Identifying the cause of cardiac arrest through coronary computed tomography angiography with high heart rate under venoarterial extracorporeal membrane oxygenation support: a case description
Introduction
Extracorporeal membrane oxygenation (ECMO) is an enhanced cardiopulmonary bypass technique used to sustain life and allow for further treatment during severe cardiopulmonary failure (1). Several randomized studies conducted in various clinical settings have demonstrated that ECMO is a life-saving technology that can reliably improve survival rates among pediatric and adult patients (2-5). With technical advancements in the hardware design and monitoring platform, the application of ECMO has been largely broadened over the past two decades (6,7).
Cardiac hemodynamic assessment is crucial for patients under ECMO. However, these patients are mostly immobilized and intubated, ruling out the application of most imaging techniques of cardiac hemodynamic assessment, such as ultrasound, computed tomography angiography (CTA), magnetic resonance imaging (MRI), and emission computed tomography (ECT) (8). Coronary CTA (CCTA) has been considered the most effective noninvasive method for evaluating coronary arteries, but traditional CCTA requires heart rate control and respiratory coordination, which are challenging under ECMO support (9). ECMO can change blood flow, causing difficulties in the venous injection of contrast agents for CCTA examinations (10). As a result, patients under ECMO are underserved in terms of CCTA examinations.
We report a case of successful CCTA scanning of a patient with a high heart rate [>80 beats per minute (bpm)] under venoarterial ECMO (VA-ECMO) support. We determined the location of watershed area by monitoring the pulsations of the carotid and radial arteries. The VA-ECMO flow was reduced to withdraw the ECMO watershed. We increased the flow rate of contrast agent injection via the elbow median vein to superior vena cava. A large pitch fast large-area scan hardware (FLASH) dual-phase imaging scan with electrocardiogram gating was used to complete the CCTA examination of a patient who could not coordinate breathing and heart rate. To the best of our knowledge, this is the first report of a high heart rate CCTA examination performed under VA-ECMO support. In addition, we reviewed the principles of VA-ECMO and summarized the considerations for performing CCTA examinations under VA-ECMO support.
Case presentation
All procedures performed in this study were in accordance with the ethical standards of the institutional and/or national research committee(s) and with the Helsinki Declaration (as revised in 2013). Written informed consent was obtained from the patient for the publication of this case report and accompanying images. A copy of the written consent is available for review by the editorial office of this journal.
The patient was a 38-year-old male who experienced chest tightness and palpitations while giving a speech outdoors but did not take any measures to manage it. Fifteen minutes later, he suddenly lost consciousness and was unresponsive. His companions called for an ambulance that arrived after 5 min. The emergency medical team determined that the patient had undergone cardiac arrest and immediately began cardiopulmonary resuscitation (CPR). After 20 min of CPR, the electrocardiogram revealed ventricular fibrillation. Defibrillation was attempted, but the patient did not regain a spontaneous rhythm. The patient was transported to the local hospital where emergency endotracheal intubation was performed. Intravenous administration of epinephrine via a 10-mg pump at 30 mL/h was initiated and CPR was continued. One hour after the cardiac arrest, the patient regained a spontaneous rhythm. Two defibrillation attempts were made during this period due to recurrent ventricular fibrillation. Four hours later, the patient was transferred to the Second Affiliated Hospital of Zhejiang University with a diagnosis of “cardiac arrest after CPR”. Upon admission, the patient had a heart rate of 89 bpm, a respiratory rate of 17 breaths per minute, and a blood pressure of 95/67 mmHg (epinephrine infusion at a rate of 10 mg/h to counteract arrhythmias).
The remarkable results of the emergency laboratory tests were as follows: a high level of N-terminal pro-B-type natriuretic peptide (NT-proBNP) of 1,264 pg/mL, a creatine phosphokinase level elevated to 3,330 U/L, a platelet count decreased to 88×109/L, a prothrombin time (PT) increased to 15.2 s, an activated partial thromboplastin time (APTT) increased to 71.8 s, a thrombin time (TT) increased to 80.4 s, a troponin T level elevated to 0.585 ng/mL, a C-reactive protein (CRP) level elevated to 76.1 mg/L, an interleukin 6 (IL-6) level elevated to 2,134.00 pg/mL, and wide QRS complex on the electrocardiogram. Bedside ultrasound indicated severely decreased cardiac contractility. Considering the possibility of recurrent cardiac arrest, we considered the patient a candidate for VA-ECMO implantation. With the consent of the family, VA-ECMO was implanted under anesthesia, with the venous drainage cannula inserted in the right femoral vein to the inferior vena cava, and the arterial return cannula inserted in the right femoral artery. The flow rate, rotational speed, gas flow rate, and oxygen concentration were 3.05 L/min, 3,500 rpm, 3 L/min, and 80%, respectively. Heparin was administered at a rate of 400 units per hour. The patient was placed on a mechanical ventilation system with endotracheal intubation where volume-controlled ventilation was used with a tidal volume of 420 mL, a fraction of inspired oxygen (FiO2) of 40%, an inspiratory to expiratory (I:E) ratio of 1:2, and a positive end-expiratory pressure (PEEP) of 5 cmH2O. Meanwhile, the target temperature therapy was continued. The patient was a lecturer and experienced significant work-related stress. Therefore, the diagnoses considered for the patient included acute coronary syndrome, fulminant myocarditis (FM), cardiogenic shock, malignant arrhythmias, and postcardiac arrest syndrome.
After admission, the patient underwent an echocardiogram within 1 hour, which revealed diffuse left ventricular hypokinesis with mild left ventricular dilatation but no other abnormalities. Emergency CT scans of the head and abdomen showed no significant findings, while a chest CT scan revealed pleural effusion with associated lung atelectasis. At 4 hours after admission, the phosphokinase level decreased to 2,888 U/L (reference range: <164 U/L), and troponin T levels were measured and found to be 2.330 ng/mL (above the normal range), which further increased to 2.380 ng/mL at 8 hours after admission. No relevant features were observed on electrocardiography. Based on these findings, myocarditis, myocardial infarction, or pulmonary embolism were suspected, and a definitive diagnosis was needed for appropriate treatment. Bedside thoracic ultrasonography and bilateral venous and arterial Doppler ultrasonography of the lower extremity were performed, which showed normal lung ventilation and normal lower extremity vasculature, ruling out the possibility of pulmonary embolism. Considering that the patient had been in cardiac arrest for 1 hour and there was abnormal coagulation function, invasive diagnostic procedures with ECMO support (e.g., percutaneous coronary intervention and myocardial biopsy) involved a higher degree of risk. Meanwhile, the patient’s family refused invasive diagnosis. The presence of ferromagnetic devices (ECMO, ventilator, etc.) made it impossible for the patient to undergo cardiac MRI. Therefore, CCTA was used as an alternative diagnostic method to determine whether the patient had myocarditis or myocardial infarction (11).
To facilitate CCTA examination under ECMO support, a multidisciplinary approach was proposed based on interdepartmental collaboration with the experts in ultrasound, clinical medicine, radiology, and nursing. The patient’s cardiac output function was assessed using cardiac ultrasound at 1 hour, 0.5 days, 1 day, and 1.5 days after admission, which showed left ventricular output improvement of 22%, 26%, 26%, and 35%, respectively. Considering the patient’s significant cardiac function recovery, in multidisciplinary panel discussion, it was confirmed to attempt CCTA examination. As the ECMO venous cannula was ideally positioned in the inferior vena cava, away from the right atrium, no adjustment in position was necessary. A high-pressure venous catheter was inserted via the right antecubital vein and advanced into the right atrium to ensure rapid contrast agent delivery to the heart. Four monitoring points were established at the left carotid artery, left radial artery, right carotid artery, and right femoral artery to assess arterial pulsations, aiding in determining potential watershed locations. To reduce the renal damage induced by iodinated contrast agents, we initiated continuous renal replacement therapy (CRRT) and connected it to ECMO.
Two days after admission, CCTA was performed under cardiac gating. The imaging was conducted using a dual source CT scanner (SOMATOM Force, Siemens Healthineers, German) with 192 slices and CARE kV and CARE mAs current modulation. The pitch was set at 3.6, and the collimator width was 0.6 mm. The scan range extended from 2 cm below the bifurcation of the pulmonary artery to the diaphragm. Contrast agent was administered with a double-barrel high-pressure injector (OptiVantage, Guerbet, Princeton, NJ, USA) through the venous catheter into the right antecubital vein at a flow rate of 6.0 mL/s, with 80 mL of contrast agent being administered and followed by a 40-mL saline flush. A region of interest (ROI) was placed in the upper right quadrant of the ascending aorta, with the threshold set at 210 Hounsfield units (HU). To minimize the influence of respiration and heartbeats on the images, a novel approach of dual-phase and dual-direction continuous FLASH high-pitch scanning was employed. The nitrate treatment was not performed considering its possible effect on blood pressure. The two phases consisted of an early arterial phase and a late arterial phase. After the ROI threshold of 210 HU was reached, the first scan was automatically triggered during the early systolic phase. The scan direction was from head to foot. Immediately afterward, the second scan was initiated during the late systolic phase, with the direction from foot to head. To minimize the influence of ECMO retrograde flow, the VA-ECMO flow rate was reduced to 2.5 L/min, and arterial pulsations were observed in the left carotid artery, right carotid artery, and right femoral artery, ensuring that the ECMO flow did not interfere with cardiac blood flow. Meanwhile, the contrast agent flow rate was increased to 6.0 mL/s to optimize opacification of the proximal ascending aorta, pushing the watershed caused by VA-ECMO as far distally as possible to enable clear visualization of the coronary arteries. During the scan, the patient’s heart rate was 100 bpm. The imaging data were postprocessed on a postprocessing workstation (Syngo.via, Siemens Healthineers) with a slice thickness of 0.75 mm to generate various image reconstructions, including multiplanar reformation (MPR) and volume rendering (VR). The results indicated normal coronary artery courses without significant stenosis, conclusively ruling out myocardial infarction (Figure 1). Consequently, the primary consideration for underlying cause shifted to myocarditis. At 8:00 AM of the third day after admission, the ECMO flow rate was adjusted to 2.5 L/min and then reduced to 1.5 L/min after 2 hours and to 1 L/min after 4 hours. Subsequent cardiac ultrasound reassessment revealed acceptable cardiac function and indications for ECMO weaning. At 4:00 PM the patient was successfully decannulated from ECMO. Enhanced cardiovascular magnetic resonance (CMR) was performed at 15 days after admission since the patient refused myocardial biopsy. The results indicated left ventricular enlargement, slight myocardial thinning in the apex of the left ventricle, and subepicardial enhancement in delayed scanning, which confirmed the diagnosis of FM. Although the optimal timing for hormone pulse therapy was missed, based on peer experience, we attempted hormone pulse therapy to improve the patient’s prognosis as much as possible (12-14). Subsequently, the patient received steroid pulse therapy (500 mg/day of methylprednisolone for the first 3 days, followed by 240 mg/day for the intermediate 3 days, and oral prednisolone tapering from 125 mg/day for the last 3 days). The patient’s vital signs remained relatively stable during the later stages of hospitalization. Ultimately, enhanced CMR revealed acute myocarditis, leading to the definitive diagnosis that determined the patient’s ability to recover and be discharged. The patient recovered sufficiently to be discharged on the 30th day after admission and was followed up at the hospital at 3, 6, 9, and 12 months after discharge (Figure 2).
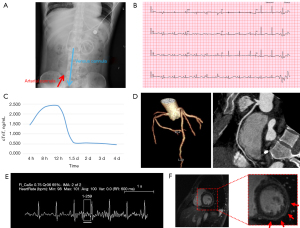
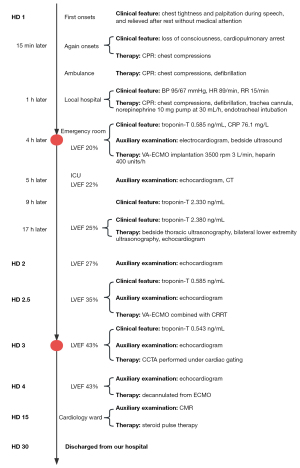
Discussion
Venovenous ECMO (VV-ECMO) and VA-ECMO cannulation are two major ECMO strategies (15,16). VV-ECMO is primarily used for the treatment of acute respiratory failure or severe lung dysfunction, wherein blood is withdrawn from the systemic venous circulation, oxygenated, and returned to the systemic venous circulation. VA-ECMO (Figure 3), on the other hand, is a temporary mechanical circulatory support employed for patients with cardiac arrest, cardiogenic shock, or heart failure (15,17,18). VA-ECMO serves as a salvage therapy, stabilizing patients with compromised hemodynamics for days or weeks (19). Here, we report a case of successful treatment of an ECMO-supported patient with FM-induced cardiac arrest, which was diagnosed using CCTA under ECMO support. Performing CCTA under ECMO is challenging but it enables definitive diagnosis and evaluation of therapeutic efficacy in patients with ECMO (20). This 38-year-old patient experienced out-of-hospital critical cardiac arrest with absolute indication for ECMO. The patient underwent 1 hour of cardiac arrest with no heartbeat or respiration, and the survival rate is negatively associated to the duration of cardiac arrest (21). Nevertheless, prompt identification of the cause of cardiac arrest greatly contributed to the patient’s excellent survival outcome. This case provides clinical physicians with valuable insights into ECMO treatment in terms of diagnosis and therapeutic interventions.
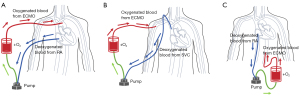
The most common cause of cardiac arrest and cardiogenic shock is acute myocardial infarction, with a small proportion attributed to acute FM particularly among young individuals (22). FM is a rare syndrome characterized by sudden and severe diffuse inflammation of the heart, often leading to cardiogenic shock, ventricular arrhythmias, or multiorgan system failure (23,24). In real-world scenarios, the diagnosis of FM has undoubtedly shifted from primarily relying on biopsy, to utilizing CMR, and to characterizing myocardial tissue changes and measuring cardiac troponin levels for identifying myocardial injury (23,25,26). However, for this patient, myocardial biopsy was not feasible, and CMR is not the initial diagnostic modality for critically ill patients. The patient in question was a 30-year-old lecturer with high-risk factors for myocardial infarction, with significantly elevated cardiac troponin levels and wide QRS complexes on electrocardiography after admission. Therefore, acute myocardial infarction rather than FM was considered as the initial clinical diagnosis. Coronary artery bypass was considered when the patient’s cardiac troponin-T continued to rise. Cardiac bypass surgery under ECMO support remains a highly risky procedure (27). We urgently conducted CCTA under ECMO support to identify the means for a safe and efficient intervention. CCTA was used to determine if the patient’s sudden cardiac arrest was caused by obstructive myocardial infarction, as CCTA has a strong negative predictive value for obstructive coronary artery disease (CAD). Due to the aforementioned limitations, the possibility of other conditions, including cardiac sarcoidosis, dilated myocardiopathy, and vascular spasm angina could not be excluded. It has been reported that CCTA can also identify prognostically important nonobstructive CAD, such as vascular spasm angina (28,29). CCTA is becoming a frontline strategy for chest pain patients and has been applied in the diagnosis of dilated cardiomyopathy (30,31). CCTA might be used in conjunction with echocardiography to exclude cardiac sarcoidosis. Although echocardiography is not a sensitive tool for diagnosing cardiac sarcoidosis, due to its high specificity, it can be used to identify cardiac dysfunction caused by cardiac sarcoidosis (32). The noninvasive, accurate diagnosis of the etiology of cardiac arrest in emergent clinical scenarios warrants further investigation.
In VA-ECMO, blood is typically withdrawn via the venous cannula of the ECMO system from the inferior vena cava or right atrium, posing challenges for intravenous injection of contrast agents during CCTA examination (33). It is possible for intracardiac contrast agent to be aspirated by the venous cannula of the ECMO circuit. To overcome this issue, we propose two methods for CTA imaging under VA-ECMO support (Figure 4). The first method involves inserting the venous cannula for contrast agent injection through the brachiocephalic vein and superior vena cava into the right atrium, while positioning the ECMO venous cannula as far away from the right atrium as possible. This method relies on the inherent cardiac blood flow for imaging. Therefore, increasing the flow rate and volume of contrast agent is necessary to ensure its mixture with blood in the cardiac chambers before pumped out. An alternative is a higher concentration of contrast agent with a reduced ECMO flow rate. The second method includes inserting the venous cannula for contrast agent injection through the brachiocephalic vein and the superior vena cava into the superior vena cava, while positioning the ECMO venous cannula in the inferior vena cava, leading to retrograde delivery of the contrast agent into the aorta by ECMO. In this method, the native blood flow of the heart is not mixed with the contrast agent, which may affect the final image quality. Both methods should be carefully considered based on the patient’s cardiac function.
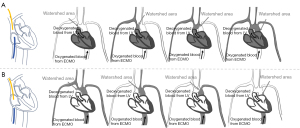
In CCTA under VA-ECMO support, it is crucial to consider the presence of the watershed phenomenon (34,35). ECMO provides retrograde blood flow from the femoral artery or iliac artery back into the thoracic aorta (36). The location of the watershed area can be anywhere between the aortic root and the diaphragm, depending on the relative output of the left ventricle (LV) compared to the retrograde ECMO flow. When antegrade LV output is high, the watershed area is located in the distal segment (near the diaphragm). When LV output is relatively low compared to ECMO flow, the watershed area is more proximal (closer to the aortic root) (37). Identifying the location of the watershed area before CTA examination is important, as it determines the farthest point to which blood pumped by the heart can reach. Clinically, the accurate detection of watershed depends on implanted arterial line monitoring and contrast-enhanced ultrasound (15,38). Here we propose a method applicable for clinical practice. Since ECMO blood flow is non-pulsatile, the presence of pulsation can be used as a benchmark of watershed. Meanwhile, the direction (from LV or ECMO) the contrast agent enters the arterial circulation is an important consideration (Figure 4). In this case, we inserted the venous cannula for contrast agent injection through the brachiocephalic vein and superior vena cava into the superior vena cava while positioning the ECMO venous cannula in the right atrium. Before adjusting the ECMO flow rate, we observed pulsation in the right carotid artery and right radial artery. After reducing the ECMO flow rate, we also observed pulsation in the left carotid artery and left radial artery, indicating that the watershed had been pushed away from the heart. However, it should be noted that maintaining a low ECMO flow rate may result in cerebral hypoxia even with a ventilator for oxygenation. Therefore, it is important to minimize the duration of ECMO deceleration, which requires close collaboration between the ECMO and radiology teams. We recommend reducing the ECMO flow rate after acquiring localization and non-contrast images. This can aid in limiting the ECMO deceleration time within 12 min and reducing the risk of hypoxia.
We recommend evaluating cardiac function prior to the CCTA examination under VA-ECMO support. Despite reduced LV output, it can still meet the requirements for CCTA examination since the coronary arteries are located at the aortic orifice. The contrast agent can be administrated at a high injection rate to achieve optimal filling of the native coronary arteries. The presence of nonuniform enhancement after contrast agent injection depends on factors such as native ejection fraction, direction and rate of contrast agent flow, total amount of contrast agent, and scan delay time. A dual-source CT can achieve a temporal resolution of 66 ms (39) and enable CCTA examination in patients under ECMO (40). FLASH spiral acquisition with electrocardiogram gating has a high success rate in patients with a heart rate below 80 bpm (41). However, we adopted a continuous dual-phase scanning approach for higher reliability.
CT examination is applicable for patients under ECMO without significant complications to disclose unique information compared with other common imaging modalities including X-ray and bedside ultrasound. According to our experience, this case was not a routine event, as the scans are conducted in the hospital’s radiology department. It requires a dedicated multidisciplinary team to transfer the patient along with the working ECMO equipment from the intensive care unit to the CT scan room in approximately 3 min.
Conclusions
The successful treatment of this patient can be attributed to the prompt assistance of ECMO for circulatory support and the establishment of a clear diagnosis. This case highlights the importance of a multidisciplinary approach to diagnosis, serving as a reference for clinical practitioners encountering similar cases.
Acknowledgments
Funding: This study was supported by
Footnote
Conflicts of Interest: All authors have completed the ICMJE uniform disclosure form (available at https://qims.amegroups.com/article/view/10.21037/qims-23-1425/coif). The authors have no conflicts of interest to declare.
Ethical Statement: The authors are accountable for all aspects of the work in ensuring that questions related to the accuracy or integrity of any part of the work are appropriately investigated and resolved. All procedures performed in this study were in accordance with the ethical standards of the institutional and/or national research committee(s) and with the Helsinki Declaration (as revised in 2013). Written informed consent was obtained from the patient for the publication of this case report and accompanying images. A copy of the written consent is available for review by the editorial office of this journal.
Open Access Statement: This is an Open Access article distributed in accordance with the Creative Commons Attribution-NonCommercial-NoDerivs 4.0 International License (CC BY-NC-ND 4.0), which permits the non-commercial replication and distribution of the article with the strict proviso that no changes or edits are made and the original work is properly cited (including links to both the formal publication through the relevant DOI and the license). See: https://creativecommons.org/licenses/by-nc-nd/4.0/.
References
- Peek GJ, Mugford M, Tiruvoipati R, Wilson A, Allen E, Thalanany MM, Hibbert CL, Truesdale A, Clemens F, Cooper N, Firmin RK, Elbourne D. CESAR trial collaboration. Efficacy and economic assessment of conventional ventilatory support versus extracorporeal membrane oxygenation for severe adult respiratory failure (CESAR): a multicentre randomised controlled trial. Lancet 2009;374:1351-63. Erratum in: Lancet 2009;374:1330.
- Napp LC, Kühn C, Bauersachs J. ECMO in cardiac arrest and cardiogenic shock. Herz 2017;42:27-44. [Crossref] [PubMed]
- Jacquot A, Lepage X, Merckle L, Girerd N, Levy B. Protocol for a multicentre randomised controlled trial evaluating the effects of moderate hypothermia versus normothermia on mortality in patients with refractory cardiogenic shock rescued by venoarterial extracorporeal membrane oxygenation (VA-ECMO) (HYPO-ECMO study). BMJ Open 2019;9:e031697. [Crossref] [PubMed]
- Ostadal P, Rokyta R, Karasek J, Kruger A, Vondrakova D, Janotka M, Naar J, Smalcova J, Hubatova M, Hromadka M, Volovar S, Seyfrydova M, Jarkovsky J, Svoboda M, Linhart A, Belohlavek J. ECMO-CS Investigators. Extracorporeal Membrane Oxygenation in the Therapy of Cardiogenic Shock: Results of the ECMO-CS Randomized Clinical Trial. Circulation 2023;147:454-64. [Crossref] [PubMed]
- Schrage B, Becher PM, Bernhardt A, Bezerra H, Blankenberg S, Brunner S, et al. Left Ventricular Unloading Is Associated With Lower Mortality in Patients With Cardiogenic Shock Treated With Venoarterial Extracorporeal Membrane Oxygenation: Results From an International, Multicenter Cohort Study. Circulation 2020;142:2095-106. [Crossref] [PubMed]
- Chaturvedi RR, Macrae D, Brown KL, Schindler M, Smith EC, Davis KB, Cohen G, Tsang V, Elliott M, de Leval M, Gallivan S, Goldman AP. Cardiac ECMO for biventricular hearts after paediatric open heart surgery. Heart 2004;90:545-51. [Crossref] [PubMed]
- de Waha S, Graf T, Desch S, Fuernau G, Eitel I, Pöss J, Jobs A, Stiermaier T, Ledwoch J, Wiedau A, Lurz P, Schuler G, Thiele H. Outcome of elderly undergoing extracorporeal life support in refractory cardiogenic shock. Clin Res Cardiol 2017;106:379-85. [Crossref] [PubMed]
- Georgiopoulos G, Aimo A, Barison A, Magkas N, Emdin M, Masci PG. Imaging predictors of incident heart failure: a systematic review and meta-analysis. J Cardiovasc Med (Hagerstown) 2021;22:378-87. [Crossref] [PubMed]
- Troupis JM, Singh Pasricha S, Gunaratnam K, Nasis A, Cameron J, Seneviratne S. Cardiomyopathy and cardiac computed tomography: what the radiologist needs to know. Clin Radiol 2013;68:e49-58. [Crossref] [PubMed]
- Douraghi-Zadeh D, Logaraj A, Lazoura O, Downey K, Gill S, Finney SJ, Padley S. Extracorporeal membrane oxygenation (ECMO): Radiographic appearances, complications and imaging artefacts for radiologists. J Med Imaging Radiat Oncol 2021;65:888-95. [Crossref] [PubMed]
- Cooper LT Jr. Myocarditis. N Engl J Med 2009;360:1526-38. [Crossref] [PubMed]
- Nakamura M, Imamura T, Hida Y, Oshima A, Yokoyama S, Doi T, Fukahara K, Kinugawa K. A case of chronic myocarditis remitted by immunosuppressive and central extracorporeal membrane oxygenation therapy. J Cardiol Cases 2022;25:330-4. [Crossref] [PubMed]
- Pussadhamma B, Tipparot T, Chaosuwannakit N, Mahakkanukrauh A, Suwannaroj S, Nanagara R, Foocharoen C. Clinical Outcomes of Myocarditis after Moderate-Dose Steroid Therapy in Systemic Sclerosis: A Pilot Study. Int J Rheumatol 2020;2020:8884442. [Crossref] [PubMed]
- Kawano H, Motokawa T, Kurohama H, Okano S, Akashi R, Yonekura T, Ikeda S, Izumikawa K, Maemura K. Fulminant Myocarditis 24 Days after Coronavirus Disease Messenger Ribonucleic Acid Vaccination. Intern Med 2022;61:2319-25. [Crossref] [PubMed]
- Rao P, Khalpey Z, Smith R, Burkhoff D, Kociol RD. Venoarterial Extracorporeal Membrane Oxygenation for Cardiogenic Shock and Cardiac Arrest. Circ Heart Fail 2018;11:e004905. [Crossref] [PubMed]
- Kohler K, Valchanov K, Nias G, Vuylsteke A. ECMO cannula review. Perfusion 2013;28:114-24. [Crossref] [PubMed]
- Eckman PM, Katz JN, El Banayosy A, Bohula EA, Sun B, van Diepen S. Veno-Arterial Extracorporeal Membrane Oxygenation for Cardiogenic Shock: An Introduction for the Busy Clinician. Circulation 2019;140:2019-37. [Crossref] [PubMed]
- Mariscalco G, Salsano A, Fiore A, Dalén M, Ruggieri VG, Saeed D, et al. Peripheral versus central extracorporeal membrane oxygenation for postcardiotomy shock: Multicenter registry, systematic review, and meta-analysis. J Thorac Cardiovasc Surg 2020;160:1207-1216.e44. [Crossref] [PubMed]
- Tsangaris A, Alexy T, Kalra R, Kosmopoulos M, Elliott A, Bartos JA, Yannopoulos D. Overview of Veno-Arterial Extracorporeal Membrane Oxygenation (VA-ECMO) Support for the Management of Cardiogenic Shock. Front Cardiovasc Med 2021;8:686558. [Crossref] [PubMed]
- Aboul Nour H, Poyiadji N, Mohamed G, Alsrouji OK, Ramadan AR, Griffith B, Marin H, Chebl AB. Challenges of acute phase neuroimaging in VA-ECMO, pitfalls and alternative imaging options. Interv Neuroradiol 2021;27:434-9. [Crossref] [PubMed]
- Welbourn C, Efstathiou N. How does the length of cardiopulmonary resuscitation affect brain damage in patients surviving cardiac arrest? A systematic review. Scand J Trauma Resusc Emerg Med 2018;26:77. [Crossref] [PubMed]
- Harjola VP, Lassus J, Sionis A, Køber L, Tarvasmäki T, Spinar J, Parissis J, Banaszewski M, Silva-Cardoso J, Carubelli V, Di Somma S, Tolppanen H, Zeymer U, Thiele H, Nieminen MS, Mebazaa A. CardShock Study Investigators; GREAT network. Clinical picture and risk prediction of short-term mortality in cardiogenic shock. Eur J Heart Fail 2015;17:501-9. Erratum in: Eur J Heart Fail 2015;17:984. [Crossref] [PubMed]
- Hang W, Chen C, Seubert JM, Wang DW. Fulminant myocarditis: a comprehensive review from etiology to treatments and outcomes. Signal Transduct Target Ther 2020;5:287. [Crossref] [PubMed]
- Ginsberg F, Parrillo JE. Fulminant myocarditis. Crit Care Clin 2013;29:465-83. [Crossref] [PubMed]
- Sharma AN, Stultz JR, Bellamkonda N, Amsterdam EA. Fulminant Myocarditis: Epidemiology, Pathogenesis, Diagnosis, and Management. Am J Cardiol 2019;124:1954-60. [Crossref] [PubMed]
- Ammirati E, Veronese G, Bottiroli M, Wang DW, Cipriani M, Garascia A, Pedrotti P, Adler ED, Frigerio M. Update on acute myocarditis. Trends Cardiovasc Med 2021;31:370-9. [Crossref] [PubMed]
- Abdalghafoor T, Shoman B, Salah Omar A, Shouman Y, Almulla A. Urgent coronary artery bypass graft surgery supported by veno-arterial extracorporeal membrane oxygenation: a report of two cases. Perfusion 2022;37:633-8. [Crossref] [PubMed]
- Abdelrahman KM, Chen MY, Dey AK, Virmani R, Finn AV, Khamis RY, Choi AD, Min JK, Williams MC, Buckler AJ, Taylor CA, Rogers C, Samady H, Antoniades C, Shaw LJ, Budoff MJ, Hoffmann U, Blankstein R, Narula J, Mehta NN. Coronary Computed Tomography Angiography From Clinical Uses to Emerging Technologies: JACC State-of-the-Art Review. J Am Coll Cardiol 2020;76:1226-43. [Crossref] [PubMed]
- Kang KM, Choi SI, Chun EJ, Kim JA, Youn TJ, Choi DJ. Coronary vasospastic angina: assessment by multidetector CT coronary angiography. Korean J Radiol 2012;13:27-33. [Crossref] [PubMed]
- Slavich M, Florian A, Bogaert J. The emerging role of magnetic resonance imaging and multidetector computed tomography in the diagnosis of dilated cardiomyopathy. Insights Imaging 2011;2:453-69. [Crossref] [PubMed]
- Andreini D, Pontone G, Pepi M, Ballerini G, Bartorelli AL, Magini A, Quaglia C, Nobili E, Agostoni P. Diagnostic accuracy of multidetector computed tomography coronary angiography in patients with dilated cardiomyopathy. J Am Coll Cardiol 2007;49:2044-50. [Crossref] [PubMed]
- Mankad P, Mitchell B, Birnie D, Kron J. Cardiac Sarcoidosis. Curr Cardiol Rep 2019;21:152. [Crossref] [PubMed]
- Shen J, Tse JR, Chan F, Fleischmann D. CT Angiography of Venoarterial Extracorporeal Membrane Oxygenation. Radiographics 2022;42:23-37. [Crossref] [PubMed]
- Honore PM, Barreto Gutierrez L, Kugener L, Redant S, Attou R, Gallerani A, De Bels D. Risk of harlequin syndrome during bi-femoral peripheral VA-ECMO: should we pay more attention to the watershed or try to change the venous cannulation site? Crit Care 2020;24:450. [Crossref] [PubMed]
- Hoeper MM, Tudorache I, Kühn C, Marsch G, Hartung D, Wiesner O, Boenisch O, Haverich A, Hinrichs J. Extracorporeal membrane oxygenation watershed. Circulation 2014;130:864-5. [Crossref] [PubMed]
- Prabhu S, Shanmugasundaram B, Shetty R, Rao S, Karl TR. Harlequin effect and central veno-arterial extracorporeal life support. Cardiol Young 2023;33:2181-4. [Crossref] [PubMed]
- Nezami FR, Khodaee F, Edelman ER, Keller SP. A Computational Fluid Dynamics Study of the Extracorporeal Membrane Oxygenation-Failing Heart Circulation. ASAIO J 2021;67:276-83. [Crossref] [PubMed]
- Buchtele N, Staudinger T, Schwameis M, Schörgenhofer C, Herkner H, Hermann AUltraECMO investigators. Feasibility and safety of watershed detection by contrast-enhanced ultrasound in patients receiving peripheral venoarterial extracorporeal membrane oxygenation: a prospective observational study. Crit Care 2020;24:126. [Crossref] [PubMed]
- Schmidt B, Flohr T. Principles and applications of dual source CT. Phys Med 2020;79:36-46. [Crossref] [PubMed]
- Tang PH, Du BJ, Fang XM, Hu XY, Qian PY, Gao QS. Submillisievert coronary CT angiography with adaptive prospective ECG-triggered sequence acquisition and iterative reconstruction in patients with high heart rate on the dual-source CT. J Xray Sci Technol 2016;24:807-20. [Crossref] [PubMed]
- Miller RJH, Eisenberg E, Friedman J, Cheng V, Hayes S, Tamarappoo B, Thomson L, Berman DS. Impact of heart rate on coronary computed tomographic angiography interpretability with a third-generation dual-source scanner. Int J Cardiol 2019;295:42-7. [Crossref] [PubMed]