Myocardial extracellular volume derived from contrast-enhanced chest computed tomography in longitudinal evaluation of cardiac toxicity in patients treated with immune checkpoint inhibitors
Introduction
Immune checkpoint inhibitors (ICIs) represent a recent research hotspot, which can clearly improve clinical efficacy. First-line immunotherapy has remarkably advanced the therapeutic opportunities (1,2). However, ICIs have been associated with drug-related toxicity, such as myocarditis, which is a rare but terrible complication that may lead to life-threatening heart failure, cardiogenic shock, and arrhythmia (3-7). As of April 2016, among more than 20,000 patients treated with navulizumab and/or epilimumab, 0.09% of all adverse events involved myocarditis, and 0.03% involved fatal myocarditis (4,8). From 2013 to 2018, 122 cases of myocarditis related to ICIs were recorded in the World Health Organization (WHO) Patient Safety Report Database (6), 84% of which were serious cases, and among whom combination therapy was more common than single immunotherapy (66% vs. 40%). The ratio of fatal and general myocarditis caused by combination therapy is approximately 8:2. In addition, the analysis showed that the prevalence of myocarditis after ICIs has increased steadily over the years, for which the medical burden is increasing. This tendency may be attributed to various factors including increased awareness of physicians, advanced imaging technique and is expected to become more extreme in the near future. As ICIs will be administered to more and more people, even though the percentage of total patients treated is still low, the absolute number of individuals with myocarditis is expected to increase gradually (9).
At present, the external clinical phenotype of ICIs-related myocarditis may be the coexistence of 1 or more of the following cardiovascular diseases: heart failure, various arrhythmias, myocarditis-pericarditis, coronary artery disease, sudden death of cardiomyopathy (10). The diagnosis of myocarditis is also complex, based on clinical manifestations and electrocardiography (ECG), transthoracic echocardiography (TTE), cardiac biomarkers, and the results of exclusion of acute coronary syndrome using coronary angiography. Although the gold standard for determining myocarditis is still endocardial myocardial biopsy, it is usually difficult to obtain. Cancer therapy-related cardiac dysfunction (CTRCD) is often used to cover the cardiac damage caused by different anti-tumor treatments (including chemotherapy, targeted drugs, immunotherapy, and radiotherapy). According to the consensus of experts from the American Society of Echocardiography and the European Association of Cardiovascular Imaging, CTRCD is defined as the decrease of left ventricular ejection fraction (LVEF) from baseline by ≥10% or <55% (11) during the follow-up period. Clinically, cardiac troponin T (cTnT), brain natriuretic peptide (BNP), and heart rate can be used to assist diagnosis. However, the disadvantages of the above indicators include the following: (I) many diseases that affect the heart may cause the concentration of cTnT and BNP to be higher than the reference upper limit, such as acute coronary syndrome and pericarditis, and there is the possibility of false negative; (II) in clinical practice, if there is a decrease in LVEF or an abnormal increase in BNP, the heart damage is irreversible and may have developed into heart failure. Therefore, in order to reduce the risk of CTRCD, early identification and monitoring of cardiac toxicity caused by ICIs is very important. At present, there is a lack of imaging examination to assist identification of the occurrence of myocarditis early and effectively.
In recent years, the measurement of extracellular volume (ECV) score calculated by cardiac magnetic resonance (CMR) T1 mapping has become a reliable and non-invasive method to evaluate diffuse myocardial fibrosis caused by myocardial toxicity at the early stage of the disease (12,13). ECV score represents the percentage of myocardium not composed of cells and increases when pathological substances accumulate, such as fibrosis or amyloidosis (8,14). The myocardial ECV value can directly quantify the local and diffuse myocardial damage caused by ischemia or non-ischemia. A study has found that the ECV value has the same merit as LVEF for the prognostic evaluation of myocardial disease (15). Incorporating ECV measurement into clinical CMR routine will help to monitor the changes of ECV over time, thus helping to understand the role of myocardial fibrosis in the process of myocardial disease progression to heart failure. Previous research has shown that CMR-ECV can predict the occurrence of myocarditis caused by cardiotoxic chemotherapeutic drugs such as doxorubicin early and effectively (16). Another study also showed that the elevated myocardial ECV closely correlates with diastolic dysfunction, which can be displayed by CMR (17). However, CMR has many disadvantages, such as long scan time, high heart rate requirements, and unsuitability for patients with claustrophobia; CMR imaging has not been widely utilized in clinical practice in medical institutions. Therefore, compared with CMR, using computed tomography (CT) to complete cardiac scanning may be a more reliable alternative method: (I) CT is very easy to obtain; (II) the measured value of ECV fraction derived from CT can reflect the pathological changes of tumor microvascular environment, tumor angiogenesis, extracellular matrix, and cell density (18); (III) the measured value of ECV score derived from CT can effectively reflect the components of myocardial histology (19), and has a significant correlation with the percentage of fibrosis in myocardial histology (19); (IV) there is also a good correlation between the myocardial ECV value measured by CMR and the myocardial ECV value measured by cardiac CT (20,21). The reason may be that the iodine contrast agent of CT and gadolinium contrast agent of magnetic resonance imaging (MRI) are both extracellular interstitial contrast agents; (V) CT has higher spatial resolution in detecting fibrosis. Furthermore, myocardial tissue characterization with photon-counting detector CT-based quantitative ECV analysis showed a strong correlation to MRI. Photon-counting CT enables accurate ECV quantification using certain scan at low radiation dose (22).
Therefore, the purpose of this study was to evaluate the value of cardiac ECV with enhanced chest CT for longitudinal evaluation of cardiac toxicity in patients receiving immunotherapy. We present this article in accordance with the Materials Design Analysis Reporting (MDAR) reporting checklist (available at https://qims.amegroups.com/article/view/10.21037/qims-23-850/rc).
Methods
Study population
This study was approved by the Ethics Committee of the Chongqing University Cancer Hospital. Due to the design of the retrospective study, the necessity of written informed consent was waived. The study was conducted in accordance with the Declaration of Helsinki (as revised in 2013). Patients with malignant tumors, including lung cancer and esophageal cancer, who received immunotherapy programmed cell death protein 1 and programmed cell death ligand 1 (PD-1 and PD-L1) from December 2020 to August 2022 were included. Case recruitment was conducted by two oncologists with more than 14 years of experience. The inclusion criteria were as follows: (I) pathological diagnosis of lung or esophageal cancer (stage III–IV); (II) standardized treatment of ICIs according to guideline; (III) received thoracic contrast-enhanced CT examination at least twice, including baseline (within 2 weeks before ICIs treatment) and the first follow-up (within 1–3 months after the first ICIs treatment). The exclusion criteria were as follows: (I) dubious diagnosis of cardiac toxicity because of the presence of left-sided lung cancer and esophageal cancer which might be suitable for radiotherapy; (II) active or previous heart disease history (such as myocardial infarction, heart failure, primary cardiomyopathy, valvular heart disease, or cardiac sarcoidosis); (III) heart risk factors, such as hypertension or diabetes mellitus; (IV) thoracic CT image has noticeable artifacts; (V) allergy to CT contrast media. Finally, 500 tumor patients receiving ICIs were included. We included the patients who had undergone thoracic contrast-enhanced CT scan within 1–3 months [mean 56 days, interquartile range (IQR), 31–89 days], 3–6 months (mean 145 days, IQR, 91–180 days), and about 12 months (mean 353 days, IQR, 250–367 days) after the first treatment into the first to the third follow-up. The research flow chart is shown in Figure 1.
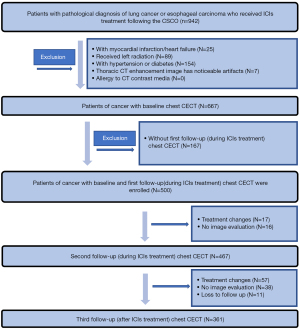
From the patient’s medical records, we collected the patient’s age, height, weight, gender, blood lipids, blood glucose, blood pressure, disease stage, pathological type, and immunotherapy drugs.
CT acquisition protocol
According to the clinical management guidelines for lung cancer and esophageal cancer, it is recommended that patients receive contrast-enhanced thoracic CT examination. CT examination was performed on two different 128 row CT scanners (SOMATOM Definition AS+, Siemens Medical, Erlangen, Germany; SOMATOM Drive, Siemens Healthineers, Erlangen, Germany). The patient was asked to hold their breath during the acquisition, and all scans were performed in the craniocaudal (top-down) direction. The SOMATOM definition AS+ and SOMATOM drive parameters were as follows: tube voltage, 120 kVp; reference tube current, 110 mA; detector collimation, 0.625–1.5 mm; layer spacing, 1.375–1.5. Mediastinal algorithm was used to reconstruct image data with a slice thickness of 1 cm. All patients received enhanced CT scanning of the whole chest including the heart. In our hospital, the follow-up CT scan of lung or esophageal cancer patients routinely uses ungated CT scan, consistent with our protocols.
A non-ionic iodinated contrast agent (Ioversol, 320 mg/mL iodine, HENGRUI Medicine, Jiangsu, China) with total injected volume of 60–80 mL was injected intravenously at a dose of 1.5 mL/kg and a flow rate of 2.5 mL/sec, followed by 30 mL of saline solution at the same flow rate. The automatic exposure control (CARE Dose 4D, Siemens Healthineers, Forchheim, Germany) was used to determine enhanced scanning. Specifically, after the injection of contrast agent, low-dose monitoring images were obtained on the uniaxial sections of the aorta. After injection, the threshold of the descending aorta was 100 Hounsfield units (HU) to trigger arterial phase scanning. The portal phase scan was performed approximately 80 seconds after the descending aorta reached 100 HU.
Image analysis of CT
All images were independently evaluated by two radiologists with 10 and 15 years of experience, respectively. First, the observer selected the slice that could best visualize the left ventricular septum longitudinally on the portal veinous phrase image. Secondly, they manually delineated the region of interest (ROI) in the middle of the interventricular septum. Next, they drew another ROI at the same level in the heart blood pool of the left ventricle to avoid myocardium as much as possible. Then, the 2 ROIs drawn on the portal venous phase image in the previous step were duplicated and placed at similar positions in the unenhanced ventricular septum and blood pool (Figure 2) (23). Due to the influence of free breathing, we chose the interventricular septum at the thickest part of the myocardium during measurement to reduce partial volume effects, respiratory and cardiac movements, and improve the reliability of ECV measurement.
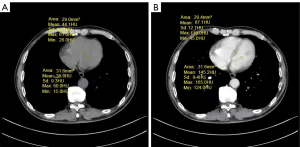
Based on the previous study (24), the septal ECV was calculated using the following formula:
Where myo = myocardium, pre = pre-contrast, and post = post-contrast.
LVEF acquisition and CTRCD definition
All patients had LVEF measured by 2-dimensional echocardiography (2DE; Philips Ultrasound System, Q7, Philips Healthcare, Amsterdam, The Netherlands) within 7 days before or after CT scan.
CTRCD was defined as a ≥10% reduction in LVEF from baseline to <55% by 2DE during the follow-up, according to the American Society of Echocardiography and the European Association of Cardiovascular Imaging expert consensus (11).
The sample collection method of cTnT: collect 2 mL of venous blood of all fasting participants, and use ethylene diamine tetraacetic acid (EDTA) for anticoagulation to detect cTnT. Instruments and reagents: cTnT analysis was performed with Roche fully automatic electrochemiluminescence immunoassay system (Roche, Basel, Switzerland) and supporting Elecsys Troponin T hs STAT. Reference interval: cTnT: the data established in the reference range are from 533 healthy volunteers (age range: 20–71 years), according to Roche Cobas Elecsys high-sensitivity cardiac troponin T (hs-cTnT) test the upper limit (99th percentile) was 14 ng/L, and the 95% confidence interval (CI) was 12.7–24.9 ng/L.
Statistical analysis
The statistical software SPSS 25.0 (IBM Corp., Armonk, NY, USA) was utilized for statistical analyses. The Kolmogorov-Smirnov test was utilized for assessment of the normality of the quantitative data distribution. Continuous data were summarized as the mean ± SD or median and IQR; categorical data were expressed as frequencies or percentages. Student’s t-tests or chi-square tests were used in comparisons between patients with and without CRTCD. The differences among ECVs and cTnTs at baseline and at different follow-up times were compared using a one-way analysis of variance (ANOVA) with the Bonferroni post hoc test. The longitudinal change of ECV and cTnT with time was represented by a line chart. The assessment of the intra- and inter-observer reproducibility of CT-derived ECVs were performed by the interclass correlation coefficient (ICC). ICC was calculated for evaluation of interobserver agreement for the ECV (ICC =0.00–0.20, poor correlation; ICC =0.21–0.40, fair correlation; ICC =0.41–0.60, moderate correlation; ICC =0.61–0.80, good correlation; ICC =0.81–1.00, excellent correlation). Bland-Altman analyses were utilized to evaluate data consistency. In all other tests, a 2-sided P value of <0.05 was considered to indicate a significant difference.
Results
Study population
After applying the exclusion criteria, 500 patients with malignant tumors were included in the study cohort. The regimen and dosage were adjusted according to clinical guidelines. Baseline patient characteristics are summarized in Table 1. Each patient received chest contrast-enhanced CT examination several times before the treatment of immunosuppressive drugs at the immune checkpoint (“baseline”) and after the first treatment of immunosuppressive drugs at the immune checkpoint. Before the first treatment, ECV, cTnT, and LVEF (ECV0, cTnT0, and LVEF0) were measured. During the treatment, ECV1, cTnT1, and LVEF1 were measured at 1–3 months (mean 56 days; IQR, 31–89 days); ECV2, cTnT2, and LVEF2 were measured at about 3–6 months (mean 145 days; IQR, 91–180 days); ECV3, cTnT3, and LVEF3 were measured at about 12 months (mean 353 days; IQR, 250–367 days) (Figure 1). The number of patients at the first, second, and third follow-up was 500, 467, and 361 respectively. The CTRCD (+) group represents the patients who showed CTRCD during the treatment of ICIs, and the CTRCD (−) group represents the patients who did not have CTRCD, including 451 patients in the CTRCD (−) group and 49 patients in the CTRCD (+) group. The age of the first CT examination of all patients was 60.98±8.46 years. The mean hematocrit before treatment was 39.27%±5.57%, whereas ECG or echocardiography showed no abnormality.
Table 1
Variable | Entire cohort (N=500) | CTRCD (−) (N=451) | CTRCD (+) (N=49) | P |
---|---|---|---|---|
Sex | 0.833 | |||
Male | 408 | 362 | 46 | |
Female | 92 | 89 | 3 | |
Age at cancer diagnosis, years | 60.98±8.46 | 61.00±8.66 | 60.80±6.40 | 0.871 |
Height, cm | 166.04±6.87 | 166.04±6.81 | 166.04±7.50 | 0.926 |
Weight, kg | 64.64±7.92 | 64.53±7.88 | 65.61±8.27 | 0.476 |
Heart rate, beats/min | 83.60±6.44 | 84.17±6.92 | 82.84±6.52 | 0.298 |
Systolic pressure, mmHg | 108.55±22.60 | 108.77±22.87 | 106.53±20.03 | 0.649 |
Diastolic pressure, mmHg | 76.98±10.55 | 77.10±10.61 | 75.92±10.07 | 0.463 |
Serum lipids | ||||
TG, mmol/L | 1.62±1.32 | 1.63±1.35 | 1.50±0.95 | 0.332 |
TC, mmol/L | 4.91±1.04 | 4.90±1.02 | 4.94±1.20 | 0.722 |
HDL, mmol/L | 1.57±0.38 | 1.58±0.38 | 1.51±0.35 | 0.061 |
LDL, mmol/L | 2.73±0.81 | 2.72±0.80 | 2.80±0.85 | 0.365 |
ALT, U/L | 25.21±23.44 | 25.07±23.18 | 26.67±26.06 | 0.510 |
AST, U/L | 27.97±29.23 | 27.66±29.23 | 31.21±29.16 | 0.241 |
FBG, mmol/L | 4.85±0.64 | 4.86±0.64 | 4.83±0.61 | 0.659 |
Histologic type | 0.565 | |||
Lung adenocarcinoma | 233 (46.60) | 214 (47.45) | 19 (38.78) | |
Squamous cell lung carcinoma | 128 (25.60) | 106 (23.50) | 22 (44.90) | |
Small cell lung carcinoma | 42 (8.40) | 41 (9.09) | 1 (2.04) | |
Other types of lung cancer | 12 (2.40) | 11 (2.44) | 1 (2.04) | |
Squamous cell esophageal carcinoma | 84 (16.80) | 78 (17.29) | 6 (12.24) | |
Other types of esophageal cancer | 1 (0.20) | 1 (0.20) | 0 (0) | |
Clinical stage | 0.833 | |||
III | 29 (5.80) | 29 (6.43) | 0 (0) | |
IV | 471 (94.20) | 422 (93.57) | 49 (100.00) | |
PD-L1 | 40 (8.00) | 39 (8.65) | 1 (2.04) | |
PD-1 | 459 (91.80) | 411 (91.13) | 48 (97.96) | |
CTLA-4 | 1 (0.20) | 1 (0.22) | 0 | |
LVEF by 2DE, % | 68.51±4.81 | 68.37±4.69 | 69.77±5.64 | 0.053 |
Data are presented as mean ± SD, N or n (%). P value between group CTRCD (−) and CTRCD (+). CTRCD (−), patients without CTRCD; CTRCD (+), patients with CTRCD; CTRCD, cancer therapy-related cardiac dysfunction; TG, triglyceride; TC, cholesterol; HDL, high-density lipoprotein; LDL, low density lipoprotein; ALT, alanine transaminase; AST, aspartate aminotransferase; FBG, fasting blood glucose; LVEF, left ventricular ejection fraction; 2DE, 2-dimensional echocardiography; SD, standard deviation.
Incidence rate and duration of CTRCD
The mean baseline LVEF of 500 patients with malignant tumors was 68.51%±4.81%. None of the participants had an LVEF lower than 55% as measured by 2DE before treatment. During ICIs treatment and follow-up, 49 patients (9.80%) developed CTRCD. However, during baseline 2DE, there was no statistically significant difference in mean LVEF between the CTRCD (+) and CTRCD (−) groups (68.37%±4.69% vs. 69.77%±5.64%, P=0.053). Among 49 patients with CTRCD during or after ICIs treatment, 2 (4.08%) were diagnosed at 1–3 months, 6 (12.24%) at 3–6 months, and 41 (83.67%) around 12 months. When meeting the CTRCD criteria, the mean 2DE LVEF in the CTRCD (+) group was 63.84%±4.78%.
Changes of cardiac cTnT with time in all patients
A total of 500 patients underwent the second cTnT test during the treatment follow-up. The average cTnT1 measurement was significantly higher than the baseline (cTnT1: N1=500, P<0.001). Among the total of 467 patients who received a third cTnT test about 145 days from the baseline, cTnT2 was significantly higher than cTnT1 and cTnT0 (mean cTnT2: 12.24±13.59, N2=467, P<0.001). Among the total of 361 patients received a fourth cTnT3 follow-up measurement, it was observed that cTnT3 was significantly higher than cTnT0, cTnT1, and cTnT2 (cTnT3: 14.54±14.49, N3=361, P<0.001). The change of cTnT with time in all patients is shown in Table 2.
Table 2
Test | Baseline (N0=500) |
1–3 months (N1=500) | 3–6 months (N2=467) | About 12 months (N3=361) | Pa | Pb | Pc |
---|---|---|---|---|---|---|---|
LVEF, % | 68.51±4.81 | 68.77±4.30 | 68.16±3.59 | 66.23±4.20 | 0.095 | 0.062 | <0.001 |
cTnT, ng/L | 7.42±3.95 | 10.05±11.40 | 12.24±13.59 | 14.54±14.49 | <0.001 | <0.001 | <0.001 |
ECV, % | 47.14±7.48 | 50.85±6.79 | 53.44±6.96 | 52.64±7.58 | <0.001 | <0.001 | <0.001 |
Data are presented as mean ± SD. Pa: comparison between values at 1–3 months and baseline; Pb: comparison between values at 3–6 months and baseline; Pc: comparison of values at about 12 months with baseline. LVEF, left ventricular ejection fraction; cTnT, cardiac troponin T; ECV, extracellular volume; SD, standard deviation.
Changes of myocardial ECV with time in all patients
The mean baseline ECV of left ventricular myocardium was 47.14%±7.48% (ECV0: 47.14%±7.48%, N0=500) in the baseline CT examination of 500 patients before immunosuppressant treatment. A total of 500 patients received a second CT examination during treatment follow-up. The mean ECV1 was significantly higher than the baseline (ECV1: 50.85%±6.79%, N1=500, P<0.001). A total of 467 patients received a third CT scan ECV2, which was significantly higher than ECV1 and ECV0 (mean ECV2: 53.44%±6.96%, N2=467, P<0.001). A total of 361 patients received a fourth CT follow-up measurement. It was observed that ECV3 was significantly higher than ECV0 and ECV1 (ECV3: 52.64%±7.58%, N3=361, P<0.001). The changes of myocardial ECV with time in all patients are shown in Table 2. Examples of ECV measurements are shown in Figure 3.
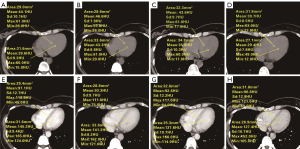
Change of cTnT with time in patients with CTRCD and patients without CTRCD
During the whole study period, it was found that the change of cTnT with time was different in patients with and without CTRCD, as shown in Table 3. Figure 4 displays the cTnT measurements of patients with and without CTRCD.
Table 3
Test | All | CTRCD (−) | CTRCD (+) | P1 | P2 | P3 |
---|---|---|---|---|---|---|
cTnT0, % | 7.42±3.95 | 7.33±3.99 | 8.22±3.58 | – | – | 0.134 |
cTnT1, % | 10.05±11.40 | 9.18±6.69 | 18.06±29.30 | <0.001 | <0.001 | <0.001 |
cTnT2, % | 12.24±13.59 | 10.24±7.01 | 29.34±32.15 | <0.001 | <0.001 | <0.001 |
cTnT3, % | 14.54±14.49 | 12.32±8.67 | 28.66±29.21 | <0.001 | <0.001 | <0.001 |
Data are presented as mean ± SD. P1: P-value of cTnT compared with the baseline in group CTRCD (−); P2: P value of cTnT compared with the baseline in group CTRCD (+); P3: P-value between group CTRCD (−) and CTRCD (+). CTRCD, cancer therapy-related cardiac dysfunction; CTRCD (−), patients without CTRCD; CTRCD (+), patients with CTRCD; cTnT, cardiac troponin T; SD, standard deviation.
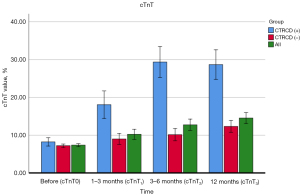
Changes of myocardial ECV with time in patients with and without CTRCD
During the whole study period, it was found that the changes of CT-ECV over time were different in patients with and without CTRCD, as shown in Table 4. ECV measurements in patients with and without CTRCD are shown in Figure 5.
Table 4
Test | All | CTRCD (−) | CTRCD (+) | P1 | P2 | P3 |
---|---|---|---|---|---|---|
ECV0, % | 47.14±7.48 | 47.10±7.48 | 47.54±7.52 | – | – | 0.698 |
ECV1, % | 50.85±6.79 | 50.65±6.72 | 52.75±7.15 | <0.001 | <0.001 | 0.039 |
ECV2, % | 53.44±6.96 | 53.22±6.98 | 55.37±6.56 | <0.001 | <0.001 | 0.041 |
ECV3, % | 52.64±7.58 | 52.24±7.43 | 55.14±8.11 | <0.001 | <0.001 | 0.013 |
Data are presented as mean ± SD. P1: P value of ECV compared with the baseline in group CTRCD (−); P2: P value of ECV compared with the baseline in group CTRCD (+); P3: P value between group CTRCD (−) and CTRCD (+). ECV, extracellular volume; CTRCD, cancer therapy-related cardiac dysfunction; CTRCD (−), patients without CTRCD; CTRCD (+), patients with CTRCD; SD, standard deviation.
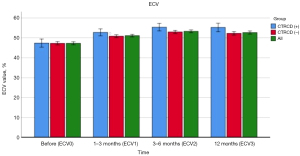
Changes in cTnT and ECV in all patients with time and in patients with CTRCD (+)
Changes in cTnT and ECV in all patients with time are shown in Figure 6; and changes in cTnT and ECV in patients with CTRCD (+) are shown in Figure 7.
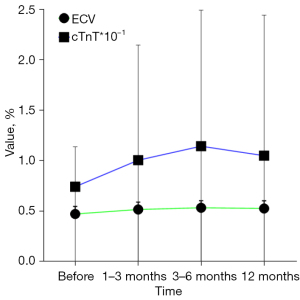
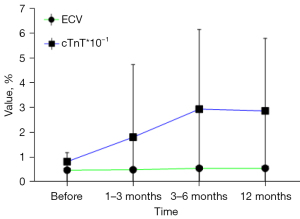
Reproducibility
A total of 79 patients were evaluated for reproducibility analysis of ECV0 before treatment. The consistency between and within observers was good, and the ICC ranged from 0.771 to 0.944.
Discussion
Our data on malignant tumor patients are relatively comprehensive: changes in LVEF, cTnT, and CT-ECV can be observed in the whole cycle of 1–3, 3–6, and about 12 months before and after ICIs treatment. Our results show that LVEF does not change significantly from 1–3 and 3–6 months after treatment compared with that before treatment, whereas CT-derived ECV can be as sensitive as cTnT, which changes significantly in the first 1–3 months after treatment. Our results are similar to the results of some previous studies (25,26), which support that ECV is more sensitive than LVEF in monitoring the cardiotoxicity induced by ICIs, and can be used as a biomarker for monitoring the early cardiotoxicity of ICIs, with higher imaging diagnostic value.
We observed no significant difference between the baseline of myocardial ECV and cTnT, and the change of LVEF was significantly higher than the baseline at any time after treatment in patients without CTRCD (−) and CTRCD (+). Considering that ICIs treatment may be different from other treatments, as long as ICIs treatment may cause myocardial damage, regardless of whether CTRCD (+) occurs later, this kind of damage can be recognized in the early 1–3 months after treatment, and the changes in ECV and cTnT values of CTRCD (+) patients are more severe than those of CTRCD (−) patients. Moreover, it can be seen that the changes in ECV seems to be more significant than cTnT in all patients, not just those with CTRCD. It is still necessary to further investigate whether the use of ECV alone is more suitable as a marker for myocardial damage than cTnT, or whether cTnT is suitable as an auxiliary marker for ECV diagnosis of myocardial damage. Since this was a retrospective study, no cardiac pathological results were obtained from patients with CTRCD (+). Although biopsy can be performed in future prospective studies, it is more beneficial to guide clinical work if the cut-off value of CT-ECV as an imaging biomarker of cardiac toxicity can be obtained.
It is worth noting that in this study, we noticed a decrease in ECV3 at the third follow-up compared with ECV2 at the second follow-up. An animal model experiment reported that in addition to fibrosis, degenerative myocardial changes, vacuoles, and interstitial edema also contribute to the increase of early ECV (27,28), indicating that myocardial injury precedes the development of fibrosis. Therefore, the lower ECV3 compared with ECV2 may be caused by the regression of interstitial edema in our study. Therefore, ECV may reflect sub-clinical myocardial injury induced by ICIs before myocardial fibrosis and LVEF decrease.
This study had some limitations. First, this was a single center study, although its sample size is acceptable. Several patients were excluded because of the lack of hematocrit or respiratory artifacts that significantly affected the measurement of ECV, or because they did not receive baseline examinations in our institution. Second, our research comprised a longitudinal observational experiment in which ECV of left ventricular septum and cTnT are measured at various time points; obviously, a longer observation period would yield more information. Third, owing to the retrospective nature of the study, some patients were lost at each follow-up time point. Fourth, we included patients with lung cancer and esophageal cancer, and did not conduct CT-ECV subgroup analysis on the cancer types. It could not be determined whether the changes of CT-ECV were consistent in different cancer types and different pathological types; further large sample size analysis is needed. Fifth, in this retrospective study, where a combination of chemotherapy drugs was used in conjunction with immunotherapy, it is inevitable that chemotherapy drugs may have had an impact on the heart. However, as the chemotherapy drugs we use in clinical practice do not contain drugs with irreversible cardiac toxicity (e.g., anthracycline, cyclophosphamide), they have a reversible impact on heart and cannot have a significant impact. Lastly, it is critical to note that the ECV in our study was measured using thoracic contrast-enhanced CT, including cardiac images, rather than specific cardiac CT. However, a study has confirmed the possibility of contrast-enhanced CT in measuring left ventricular ECV (29). In our study, images were acquired from portal phase, and the principle of images acquisition from portal phase has been addressed in various articles (16). It is true that there is a strong correlation between the values measured on pulmonary vein scans and those obtained from late scans, however usually a later scan is preferred. Since this was a retrospective study, no cardiac pathological results were obtained from patients with CTRCD; cardiac biopsy could be performed in a future prospective study. Despite these limitations, the myocardial ECV measured in our study is consistent with the previously reported myocardial ECV (30), indicating that ECV obtained by thoracic contrast-enhanced CT is feasible.
Conclusions
CT-derived ECV can be used as an imaging biomarker to monitor the cardiotoxicity of ICIs in tumor patients. In addition, changes in ECV may help to identify patients with cardiotoxicity of ICIs before significant reduction in LVEF, thus allowing treatment adjustment and consideration of other treatment options.
Acknowledgments
Funding: This work was supported by
Footnote
Reporting Checklist: The authors have completed the MDAR reporting checklist. Available at https://qims.amegroups.com/article/view/10.21037/qims-23-850/rc
Conflicts of Interest: All authors have completed the ICMJE uniform disclosure form (available at https://qims.amegroups.com/article/view/10.21037/qims-23-850/coif). The authors have no conflicts of interest to declare.
Ethical Statement: The authors are accountable for all aspects of the work in ensuring that questions related to the accuracy or integrity of any part of the work are appropriately investigated and resolved. This study was approved by the Ethics Committee of Chongqing University Cancer Hospital and has been granted exemption from consent due to the retrospective nature of this study. The study was conducted in accordance with the Declaration of Helsinki (as revised in 2013).
Open Access Statement: This is an Open Access article distributed in accordance with the Creative Commons Attribution-NonCommercial-NoDerivs 4.0 International License (CC BY-NC-ND 4.0), which permits the non-commercial replication and distribution of the article with the strict proviso that no changes or edits are made and the original work is properly cited (including links to both the formal publication through the relevant DOI and the license). See: https://creativecommons.org/licenses/by-nc-nd/4.0/.
References
- Reck M, Remon J, Hellmann MD. First-Line Immunotherapy for Non-Small-Cell Lung Cancer. J Clin Oncol 2022;40:586-97. [Crossref] [PubMed]
- Garassino MC, Mazieres J, Reck M, Chouaid C, Bischoff H, Reinmuth N, Cove-Smith L, Mansy T, Cortinovis D, Migliorino MR, Delmonte A, Sánchez JG, Chara Velarde LE, Bernabe R, Paz-Ares L, Perez ID, Trunova N, Foroutanpour K, Faivre-Finn C. Durvalumab After Sequential Chemoradiotherapy in Stage III, Unresectable NSCLC: The Phase 2 PACIFIC-6 Trial. J Thorac Oncol 2022;17:1415-27. [Crossref] [PubMed]
- Spallarossa P, Meliota G, Brunelli C, Arboscello E, Ameri P, Dessalvi CC, Grossi F, Deidda M, Mele D, Sarocchi M, Bellodi A, Madonna R, Mercuro G. Potential cardiac risk of immune-checkpoint blockade as anticancer treatment: What we know, what we do not know, and what we can do to prevent adverse effects. Med Res Rev 2018;38:1447-68. [Crossref] [PubMed]
- Johnson DB, Balko JM, Compton ML, Chalkias S, Gorham J, Xu Y, et al. Fulminant Myocarditis with Combination Immune Checkpoint Blockade. N Engl J Med 2016;375:1749-55. [Crossref] [PubMed]
- Mahmood SS, Fradley MG, Cohen JV, Nohria A, Reynolds KL, Heinzerling LM, Sullivan RJ, Damrongwatanasuk R, Chen CL, Gupta D, Kirchberger MC, Awadalla M, Hassan MZO, Moslehi JJ, Shah SP, Ganatra S, Thavendiranathan P, Lawrence DP, Groarke JD, Neilan TG. Myocarditis in Patients Treated With Immune Checkpoint Inhibitors. J Am Coll Cardiol 2018;71:1755-64. [Crossref] [PubMed]
- Salem JE, Manouchehri A, Moey M, Lebrun-Vignes B, Bastarache L, Pariente A, Gobert A, Spano JP, Balko JM, Bonaca MP, Roden DM, Johnson DB, Moslehi JJ. Cardiovascular toxicities associated with immune checkpoint inhibitors: an observational, retrospective, pharmacovigilance study. Lancet Oncol 2018;19:1579-89. [Crossref] [PubMed]
- Shalata W, Abu-Salman A, Steckbeck R, Mathew Jacob B, Massalha I, Yakobson A. Cardiac Toxicity Associated with Immune Checkpoint Inhibitors: A Systematic Review. Cancers (Basel) 2021.
- Haaf P, Garg P, Messroghli DR, Broadbent DA, Greenwood JP, Plein S. Cardiac T1 Mapping and Extracellular Volume (ECV) in clinical practice: a comprehensive review. J Cardiovasc Magn Reson 2016;18:89. [Crossref] [PubMed]
- Neilan TG, Rothenberg ML, Amiri-Kordestani L, Sullivan RJ, Steingart RM, Gregory W, Hariharan S, Hammad TA, Lindenfeld J, Murphy MJ, Moslehi JJCheckpoint Inhibitor Safety Working Group. Myocarditis Associated with Immune Checkpoint Inhibitors: An Expert Consensus on Data Gaps and a Call to Action. Oncologist 2018;23:874-8. [Crossref] [PubMed]
- Esposito R, Fedele T, Orefice S, Cuomo V, Prastaro M, Canonico ME, Ilardi F, De Stefano F, Fiorillo L, Santoro C, Esposito G. An Emergent Form of Cardiotoxicity: Acute Myocarditis Induced by Immune Checkpoint Inhibitors. Biomolecules 2021.
- Plana JC, Galderisi M, Barac A, Ewer MS, Ky B, Scherrer-Crosbie M, et al. Expert consensus for multimodality imaging evaluation of adult patients during and after cancer therapy: a report from the American Society of Echocardiography and the European Association of Cardiovascular Imaging. J Am Soc Echocardiogr 2014;27:911-39. [Crossref] [PubMed]
- Neilan TG, Coelho-Filho OR, Shah RV, Feng JH, Pena-Herrera D, Mandry D, Pierre-Mongeon F, Heydari B, Francis SA, Moslehi J, Kwong RY, Jerosch-Herold M. Myocardial extracellular volume by cardiac magnetic resonance imaging in patients treated with anthracycline-based chemotherapy. Am J Cardiol 2013;111:717-22.
- Tham EB, Haykowsky MJ, Chow K, Spavor M, Kaneko S, Khoo NS, Pagano JJ, Mackie AS, Thompson RB. Diffuse myocardial fibrosis by T1-mapping in children with subclinical anthracycline cardiotoxicity: relationship to exercise capacity, cumulative dose and remodeling. J Cardiovasc Magn Reson 2013;15:48. [Crossref] [PubMed]
- Cannaò PM, Altabella L, Petrini M, Alì M, Secchi F, Sardanelli F. Novel cardiac magnetic resonance biomarkers: native T1 and extracellular volume myocardial mapping. Eur Heart J Suppl 2016;18:E64-71. [Crossref] [PubMed]
- Messroghli DR, Walters K, Plein S, Sparrow P, Friedrich MG, Ridgway JP, Sivananthan MU. Myocardial T1 mapping: application to patients with acute and chronic myocardial infarction. Magn Reson Med 2007;58:34-40. [Crossref] [PubMed]
- Tu C, Shen H, Liu R, Wang X, Li X, Yuan X, Chen Q, Wang Y, Ran Z, Lan X, Zhang X, Lin M, Zhang J. Myocardial extracellular volume derived from contrast-enhanced chest computed tomography for longitudinal evaluation of cardiotoxicity in patients with breast cancer treated with anthracyclines. Insights Imaging 2022;13:85. [Crossref] [PubMed]
- Cannizzaro MT, Inserra MC, Passaniti G, Celona A, D'Angelo T, Romeo P, Basile A. Role of advanced cardiovascular imaging in chemotherapy-induced cardiotoxicity. Heliyon 2023;9:e15226. [Crossref] [PubMed]
- Deux JF, Nouri R, Tacher V, Zaroui A, Derbel H, Sifaoui I, Chevance V, Ridouani F, Galat A, Kharoubi M, Oghina S, Guendouz S, Audureau E, Teiger E, Kobeiter H, Damy T. Diagnostic Value of Extracellular Volume Quantification and Myocardial Perfusion Analysis at CT in Cardiac Amyloidosis. Radiology 2021;300:326-35. [Crossref] [PubMed]
- Bandula S, White SK, Flett AS, Lawrence D, Pugliese F, Ashworth MT, Punwani S, Taylor SA, Moon JC. Measurement of myocardial extracellular volume fraction by using equilibrium contrast-enhanced CT: validation against histologic findings. Radiology 2013;269:396-403. [Crossref] [PubMed]
- Nacif MS, Kawel N, Lee JJ, Chen X, Yao J, Zavodni A, Sibley CT, Lima JA, Liu S, Bluemke DA. Interstitial myocardial fibrosis assessed as extracellular volume fraction with low-radiation-dose cardiac CT. Radiology 2012;264:876-83. [Crossref] [PubMed]
- Liu P, Lin L, Xu C, Han Y, Lin X, Hou Y, Lu X, Vembar M, Jin Z, Wang Y. Quantitative analysis of late iodine enhancement using dual-layer spectral detector computed tomography: comparison with magnetic resonance imaging. Quant Imaging Med Surg 2022;12:310-20. [Crossref] [PubMed]
- Mergen V, Sartoretti T, Klotz E, Schmidt B, Jungblut L, Higashigaito K, Manka R, Euler A, Kasel M, Eberhard M, Alkadhi H. Extracellular Volume Quantification With Cardiac Late Enhancement Scanning Using Dual-Source Photon-Counting Detector CT. Invest Radiol 2022;57:406-11. [Crossref] [PubMed]
- Monti CB, Zanardo M, Bosetti T, Alì M, De Benedictis E, Luporini A, Secchi F, Sardanelli F. Assessment of myocardial extracellular volume on body computed tomography in breast cancer patients treated with anthracyclines. Quant Imaging Med Surg 2020;10:934-44.
- Miller CA, Naish JH, Bishop P, Coutts G, Clark D, Zhao S, Ray SG, Yonan N, Williams SG, Flett AS, Moon JC, Greiser A, Parker GJ, Schmitt M. Comprehensive validation of cardiovascular magnetic resonance techniques for the assessment of myocardial extracellular volume. Circ Cardiovasc Imaging 2013;6:373-83. [Crossref] [PubMed]
- Hong YJ, Park HS, Park JK, Han K, Park CH, Kim TK, Yoo SJ, Lee JY, Kim PK, Hur J, Lee HJ, Kim YJ, Suh YJ, Paek MY, Choi BW. Early Detection and Serial Monitoring of Anthracycline-Induced Cardiotoxicity Using T1-mapping Cardiac Magnetic Resonance Imaging: An Animal Study. Sci Rep 2017;7:2663. [Crossref] [PubMed]
- Si-Mohamed SA, Restier LM, Branchu A, Boccalini S, Congi A, Ziegler A, Tomasevic D, Bochaton T, Boussel L, Douek PC. Diagnostic Performance of Extracellular Volume Quantified by Dual-Layer Dual-Energy CT for Detection of Acute Myocarditis. J Clin Med 2021;10:3286. [Crossref] [PubMed]
- Dudnakova TV, Lakomkin VL, Tsyplenkova VG, Shekhonin BV, Shirinsky VP, Kapelko VI. Alterations in myocardial cytoskeletal and regulatory protein expression following a single Doxorubicin injection. J Cardiovasc Pharmacol 2003;41:788-94. [Crossref] [PubMed]
- Galán-Arriola C, Lobo M, Vílchez-Tschischke JP, López GJ, de Molina-Iracheta A, Pérez-Martínez C, Agüero J, Fernández-Jiménez R, Martín-García A, Oliver E, Villena-Gutierrez R, Pizarro G, Sánchez PL, Fuster V, Sánchez-González J, Ibanez B. Serial Magnetic Resonance Imaging to Identify Early Stages of Anthracycline-Induced Cardiotoxicity. J Am Coll Cardiol 2019;73:779-91. [Crossref] [PubMed]
- Capra D, Monti CB, Luporini AG, Lombardi F, Gumina C, Sironi A, Asti ELG, Bonavina L, Secchi F, Sardanelli F. Computed tomography-derived myocardial extracellular volume: an early biomarker of cardiotoxicity in esophageal cancer patients undergoing radiation therapy. Insights Imaging 2020;11:120. [Crossref] [PubMed]
- Kurita Y, Kitagawa K, Kurobe Y, Nakamori S, Nakajima H, Dohi K, Ito M, Sakuma H. Estimation of myocardial extracellular volume fraction with cardiac CT in subjects without clinical coronary artery disease: A feasibility study. J Cardiovasc Comput Tomogr 2016;10:237-41. [Crossref] [PubMed]