection. Int J Biomed Imaging 2022;2022:8705531. [Crossref] [PubMed]
- Metin Y, Orhan Metin N, Kul S, Taşçı F, Özdemir O, Küpeli A. High-resolution diffusion-weighted imaging compared with conventional diffusion-weighted imaging and dynamic contrast-enhanced magnetic resonance imaging with regard to image quality and assessment of breast cancer morphology. Diagn Interv Radiol 2023;29:251-9. [Crossref] [PubMed]
- Li X, Wang H, Gao J, Jiang L, Chen M. Quantitative apparent diffusion coefficient metrics for MRI-only suspicious breast lesions: any added clinical value? Quant Imaging Med Surg 2023;13:7092-104. [Crossref] [PubMed]
- Nguyen VT, Rahbar H, Olson ML, Liu CL, Lehman CD, Partridge SC. Diffusion-weighted imaging: Effects of intravascular contrast agents on apparent diffusion coefficient measures of breast malignancies at 3 Tesla. J Magn Reson Imaging 2015;42:788-800. [Crossref] [PubMed]
- Fanariotis M, Tsougos I, Vlychou M, Fezoulidis I, Vassiou K. Contrast-enhanced and unenhanced diffusion-weighted imaging of the breast at 3 T. Clin Radiol 2018;73:928-35. [Crossref] [PubMed]
- Janka R, Hammon M, Geppert C, Nothhelfer A, Uder M, Wenkel E. Diffusion-weighted MR imaging of benign and malignant breast lesions before and after contrast enhancement. Rofo 2014;186:130-5. [Crossref] [PubMed]
- Mann RM, Cho N, Moy L. Breast MRI: State of the Art. Radiology 2019;292:520-36. [Crossref] [PubMed]
- Min Q, Shao K, Zhai L, Liu W, Zhu C, Yuan L, Yang J. Differential diagnosis of benign and malignant breast masses using diffusion-weighted magnetic resonance imaging. World J Surg Oncol 2015;13:32. [Crossref] [PubMed]
- Ramtohul T, Tescher C, Vaflard P, Cyrta J, Girard N, Malhaire C, Tardivon A. Prospective Evaluation of Ultrafast Breast MRI for Predicting Pathologic Response after Neoadjuvant Therapies. Radiology 2022;305:565-74. [Crossref] [PubMed]
- Taheri S, Shah NJ, Rosenberg GA. Analysis of pharmacokinetics of Gd-DTPA for dynamic contrast-enhanced magnetic resonance imaging. Magn Reson Imaging 2016;34:1034-40. [Crossref] [PubMed]
- Ha R, Chang P, Mutasa S, Karcich J, Goodman S, Blum E, Kalinsky K, Liu MZ, Jambawalikar S. Convolutional Neural Network Using a Breast MRI Tumor Dataset Can Predict Oncotype Dx Recurrence Score. J Magn Reson Imaging 2019;49:518-24. [Crossref] [PubMed]
- Mastarone DJ, Harrison VS, Eckermann AL, Parigi G, Luchinat C, Meade TJ. A modular system for the synthesis of multiplexed magnetic resonance probes. J Am Chem Soc 2011;133:5329-37. [Crossref] [PubMed]
- Ramadan S, Mulkern RV. Comment on ADC reductions in postcontrast breast tumors. J Magn Reson Imaging 2010;31:262-author reply 263-4. [Crossref] [PubMed]
- Pesapane F, Rotili A, Penco S, Montesano M, Agazzi GM, Dominelli V, Trentin C, Pizzamiglio M, Cassano E. Inter-Reader Agreement of Diffusion-Weighted Magnetic Resonance Imaging for Breast Cancer Detection: A Multi-Reader Retrospective Study. Cancers (Basel) 2021;13:1978. [Crossref] [PubMed]
- Thomassin-Naggara I, De Bazelaire C, Chopier J, Bazot M, Marsault C, Trop I. Diffusion-weighted MR imaging of the breast: advantages and pitfalls. Eur J Radiol 2013;82:435-43. [Crossref] [PubMed]
- Chen X, He XJ, Jin R, Guo YM, Zhao X, Kang HF, Mo LP, Wu Q. Conspicuity of breast lesions at different b values on diffusion-weighted imaging. BMC Cancer 2012;12:334. [Crossref] [PubMed]