Accuracy of 3.0T magnetic resonance angiography for the detection of arteriovenous fistula dysfunction in hemodialysis patients requiring interventional therapy: a prospective study
Introduction
The maintenance of good hemodialysis access to upper limb arteriovenous fistula (AVF) is the basis of treatment for patients with end-stage renal failure (1). In hemodialysis patients with long-term dialysis treatment, due to the existence of vascular arteriosclerosis, repeated puncture, compression, infection, and other factors, vascular intimal hyperplasia and thrombosis, upper limb dialysis pathway stenosis eventually develops (2,3). Non-invasive imaging methods are often required to determine vascular lesions. Color Doppler ultrasonography (CDUS) is widely applied as a convenient, real-time imaging method to display stenotic lesions, and measure the hemodynamic parameters, and show the thrombi (4). However, sonography has certain limitations, including a possible overestimation of the degree of AVF stenosis, poor accuracy in detecting nonthrombotic venous outflow occlusions and the variability in the diagnostic quality depending on the ultrasonographer experiences (5). More importantly, CDUS is difficult to use as a guide for endovascular treatment plans due to its inability to display vascular access (6). In addition to ultrasound, enhanced imaging modalities, including computer tomography angiography (CTA) or magnetic resonance angiography (MRA), show more accurately the AVF dysfunction lesion than CDUS, with sensitivity ranging between 90.2–98.7% and specificity between 92.2–98.0% (7-12), however contrast-enhanced examination may run the risk of nephrotoxicity and nephrogenic systemic fibrosis (13,14). Digital subtraction angiography (DSA) is the “gold standard” for the diagnosis of vascular diseases and can provide dynamic flow images for further interventional therapy, but it is invasive, involves X-ray radiation exposure and uses contrast agents. Therefore, a safe and reliable imaging method is urgently needed for the accurate detection of AVF dysfunction in hemodialysis patients who may need further interventional treatment.
As a contrast-free method, time-of-flight magnetic resonance angiography (TOF-MRA), based on the “flow effect” of MR, has generated promising results for the diagnosis of intracranial artery diseases, such as aneurysm, atherosclerotic stenosis and arteriovenous shunt (15-18). In this way, a clear difference is formed between the saturated quiescent tissue and the unsaturated flowing blood to display the blood vessels (19). However, conventional TOF-MRA can only display unidirectional blood flow signals and is seldom used for displaying venous vessels. The vascular imaging with the use of AVF needs to show the involved arterial blood inflow, anastomosis and venous outflow, which may present a greater challenge for TOF MRA imaging. Gonzalez et al. reported TOF-MRA can be employed to evaluate non-maturing AVFs, however, the study did not evaluate for dysfunctional AVF and the number of subjects in that study was small (20). In this study, the pre-saturated sequence in the venous direction of the conventional TOF-MRA sequence was removed so that all the blood flow signals in the arterial and venous directions could be acquired. This study aims to apply this improved TOF-MRA to diagnose stenotic or occluded vascular lesions of dialysis access, to provide a safe and effective vascular imaging method for endovascular treatment planning for patients with dysfunctional AVFs. We present this article in accordance with the STARD reporting checklist (available at https://qims.amegroups.com/article/view/10.21037/qims-23-1505/rc).
Methods
Study design and patient population
The study was approved by the Institutional Review Board of Shanghai Sixth People’s Hospital affiliated to Shanghai Jiao Tong University School of Medicine (No. 2018-11-12) and was conducted in accordance with the Declaration of Helsinki (as revised in 2013). All patients provided written informed consent. This prospective study included individuals receiving maintenance dialysis in the nephrology unit at Shanghai Sixth People’s Hospital who were suspected of having dysfunctional AVFs, from June 2018 to March 2021 (Clinical trial No. NCT04312711). The following were the inclusion criteria for patients with dysfunctional hemodialysis access: (I) swelling in the limbs, reduction of pulsation and tremor at the anastomotic site, and reduction of the murmur; (II) both the venous pressure and the negative pressure in the arteries increased, resulting in a decrease in the blood flow through the fistula to less than 500 mL/min; (III) during dialysis, it was challenging to puncture the hemodialysis site. The exclusion criteria included: (I) prior stent or artificial blood vessel implantation; (II) contraindications to magnetic resonance; (III) platelet (PLT) level <60×109/L or international normalized ratio (INR) >1.5; (IV) pregnancy or lactation; and (V) functional failure of important organs or other serious diseases, excluding renal failure. Figure 1 presents a comprehensive flowchart outlining the process of selecting patients in details.
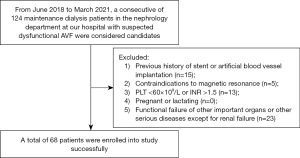
Imaging protocol
CDUS
Two sonographers of 12 and 8 years of experience independently conducted ultrasonography studies in the Department of Ultrasonography. Using 5- and 7-mHz linear array scanners (Aspen Advanced; Siemens Healthcare, Erlangen, Germany), the complete assessment of the hemodialysis access fistula was conducted, starting from the feeding artery at the axillary artery level and extending to the draining vein at the subclavian vein level. The tests were conducted in both vertical and horizontal directions. At every examination level, spectral waveforms were acquired, and color Doppler interrogation was employed during the examinations. The calculation and recording of peak systolic velocity (PSV) and the position of any constricted regions were performed. The criteria for PSV were identical for the inflow, shunt proper (such as anastomotic sites), and outflow tract. Whenever feasible, measurements were acquired from waveforms with an insonation angle below 60°. The narrowing was deemed substantial if the PSV exceeded 375 cm/s or if there was a reduction of 50% or more on the grayscale imaging. The localizations of the stenoses were drawn on a form where all data were recorded.
MRA
A superficial body array matrix coil was used to perform MRA on a 3-T system (Skyra, Siemens Healthcare, Erlangen, Germany). The imaging was conducted while the patient was in a supine position, ensuring that the target arm, which held the fistula, was positioned as close to the center of the examination bed as feasible. The TOF-MRA scan lasted approximately 5 minutes and covered a transverse slab from the elbow joint to 5 cm below the anastomosis. It utilized acquisition parameters including a repetition time of 23 ms, an echo time of 3.5 ms, a flip angle of 70°, a volume of interest measuring 200×200×200 mm3, a section thickness of 2 mm, an acquisition matrix of 1,024×1,024, and consisted of 180 slices. Using the TOF images, two highly experienced radiologists made an initial assessment of the stenosis location. Using an automated subtraction technique, maximum intensity projection (MIP) images were reconstructed for all three stations in the coronal orientation. These images were then combined into a single image using a specialized workstation called Syngo® MR from Siemens Healthcare. The schematic illustration of the TOF-MRA scan protocol is present in Figure 2.
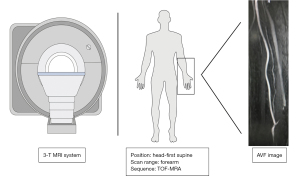
Interventional radiologists (with 17 and 15 years of expertise, respectively) performed the DSAs using the digital angiography unit (Artis zee; Siemens Medical Solutions), within an average of 10 [1–14] days following the ultrasonography and MRA. A method of entry was utilized on either the venous or brachial artery. A catheter with a diameter of 5-French (1.70 mm) was used to inject an iodine-based contrast agent (Imeron 350; Bracco Imaging, Shanghai, China). The interventional radiologist selected the anteroposterior views based on their own judgment. Pictures of feeding artery, fistula and distal venous outflow were obtained. Following the angiogram, PTA was conducted on the regions with stenoses exceeding 50% or experiencing complete occlusion.
Image analysis
The MRA and DSA images were evaluated by two experienced radiologists, one with 10 years of experience and the other with 15 years of experience in assessing vascular images. They conducted the evaluations without knowledge of the patient’s identity, medical history, or symptoms. The MRA data sets were assessed using a specialized computer (Syngo® MR, Siemens Healthcare), while the DSA images were analyzed on a different computer (SyngoXWP; Siemens Healthcare).
All regions were assessed for the diagnostic performance and image quality of TOF-MRA. The AVF architecture was divided into three regions for all participants: arterial inflow, anastomosis (including 1 cm length of vessel on both sides of the anastomosis), and venous outflow. In this study, the analysis did not include the proximal half of the brachiocephalic vein and superior caval vein, which are the main part of the venous outflow, as they were not visible in the TOF-MRA imaging.
The image quality of the pictures acquired at each of the three areas was assessed on a scale of 0–4 using the Likert rating system. 0 for an image that is considered ‘nondiagnostic’; 1 for “poor” quality, due to severe image artifacts, significant venous contamination, and/or inadequate vascular signals, which makes the observer lack confidence; 2 for “fair” quality, and the observer has slight confidence due to small imperfections, mild to moderate interference from veins, and/or a reasonably consistent signal from blood vessels; 3 for “good” quality, with the observer can be assured of a high standard of quality; 4 for an “excellent” quality, which is given for a superior quality scan that is free from or has minimal presence of venous contamination, lacks any artifacts, and exhibits consistent vascular signals, thus instilling a strong sense of confidence in the observer.
An electronic caliper was utilized to grade the stenosis of lesions on CDUS, MRA, and DSA. In all regions (Figures 3-5), the sonographer or radiologist ascertained the presence of stenosis or occlusion. The level of narrowing was evaluated using a visual scale where 0 represents a fully unobstructed vessel wall, 1 indicates less than 50% narrowing, 2 signifies 50–75% narrowing, 3 denotes 75–99% narrowing, and 4 represents complete blockage (segmental and total). Only the most critical narrowing per region was assessed for analysis purposes. Stenoses that had a luminal constriction greater than 50% were deemed to be clinically significant in terms of blood flow. If the stenosis score on TOF-MRA and CDUS was higher than that on DSA, it was deemed as an overestimation; otherwise, it was considered an underestimation (Figure 6).
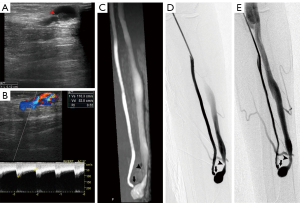
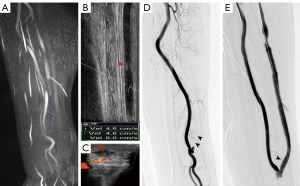
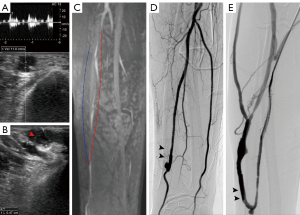
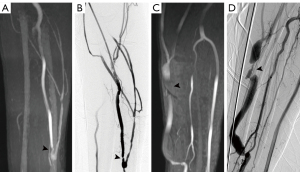
Statistical analysis
The mean ± standard deviation was used to report the Likert scores for the diagnostic image quality, and they were compared using the paired t-test. DSA was regarded as the benchmark. Proportions of sensitivities, specificities, positive predictive values (PPVs), and negative predictive values (NPVs) for detecting significant (50–100%) stenosis were displayed as both whole-region and per-region. The corresponding 95% confidence intervals (CIs) were also included for each estimate. The level of agreement between observers in determining significant stenosis was assessed by computing the k statistic. The Cohen’s kappa coefficient (κ) was computed, where κ>0.80 was considered as ‘almost perfect’, values between 0.61 and 0.80 were considered ‘substantial’, and values between 0.41 and 0.60 were considered ‘moderate’. Significance was attributed to values with a two-sided P value less than 0.05. SPSS v19.0 (IBM, Armonk, NY, USA) was utilized for the statistical analyses.
Results
Between June 2018 and March 2021, a total of 68 patients with 204 vessel segments who underwent TOF-MRA, CDUS and DSA examinations and met the inclusion criteria were prospectively included in our study, and their clinical baseline characteristics are listed in Table 1. The technique success for TOF-MRA scan was 100%. The mean acquisition time of TOF-MRA was 5.4±0.9 minutes, and the mean examination time of CDUS was 12.5±4.6 minutes. Imaging parameters for TOF-MRA, CDUS, and DSA are listed in Table 2.
Table 1
Characteristic | Participants |
---|---|
Age (years) | 61.4±12.2 |
Sex (male) | 47 (69.1) |
Hypertension | 59 (86.8) |
Hyperlipidemia | 3 (4.4) |
Smoking | 13 (19.1) |
Cause of end-stage kidney disease | |
Diabetes | 14 (20.6) |
Glomerulonephritis | 20 (29.4) |
Both diabetes and glomerulonephritis | 21 (30.9) |
Polycystic kidney disease | 2 (2.9) |
Other* | 2 (2.9) |
Unknown | 9 (13.2) |
Usable time of AVF (months) | 21.2±20.7 |
Unless otherwise indicated, the data are expressed as the number of patients, and the data in parentheses are percentages. The data are expressed as the mean ± standard deviation. *, nephropathy of amyloidosis (n=1), renal carcinoma (n=1). AVF, arteriovenous fistula.
Table 2
TOF-MRA | CDUS | DSA |
---|---|---|
Repetition time (23 ms) | Scaner (linear array) | Tube voltage (69 KV) |
Echo time (3.5 ms) | Frequency (5- and 7-mHz) | Tube current (37 mA) |
Flip angle (70°) | Pulse width (4.8 ms) | |
Volume of interest (200×200×200 mm3) | Frequency (7.5 f/s) | |
Section thickness (2 mm) | Image pixel spacing (0.75×0.75) | |
Acquisition matrix (1,024×1,024) | ||
Slices (180) |
TOF-MRA, time-of-flight magnetic resonance angiography; CDUS, color Doppler ultrasonography; DSA, digital subtraction angiography.
Image quality analysis
The patient-based analysis of image quality of TOF-MRA revealed that 2 patients were scored as grade 2, 44 patients as grade 3 and 22 patients as grade 4. The per-region-based analysis found that the average image quality score was 2.5±0.8, with 129 regions (63.2%) shows good quality, and only 3 regions (1.5%) considered nondiagnostic by TOF-MRA. On the basis of the lesion type, a significant difference of image quality was found between the patients with and the patients without total occlusive segments (1.8±0.8 vs. 2.7±0.6, P<0.001). However, between lesions with different stenotic types, the image quality analysis did not reveal any significant difference (grade 2, 3.0±0.3 vs. grade 3 2.8±0.6, P=0.576). Further region-based analyses indicated that the image quality of TOF-MRA showed no significant difference at the different sites (feeding artery, 2.5±0.7; fistula, 2.5±0.8; draining veins, 2.5±0.8; P>0.99).
Diagnostic performance comparison of TOF-MRA and CDUS
Regarding the lesion location classification based on DSA, a total of 122 segments with >50% stenosis or occlusion were confirmed. Feeding arteries were involved in 2 patients, fistulas in 4 patients, draining veins in 14 patients, both feeding arteries and fistulas in 2 patients, both fistulas and draining veins in 40 patients and whole regions in 6 patients. Taking DSA as a reference standard, the stenosis severity was overestimated in 15 segments on TOF-MRA and in 8 segments on CDUS. The stenosis severity was underestimated in 4 segments using TOF-MRA and in 27 segments using CDUS (Figure 5). The whole-region comparison of the lesion stenosis between TOF-MRA and CDUS indicated a significant difference in the sensitivity [99.1% (116 of 117) vs. 82.9% (97 of 117), P<0.001] and NPV [98.8% (81 of 82) vs. 80.4% (82 of 102), P<0.001], but they had similar specificity [93.1% (81 of 87) vs. 94.3% (82 of 87), P=0.755] and PPV [95.1% (116 of 122) vs. 95.1% (97 of 102); P=0.996]. In the per-region-based analyses, compared to CDUS, TOF-MRA yielded higher sensitivity in the fistula region [98.1% (51 of 52) vs. 80.8% (42 of 52), P=0.004] and in the draining vein region [100.0% (60 of 60) vs. 85.0% (51 of 60), P=0.003], but this was not seen in the feeding artery region due to the relatively small number of involved regions (5 cases) (Table 3).
Table 3
Parameter | TOF-MRA vs. DSA | CDUS vs. DSA | P |
---|---|---|---|
Whole-region | |||
Sensitivity | 99.1% (94.6–100.0%) | 82.9% (74.6–89.0%) | <0.001* |
Specificity | 93.1% (85.0–97.1%) | 94.3% (86.5–97.9%) | 0.755 |
PPV | 95.1% (89.2–98.0%) | 95.1% (88.4–98.2%) | 0.996 |
NPV | 98.8% (92.5–99.9%) | 80.4% (71.1–87.3%) | <0.001* |
Per region | |||
Feeding artery | |||
Sensitivity | 100.0% (46.3–100.0%) | 80.0% (29.9–98.9%) | >0.99 |
Specificity | 93.5% (83.5–97.9%) | 96.8% (87.8–99.4%) | 0.68 |
PPV | 55.6% (22.7–84.7%) | 66.7% (24.1–94.0%) | >0.99 |
NPV | 100.0% (92.3–100.0%) | 98.4% (90.0–99.9%) | >0.99 |
Fistula | |||
Sensitivity | 98.1% (88.4–99.9%) | 80.8% (67.0–89.9%) | 0.004* |
Specificity | 88.2% (62.3–97.8%) | 88.2% (62.3–97.9%) | >0.99 |
PPV | 96.2% (85.9–99.3%) | 95.5% (83.3–99.2%) | >0.99 |
NPV | 93.8% (67.7–99.7%) | 60.0% (38.9–78.2%) | 0.028* |
Draining vein | |||
Sensitivity | 100.0% (92.5–100.0%) | 85.0% (72.9–92.5%) | 0.003* |
Specificity | 100.0% (59.8–100.0%) | 87.5% (46.7–99.3%) | >0.99 |
PPV | 100.0% (92.5–100.0%) | 98.1% (88.4–99.9%) | 0.464 |
NPV | 100.0% (59.8–100.0%) | 43.8% (20.1–69.4%) | 0.009* |
Data are expressed as the diagnostic performance of TOF-MRA and CDUS using DSA as the reference standard with the 95% confidence intervals in parentheses. *, represents significant difference. TOF-MRA, time-of-flight magnetic resonance angiography; CDUS, color Doppler ultrasonography; AVF, arteriovenous fistula; DSA, digital subtraction angiography; PPV, positive predictive value; NPV, negative predictive value.
Interobserver agreement
The κ values of the diagnostic performance and image quality indicated good agreement between both readers. With regard to the diagnostic performance, the κ value for TOF-MRA was 0.975 and for CDUS, it was 0.964. With respect to the image quality of TOF-MRA, the κ value was 0.866.
Discussion
In the present study, TOF-MRA was a feasible technique in patients with suspected hemodialysis access stenosis for subsequent angioplasty treatment plans. Using DSA as the reference standard, the diagnostic accuracy of TOF-MRA was high (sensitivity of 98.3–99.1% and specificity of 93.1–95.4%), which was superior to CDUS. The image quality of TOF-MRA was also satisfactory, with a score of 2.5±0.8.
When AVF dysfunction is present, precise imaging of the entire hemodialysis access and identifying the specific stenosis lesions play an important role. CDUS has proven to be a valuable and reliable screening tool in managing clinically suspected hemodialysis access stenosis. The results of CDUS in this study were similar to those reported in previous literature (21), and we found an 82.9% sensitivity and a 94.3% specificity. However, when further endovascular treatment is required, CDUS has limitations with respect to the depiction of vascular tree vessels for definitive therapy planning. Consequently, CTA or contrast enhanced MRA (CE-MRA) showed a better performance than CDUS for this purpose. Doelman et al. reported that the sensitivity and specificity of CE-MRA with DSA for the detection of significant (>50%) stenoses in a failing dialysis accesses were 96% and 98%, respectively (7), which indicated that CE-MRA can be used for the evaluation of a failing AVF access. CTA also demonstrated a sensitivity ranging between 90.2–98.7% and a specificity between 92.2–97.5% (8-12). Nonetheless, what cannot be ignored is that the contrast-enhanced MR technique requires venous puncture, whereas the Dialysis Outcome Quality Initiative guidelines indicate that puncture of peripheral veins should be avoided for venous preservation (22). In addition, gadolinium or iodic contrast medium may have some nephrotoxicity properties in patients with residual renal function (14,23,24); therefore, the routine use of CE-MRA or CTA is restricted in the clinical setting for symptomatic AVF evaluation.
TOF-MRA, as a nonenhanced MR angiography technique, has been carried out for the assessment of cerebrovascular disease (25,26) and is seldom used for hemodialysis access since TOF-MRA is unable to adequately depict the venous vessels. When TOF-MRA was applied for cerebrovasculature, a presaturation pulse was used inferior to the slice to suppress the signal from venous blood, and the presaturation bands were omitted in our study to allow simultaneous visualization of both arterial and venous blood flow. Jin et al. assessed the diagnostic value of TOF-MRA in comparison to multidetector CTA, and the study yielded a 96.4% sensitivity and a 94.3–97.1% specificity (27). In our study, the region-based analyses of significant stenosis (50–100%) for TOF-MRA showed a 99.1% sensitivity and a 93.1% specificity, which is consistent with the previous study. The stenosis severity of 15 segments using TOF-MRA was overestimated in our study, and in contrast, CDUS overestimated the stenosis severity in only 8 segments. The reason for the TOF-MRA overestimation in the stenosis of AVF was attributed to the flow voids caused by poststenotic intravoxel phase dispersion. Interestingly, due to the relatively lower sensitivity of CDUS compared with TOF-MRA (82.9% vs. 99.1%, P<0.001), the stenosis severity was underestimated in 27 segments by CDUS, and only 4 segments by TOF-MRA were underestimated. Taken together, these above results confirmed the notion that TOF-MRA is a promising method for the assessment of dysfunctional AVF before further endovascular treatment.
However, challenges of TOF-MRA for AVF evaluation still exist. The patients with total occlusive AVF segments showed significantly lower image quality scores than those without total occlusive AVF segments (1.8±0.8 vs. 2.7±0.6, P<0.001) in this study. As a potential mechanism, there is two-way blood flow in the vessels if the AVF is completely occlusive, which leads to the confusion of determining the blood signals; moreover, as an essential pitfall of TOF-MRA, it is insensitive to slow blood flow, which becomes desaturated similar to stationary tissue, resulting in a signal loss from the vessel (28). Therefore, since the flow rate of blood is slowed down in the occlusive AVF and the hemodynamic signals are too sluggish to be detected within the occlusive vessels, a poor image quality might be the result. Since black-blood T1WI, also known as vessel wall enhancement (VWE), is a reliable examination method to enable analysis of vessel wall, it might be an alternative method for detecting total occlusive AVF (29). Although VWE has been used widely in the evaluation of intracranial vasculopathies, it requires a relatively long examination time and gadolinium-based contrast media, limiting its practical applications for patients with end-stage renal failure. Another challenge of TOF-MRA is its relatively long acquisition time. The TOF-MRA examination time was approximately 5 minutes at our center, sometimes it was difficult for the patients with end-stage renal disease to maintain long periods of immobility, which may result in a decrease in the image quality. Moreover, the MR scan area included only the elbow joint to 5 cm below the anastomosis and the remaining AVF access was not included. As we consider that the majority of stenoses of dysfunctional AVFs were located in a forearm vessel, and only one patient who had a brachiocephalic vein occlusion with a temporary dialysis cannulation history was excluded from the analysis.
Our study had limitations. The single-center experience with a limited number of included patients may not be able to investigate the feasibility and accuracy of TOF-MRA for the diagnosis of AVF dysfunction fully. Moreover, further studies are required to investigate whether TOF-MRA may improve the performance of subsequent endovascular treatment. Finally, patients with arteriovenous grafts, which are commonly seen in clinical practice, were not enrolled in this study.
Conclusions
In conclusion, by using DSA as reference, this study showed that TOF-MRA was superior to CDUS in accurately diagnosing dysfunctional AVFs in hemodialysis patients, which may facilitate subsequent endovascular interventions.
Acknowledgments
The abstract of this study was published in the CIRSE Congress 2023 book of Abstracts.
Funding: This study was supported by
Footnote
Reporting Checklist: The authors have completed the STARD reporting checklist. Available at https://qims.amegroups.com/article/view/10.21037/qims-23-1505/rc
Conflicts of Interest: All authors have completed the ICMJE uniform disclosure form (available at https://qims.amegroups.com/article/view/10.21037/qims-23-1505/coif). The authors have no conflicts of interest to declare.
Ethical Statement: The authors are accountable for all aspects of the work in ensuring that questions related to the accuracy or integrity of any part of the work are appropriately investigated and resolved. The study was conducted in accordance with the Declaration of Helsinki (as revised in 2013). The study was approved by the Institutional Review Board of Shanghai Sixth People’s Hospital affiliated to Shanghai Jiao Tong University School of Medicine (No. 2018-11-12), and all patients provided written informed consent.
Open Access Statement: This is an Open Access article distributed in accordance with the Creative Commons Attribution-NonCommercial-NoDerivs 4.0 International License (CC BY-NC-ND 4.0), which permits the non-commercial replication and distribution of the article with the strict proviso that no changes or edits are made and the original work is properly cited (including links to both the formal publication through the relevant DOI and the license). See: https://creativecommons.org/licenses/by-nc-nd/4.0/.
References
- Allon M. Vascular Access for Hemodialysis Patients: New Data Should Guide Decision Making. Clin J Am Soc Nephrol 2019;14:954-61. [Crossref] [PubMed]
- Ravani P, Palmer SC, Oliver MJ, Quinn RR, MacRae JM, Tai DJ, Pannu NI, Thomas C, Hemmelgarn BR, Craig JC, Manns B, Tonelli M, Strippoli GF, James MT. Associations between hemodialysis access type and clinical outcomes: a systematic review. J Am Soc Nephrol 2013;24:465-73. [Crossref] [PubMed]
- Viecelli AK, Mori TA, Roy-Chaudhury P, Polkinghorne KR, Hawley CM, Johnson DW, Pascoe EM, Irish AB. The pathogenesis of hemodialysis vascular access failure and systemic therapies for its prevention: Optimism unfulfilled. Semin Dial 2018;31:244-57. [Crossref] [PubMed]
- Mudoni A, Caccetta F, Caroppo M, Musio F, Accogli A, Zacheo MD, Burzo MD, Gallieni M, Nuzzo V. Echo color Doppler ultrasound: a valuable diagnostic tool in the assessment of arteriovenous fistula in hemodialysis patients. J Vasc Access 2016;17:446-52.
- Pietryga JA, Little MD, Robbin ML. Sonography of Arteriovenous Fistulas and Grafts. Semin Dial 2017;30:309-18. [Crossref] [PubMed]
- Stoumpos S, Tan A, Hall Barrientos P, Stevenson K, Thomson PC, Kasthuri R, Radjenovic A, Kingsmore DB, Roditi G, Mark PB, Ferumoxytol MR. Angiography versus Duplex US for Vascular Mapping before Arteriovenous Fistula Surgery for Hemodialysis. Radiology 2020;297:214-22. [Crossref] [PubMed]
- Doelman C, Duijm LE, Liem YS, Froger CL, Tielbeek AV, Donkers-van Rossum AB, Cuypers PW, Douwes-Draaijer P, Buth J, van den Bosch HC. Stenosis detection in failing hemodialysis access fistulas and grafts: comparison of color Doppler ultrasonography, contrast-enhanced magnetic resonance angiography, and digital subtraction angiography. J Vasc Surg 2005;42:739-46. [Crossref] [PubMed]
- Meyer M, Geiger N, Benck U, Rose D, Sudarski S, Ong MM, Schoenberg SO, Henzler T. Imaging of Patients with Complex Hemodialysis Arterio-Venous Fistulas using Time-Resolved Dynamic CT Angiography: Comparison with Duplex Ultrasound. Sci Rep 2017;7:12563. [Crossref] [PubMed]
- Dimopoulou A, Raland H, Wikström B, Magnusson A. MDCT angiography with 3D image reconstructions in the evaluation of failing arteriovenous fistulas and grafts in hemodialysis patients. Acta Radiol 2011;52:935-42. [Crossref] [PubMed]
- Ko SF, Huang CC, Ng SH, Lee TY, Hsieh MJ, Lee FY, Chen MC, Sheen-Chen SM, Lee CH. MDCT angiography for evaluation of the complete vascular tree of hemodialysis fistulas. AJR Am J Roentgenol 2005;185:1268-74. [Crossref] [PubMed]
- Heye S, Maleux G, Claes K, Kuypers D, Oyen R. Stenosis detection in native hemodialysis fistulas with MDCT angiography. AJR Am J Roentgenol 2009;192:1079-84. [Crossref] [PubMed]
- Ye C, Mao Z, Rong S, Zhang Y, Mei C, Li H, Dong S. Multislice computed tomographic angiography in evaluating dysfunction of the vascular access in hemodialysis patients. Nephron Clin Pract 2006;104:c94-100. [Crossref] [PubMed]
- Briguori C, Colombo A, Airoldi F, Morici N, Sangiorgi GM, Violante A, Focaccio A, Montorfano M, Carlino M, Condorelli G, Ricciardelli B. Nephrotoxicity of low-osmolality versus iso-osmolality contrast agents: impact of N-acetylcysteine. Kidney Int 2005;68:2250-5. [Crossref] [PubMed]
- Ronco F, Tarantini G, McCullough PA. Contrast induced acute kidney injury in interventional cardiology: an update and key guidance for clinicians. Rev Cardiovasc Med 2020;21:9-23. [Crossref] [PubMed]
- HaiFeng L. YongSheng X, YangQin X, Yu D, ShuaiWen W, XingRu L, JunQiang L. Diagnostic value of 3D time-of-flight magnetic resonance angiography for detecting intracranial aneurysm: a meta-analysis. Neuroradiology 2017;59:1083-92. [Crossref] [PubMed]
- Kim DK, Verdoorn JT, Gunderson TM, Huston Iii J, Brinjikji W, Lanzino G, Lehman VT. Comparison of non-contrast vessel wall imaging and 3-D time-of-flight MRA for atherosclerotic stenosis and plaque characterization within intracranial arteries. J Neuroradiol 2020;47:266-71. [Crossref] [PubMed]
- Sakata A, Fushimi Y, Okada T, Nakajima S, Hinoda T, Speier P, Schmidt M, Forman C, Yoshida K, Kataoka H, Miyamoto S, Nakamoto Y. Evaluation of cerebral arteriovenous shunts: a comparison of parallel imaging time-of-flight magnetic resonance angiography (TOF-MRA) and compressed sensing TOF-MRA to digital subtraction angiography. Neuroradiology 2021;63:879-87. [Crossref] [PubMed]
- Sakata A, Sakamoto R, Fushimi Y, Nakajima S, Hinoda T, Oshima S, Wetzl J, Schmidt M, Okawa M, Yoshida K, Miyamoto S, Nakamoto Y. Low-dose contrast-enhanced time-resolved angiography with stochastic trajectories with iterative reconstruction (IT-TWIST-MRA) in brain arteriovenous shunt. Eur Radiol 2022;32:5392-401. [Crossref] [PubMed]
- Tang H, Hu N, Yuan Y, Xia C, Liu X, Zuo P, Stalder AF, Schmidt M, Zhou X, Song B, Sun J. Accelerated Time-of-Flight Magnetic Resonance Angiography with Sparse Undersampling and Iterative Reconstruction for the Evaluation of Intracranial Arteries. Korean J Radiol 2019;20:265-74. [Crossref] [PubMed]
- Gonzalez AJ, Casey KM, Drinkwine BJ, Weiss JS. Series of Noncontrast Time-of-Flight Magnetic Resonance Angiographies to Identify Problems with Arteriovenous Fistula Maturation. Ann Vasc Surg 2016;30:93-9. [Crossref] [PubMed]
- Wiese P, Nonnast-Daniel B. Colour Doppler ultrasound in dialysis access. Nephrol Dial Transplant 2004;19:1956-63. [Crossref] [PubMed]
- Ikizler TA, Burrowes JD, Byham-Gray LD, Campbell KL, Carrero JJ, Chan W, Fouque D, Friedman AN, Ghaddar S, Goldstein-Fuchs DJ, Kaysen GA, Kopple JD, Teta D, Yee-Moon Wang A, Cuppari L. KDOQI Clinical Practice Guideline for Nutrition in CKD: 2020 Update. Am J Kidney Dis 2020;76:S1-S107. [Crossref] [PubMed]
- Wiginton CD, Kelly B, Oto A, Jesse M, Aristimuno P, Ernst R, Chaljub G. Gadolinium-based contrast exposure, nephrogenic systemic fibrosis, and gadolinium detection in tissue. AJR Am J Roentgenol 2008;190:1060-8. [Crossref] [PubMed]
- McCullough PA, Choi JP, Feghali GA, Schussler JM, Stoler RM, Vallabahn RC, Mehta A. Contrast-Induced Acute Kidney Injury. J Am Coll Cardiol 2016;68:1465-73. [Crossref] [PubMed]
- Li MH, Li YD, Gu BX, Cheng YS, Wang W, Tan HQ, Chen YC. Accurate diagnosis of small cerebral aneurysms ≤5 mm in diameter with 3.0-T MR angiography. Radiology 2014;271:553-60. [Crossref] [PubMed]
- Zhang Z, Wang Y, Zhou S, Li Z, Peng Y, Gao S, Zhu G, Wu F, Wu B. The automatic evaluation of steno-occlusive changes in time-of-flight magnetic resonance angiography of moyamoya patients using a 3D coordinate attention residual network. Quant Imaging Med Surg 2023;13:1009-22. [Crossref] [PubMed]
- Jin WT, Zhang GF, Liu HC, Zhang H, Li B, Zhu XQ. Non-contrast-enhanced MR angiography for detecting arteriovenous fistula dysfunction in haemodialysis patients. Clin Radiol 2015;70:852-7. [Crossref] [PubMed]
- Kiruluta AJM, González RG. Magnetic resonance angiography: physical principles and applications. Handb Clin Neurol 2016;135:137-49. [Crossref] [PubMed]
- McNally JS, Sakata A, Alexander MD, Dewitt LD, Sonnen JA, Menacho ST, Stoddard GJ, Kim SE, de Havenon AH. Vessel Wall Enhancement on Black-Blood MRI Predicts Acute and Future Stroke in Cerebral Amyloid Angiopathy. AJNR Am J Neuroradiol 2021;42:1038-45. [Crossref] [PubMed]