The relationship between glycemic risk and longitudinal changes in total physiological atherosclerotic burden in patients with coronary artery disease
Introduction
In recent decades, the functional assessment of coronary stenosis by using invasive fractional flow reserve (FFR) has been widely adopted in clinical practice, with substantial evidence supporting the benefits of coronary physiology-guided revascularization in patients with ischemic heart disease (1,2). However, FFR has several limitations, including increased procedural time and cost, the need to rely on pressure wires, and the need to induce hyperemia with pharmacological agents (3,4). The quantitative flow ratio (QFR), derived from three-dimensional (3D) coronary artery reconstruction and fluid dynamics computations from the routine angiogram, has emerged as an alternative approach to evaluate the functional significance of coronary stenosis, exhibiting a comparable diagnostic performance with invasive FFR (5,6). Additionally, recent studies have revealed that the sum of FFR or QFR values in the three major coronary arteries (left anterior descending artery, left circumflex artery, and right coronary artery) can serve as a surrogate marker of total physiological atherosclerotic burden, providing improved risk stratification for patients with coronary artery disease (CAD) (7,8).
It is widely recognized that diabetes mellitus (DM) is strongly associated with an increased risk of atherosclerotic cardiovascular disease (9). Furthermore, previous studies have indicated that intensive glycemic control can reduce the risk of CAD and adverse cardiovascular events (10). However, the interplay between glycemic status and the coronary physiology has not been well-investigated. A recent prospective pilot study reported that alogliptin, a dipeptidyl peptidase-4 inhibitor, could not lead to improvements in coronary physiology as assessed by FFR derived from coronary computed tomography angiography (CTA). It is important to note that the sample size of the study was relatively small, and there were no significant changes in glycemic control levels, as indicated by glycosylated hemoglobin (HbA1c), following alogliptin treatment (11). Therefore, the current study aimed to investigate the relationship between diabetes, glycemic control status, and the progression of physiologic atherosclerotic burden through serial QFR measurements in the three major coronary arteries. We present this article in accordance with the STROBE reporting checklist (available at https://qims.amegroups.com/article/view/10.21037/qims-23-1160/rc).
Methods
Study population
This retrospective analysis included patients who underwent serial coronary angiography (CAG) at interval of ≥6 months between January 2016 and December 2021 at Tongji Hospital of Tongji University. A total of 1,043 patients who had visible plaques with percent diameter stenosis between 20% and 90% in all 3 major epicardial arteries were screened. Patients were excluded if they met any of the following criteria: (I) previous or planned coronary artery bypass graft surgery (n=23); (II) acute myocardial infarction (MI) within 72 hours at baseline, or patients who experienced adverse cardiac events between the two CAG measurements (n=125); (III) incomplete clinical information or laboratory data (n=116); (IV) significant left main disease, ostial lesions, or diminutive coronary artery (n=86); and (V) insufficient angiographic imaging quality or other reasons rendering them unsuitable for QFR analysis (n=163). Finally, a total of 530 patients who had undergone successful serial QFR measurements at both the index and follow-up procedures were enrolled. This study was conducted in accordance with the Declaration of Helsinki (as revised in 2013). The study was reviewed and approved by the Institutional Review Board of Tongji Hospital, Tongji University (No. K-W-2020-016), and the requirement for written informed consent was waived due to the retrospective nature of the study.
Clinical data collection and definition of DM
Baseline demographics and laboratory parameters of all patients were obtained by reviewing the hospital database. The doctors who collected the clinical data were blinded to the angiographic parameters. The diagnosis of DM was determined based on HbA1c or fasting plasma glucose level, and an oral glucose tolerance test was performed if still in doubt. A diagnosis of DM was defined by meeting one of the following criteria: (I) previous physician-assigned DM diagnosis; (II) current use of hypoglycemic medications; (III) HbA1c level measured during index hospitalization ≥6.5%; (IV) fasting plasma glucose ≥7.0 mmol/L or 2-hour plasma glucose of oral glucose tolerance test ≥11.1 mmol/L (12). Among patients with DM, we further categorized those with a follow-up HbA1c level of <7.0% as optimal glycemic control (13).
Angiographic and QFR assessments
Invasive CAG and percutaneous coronary intervention (PCI) were performed according to the standard of care on the basis of local practice. The Gensini score, a specific angiographic scoring system for quantifying the anatomical severity of CAD, was calculated based on baseline and follow-up angiograms (14). Murray law-based QFR (µQFR) analysis was blindly and independently conducted by experienced analysts with more than 3 years of experience in QFR analysis using the QFR system software (AngioPlus Core; Pulse Medical Imaging Technology, Shanghai, China), as previously described (15). In brief, a key frame with the clearest visualization of coronary stenosis was selected and transferred to the QFR system. The lumen contour of the interrogated vessel and its side branches with diameters ≥1 mm were automatically delineated, and the reference diameter function was reconstructed according to the Murray fractal law (16). Manual correction of the lumen contour was applied when necessary. Subsequently, the µQFR value was automatically computed for both major epicardial arteries and their side branches. The QFR computations were based on the contrast flow model, utilizing calculations of contrast flow velocity and pressure drop derived from fluid dynamics equations (15,17). QFR measurements were recorded for all three major coronary arteries (left anterior descending artery, left circumflex artery, and right coronary artery) at both index and follow-up procedures. We calculated 3-vessel QFR (3V-QFR) as the sum of the µQFR values measured in the three major coronary arteries. If a PCI was performed during the index procedure, the post-PCI QFR value was used to calculate 3V-QFR (7). The change in 3V-QFR (Δ3V-QFR) was defined as the follow-up 3V-QFR minus the baseline 3V-QFR. Based on pervious report, comparisons in terms of long-term clinical outcomes were made using a cutoff value of −0.05 for serial change in 3V-QFR value (18). In the present study, we defined functional progression of CAD as a Δ3V-QFR value ≤−0.05.
Statistical analysis
Continuous variables are presented as median with interquartile range (25% to 75%), and compared between groups using Wilcoxon signed rank test, Mann-Whitney U test, or Kruskal-Wallis H test as appropriate. Categorical variables were summarized as absolute counts and percentages, and compared between groups using Pearson’s chi-square or Fisher’s exact test. Logistic regression analysis was used to identify the independent predictors of coronary functional progression. Adjustments were made for non-modifiable factors including age, sex, interval time between the two CAG measurements, as well as variables with a P value <0.05 in the univariable model. Odds ratio (OR) with corresponding 95% confidence interval (CI) were reported for each unit increase in continuous HbA1c level and for categorical variables based on HbA1c tertiles. To assess the linear relationship between HbA1c tertiles and functional progression, P values for trends were calculated across the ordered follow-up HbA1c tertiles (19). Receiver operating characteristic (ROC) curve analysis was performed to determine the cut-off value of HbA1c for functional progression. We performed the subgroup analyses of participants according to the diabetic status. The ROC curve analysis was also utilized to assess the predictive performance for functional progression in patients with and without DM. A two-tailed P value <0.05 was considered statistically significant. All statistical analyses were performed using SPSS version 26.0 (IBM Corp., Armonk, NY, USA).
Results
Patient characteristics
A total of 530 patients who met all clinical and imaging enrollment criteria were included in the study (Figure 1). The median time interval between the two CAG measurements was 12.1 (10.6, 14.3) months. Among the full patient cohort, the median age was 65 [59, 72] years, 380 (71.7%) were male, and 219 (41.3%) had diabetes. The patient characteristics stratified by Δ3V-QFR levels are described in Table 1. One hundred and sixty-nine (31.9%) patients showed functional progression (Δ3V-QFR ≤−0.05) during the follow-up. The median Δ3V-QFR was −0.09 (−0.13, 0.06) for patients with functional progression and 0 (−0.02, 0.02) for those with preserved physiological status. Patients with coronary functional progression had a higher prevalence of prior MI (27.2% vs. 18.3%, P=0.019), and multivessel disease (66.3% vs. 57.1%, P=0.044) compared with the non-progression group. There were no differences in other characteristics between the two groups, including the statin use at both baseline and follow-up (all P>0.05).
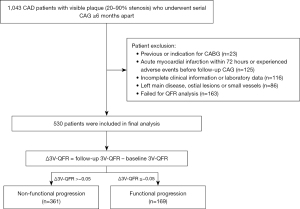
Table 1
Variables | Total (n=530) | Δ3V-QFR >−0.05 (n=361) | Δ3V-QFR ≤−0.05 (n=169) | P value |
---|---|---|---|---|
Δ3V-QFR | −0.02 [−0.06, 0.01] | 0 [−0.02, 0.02] | −0.09 [−0.13, 0.06] | <0.001 |
Inter-CAG period (months) | 12.1 [10.6, 14.3] | 12.1 [10.5, 14.3] | 12.2 [11.0, 14.2] | 0.575 |
Age (years) | 65 [59, 72] | 65 [59, 72] | 66 [60, 72] | 0.249 |
Male | 380 (71.7) | 256 (70.9) | 124 (73.4) | 0.558 |
Body mass index (kg/m2) | 24.8 [22.5, 26.6] | 24.7 [22.4, 26.8] | 25.1 [22.6, 26.9] | 0.277 |
Current or former smoker | 285 (53.8) | 188 (52.1) | 97 (57.4) | 0.252 |
Clinical presentation | 0.929 | |||
Asymptomatic ischemia | 68 (12.8) | 48 (13.3) | 20 (11.8) | |
Stable angina | 211 (39.8) | 144 (39.9) | 67 (39.6) | |
Unstable angina | 198 (37.4) | 132 (36.6) | 66 (39.1) | |
Post-MI within 1 month | 53 (10.0) | 37 (10.2) | 16 (9.5) | |
DM | 219 (41.3) | 140 (38.8) | 79 (46.7) | 0.083 |
Hypertension | 375 (70.8) | 249 (69.0) | 126 (74.6) | 0.188 |
Hyperlipidemia | 306 (57.7) | 201 (55.7) | 105 (62.1) | 0.161 |
Previous MI | 112 (21.1) | 66 (18.3) | 46 (27.2) | 0.019 |
Previous stroke | 52 (9.8) | 34 (9.4) | 18 (10.7) | 0.657 |
Previous or index PCI | 476 (89.8) | 328 (90.0) | 148 (87.6) | 0.244 |
Multivessel disease | 318 (60.0) | 206 (57.1) | 112 (66.3) | 0.044 |
Medications at baseline CAG | ||||
Antiplatelet agent | 526 (99.2) | 359 (99.4) | 167 (98.8) | 0.596 |
Statin use | 517 (97.5) | 351 (97.2) | 166 (98.2) | 0.763 |
Insulin use | 48 (9.1) | 28 (7.8) | 20 (11.8) | 0.127 |
Medications at follow-up CAG | ||||
Antiplatelet agent | 515 (97.1) | 351 (97.2) | 164 (97.0) | >0.999 |
Statin use | 520 (98.1) | 352 (97.5) | 168 (99.4) | 0.181 |
Insulin use | 53 (10.0) | 30 (8.3) | 23 (13.6) | 0.058 |
Data are expressed as n (%) or median [25th, 75th percentiles]. 3V-QFR, 3-vessel quantitative flow ratio; CAG, coronary angiography; MI, myocardial infarction; DM, diabetes mellitus; PCI, percutaneous coronary intervention.
Table 2 presents the baseline and follow-up laboratory tests and angiographic findings. The follow-up levels of high sensitivity C-reactive protein, total cholesterol, triglycerides, and low-density lipoprotein cholesterol (LDL-C) were significantly decreased compared to baseline (all P<0.001), whereas the follow-up HbA1c was slightly higher than baseline [6.3 (5.9, 7.0) vs. 6.2 (5.8, 6.9), P=0.002]. No significant changes were observed in estimated glomerular filtration rate and high-density lipoprotein cholesterol (HDL-C) between baseline and follow-up levels. At follow-up, Gensini score values were significantly higher [23.5 (16.0, 31.5) vs. 21.5 (15.5, 29.0), P<0.001], whereas 3V-QFR values were significantly lower than baseline [2.84 (2.75, 2.89) vs. 2.86 (2.79, 2.90), P<0.001], indicating consistent progression in anatomic and functional severity.
Table 2
Variables | Baseline | Follow-up | Change from baseline | P value |
---|---|---|---|---|
eGFR (mL/min/1.73 m2) | 83.7 (71.1, 96.0) | 84.4 (72.0, 97.9) | 1.1 (−8.7, 11.9) | 0.070 |
hs-CRP (mg/L) | 1.84 (0.81, 3.81) | 1.09 (0.41, 2.44) | −0.37 (−2.14, −0.01) | <0.001 |
HbA1c (%) | 6.2 (5.8, 6.9) | 6.3 (5.9, 7.0) | 0.1 (−0.2, 0.3) | 0.002 |
Total cholesterol (mmol/L) | 4.23 (3.52, 4.92) | 3.40 (2.90, 3.99) | −0.70 (−1.56, −0.04) | <0.001 |
Triglycerides (mmol/L) | 1.34 (0.99, 1.95) | 1.16 (0.87, 1.69) | −0.14 (−0.52, 0.13) | <0.001 |
HDL-C (mmol/L) | 1.01 (0.88, 1.19) | 1.03 (0.86, 1.21) | 0.01 (−0.11, 0.12) | 0.310 |
LDL-C (mmol/L) | 2.79 (2.18, 3.34) | 2.05 (1.68, 2.54) | −0.53 (−1.29, −0.01) | <0.001 |
Gensini score | 21.5 (15.5, 29.0) | 23.5 (16.0, 31.5) | 1.0 (0, 3.0) | <0.001 |
3V-QFR | 2.86 (2.79, 2.90) | 2.84 (2.75, 2.89) | −0.02 (−0.06, 0.01) | <0.001 |
Data are expressed as median (25th, 75th percentiles). eGFR, estimated glomerular filtration rate; hs-CRP, high sensitivity C-reactive protein; HbA1c, glycosylated hemoglobin; HDL-C, high-density lipoprotein cholesterol; LDL-C, low-density lipoprotein cholesterol; 3V-QFR, 3-vessel quantitative flow ratio.
Associate between glycemic status and coronary functional progression
The results of univariate and multivariate logistic regression analyses are shown in Table S1. The univariate analysis indicated that the follow-up level of HbA1c (OR, 1.338; 95% CI: 1.151–1.555; P<0.001), but not the history of DM (OR, 1.386; 95% CI: 0.958–2.004; P=0.083), was a potential factor associated with accelerated functional progression of CAD. Furthermore, after adjustment for age, sex, interval time between CAG measurements, and significant variables in univariable model (including previous MI, multivessel disease, follow-up levels of triglycerides, and LDL-C), the on-treatment HbA1c level remained a strong determinant of coronary functional progression (OR, 1.263; 95% CI: 1.078–1.479; P=0.004).
Associations between serum HbA1c level and coronary functional progression in patients with or without DM
Univariate and multivariate logistic regression models were performed to investigate the correlations between follow-up HbA1c level and disease progression in subgroups with or without DM. In the non-DM group, the follow-up HbA1c level was found not to be a significant predictor for coronary functional progression in the fully adjusted model (OR, 1.654; 95% CI: 0.857–3.192; P=0.133). However, in the DM group, the follow-up HbA1c (each 1% increase) was independently associated with higher risk of functional progression as a continuous variable (OR, 1.353; 95% CI: 1.082–1.693; P=0.008). After fully adjusting for confounding risk factors, an increased serum level of follow-up HbA1c (tertile 3) was associated with an increased risk of functional progression (P for trend =0.032). Patients in the highest tertile of follow-up HbA1c had a significantly higher risk of functional progression (OR, 2.661; 95% CI: 1.281–5.526; P=0.009) (Table 3).
Table 3
Groups | Tertile 1 | Tertile 2 | Tertile 3 | Ptrend | 1% increase | P value |
---|---|---|---|---|---|---|
Overall (n=530) | ||||||
Unadjusted | 1.0 | 1.297 (0.818–2.057) | 2.275 (1.439–3.599) | 0.001 | 1.338 (1.151–1.555) | <0.001 |
Model 1 | 1.0 | 1.268 (0.796–2.019) | 2.229 (1.405–3.535) | 0.002 | 1.336 (1.149–1.554) | <0.001 |
Model 2 | 1.0 | 1.161 (0.719–1.874) | 1.879 (1.160–3.043) | 0.025 | 1.263 (1.078–1.479) | 0.004 |
Non-DM (n=311) | ||||||
Unadjusted | 1.0 | 1.099 (0.613–1.969) | 1.998 (1.079–3.699) | 0.067 | 1.924 (1.017–3.641) | 0.044 |
Model 1 | 1.0 | 1.066 (0.593–1.917) | 1.959 (1.036–3.704) | 0.088 | 1.920 (1.006–3.664) | 0.048 |
Model 2 | 1.0 | 1.079 (0.593–1.963) | 1.703 (0.882–3.286) | 0.255 | 1.654 (0.857–3.192) | 0.133 |
DM (n=219) | ||||||
Unadjusted | 1.0 | 1.890 (0.931–3.837) | 2.849 (1.434–5.658) | 0.011 | 1.381 (1.119–1.704) | 0.003 |
Model 1 | 1.0 | 1.845 (0.903–3.773) | 2.901 (1.456–5.782) | 0.010 | 1.383 (1.120–1.708) | 0.003 |
Model 2 | 1.0 | 1.761 (0.828–3.746) | 2.661 (1.281–5.526) | 0.032 | 1.353 (1.082–1.693) | 0.008 |
Data are expressed as OR (95% CI). Laboratory variables were measured at follow-up CAG. Model 1 adjusted for age, sex, and inter-CAG period; model 2 adjusted for all factors in model 1 plus clinical factors with P<0.05 in univariable analysis (previous MI, multivessel disease, follow-up levels of triglyceride, LDL-C and HbA1c). HbA1c, glycosylated hemoglobin; DM, diabetes mellitus; OR, odds ratio; CI, confidence interval; CAG, coronary angiography; MI, myocardial infarction; LDL-C, low-density lipoprotein cholesterol.
ROC analysis demonstrated that follow-up HbA1c had a good diagnostic value in discriminating patients with functional progression from those without progression [area under the curve (AUC), 0.599; 95% CI: 0.546–0.651; P<0.001] in the entire cohort (Figure 2A). Similar diagnostic value of AUC was observed in subgroup analysis for DM patients (AUC, 0.630; 95% CI: 0.554–0.707; P=0.001), with a best cut-off value of 7.15%. However, there was no significant diagnostic value observed in non-DM patients (AUC, 0.571; 95% CI: 0.499–0.643; P=0.054) (Figure 2B). Subgroup analysis further revealed that DM patients without optimal glycemic control (HbA1c ≥7.0%) had significantly lower Δ3V-QFR values compared to DM patients with optimal glycemic control (HbA1c <7.0%) or non-DM patients [−0.03 (−0.09, 0) vs. −0.02 (−0.05, 0.01) vs. −0.02 (−0.05, 0.02); overall P=0.002). However, no significant difference in the Δ3V-QFR levels was observed between DM patients with optimal glycemic control and non-DM patients (P>0.05) (Figure 3).
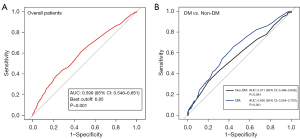
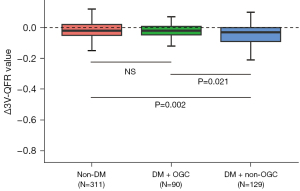
Discussion
To the best of our knowledge, this is the first study to utilize serial angiography-derived QFR to explore the impact of glycemic status on physiological progression of atherosclerotic disease. Our major findings indicate that on-treatment HbA1c, rather than history of DM, was an independent predictor of coronary functional progression even after adjusting for confounding risk factors. Notably, we observed that DM patients who achieved the optimal HbA1c target (<7.0%) showed slower functional progression compared to those with poor glycemic control.
Over the last few decades, atherosclerosis has been widely recognized as a systematic disease presented with a chronic and progressive process, with plaque progression representing a necessary but modifiable step between subclinical atherosclerosis and acute clinical events (20). Consequently, there has been growing interest in studying the natural evolution of coronary atherosclerosis and assessing changes in plaque volume and composition in response to medical therapy by using invasive intracoronary imaging or noninvasive coronary CTA (21,22). Although coronary physiology testing has emerged as a valuable approach in the evaluation of lesion severity and in the management of CAD patients, the clinical implications of coronary physiological changes have not been extensively studied. A previous study described a slow longitudinal physiological progression, as evaluated by per-vessel FFR, with a median decrease of 0.007 per year (23). In the present study, we utilized serial changes in QFR values across all three major coronary arteries as a marker of progression in total physiologic atherosclerotic burden, giving the previous evidence supporting the prognostic value of 3-vessel physiological assessments in CAD patients (7,8,24). Our results demonstrated a similarly slow decline in coronary physiology at patient level, as quantified by a median Δ3V-QFR of −0.02 during 1-year follow-up (Table 2), aligning with the previous serial FFR study (23,25). Notably, QFR is a novel physiological index derived from routine CAG. It holds a significant advantage by reducing the cost, time, and potential risk associated with placing a pressure wire into a coronary artery when compared to the invasive FFR (3). As a result, this image-based computational QFR bears the potential to facilitate the wider adoption of physiological assessments in the catheterization laboratory, particularly in the context of monitoring the functional progression of atherosclerotic disease.
Plaque progression of coronary atherosclerotic disease can be influenced by multiple clinical factors. In the present study, we observed that prior history of MI, follow-up levels of serum triglycerides, LDL-C, and HbA1c were independently associated with an increased risk of functional progression after adjusting for confounders (Table S1). These findings highlight the potential benefits of optimal control of blood lipid and glucose levels in improving coronary physiology. Several studies have demonstrated the relationship between LDL-C control and functional progression, with evidence suggesting that achievement of optimal LDL-C levels can lead to improvements in FFR or QFR values in patients with CAD (25-27). In line with these studies, our results also showed that coronary physiological burden could be modified by achieving optimal levels of LDL-C. In addition to LDL-C, serum triglyceride, a biomarker for triglyceride-rich lipoproteins, has been considered as a marker of residual cardiovascular risk. Previous studies have shown that elevated triglyceride levels are associated with a higher risk of atherosclerotic cardiovascular disease events in patients receiving statin therapy (28,29). A recent trial involving 47 patients with elevated fasting triglyceride levels demonstrated that a high dose of icosapent ethyl could improve coronary physiology. However, the treatment of icosapent ethyl did not lead to a significant difference in the reduction of triglyceride levels compared to the control group (30). In the present study, we observed an association between follow-up triglyceride levels and coronary physiological progression assessed by seral QFR measurements. Hence, future large-scale prospective studies utilizing serial coronary physiological measurements are warranted to further understand the relationship between the functional progression and residual cardiovascular risk. Nevertheless, these results suggest that monitoring serial changes in 3V-QFR could serve as a surrogate marker for assessing the response to medical therapies in patients with CAD.
Diabetes is a well-established risk factor for cardiovascular atherosclerotic disease and subsequent adverse events. Previous studies have reported the relationship between DM and rapid progression in atheroma volume or coronary calcification (31-33). However, these studies did not consider the glycemic control status of DM patients, and several studies have shown that plaque progression and clinical events can be prevented through glycemic control as indicated by serum HbA1c levels (10,34,35). A recent meta-analysis of cardiovascular outcome trials involving 40,346 patients revealed that an intensive glucose-lowering approach resulted in a 14% reduction in the risk of major adverse cardiovascular events compared with conventional treatment. Additionally, higher HbA1c values during the first year of diabetes diagnosis were associated with an increased risk of adverse cardiovascular events (36). In the current study, we demonstrated that glycemic status as reflected by follow-up HbA1c levels is an independent risk factor of coronary functional progression, whereas diagnosis of DM was not significantly associated with functional progression (Table 3). Importantly, in patients with DM, an optimal HbA1c goal achievement leads to physiological improvement (Figure 3). These results are consistent with prior imaging studies that utilized serial coronary CTA or intravascular ultrasound (IVUS) measurements to assess the relationship between coronary atheroma progression and glycemic control level (34,37,38). In the prospective PERISCOPE (Pioglitazone Effect on Regression of Intravascular Sonographic Coronary Obstruction Prospective Evaluation) trial, pioglitazone treatment, compared with glimepiride, resulted in a significantly greater reduction in HbA1c levels and was associated with a lower rate of plaque progression (37). Furthermore, several observational studies have reported that individuals with higher HbA1c levels exhibited a greater plaque progression incidence (34,38). Our results, together with the aforementioned studies, support the beneficial effects of intensive glycemic control in halting coronary plaque progression. These findings emphasize the importance of closely monitoring of glycemic status and the clinical necessity for intensive medical therapies to manage residual glycemic risk in patients with CAD, particularly for those with uncontrolled diabetes.
Several limitations of the present study should be considered. First, in this single-center, retrospective observational study, selection bias might have been introduced due to the rigorous inclusion and exclusion criteria. Second, invasive pressure wire-based FFR, which is considered the gold standard for coronary physiological measurement, was not acquired in this study. However, angiography-derived QFR has been extensively validated and bears promising potential for clinical application (5,39). Third, a large proportion of the patients underwent PCI in at least one coronary artery. Therefore, our data may not accurately reflect the natural history of the physiological progression of atherosclerosis disease. Further prospective studies are required to address this issue among CAD patients receiving medication treatments. Fourth, the AUC of follow-up HbA1c levels for predicting coronary functional progression was relatively low, possibly due to the small sample size, limiting its clinical significance for detecting plaque progression by monitoring glycemic status. In fact, the underlying mechanisms of plaque progression are complex and involve multiple drivers (40). Future large-scale studies are needed to develop a more robust predictive model by incorporating various risk factors. Lastly, due to the observational nature of our study, the medications for dyslipidemia, diabetes, and longitudinal HbA1c control levels between CAG assessments were not available. Thus, we were unable to adjust these potential residual confounders that may contribute to plaque progression. Future prospective studies are needed to explore the effects of glycemic control with medication therapies on the functional progression of CAD.
Conclusions
In patients with established CAD, higher on-treatment HbA1c levels were independently associated with an accelerated coronary functional progression rate as assessed by serial 3V-QFR measurements. Patients with DM who achieved the HbA1c target had a slower rate of functional progression than those with poor glycemic control. These findings highlight the importance of closely monitoring of glycemic status in clinical practice and support the need to modulate glycemic risk to prevent disease progression and subsequent atherosclerotic events.
Acknowledgments
Funding: This study was supported by
Footnote
Reporting Checklist: The authors have completed the STROBE reporting checklist. Available at https://qims.amegroups.com/article/view/10.21037/qims-23-1160/rc
Conflicts of Interest: All authors have completed the ICMJE uniform disclosure form (available at https://qims.amegroups.com/article/view/10.21037/qims-23-1160/coif). X.L. reports grant support from the National Natural Science Foundation of China (No. 82170346) and the Shanghai Municipal Health Commission (No. 2019LJ10). F.C. reports grant support from the Shanghai Science and Technology Committee (No. 22Y11909800). J.C. reports grant support from the China Scholarship Council (No. 202206260277). The other authors have no conflicts of interest to declare.
Ethical Statement: The authors are accountable for all aspects of the work, including ensuring that any questions related to the accuracy or integrity of any part of the work have been appropriately investigated and resolved. This study was conducted in accordance with the Declaration of Helsinki (as revised in 2013). The study was reviewed and approved by the Institutional Review Board of Tongji Hospital, Tongji University (No. K-W-2020-016), and the requirement for written informed consent was waived due to the retrospective nature of the study.
Open Access Statement: This is an Open Access article distributed in accordance with the Creative Commons Attribution-NonCommercial-NoDerivs 4.0 International License (CC BY-NC-ND 4.0), which permits the non-commercial replication and distribution of the article with the strict proviso that no changes or edits are made and the original work is properly cited (including links to both the formal publication through the relevant DOI and the license). See: https://creativecommons.org/licenses/by-nc-nd/4.0/.
References
- Johnson NP, Tóth GG, Lai D, Zhu H, Açar G, Agostoni P, et al. Prognostic value of fractional flow reserve: linking physiologic severity to clinical outcomes. J Am Coll Cardiol 2014;64:1641-54. [Crossref] [PubMed]
- Tonino PA, De Bruyne B, Pijls NH, Siebert U, Ikeno F, van't Veer M, Klauss V, Manoharan G, Engstrøm T, Oldroyd KG, Ver Lee PN, MacCarthy PA, Fearon WFFAME Study Investigators. Fractional flow reserve versus angiography for guiding percutaneous coronary intervention. N Engl J Med 2009;360:213-24. [Crossref] [PubMed]
- De Maria GL, Garcia-Garcia HM, Scarsini R, Hideo-Kajita A, Gonzalo López N, Leone AM, et al. Novel Indices of Coronary Physiology: Do We Need Alternatives to Fractional Flow Reserve? Circ Cardiovasc Interv 2020;13:e008487. [Crossref] [PubMed]
- Chu J, Lin H, Yan W, Yuan D, Lai Y, Liu X. Angiographic quantitative flow ratio in acute coronary syndrome: beyond a tool to define ischemia-causing stenosis-a literature review. Cardiovasc Diagn Ther 2022;12:892-907. [Crossref] [PubMed]
- Xu B, Tu S, Qiao S, Qu X, Chen Y, Yang J, Guo L, Sun Z, Li Z, Tian F, Fang W, Chen J, Li W, Guan C, Holm NR, Wijns W, Hu S. Diagnostic Accuracy of Angiography-Based Quantitative Flow Ratio Measurements for Online Assessment of Coronary Stenosis. J Am Coll Cardiol 2017;70:3077-87. [Crossref] [PubMed]
- Westra J, Tu S, Winther S, Nissen L, Vestergaard MB, Andersen BK, et al. Evaluation of Coronary Artery Stenosis by Quantitative Flow Ratio During Invasive Coronary Angiography: The WIFI II Study (Wire-Free Functional Imaging II). Circ Cardiovasc Imaging 2018;11:e007107. [Crossref] [PubMed]
- Lee JM, Koo BK, Shin ES, Nam CW, Doh JH, Hwang D, et al. Clinical implications of three-vessel fractional flow reserve measurement in patients with coronary artery disease. Eur Heart J 2018;39:945-51. [Crossref] [PubMed]
- Hamaya R, Hoshino M, Kanno Y, Yamaguchi M, Ohya H, Sumino Y, Kanaji Y, Usui E, Murai T, Lee T, Yonetsu T, Hirao K, Kakuta T. Prognostic implication of three-vessel contrast-flow quantitative flow ratio in patients with stable coronary artery disease. EuroIntervention 2019;15:180-8. [Crossref] [PubMed]
- Huxley R, Barzi F, Woodward M. Excess risk of fatal coronary heart disease associated with diabetes in men and women: meta-analysis of 37 prospective cohort studies. BMJ 2006;332:73-8. [Crossref] [PubMed]
- Hayward RA, Reaven PD, Wiitala WL, Bahn GD, Reda DJ, Ge L, McCarren M, Duckworth WC, Emanuele NV. VADT Investigators. Follow-up of glycemic control and cardiovascular outcomes in type 2 diabetes. N Engl J Med 2015;372:2197-206. [Crossref] [PubMed]
- Nozue T, Takamura T, Fukui K, Sozu T, Tanaka Y, Hibi K, Kishi S, Michishita I. Changes in coronary atherosclerosis, composition, and fractional flow reserve evaluated by coronary computed tomography angiography in patients with type 2 diabetes. Int J Cardiol Heart Vasc 2018;19:46-51. [Crossref] [PubMed]
- American Diabetes Association. 2. Classification and Diagnosis of Diabetes: Standards of Medical Care in Diabetes-2019. Diabetes Care 2019;42:S13-28. [Crossref] [PubMed]
- ElSayed NA, Aleppo G, Aroda VR, Bannuru RR, Brown FM, Bruemmer D, et al. 6. Glycemic Targets: Standards of Care in Diabetes-2023. Diabetes Care 2023;46:S97-S110. [Crossref] [PubMed]
- Gensini GG. A more meaningful scoring system for determining the severity of coronary heart disease. Am J Cardiol 1983;51:606. [Crossref] [PubMed]
- Tu S, Ding D, Chang Y, Li C, Wijns W, Xu B. Diagnostic accuracy of quantitative flow ratio for assessment of coronary stenosis significance from a single angiographic view: A novel method based on bifurcation fractal law. Catheter Cardiovasc Interv 2021;97:1040-7. [Crossref] [PubMed]
- Tu S, Echavarria-Pinto M, von Birgelen C, Holm NR, Pyxaras SA, Kumsars I, Lam MK, Valkenburg I, Toth GG, Li Y, Escaned J, Wijns W, Reiber JH. Fractional flow reserve and coronary bifurcation anatomy: a novel quantitative model to assess and report the stenosis severity of bifurcation lesions. JACC Cardiovasc Interv 2015;8:564-74. [Crossref] [PubMed]
- Tu S, Westra J, Yang J, von Birgelen C, Ferrara A, Pellicano M, Nef H, Tebaldi M, Murasato Y, Lansky A, Barbato E, van der Heijden LC, Reiber JHC, Holm NR, Wijns WFAVOR Pilot Trial Study Group. Diagnostic Accuracy of Fast Computational Approaches to Derive Fractional Flow Reserve From Diagnostic Coronary Angiography: The International Multicenter FAVOR Pilot Study. JACC Cardiovasc Interv 2016;9:2024-35. [Crossref] [PubMed]
- Chu J, Yuan D, Lai Y, Ye W, Liu L, Lin H, Ping F, Zhu G, Chen F, Yao Y, Yan W, Liu X. Prognostic Implications of Changes in Total Physiological Atherosclerotic Burden in Patients With Coronary Artery Disease-A Serial QFR Study. Angiology 2023; Epub ahead of print. [Crossref]
- Hagström E, Steg PG, Szarek M, Bhatt DL, Bittner VA, Danchin N, Diaz R, Goodman SG, Harrington RA, Jukema JW, Liberopoulos E, Marx N, McGinniss J, Manvelian G, Pordy R, Scemama M, White HD, Zeiher AM, Schwartz GGODYSSEY OUTCOMES Investigators. Apolipoprotein B, Residual Cardiovascular Risk After Acute Coronary Syndrome, and Effects of Alirocumab. Circulation 2022;146:657-72. [Crossref] [PubMed]
- Ahmadi A, Argulian E, Leipsic J, Newby DE, Narula J. From Subclinical Atherosclerosis to Plaque Progression and Acute Coronary Events: JACC State-of-the-Art Review. J Am Coll Cardiol 2019;74:1608-17. [Crossref] [PubMed]
- Nicholls SJ, Hsu A, Wolski K, Hu B, Bayturan O, Lavoie A, Uno K, Tuzcu EM, Nissen SE. Intravascular ultrasound-derived measures of coronary atherosclerotic plaque burden and clinical outcome. J Am Coll Cardiol 2010;55:2399-407. [Crossref] [PubMed]
- Motoyama S, Ito H, Sarai M, Kondo T, Kawai H, Nagahara Y, Harigaya H, Kan S, Anno H, Takahashi H, Naruse H, Ishii J, Hecht H, Shaw LJ, Ozaki Y, Narula J. Plaque Characterization by Coronary Computed Tomography Angiography and the Likelihood of Acute Coronary Events in Mid-Term Follow-Up. J Am Coll Cardiol 2015;66:337-46. [Crossref] [PubMed]
- Xaplanteris P, Ntalianis A, De Bruyne B, Strisciuglio T, Pellicano M, Ciccarelli G, Milkas A, Barbato E. Coronary lesion progression as assessed by fractional flow reserve (FFR) and angiography. EuroIntervention 2018;14:907-14. [Crossref] [PubMed]
- Fournier S, Collet C, Xaplanteris P, Zimmermann FM, Toth GG, Tonino PAL, Pijls NHJ, Colaiori I, Di Gioia G, Barbato E, Jüni P, Fearon WF, De Bruyne B. Global Fractional Flow Reserve Value Predicts 5-Year Outcomes in Patients With Coronary Atherosclerosis But Without Ischemia. J Am Heart Assoc 2020;9:e017729. [Crossref] [PubMed]
- Yu M, Dai X, Yu L, Lu Z, Shen C, Tao X, Zhang J. Hemodynamic Change of Coronary Atherosclerotic Plaque After Statin Treatment: A Serial Follow-Up Study by Computed Tomography-Derived Fractional Flow Reserve. J Am Heart Assoc 2020;9:e015772. [Crossref] [PubMed]
- Takenaka H, Okamura T, Miyazaki Y, Fujimura T, Ono A, Nakamura T, Tateishi H, Mochizuki M, Akase H, Suetomi T, Uchinoumi H, Oda T, Yano M. Serial changes in the quantitative flow ratio in patients with intermediate residual stenosis after percutaneous coronary intervention. Heart Vessels 2022;37:363-73. [Crossref] [PubMed]
- Lee CH, Hwang J, Kim IC, Cho YK, Park HS, Yoon HJ, Kim H, Han S, Hur SH, Kim KB, Kim JY, Chung JW, Lee JM, Doh JH, Shin ES, Koo BK, Nam CW. Effect of Atorvastatin on Serial Changes in Coronary Physiology and Plaque Parameters. JACC Asia 2022;2:691-703. [Crossref] [PubMed]
- Ambrosy AP, Yang J, Sung SH, Allen AR, Fitzpatrick JK, Rana JS, Wagner J, Philip S, Abrahamson D, Granowitz C, Go AS. Triglyceride Levels and Residual Risk of Atherosclerotic Cardiovascular Disease Events and Death in Adults Receiving Statin Therapy for Primary or Secondary Prevention: Insights From the KP REACH Study. J Am Heart Assoc 2021;10:e020377. [Crossref] [PubMed]
- Nordestgaard BG, Varbo A. Triglycerides and cardiovascular disease. Lancet 2014;384:626-35. [Crossref] [PubMed]
- Rabbat MG, Lakshmanan S, Benjamin MM, Doros G, Kinninger A, Budoff MJ, Bhatt DL. Benefit of icosapent ethyl on coronary physiology assessed by computed tomography angiography fractional flow reserve: EVAPORATE-FFRCT. Eur Heart J Cardiovasc Imaging 2023;24:866-73. [Crossref] [PubMed]
- Nicholls SJ, Tuzcu EM, Kalidindi S, Wolski K, Moon KW, Sipahi I, Schoenhagen P, Nissen SE. Effect of diabetes on progression of coronary atherosclerosis and arterial remodeling: a pooled analysis of 5 intravascular ultrasound trials. J Am Coll Cardiol 2008;52:255-62. [Crossref] [PubMed]
- Nakajima A, Araki M, Kurihara O, Minami Y, Soeda T, Yonetsu T, Higuma T, Kakuta T, McNulty I, Lee H, Malhotra R, Nakamura S, Jang IK. Predictors for Rapid Progression of Coronary Calcification: An Optical Coherence Tomography Study. J Am Heart Assoc 2021;10:e019235. [Crossref] [PubMed]
- Won KB, Han D, Lee JH, Lee SE, Sung JM, Choi SY, Chun EJ, Park SH, Han HW, Sung J, Jung HO, Chang HJ. Evaluation of the impact of glycemic status on the progression of coronary artery calcification in asymptomatic individuals. Cardiovasc Diabetol 2018;17:4. [Crossref] [PubMed]
- Won KB, Lee BK, Lin FY, Hadamitzky M, Kim YJ, Sung JM, et al. Glycemic control is independently associated with rapid progression of coronary atherosclerosis in the absence of a baseline coronary plaque burden: a retrospective case-control study from the PARADIGM registry. Cardiovasc Diabetol 2022;21:239. [Crossref] [PubMed]
- Won KB, Han D, Lee JH, Lee SE, Sung JM, Choi SY, Chun EJ, Park SH, Han HW, Sung J, Jung HO, Chang HJ. Impact of optimal glycemic control on the progression of coronary artery calcification in asymptomatic patients with diabetes. Int J Cardiol 2018;266:250-3. [Crossref] [PubMed]
- Prattichizzo F, de Candia P, De Nigris V, Nicolucci A, Ceriello A. Legacy effect of intensive glucose control on major adverse cardiovascular outcome: Systematic review and meta-analyses of trials according to different scenarios. Metabolism 2020;110:154308. [Crossref] [PubMed]
- Nissen SE, Nicholls SJ, Wolski K, Nesto R, Kupfer S, Perez A, Jure H, De Larochellière R, Staniloae CS, Mavromatis K, Saw J, Hu B, Lincoff AM, Tuzcu EM. PERISCOPE Investigators. Comparison of pioglitazone vs glimepiride on progression of coronary atherosclerosis in patients with type 2 diabetes: the PERISCOPE randomized controlled trial. JAMA 2008;299:1561-73. [Crossref] [PubMed]
- Dykun I, Bayturan O, Carlo J, Nissen SE, Kapadia SR, Tuzcu EM, Nicholls SJ, Puri R. HbA1c, Coronary atheroma progression and cardiovascular outcomes. Am J Prev Cardiol 2022;9:100317. [Crossref] [PubMed]
- Xu B, Tu S, Song L, Jin Z, Yu B, Fu G, et al. Angiographic quantitative flow ratio-guided coronary intervention (FAVOR III China): a multicentre, randomised, sham-controlled trial. Lancet 2021;398:2149-59. [Crossref] [PubMed]
- Dawson LP, Lum M, Nerleker N, Nicholls SJ, Layland J. Coronary Atherosclerotic Plaque Regression: JACC State-of-the-Art Review. J Am Coll Cardiol 2022;79:66-82. [Crossref] [PubMed]