Coronary Artery Tree Description and Lesion Evaluation (CatLet) score for functional evaluation of coronary stenosis: a comparison study with pressure wire fractional flow reserve
Introduction
For patients with coronary artery disease, the presence and extent of reversible myocardial ischemia are the two most important parameters for complaints and prognosis (1-3). Fractional flow reserve (FFR), including its derivatives, such as quantitative flow ratio (QFR), computational pressure flow-derived FFR (caFFR), coronary angiography-derived FFR (FFRangio), and computed tomography-derived FFR (FFRCT), is currently the most widely used metric for identifying functional coronary artery stenosis causing myocardial ischemia (4-6). Guidelines (class I recommendation, level A evidence) support the use of FFR in guiding coronary intervention (7,8). Studies have shown that FFR is not only related to the degree of coronary stenosis but also to the blood supply territory of the affected coronary artery: the larger the supply territory is, the lower the FFR value measured from the diseased coronary artery (9,10). The limitations of using these methods, however, include the side effects of hyperemia-induced agents, additional costs, and vulnerability to microvascular resistance (11). The Coronary Artery Tree Description and Lesion Evaluation System (CatLet) angiographic scoring system (also known as Hexu) was independently developed by our research team based on the 17-segment myocardial model, the rule of competitive blood supply, and the law of flow conservation (12,13). The unique aspects of the CatLet angiographic scoring system include its abilities (I) to semiquantify the blood supply territory based solely on coronary angiography, (II) to account for coronary variations, and (III) to account for coronary stenosis. This angiographic scoring system can reasonably predict the prognosis of patients with acute myocardial infarction with high reproducibility (weighted κ value >0.8) (14) by incorporating both the degree of coronary stenosis and the subtended relative myocardial mass by the diseased coronary artery (14-18). Since the CatLet score is the product of the degree of coronary stenosis and the weighting of the affected coronary artery (equivalent to blood supply territory or relative myocardial mass), we have reason to speculate that the CatLet score can also be used for evaluation of functional coronary stenosis. We present this article in accordance with the STARD reporting checklist (available at https://qims.amegroups.com/article/view/10.21037/qims-23-1635/rc).
Methods
Study population
A total of 175 consecutive inpatients (206 target vessels) diagnosed with stable coronary artery disease admitted to the Department of Cardiology, Sichuan Science City Hospital, between December 2017 and November 2021 were retrospectively enrolled. The target vessels in this study were defined as coronary arteries with visually estimated diameter stenosis rates ≥50% and <90% (de novo lesions) on coronary angiography. The exclusion criteria included the following: (I) an FFR examination that could increase the occurrence of events, (II) patients with adenosine allergy or intolerance, (III) chronic total occlusion of coronary arteries, (IV) the presence of collateral coronary arteries, and (V) diseased coronary arteries with a diameter <2 mm.
This study complied with the Declaration of Helsinki (as revised in 2013) regarding studies on humans and was approved by the Institutional Review Board of Sichuan Science City Hospital. Written informed consent was obtained from all participants. This CatLet score-FFR study was registered on the Chinese Clinical Trial Registry (unique identifier: ChiCTR2300068351; http://www.chictr.org.cn).
Baseline data collection
The baseline information of the patients (including name, gender, age, medical history, personal history, family history, etc.), laboratory tests (including creatinine levels, total cholesterol, triglycerides, low-density lipoprotein cholesterol, etc.), electrocardiography on admission, blood pressure readings, initial results of cardiac ultrasonography, coronary angiography, and FFR values were collected and recorded in an Excel spreadsheet (Microsoft Corp.).
FFR measurement
FFR was measured according to standard protocols (19). The in vitro calibration of the pressure wire system was performed carefully for the coronary pressure–measuring system (PressureWire Certus FFR Measurement System, Abbott Laboratories). After the pressure sensor was advanced and positioned at 1 or 2 mm distal to the tip of the guiding catheter, the “Y” valve was closed and the pressure wire was flushed with normal saline. Under fluoroscopic guidance, the position of the pressure sensor was confirmed. The average pressure difference between the measured pressures by the pressure wire [pressure distal to the stenosis (Pd)] and the measured aortic pressure [(Pa)] by the guiding catheter was within ±5 mmHg and was equalized electronically. The pressure sensor was then advanced into the coronary artery and placed 2–3 cm distal to the stenosis to be assessed. The pressure (Pd) was measured at the maximal hyperemia. The FFR value was calculated as the ratio of the mean Pd to the mean Pa, which were measured simultaneously by the guiding catheter during the maximal hyperemia. After the pressure sensor was retracted to 1–2 mm distal to the tip of guiding catheter under steady maximal hyperemia, the two pressure readings were required to be identical to ensure no significant pressure drift. Maximal hyperemia was achieved by continuous intravenous infusion of adenosine triphosphate at a rate of 140–180 µg/kg/min (20). An FFR value ≤0.80 was defined as “positive”, indicating the presence of coronary artery stenosis causing reversible myocardial ischemia. An FFR value >0.80 was defined as “negative”, indicating the absence of coronary artery stenosis causing reversible myocardial ischemia (21).
Calculation of CatLet score
The principles of design and application of the CatLet angiographic scoring system have been fully described elsewhere (12,13). Its tutorial and calculator are available online (http://www.catletscore.com), and its features and calculations are briefly described in the following sections.
Seventeen-segment myocardial model
The left ventricular myocardium is evenly divided into approximately 17 segments according to the 17-segment myocardial model (22). Studies have shown that myocardial mass is proportional to actual myocardial blood flow (2). Therefore, each myocardial segment can be treated as a unit of actual myocardial blood flow. The importance of a coronary artery depends on the number of myocardial segments it supplies, regardless of its nomenclature.
Explanation of coronary anatomic variations via competitive blood supply
Coronary anatomic variations can be explained by the competitive blood supply in the coronary arteries. The left anterior descending artery (LAD) usually runs in the anterior interventricular groove, competing with the posterior descending artery (PDA), which runs in the posterior interventricular groove. A longer LAD is often associated with a shorter PDA and vice versa. The remaining two pairs of competing vessels include the diagonal branches (Dxs) competing with the obtuse marginals and the posterior lateral vessels (PLVs) originating either from the right coronary artery (RCA) or from the left circumflex (LCX) competing with each other. Understanding the sizes of the LAD, Dxs, and PLVs derived from the RCA allows for the determination of the sizes of their mirror vessels.
Semi-quantification of coronary blood supply territory
A semiquantitative description of coronary supply territory requires a reference and a preset discrete value. According to the American Heart Association’s recommendations, the 17 myocardial segments are assigned to the 3 main epicardial coronary arteries as follows: the LAD supplies 7 segments, the LCX supplies 5 segments, and the RCA supplies 5 segments. Therefore, the weight (importance or blood supply territory) of LAD, LCX, and RCA is 7.0, 5.0, and 5.0, respectively. In the CatLet angiographic scoring system, this myocardial segment assignment is used as reference. Through careful observation of coronary arteries and their branches, this myocardial segment assignment can be further subdivided into the LAD excluding the Dx, supplying 4 segments (anterior septum and apical segments); the Dx supplying 3 segments (anterior wall); the PDA of the RCA supplying 2 segments in the posterior septum; and the PLVs derived from the RCA supplying 3 myocardial segments in the inferior wall. For the LAD and the Dxs, the preset discrete value is 1.0, while that of the PLVs is 1.5. With knowledge of the reference and the preset discrete values, the sizes of the LAD, Dxs, and RCA can be redefined. Based on these three key parameters, the coronary artery type can be uniquely determined for each individual. In the CatLet angiographic scoring system, the definition of coronary artery type does not require knowledge of the LCX since the Dxs and PLVs off the RCA are the mirror vessels for the obtuse marginal and PLVs, respectively, both arising from the LCX.
In the CatLet angiographic scoring system, the LAD is classified into 3 types based on the number of supplied segments: long (5 segments), average (4 segments), and short LAD (3 segments); the Dxs are classified into 3 types: large (4 segments), intermediate (3 segments), and small (2 segments); the RCA is classified into 6 types based on the number of supplied segments: the zero-PDA type (the RCA does not supply blood to the left ventricle), the only-PDA type (the RCA only supplies the posterior interventricular septum), the small-RCA type (the RCA supplies an additional 1.5 inferior wall segments), the average-RCA type (the RCA supplies an additional three segments), the large-RCA type (the RCA supplies an additional 4.5 segments), and the super-RCA type (RCA supplies an additional 6 segments). Therefore, a total of 3×3×6=54 coronary artery types can be obtained. For each coronary artery type, the corresponding weighting factor is allocated based on the law of flow conservation, representing the blood supply territory of that vessel.
Coronary artery blood supply territory and CatLet score
Assuming a certain coronary circulation type with an average LAD and an intermediate Dx, the PLVs derived either from the RCA or the LCX compete to supply the inferior wall, inferolateral wall, and even anterolateral wall of the left ventricle. The only-PDA type of the RCA supplies only the posterior septum, which includes two myocardial segments (Figure 1A and Figure 1a). The weighting difference between the only-PDA type of the RCA and the-average RCA type (weighting factor of 5.0) as a reference (Figure 1B and Figure 1b) is 3.0, and so the weighting factor for the only-PDA type of the RCA is 2.0. If this only-PDA type of the RCA had a nonocclusive stenosis with a diameter narrowing rate of ≥50%it would receive a score of 2×2.0=4.0 according to the CatLet scoring algorithm. Similarly, with the average RCA serving as the reference (Figure 1B and Figure 1b), the super RCA supplies an additional 6 myocardial segments. Therefore, the super RCA supplies a total of 8 myocardial segments, corresponding to a weighting factor of 8.0 (Figure 1C and Figure 1c). If this RCA had a nonocclusive stenosis with a diameter narrowing rate of ≥50%, it would receive a score of 2×8.0=16 according to the CatLet scoring algorithm. In this study, only nonocclusive lesions with a diameter narrowing rate of ≥50% and vessel diameter ≥2.0 mm were scored. The degree of lesion stenosis was assessed visually. All scoring was performed by two interventional cardiologists (L.L.W. and G.Z.Z.) who had 5 years of working experience and who were fully knowledgeable about the CatLet angiographic scoring system. In case of disagreement between the two physicians’ scores, a third interventional cardiologist (Y.M.H.) was consulted for a joint decision. The scoring was completed blindly without knowledge of the FFR results. The average time interval between the scoring and the FFR measurement was more than 2 years. According to our previous study, the time required to complete the evaluation of complex (multi-vessel) coronary artery disease is about 5–10 min (12). For a diseased coronary artery, an intensively 4-week-trained interventionist can complete the scoring within 3–5 min. Figure 2 and Figures S1-S4 present a typical case with the CatLet score and FFR values.
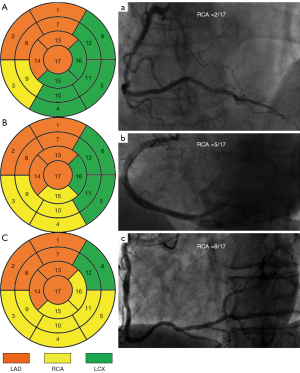
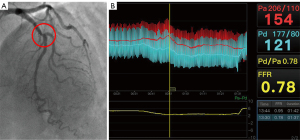
Statistical analysis
All data analysis was performed using Stata 17.0 (StataCorp). Categorical variables are presented as frequencies and percentages, and continuous variables were assessed for normality using the Kolmogorov-Smirnov test. Normally distributed continuous variables are expressed as the mean ± standard deviation, while nonnormally distributed continuous variables are expressed as the median and interquartile range (IQR). The correlation between FFR values and the CatLet score was quantified using Pearson correlation coefficient and is reported with r values. The sensitivity, specificity, and diagnostic accuracy of the CatLet score in predicting FFR ≤0.80 were calculated. The optimal diagnostic cutoff point for the CatLet score was determined using receiver operating characteristic (ROC) curve analysis based on the Youden index. Logistic regression analysis was performed to evaluate the accuracy of the CatLet score in predicting FFR ≤0.80 with different clinical features. Baseline data were collected in routine fashion for clinical practice on a daily basis, and no missing data were generated. A two-sided P value <0.05 was considered statistically significant.
Results
Baseline characteristics
Among the 260 patients, 85 were excluded for not meeting the inclusion criteria, resulting in a final inclusion of 175 patients, as shown in Figure 3. The average age was 71 years. Of the 175 patients, 74.29% were aged ≥65 years old, 70.29% were male, 64.57% had hypertension, 38.86% had a smoking history, 29.71% had a history of alcohol consumption, and 37.14% had diabetes. The median FFR value was 0.85 (IQR 0.78–0.90), and the median CatLet score was 10 (IQR 6–13). With FFR ≤0.8 as a reference, there were 66 positive target vessels and 140 negative target vessels. See Table 1 for more details.
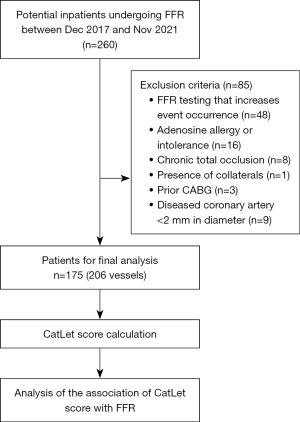
Table 1
Characteristic | Value (N=175) |
---|---|
Age, years | 71.44±10.7 |
Male sex | 123 (70.29) |
Hypertension | 113 (64.57) |
T2DM | 65 (37.14) |
Smoking | 68 (38.86) |
Drinking | 52 (29.71) |
Total cholesterol, mmol/L | 4.27±1.15 |
Triglycerides, mmol/L | 1.79±1.64 |
Lipoprotein(a), mg/L | 164.69±153.89 |
HDL-C, mmol/L | 1.29±0.42 |
LDL-C, mmol/L | 2.44±0.83 |
Cr, µmmol/L | 75.32±22.50 |
LVEF, % | 66.65±6.53 |
Body mass index, kg/m2 | 24.58±4.48 |
Diagonal size | |
Small | 8 (4.57) |
Inter. | 94 (53.71) |
Large | 73 (41.71 |
LAD length | |
Short | 11 (6.29) |
Average | 112 (64.00) |
Long | 52 (29.71) |
RCA dominance | |
PDA zero | 5 (2.86) |
PDA-only | 5 (2.86) |
Small RCA | 20 (11.43) |
Average RCA | 77 (44.00) |
Large RCA | 52 (29.71) |
Super RCA | 16 (9.14) |
Lesion number/pts | 1.61±0.98 |
Calcification | 47 (26.86) |
Calcification number/pts | 0.35±0.66 |
One-vessel disease | 125 (71.43) |
Two-vessel disease | 39 (22.29) |
Three-vessel disease | 11 (6.29) |
Bifurcation number/pts | 0.75±0.67 |
Medina 0,0,1 | 15 (8.57) |
Medina 0,1,0 | 45 (25.71) |
Medina 0,1,1 | 16 (9.14) |
Medina 1,0,0 | 16 (9.14) |
Medina 1,0,1 | 2 (1.14) |
Medina 1,1,0 | 13 (7.43) |
Medina 1,1,1 | 20 (11.43) |
Trifurcation | 0 (0) |
Tortuosity | 45 (25.71) |
Angulation | 97 (55.43) |
Thrombus | 0 (0) |
Lesion length >20 mm | 63 (36.00) |
Treated coronary arteries | |
LAD | 37 (21.40) |
LCX | 8 (4.57) |
RCA | 7 (4.00) |
Diagonal branch size, LAD length, and RCA dominance were defined previously (13). Data are expressed as the mean ± SD or as n (%). T2DM, type 2 diabetes mellitus; HDL-C, high-density lipoprotein cholesterol; LDL-C, low-density lipoprotein cholesterol; Cr, creatinine; LVEF, left ventricular ejection fraction; Inter, intermediate; LAD, left anterior descending artery; RCA, right coronary artery; PDA, posterior descending artery; LCX, left circumflex; pts, patients; SD, standard deviation.
Association between CatLet score and FFR
An overall analysis of 206 coronary arteries from 175 patients is presented in Figure 4. The CatLet score showed a significant negative correlation with FFR (r=–0.61; 95% CI: –0.69 to –0.52; P<0.01), with the data points being scattered around the regression line. The CatLet score showed an overall good agreement with FFR, accurately identifying 158 out of 206 (77%) diseased vessels. However, the CatLet score missed 6 out of 206 (3%) lesions identified by FFR as having reversible ischemia and misdiagnosed 42 (20%) lesions identified by FFR as having no reversible ischemia. Figure 4 provides a detailed overview of the relationship between the CatLet score and FFR in different coronary arteries.
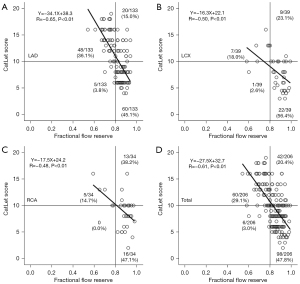
According to the Youden index, the optimal cutoff value of the CatLet score for predicting FFR ≤0.80 was determined to be 11. However, considering clinical practice, we defined the optimal cutoff value of the CatLet score as ≥10 to diagnose FFR ≤0.80. This cutoff value is more conservative in a clinical setting, with a sensitivity of 78.80% (95% CI: 67.00–87.90%) and a specificity of 85.00% (95% CI: 78.00–90.50%). The area under the ROC curve (AUC) was 0.90 (95% CI: 0.85–0.94), as shown in Table 2, which also includes the correlation between the CatLet score and FFR, as well as the sensitivity, specificity, negative likelihood ratio, and positive likelihood ratio for different coronary arteries.
Table 2
Category, n [%] | Sensitivity% (95% CI) |
Specificity% (95% CI) |
AUC (95% CI) | LR+ | LR− |
---|---|---|---|---|---|
Overall, 206 [100] | 78.80 (67.00–87.90) | 85.00 (78.00–90.50) | 0.90 (0.85–0.94) | 5.25 | 0.25 |
LAD, 133 [64] | 83.00 (70.20–91.90) | 78.80 (68.20–87.10) | 0.89 (0.83–0.94) | 3.91 | 0.22 |
LCX, 39 [19] | 62.50 (24.50–91.50) | 96.80 (83.30–99.90) | 0.91 (0.78–0.98) | 19.38 | 0.39 |
RCA, 34 [17] | 60.00 (14.70–94.70) | 89.70 (72.60–97.80) | 0.87 (0.71–0.96) | 5.80 | 0.45 |
CatLet, Coronary Artery Tree Description and Lesion Evaluation; FFR, fractional flow reserve; CI, confidence interval; AUC, area under the curve; LR+, positive likelihood ratio; LR−, negative likelihood ratio; LAD, left anterior descending artery; LCX, left circumflex; RCA, right coronary artery.
Subgroup analysis of a CatLet score ≥10 in predicting FFR ≤0.80
Table 3 provides a detailed subgroup analysis of the sensitivity, specificity, diagnostic accuracy, positive and negative likelihood ratios, and odds ratios for a CatLet score ≥10 in predicting FFR ≤0.80 based on different patient characteristics. The predictive ability of a CatLet score ≥10 for FFR ≤0.80 was generally consistent among different subgroups, with no significant interaction observed. However, there was an exception in the subgroup of patients with hypertension, among whom a CatLet score ≥10 had a significantly higher accuracy in predicting FFR ≤0.80 compared to those without hypertension.
Table 3
Characteristic | N [%] | Sensitivity % (95% CI) |
Specificity % (95% CI) |
AUC (95% CI) |
OR (95% CI) |
P value for interaction |
---|---|---|---|---|---|---|
Age, years | 0.14 | |||||
≥65 | 154 [75] | 82.61 (68.60–92.20) | 85.19 (77.10–91.30) | 0.92 (0.86–0.96) | 1.85 (1.53–2.21) | |
<65 | 52 [25] | 70.00 (45.70–88.10) | 84.37 (67.20–94.70) | 0.86 (0.74–0.94) | 1.50 (1.22–1.85) | |
Sex | 0.21 | |||||
Male | 149 [72] | 75.47 (61.70–86.20) | 86.46 (78.00–92.60) | 0.89 (0.83–0.93) | 1.66 (1.42–1.94) | |
Female | 57 [28] | 92.31 (64.00–99.80) | 81.82 (67.30–91.80) | 0.95 (0.86–0.99) | 2.32 (1.41–3.81) | |
Hypertension | 0.04 | |||||
Yes | 137 [67] | 83.33 (69.80–92.50) | 86.52 (77.60–92.80) | 0.93 (0.87–0.97) | 1.95 (1.57–2.43) | |
No | 69 [33] | 66.67 (41.00–86.70) | 82.35 (69.10–91.60) | 0.82 (0.71–0.90) | 1.45 (1.20–1.75) | |
Total cholesterol | 0.88 | |||||
Yes | 19 [9] | 69.23 (48.20–85.70) | 98.11 (89.90–100.00) | 0.91 (0.82–0.96) | 1.66 (1.07–2.58) | |
No | 187 [91] | 82.50 (67.20–92.70) | 85.06 (75.80–91.80) | 0.89 (0.82–0.94) | 1.72 (1.48–1.99) | |
Triglycerides | 0.27 | |||||
Yes | 77 [37] | 69.23 (48.20–85.70) | 98.11 (89.90–100.00) | 0.91 (0.82–0.96) | 1.57 (1.30–1.90) | |
No | 129 [63] | 82.50 (67.20–92.70) | 85.06 (75.80–91.80) | 0.89 (0.82–0.94) | 1.84 (1.50–2.26) | |
T2DM | 0.94 | |||||
Yes | 76 [37] | 84.00 (63.90–95.50) | 82.35 (69.10–91.60) | 0.90 (0.80–0.95) | 1.73 (1.37–2.17) | |
No | 130 [63] | 75.61 (59.70–87.60) | 86.52 (77.60–92.80) | 0.90 (0.84–0.95) | 1.71 (1.43–2.04) | |
Smoking | 0.91 | |||||
Yes | 85 [41] | 74.29 (56.70–87.50) | 84.00 (70.90–92.80) | 0.90 (0.81–0.95) | 1.73 (1.38–2.17) | |
No | 121 [59] | 83.87 (66.30–94.50) | 85.56 (76.60–92.10) | 0.91 (0.85–0.96) | 1.76 (1.45–2.15) | |
Body mass index, kg/m2 | 0.18 | |||||
≤25 | 125 [61] | 75.68 (58.80–88.20) | 80.68 (70.90–88.30) | 0.89 (0.83–0.94) | 1.62 (1.38–1.91) | |
>25 | 81 [39] | 82.76 (64.20–94.20) | 92.31 (81.50–97.90) | 0.92 (0.84–0.97) | 2.05 (1.51–2.79) |
CatLet, Coronary Artery Tree Description and Lesion Evaluation; FFR, fractional flow reserve; CI, confidence interval; AUC, area under the curve; OR, odds ratio; T2DM, type 2 diabetes mellitus.
Discussion
This study is the first to report that coronary stenosis in conjunction with its subtended supply territory solely based on coronary angiography without any complex fluid dynamics computation can help to identify reversible myocardial ischemia. It was found that a CatLet score ≥10 predicted FFR ≤0.80 with a good diagnostic performance, and the results showed an overall good correlation between CatLet score and FFR, with a sensitivity of 78.80% (95% CI: 67.00–87.90%), a specificity of 85.00% (95% CI: 78.00–90.50%), an AUC of 0.90 (95% CI: 0.85–0.94), a positive likelihood ratio of 5.25, and a negative likelihood ratio of 0.25. Subgroup analysis based on different coronary arteries and patient characteristics yielded similar results.
FFR not only depends on the degree of stenosis, but also on the blood supply territory of the diseased coronary artery. For the same degree of coronary stenosis, a larger blood supply territory leads to a lower FFR value and vice versa (9,10,23). The CatLet angiographic scoring system, designed based on the 17-segment myocardial model, the rule of competitive blood supply, and the law of flow conservation, can dynamically reflect coronary variations and their blood supply territories. The coronary weighting factor in the CatLet score represents the blood supply territory. The CatLet score is obtained by multiplying the stenosis degree by a fixed factor of 2.0 (a fixed coefficient of 5.0 for occlusive lesions) and the coronary weighting factor. Therefore, the calculation of CatLet score shares similar components with FFR values. This study found that CatLet Score ≥10 could predict FFR ≤0.80, verifying our hypothesis. A CatLet score ≥10 indicated that more than (inclusive) five segments (CatLet score divided by 2) were at ischemic risk. According to the 17-segment myocardial model, the RCA and LCX typically supply five segments, respectively, in the most commonly seen coronary circulation pattern, and will be treated if they are at ischemic risk in daily clinical practice. According to the Youden index in this study, the optimal cutoff value of the CatLet score for predicting FFR ≤0.80 was 11. Informed by clinical practice and statistical analysis, we chose a CatLet score of ≥10 as the cutoff to predict FFR ≤0.80. This cutoff is conservative and would lead to more patients with reversible ischemia receiving coronary intervention based on the results of coronary angiography.
In the per-vessel analysis with FFR ≤0.80 as the gold standard, a CatLet score≥10 missed 6 out of 206 (3%) positive lesions identified by FFR as having reversible ischemia and misdiagnosed 42 (20%) lesions identified by FFR as not having reversible ischemia. For the former results, the blood supply territory of the missed lesions was less than 5 segments (the number of segments equals the score divided by 2), thus having a relatively smaller impact on patients. For the latter results, misdiagnosed lesions had a blood supply territory greater than 5 segments and were often located in the proximal segments of the coronary arteries, where stenting would be conventionally indicated on coronary angiography. Therefore, stenting of these misdiagnosed lesions is less likely to pose a higher risk to patients. Detailed observation of the scatter plot revealed that most misclassified cases were distributed in the quadrant of a CatLet coronary score ≥10 and FFR >0.8. This discrepancy may be explained by the concept of the “gray zone” or “borderline” cases, in which subtle differences in diameter narrowing between 49% and 50% are difficult to distinguish in clinical practice. This slight difference in diameter narrowing leads to the “all” or “none” effects of CatLet Score. Analyzing the subgroups within different coronary arteries, we also observed this phenomenon in the LAD, LCX, and RCA. Subtle differences in diameter stenosis can account for this misclassification. The use of a more refined categorization of the degree of coronary stenosis, as is conducted in the Gensini score, may help to reduce the occurrence of misclassification (24).
The CatLet score calculation can be completed solely based on the results of coronary angiography and during the procedure within 3–5 min for a trained interventionist and without additional costs, which is in sharp contrast with the most prevalently used pressure wire FFR or its derivatives (25-28). A previous study showed that those with ≥3 abnormal myocardial segments had a reduced 6-year survival rate (29). In our study, a cutoff of ≥5 segments was used to predict abnormal FFR≤0.8 in the presence of ≥50% luminal narrowing. There are several possible explanations for this discrepancy. First, the cutoff of ≥5 segments was exploratory for this current study, and there was a small sample size. A larger ongoing study will provide a definitive cutoff for predicting myocardial ischemia as indicated by FFR. Second, the CatLet score, which assesses the degree and importance of the diseased coronary artery, is not completely equivalent to the FFR. The FFR is heavily influenced by factors such as the degree of stenosis, the affected myocardial area, microvascular resistances, and the presence or absence of collaterals. Finally, there may be certain intrinsic flaws or inaccuracies in the FFR method that also contributed to this discrepancy (30).
Some limitations to this study should be mentioned. First, as a single-center, retrospective design was used, the association between CatLet score and FFR was inevitably subject to confounding factors. Prospective, multicenter, randomized controlled trials would help to address this issue. Second, the CatLet score used in this study corrected the limitations of previous coronary anatomical scoring systems in reflecting coronary variations, but it also involved the erroneous classification of the degree of coronary stenosis into binary categories. Therefore, assigning different weights based on the degree of stenosis might improve this scoring system. Finally, this study only included patients with stable coronary disease, and therefore, the results cannot be extrapolated to other populations with coronary heart disease. Further research is needed to investigate the association between CatLet Score and FFR in different coronary artery disease populations.
Conclusions
CatLet score, solely based on visual estimation of the results of coronary angiography, had a good diagnostic performance with respect to myocardial ischemia. Its clinical values in guiding revascularization warrant further investigations.
Acknowledgments
Funding: This work was supported by the Sci-Tech Supporting Program of Jiangsu Commission of Health (grant No. M2021019 to Y.M.H.), and the Medical Sci-Tech Innovation Program for Medical Care of Suzhou City (grant No. SKY2021005 to Y.M.H.).
Footnote
Reporting Checklist: The authors have completed the STARD reporting checklist. Available at https://qims.amegroups.com/article/view/10.21037/qims-23-1635/rc
Conflicts of Interest: All authors have completed the ICMJE uniform disclosure form (available at https://qims.amegroups.com/article/view/10.21037/qims-23-1635/coif). Y.M.H. reports receiving the support from the Sci-Tech Supporting Program of Jiangsu Commission of Health (grant No. M2021019) and the Medical Sci-Tech Innovation Program for Medical Care of Suzhou City (grant No. SKY2021005). The other authors have no conflicts of interest to declare.
Ethical Statement: The authors are accountable for all aspects of the work in ensuring that questions related to the accuracy or integrity of any part of the work are appropriately investigated and resolved. The current study complied with the Declaration of Helsinki (as revised in 2013) regarding studies of humans and was approved by the Institutional Review Board of Sichuan Science City Hospital. Written informed consent was obtained from all participants.
Open Access Statement: This is an Open Access article distributed in accordance with the Creative Commons Attribution-NonCommercial-NoDerivs 4.0 International License (CC BY-NC-ND 4.0), which permits the non-commercial replication and distribution of the article with the strict proviso that no changes or edits are made and the original work is properly cited (including links to both the formal publication through the relevant DOI and the license). See: https://creativecommons.org/licenses/by-nc-nd/4.0/.
References
- Di Serafino L, Barbato E, Serino F, Svanerud J, Scalamogna M, Cirillo P, Petitto M, Esposito M, Silvestri T, Franzone A, Piccolo R, Esposito G. Myocardial mass affects diagnostic performance of non-hyperemic pressure-derived indexes in the assessment of coronary stenosis. Int J Cardiol 2023;370:84-9. [Crossref] [PubMed]
- Keulards DCJ, Fournier S, van 't Veer M, Colaiori I, Zelis JM, El Farissi M, Zimmermann FM, Collet C, De Bruyne B, Pijls NHJ. Computed tomographic myocardial mass compared with invasive myocardial perfusion measurement. Heart 2020;106:1489-94. [Crossref] [PubMed]
- Yang DH, Kang SJ, Koo HJ, Kweon J, Kang JW, Lim TH, Jung J, Kim N, Lee JG, Han S, Ahn JM, Park DW, Lee SW, Lee CW, Park SW, Park SJ, Mintz GS, Kim YH. Incremental Value of Subtended Myocardial Mass for Identifying FFR-Verified Ischemia Using Quantitative CT Angiography: Comparison With Quantitative Coronary Angiography and CT-FFR. JACC Cardiovasc Imaging 2019;12:707-17. [Crossref] [PubMed]
- Jiang J, Tang L, Du C, Leng X, He J, Hu Y, Dong L, Sun Y, Li C, Xiang J, Wang J. Diagnostic performance of AccuFFRangio in the functional assessment of coronary stenosis compared with pressure wire-derived fractional flow reserve. Quant Imaging Med Surg 2022;12:949-58. [Crossref] [PubMed]
- Dai X, Hou Y, Tang C, Lu Z, Shen C, Zhang L, Zhang J. Long-term prognostic value of the serial changes of CT-derived fractional flow reserve and perivascular fat attenuation index. Quant Imaging Med Surg 2022;12:752-65. [Crossref] [PubMed]
- Jeremias A, Kirtane AJ, Stone GW. A Test in Context: Fractional Flow Reserve: Accuracy, Prognostic Implications, and Limitations. J Am Coll Cardiol 2017;69:2748-58. [Crossref] [PubMed]
- Lawton JS, Tamis-Holland JE, Bangalore S, Bates ER, Beckie TM, et al. 2021 ACC/AHA/SCAI Guideline for Coronary Artery Revascularization: A Report of the American College of Cardiology/American Heart Association Joint Committee on Clinical Practice Guidelines. J Am Coll Cardiol 2022;79:e21-e129. [Crossref] [PubMed]
- Neumann FJ, Sousa-Uva M, Ahlsson A, Alfonso F, Banning AP, Benedetto U, Byrne RA, Collet JP, Falk V, Head SJ, Jüni P, Kastrati A, Koller A, Kristensen SD, Niebauer J, Richter DJ, Seferovic PM, Sibbing D, Stefanini GG, Windecker S, Yadav R, Zembala MOESC Scientific Document Group. 2018 ESC/EACTS Guidelines on myocardial revascularization. Eur Heart J 2019;40:87-165. [Crossref] [PubMed]
- Iqbal MB, Shah N, Khan M, Wallis W. Reduction in myocardial perfusion territory and its effect on the physiological severity of a coronary stenosis. Circ Cardiovasc Interv 2010;3:89-90. [Crossref] [PubMed]
- De Bruyne B, Sarma J. Fractional flow reserve: a review: invasive imaging. Heart 2008;94:949-59. [Crossref] [PubMed]
- Cerrato E, Mejía-Rentería H, Franzè A, Quadri G, Belliggiano D, Biscaglia S, Lo Savio L, Spataro F, Erriquez A, Giacobbe F, Vergara-Uzcategui C, di Girolamo D, Tebaldi M, Varbella F, Campo G, Escaned J. Quantitative flow ratio as a new tool for angiography-based physiological evaluation of coronary artery disease: a review. Future Cardiol 2021;17:1435-52. [Crossref] [PubMed]
- Xu MX, Teng RL, Ruddy TD, Schoenhagen P, Bartel T, Di Bartolomeo R, Aksoy O, Desai M, von Kodolitsch Y, Escaned J, McCullough PA, Vasudevan A, Shen CX, Zhao X, Zhou YF, Xu HF, Cheng XJ, He YM. The CatLet score: a new coronary angiographic scoring tool accommodating the variable coronary anatomy for the first time. J Thorac Dis 2019;11:5199-209. [Crossref] [PubMed]
- He YM, Masuda S, Gao C. Revisit of the CatLet (Hexu) angiographic scoring system: a narrative review. J Thorac Dis 2023;15:2848-58. [Crossref] [PubMed]
- Liu JM, He Y, Teng RL, Qian XD, Dai YL, Xu JP, Zhao X, Jiang TB, He YM. Inter- and intra-observer variability for the assessment of coronary artery tree description and lesion EvaluaTion (CatLet©) angiographic scoring system in patients with acute myocardial infarction. Chin Med J (Engl) 2020;134:425-30. [Crossref] [PubMed]
- Wang H, He Y, Fan JL, Li X, Zhou BY, Jiang TB, He YM. The predictive value of CatLet© angiographic scoring system for long-term prognosis in patients with acute myocardial infarction presenting > 12 h after symptom onset. Front Cardiovasc Med 2022;9:943229. [Crossref] [PubMed]
- Xu MX, Ruddy TD, Schoenhagen P, Bartel T, Di Bartolomeo R, von Kodolitsch Y, Escaned J, Shen C, He YM. The CatLet score and outcome prediction in acute myocardial infarction for patients undergoing primary percutaneous intervention: A proof-of-concept study. Catheter Cardiovasc Interv 2020;96:E220-9. [Crossref] [PubMed]
- Xu M, Wang S, Zhang Y, Zhang J, Ma J, Shen J, Tang Y, Jiang T, He Y. Residual coronary artery tree description and lesion EvaluaTion (CatLet) score, clinical variables, and their associations with outcome predictions in patients with acute myocardial infarction. Chin Med J (Engl) 2023;136:2459-67. [Crossref] [PubMed]
- Teng RL, Liu M, Sun BC, Xu JP, He Y, He YM. Age, Serum Creatinine, and Left Ventricular Ejection Fraction Improved the Performance of the CatLet Angiographic Scoring System in Terms of Outcome Predictions for Patients with Acute Myocardial Infarction: A Median 4.3-Year Follow-Up Study. Cardiology 2021;146:690-7. [Crossref] [PubMed]
- Toth GG, Johnson NP, Jeremias A, Pellicano M, Vranckx P, Fearon WF, Barbato E, Kern MJ, Pijls NH, De Bruyne B. Standardization of Fractional Flow Reserve Measurements. J Am Coll Cardiol 2016;68:742-53. [Crossref] [PubMed]
- Kubo T, Takahata M, Terada K, Mori K, Arita Y, Ino Y, Matsuo Y, Kitabata H, Shiono Y, Shimamura K, Kameyama T, Emori H, Katayama Y, Tanimoto T, Akasaka T. Retrospective Comparison of Long-Term Clinical Outcomes Between Percutaneous Coronary Intervention and Medical Therapy in Stable Coronary Artery Disease With Gray Zone Fractional Flow Reserve-COMFORTABLE Retrospective Study. Circ J 2018;82:3044-51. [Crossref] [PubMed]
- van Nunen LX, Zimmermann FM, Tonino PA, Barbato E, Baumbach A, Engstrøm T, Klauss V, MacCarthy PA, Manoharan G, Oldroyd KG, Ver Lee PN, Van't Veer M, Fearon WF, De Bruyne B, Pijls NH. Fractional flow reserve versus angiography for guidance of PCI in patients with multivessel coronary artery disease (FAME): 5-year follow-up of a randomised controlled trial. Lancet 2015;386:1853-60. [Crossref] [PubMed]
- Cerqueira MD, Weissman NJ, Dilsizian V, Jacobs AK, Kaul S, Laskey WK, Pennell DJ, Rumberger JA, Ryan T, Verani MS. Standardized myocardial segmentation and nomenclature for tomographic imaging of the heart. A statement for healthcare professionals from the Cardiac Imaging Committee of the Council on Clinical Cardiology of the American Heart Association. Circulation 2002;105:539-42. [Crossref] [PubMed]
- Kakouros N, Rybicki FJ, Mitsouras D, Miller JM. Coronary pressure-derived fractional flow reserve in the assessment of coronary artery stenoses. Eur Radiol 2013;23:958-67. [Crossref] [PubMed]
- Rampidis GP, Benetos G, Benz DC, Giannopoulos AA, Buechel RR. A guide for Gensini Score calculation. Atherosclerosis 2019;287:181-3. [Crossref] [PubMed]
- Ninomiya K, Serruys PW, Kotoku N, Zhou J, Kageyama S, Masuda S, Revaiah PC, Wang B, He X, Tsai TY, Kageyama M, Sevestre E, Sharif F, Garg S, Akasaka T, Escaned J, Patel MR, Onuma Y. Anonymous Comparison of Various Angiography-Derived Fractional Flow Reserve Software With Pressure-Derived Physiological Assessment. JACC Cardiovasc Interv 2023;16:1778-90. [Crossref] [PubMed]
- Zhang R, Wang HY, Dou K, Yin D, Zhu C, Feng L, et al. Outcomes of Functionally Complete vs Incomplete Revascularization: Insights From the FAVOR III China Trial. JACC Cardiovasc Interv 2022;15:2490-502. [Crossref] [PubMed]
- Scoccia A, Tomaniak M, Neleman T, Groenland FTW, Plantes ACZD, Daemen J. Angiography-Based Fractional Flow Reserve: State of the Art. Curr Cardiol Rep 2022;24:667-78. [Crossref] [PubMed]
- Li J, Gong Y, Wang W, Yang Q, Liu B, Lu Y, Xu Y, Huo Y, Yi T, Liu J, Li Y, Xu S, Zhao L, Ali ZA, Huo Y. Accuracy of computational pressure-fluid dynamics applied to coronary angiography to derive fractional flow reserve: FLASH FFR. Cardiovasc Res 2020;116:1349-56. [Crossref] [PubMed]
- Gaba P, Gersh BJ, Ali ZA, Moses JW, Stone GW. Complete versus incomplete coronary revascularization: definitions, assessment and outcomes. Nat Rev Cardiol 2021;18:155-68. [Crossref] [PubMed]
- Soares A, Brown DL. The fallacies of fractional flow reserve. Int J Cardiol 2020;302:34-5. [Crossref] [PubMed]