Diffusion tensor imaging of optic neuropathies: a narrative review
Introduction
The term optic neuropathy generally refers to a disease of the optic nerve, which can result from a range of causes, making the correct diagnosis and prognosis of distinct conditions important for proper treatment (1). Optic neuropathies can be either acute or chronic, with acute optic neuropathies such as traumatic optic neuropathy (TON) and optic neuritis (ON) having relatively abrupt onsets, while chronic optic neuropathies like glaucoma have a slow and prolonged development (1). Traditionally, ophthalmologists rely on measurements of the patient’s eye and retina, as well as direct tests of their vision to make a diagnosis. In recent years, magnetic resonance imaging (MRI) has become increasingly valuable in analyzing the impacts of optic neuropathies both on and beyond the optic nerve (2-7). Visualization and comparison of the downstream impact that optic neuropathies have on pre- and post-geniculate visual pathway regions as well as regions beyond the visual system form a typically untapped addition to standard neuro-ophthalmological assessments (6,8). Developing an understanding of how distinct optic neuropathies relate to MRI data metrics can improve the potential for identifying novel biomarkers. This will likely allow for a more integrated and all-encompassing understanding of these conditions with respect to severity, recovery, and treatment (6).
Reviewed optic neuropathies
We aim here to compare a range of conditions that each result in partial visual deafferentation. These conditions differ in etiology and are managed and treated by ophthalmologists in different subspecialties. Nevertheless, we posit that a direct comparison of these groups with diffusion tensor imaging (DTI) of post-retinal structures is appropriate and informative. Whether or not various DTI metrics would distinguish these conditions (based on etiology, time-since-onset, etc.) is an open and important question.
Primary open angle glaucoma (POAG) is the most common form of optic nerve damage with an incidence of 2–3%, and many reported studies of DTI investigation. Similarly, ON, while not as common as POAG, has been studied in a large number of DTI investigations. POAG and ON offer complimentary advantages for study. As a common and slowly progressive disease POAG lends itself to study outside of any emergency context, and relatively large groups of comparable patients. ON provides the opportunity to compare eyes within subjects and track the dynamics of recovery in many cases. In contrast, TON is more diverse, emergent and clinically challenging. However, it is possible that DTI could help to fill this clinical need if methods continue to develop in the future.
POAG
Glaucoma is a chronic and slowly progressing neurodegenerative disease that is the leading cause of irreversible blindness in the world (9) and is most commonly found in patients beyond the age of 55 (10). It has been characterized by loss of retinal ganglion cells (RGC) which then results in distinct changes to the optic nerve head and the retinal nerve fiber layer (11), and generally impacts the visual pathway bilaterally. The pathogenesis of glaucoma, though, is not yet fully understood. The loss of RGCs has been partially linked to an increased intraocular pressure (9,12), which in turn has been referred to as the main risk factor of glaucoma, specifically for POAG (13). POAG comprises more than 80% of glaucoma cases (9) and is the form of glaucoma focused on within this review (14,15). Although the impact of glaucoma can ultimately be severe, it can remain asymptomatic until it reaches an advanced stage, in part because the visual periphery is affected first (9,16,17). Developing any new means of early diagnosis is potentially valuable, and DTI could be considered in the future (6,7,18).
ON
ON is considered an acute inflammatory demyelinating disorder of the optic nerve (19-22), usually affecting generally healthy individuals between the ages of 18 and 50 years (21). It can be an initial sign of multiple sclerosis and is caused by an autoimmune reaction directed against the optic nerve (21). In distinction with typical glaucoma, it usually has a unilateral central field impact on the visual network (23,24). This allows for the affected side to be compared to the unaffected side with a rigorous within-subject design, as well as to controls with a between-subject design as is usually done in the reviewed POAG studies. However, given that ON patients typically recover near-normal vision over time, they are most helpfully analyzed longitudinally, and by comparison between groups based on time of onset. For example, a basic distinction between acute ON representing subjects with recent onset (e.g., no greater than a month) and remote ON representing subjects with at least one episode of ON at least 1 year prior to the current investigation has been reported (25). However, it is likely that a much finer analysis of time-since-onset is required. Unfortunately, most studies do not clearly or consistently classify their subjects into such groups. Our review has classified each reviewed study as either acute (onset within 1 month) or remote (time-since-onset typically greater than 1 year) in order to delineate the findings in a more effective manner based on the significant role that time from the onset has shown to have on the diffusivity measures (25-28).
TON
TON results from blunt or penetrating head trauma that causes either direct or indirect trauma to the optic nerve, which in turn leads to a loss of vision. It is associated with a positive relative afferent pupillary defect (except in cases of bilateral ocular involvement), impaired color vision, and visual field defects (29,30) and the effects can be most severe in cases of bilateral optic nerve involvement (29,31). Importantly, TON has shown both ipsilateral and contralateral impacts on the visual pathway (32). Direct TON involves either a “significant anatomical disruption” to the optic nerve or optic nerve avulsion (29). Indirect TON, on the other hand, is a result of forces from a distal site which extend to the optic nerve. A two-staged model has been proposed to characterize the process that proceeds indirect TON, first starting with the shearing of the RGCs, then increasing in severity by optic nerve swelling (29), which DTI may play a role in deciphering. Of clinical relevance is that in cases of TON, particularly with bilateral damage and lack of responsiveness from the patient, the main clinical tool for diagnosis (the relative afferent pupil defect) can fail, leaving physicians with no reliable tool in the acute setting.
DTI
Traditional anatomical MRI has been extensively used in previous studies to investigate the morphological changes in various brain structures that might accompany disease. The appearance or size of particular grey and white matter regions can be detected and often correlates with important functional and/or clinical variables (33-42). DTI is a specific modality of MRI which measures the flow of water within the brain (43-45). This data allows for advanced visualization of neuronal tracts, primarily composed of white matter tracts (43,46), across the brain by measuring the diffusion of water along axon clusters; this type of diffusion is sensitive to both cellular and microstructural changes (43,47).
Each voxel in a DTI volume can be represented by an ellipsoid with three principal axes and eigenvectors (48) (Figure 1). The measure along the longest axis which usually runs parallel to the measured axon is referred to as axial diffusivity (AD), while the mean magnitude of flow along the two perpendicular axes is referred to as radial diffusivity (RD). AD and RD measures have shown value in diagnosing axonal damage and myelin damage, respectively (48,49). For example, some findings indicate that incomplete myelin formation in the white matter without axonal injury is characterized by an increase in RD with an unaltered measure of AD (50). Additionally, multiple sclerosis patients often show RD increases that are likely associated with myelin loss (51), whilst normal brain development leads to a reduction of RD along white matter pathways, likely representing additional myelination (47,52,53). In contrast, decreased AD has been found to be a good biomarker of axonal damage (47,54,55) and has been associated with axonal swelling, Wallerian degeneration, and diffuse axonal injury (49). This has been shown in animal models where a reduced AD was related to acutely injured axons (56-60) and has additionally been illustrated in humans (61). We note, though, that the correlation of AD with axonal damage is less robust than RD’s correlation with demyelination (49), and is potentially associated with a non-linear interaction between pathological changes over time and severity levels (62). Finally, it is important to note that there are other factors that may lead to alterations of both RD and AD which include, for example, edema and increased extracellular space (51). Further work is essential for developing an increasingly precise mapping between cellular mechanisms and the relationship between RD and AD.
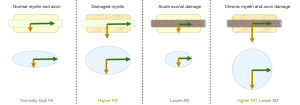
Aside from these two principal eigenvector-based measures, two additional measures can be derived from the relationship between diffusion along the principal axes, specifically fractional anisotropy (FA) and mean diffusivity (MD). FA reflects the relative ratio between the anisotropic to isotropic nature of the measured diffusion, with perfectly isotropic diffusion having a value of zero and perfectly anisotropic diffusion having a value of one (48). FA is argued to be quite sensitive towards detecting alterations of white matter integrity since it decreases when the axonal architecture is disrupted and when there is a loss of myelin (47,63); thus, it represents a loss of organized structure and coherent diffusion (44). This is because many factors including cell death, changes in myelination, and intra/extracellular water may affect FA (43,63). MD, on the other hand, has been proposed to be the simplest DTI-derived scalar(43), representing the average of all principal eigenvalues. An increased MD is commonly associated with damaged tissue because of the resulting increase in free diffusion that this elicits (44,47). A relatively high MD can characterize a high level of disorganization, while a low MD represents a highly organized structure (47,49).
Overall, the four DTI metrics can be separated into two distinct categories according to the way in which they provide information about cellular changes. FA and MD parameters are summative in nature; they describe functional disturbances broadly but may lack the precision necessary to distinguish various structural changes in the visual pathways (49,64-66). In contrast, AD and RD values are tied to directional diffusivity and are increasingly representative of microstructural and anatomical details (49,67). Nevertheless, it is important to note that these four metrics are not entirely independent: a change in one metric would be expected to affect others.
Please see the Appendix 1 for background on some technical parameters that must be selected when obtaining difwfusion MRI (dMRI) data, along with Table S1 for a summary of the parameters used throughout the reviewed studies.
Aims
The aim of this review is to assess the DTI research that has been performed to date on several unique optic neuropathies, a task which has yet to be conducted. This will be done by presenting and discussing the findings obtained by DTI on both chronic and acute forms of optic neuropathies. Specifically, we aim to deduce the general similarities and discrepancies found both within and between the reviewed optic neuropathies and make suggestions for future directions in dMRI research of optic neuropathies. We present this article in accordance with the Narrative Review reporting checklist (available at https://qims.amegroups.com/article/view/10.21037/qims-23-779/rc).
Methods
Table 1 describes the search strategy performed for this review (see also Figure 2).
Table 1
Items | Specification |
---|---|
Date of search | March 1, 2023 |
Databases and other sources searched | PubMed |
Search terms used | POAG search terms: DTI MRI + POAG, diffusion tensor + POAG, DTI + glaucoma, DTI + primary open-angle glaucoma, diffusion MRI + glaucoma, fractional anisotropy + glaucoma, mean diffusivity + glaucoma, axial diffusivity + glaucoma, radial diffusivity + glaucoma |
ON search terms: DTI MRI + optic neuritis, diffusion tensor + optic neuritis, DTI + optic neuritis, fractional anisotropy + optic neuritis, mean diffusivity + optic neuritis, axial diffusivity + optic neuritis, radial diffusivity + optic neuritis | |
TON search terms: DTI MRI + traumatic optic neuropathy, diffusion tensor + traumatic optic neuropathy, DTI + traumatic optic neuropathy, fractional anisotropy + traumatic optic neuropathy, mean diffusivity + traumatic optic neuropathy, axial diffusivity + traumatic optic neuropathy, radial diffusivity + traumatic optic neuro | |
Timeframe | 1994–2023 |
Inclusion criteria | Inclusion criteria for this review required within-group (i.e., affected vs. intact eye) and/or between-group comparisons, correlational based studies that measured at least one of the 4 DTI metrics (FA, MD, RD, and AD) within at least one of the reviewed ROIs (optic nerve, optic tracts, or optic radiations) for POAG, ON, or TON |
Selection process | 2 students (A.C.C. and M.T. conducted the review process. Both reviewed all included and excluded papers to come to agreement |
POAG, primary open-angle glaucoma; DTI, diffusion tensor imaging; MRI, magnetic resonance imaging; FA, fractional anisotropy; MD, mean diffusivity; RD, radial diffusivity; AD, axial diffusivity; ROI, region of interest; ON, optic neuritis; TON, traumatic optic neuropathy.
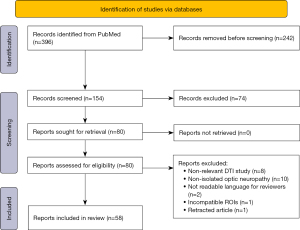
Inclusion criteria for this review required within-group (i.e., affected vs. intact eye) and/or between-group comparisons and correlational-based studies that measured at least one of the 4 DTI metrics (FA, MD, RD, and AD) within one of the selected regions of interest (ROI).
The ROIs included white matter tracts found in both pre-geniculate regions including optic nerves and optic tracts as well as post-geniculate regions, particularly the optic radiations. This allows for an estimation of the prevalence that each DTI metric has along particular segments of the visual system’s primary white matter tracts. These white matter tracts are selected as ROIs, primarily inspired by the network degeneration hypothesis (NDH) (68,69), which posits that any neurodegeneration caused by a particular neuropathy begins and spreads from the brain network that is specific to the neuropathy at hand. Hence white-matter regions specific to the primary visual system were selected and incorporated in this review. We do, though, acknowledge that optic neuropathies most likely lead to replicable neurological changes beyond the primary visual network, which may be specific to the optic neuropathy under investigation. This has been illustrated when patients with monocular blindness displayed notable differences beyond the primary visual tracts (70) and leads us to mention some of the global alterations to white matter that have been associated with the optic neuropathies being reviewed. The formal review, though, is specific to the ROIs mentioned here.
Studies were excluded if the optic neuropathy under investigation was co-occurring with another clinical condition, something which was common with ON since it is commonly associated with multiple sclerosis. This was performed in order to avoid any confounds that other clinical conditions may exert on the diffusion within the white matter. As a result, POAG, ON, and TON had 40, 15, and 3 includable studies, respectively, and their results are summarized below.
Significant results from contrast-based studies were tallied and included in the numerator of the calculation in order to determine the level of consistency of DTI metric-specific findings throughout the selected ROI of the visual system.
Results
All reviewed studies, including their respective DTI parameters and contrasts can be found Tables S2-S4 for POAG, ON, and TON, respectively.
POAG
DTI-based POAG studies included here are comprised of between-group comparisons for POAG patients and controls (66,71-80), within-POAG group comparisons (71,81,82), and correlational studies between DTI measures and ophthalmological measures (73,74,76,78,81,83-85). The contrast- and correlation-based results are distinguished based on the DTI measure and white matter segment of the visual system (see Figure 3A, Table S5).
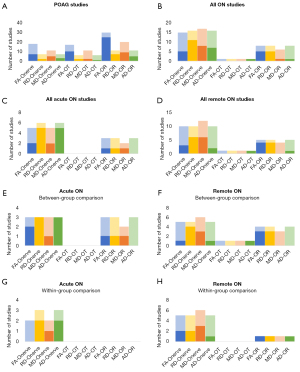
FA
Of all DTI measures, FA appears to be the most reliable biomarker for assessing POAG and has repeatedly been found to be reduced along the visual pathway. It is important to note that FA is not independent of other DTI measures since it is approximately a ratio between AD and RD making it difficult to interpret whether this finding is primarily a result of demyelination or axonal damage. Its combined effect with other DTI metrics may provide the most information considering that the highest sensitivity and specificity for differentiating severe and mild-moderate POAG was obtained from both MD and FA metrics (77).
Within the optic nerve, FA decreases were found throughout POAG progression regardless of the location along the optic nerve (71). This observation has been suggested to be the most reliable trait in diagnosing POAG (73,86). Reduced FA in the optic nerve correlates with ophthalmological measures including POAG severity (73,87,88), cup-disk-ratio (87,89), retinal nerve fiber layer thickness (RNFLT) (86,87,90), and several other measures (see Table S5 for all reviewed significant correlations). FA in the optic tract/optic chiasm correlates with POAG severity (72), cup-disk-ratio (74,78), RNFLT (74) and the mean deviation of the visual field (MDVF) perimetry score (78). In addition, reduced FA predicts a smaller lateral geniculate nucleus (LGN) (77). Some studies also reported reduced FA within the LGN (91) that correlated to the MDVF (78). Nevertheless, assessing DTI changes within the LGN, a small subcortical bundle of grey matter, may be less reliable than measuring diffusion changes within white matter.
Indeed, FA reduction found within the optic radiations is the most prevalent finding, and most reliable, throughout all DTI-related POAG research. Reduced FA in the optic radiations again correlates with POAG severity (77,83,92), cup-disk-ratio (74), RNFLT (74,76,85,93), the frequency doubling test (83) and several other measures including primary visual cortex thickness (67), optic nerve atrophy (94) and has been linked to increased morphological changes in the optic nerve head (83). Additionally, POAG subjects show fewer fiber bundles in either the inferior or superior optic radiations associated with either superior or inferior visual field defects, respectively (82).
Reductions of FA have also been found outside of the regions that were systematically reviewed here, such as in the occipital lobe white matter in general (75,80,93), the white matter (WM) connecting the visual cortex and association cortex (80), the superior parietal lobe (93), corpus callosum (94), inferior fronto-occipital fasciculus (81), anterior thalamic radiation (81), forceps minor (81,93), putamen (81), inferior longitudinal fasciculus (81), and superior longitudinal fasciculus (81). This is an indication that damage to early regions within the visual system can elicit quite distant degeneration through some form of complex reaction which is not yet fully understood (75). FA, however, has been also found to increase in some other regions beyond the visual system ROIs reviewed here which highlights the more global and complex impact that POAG may produce.
RD
RD, a diffusion metric commonly associated with demyelination, has shown replicable increases in POAG (49,54,95). Increased RD within the optic nerve was found twice (88,96), though five other studies did not obtain a significant finding. With respect to correlation studies, it was associated with a measure of optic nerve head damage that is linked to POAG severity (83). Meanwhile, RD in the optic chiasm was found to positively correlate with POAG severity (72), though this was not nearly as replicable as the correlations between increased RD in the optic radiations and ophthalmological measures including severity (76), RNFLT (76), MDVF (76), and frequency doubling test (FDT) (83). Additionally, it was mentioned that the decrease in FA within the optic radiation that is highly associated with POAG is likely driven by the increased RD (76), associated also with increased MD.
MD
Another metric that is impacted by POAG is MD, which in combination with FA has shown the best discriminatory value for POAG specification in some studies (77,97). Often, while FA values decrease for POAG subjects, MD values simultaneously increase (71,73,74,77,82,86). Increased MD showed the highest ratio of replicability out of all diffusion metrics within the optic nerve, with 5 out of 11 contrasts yielding significance. Interestingly in one study, it was shown that abnormally high MD could be a leading indicator, as it appears to be more prevalent in the proximal as opposed to the distal regions, while FA showed no such difference (71). In severe POAG, though, there was no sign of diffusion differences in the proximal and distal areas (71). Another study found that MD in the optic nerve has a higher discriminatory value than FA, particularly showing more significant correlations with cup-disk ratio, RNFTL, and nerve fiber index (89). This finding has not yet been replicated though. Although only two studies found clear evidence of increased MD in the optic tract when compared to controls, several significant correlations between ophthalmologic measures and increased MD were reported (74,78). Out of all three reviewed ROIs, increased MD was most repeatedly found in the optic radiations (with a comparable ratio of significant findings as found in the optic nerves) and was even found to reach further back into the visual cortex (98). This increased MD observed in the radiations was found to correlate with POAG severity (83), MDVF (99), visual field sensitivity (67), and multifocal visually evoked potential (67).
AD
AD yielded the least consistency out of all DTI-based metrics when reviewing POAG-specific contrasts, with some studies finding significant increases in the optic nerve (88,96,100), optic tract (85), optic radiations (67,85,101) and some finding significant decreases, particularly in the optic radiations (79,92). The observed increase in AD could be evidence of a compensatory mechanism to maintain functionality in the presence of white matter damage (51), though there is no clear consensus on the matter to date. Additionally, although the initial cause may be injured axons, there may be subsequent alterations to the cellular landscape such as demyelination, inflammation, and edema, which can lead to a secondary increase in isotropic diffusion and thus an increase in AD (60,102). This, though, is speculative and the main takeaway is that AD is the least consistent DTI metric and that more work is required to deduce if this inconsistency is related to any sort of non-linear phenomenon associated with POAG development.
POAG results—conclusions
DTI shows good potential for differentiating the effects of POAG, showing that DTI can aid in optic neuropathy detection, and severity classification within groups. Importantly, FA, RD, and MD have repeatedly been found to be altered by POAG, i.e., decreased FA, increased RD, and increased MD (Figure 3A, Table S5). In contrast, AD currently appears to show the least discriminatory value for POAG diagnosis (72,76,82). This suggests strongly that POAG is associated with myelin integrity degradation along the visual pathway (further discussed in section Potential cellular mechanisms).
ON
ON studies were composed of both acute and remote forms of ON, both of which had between-group and within-group (affected vs. contralateral side) contrasts, as well as correlational investigations performed (see Figure 3B-3H, and Table S6).
FA
When comparing ON subjects with healthy controls, decreased FA has been found in the optic nerve (103,104), and optic radiation (103,105-107) (including up to the visual cortex (105,108) (see Table S6). This decrease in FA between ON subjects and healthy controls was observable for both acute (103-105) and remote (103,106,107) forms of ON, showing that ON is associated with long term FA alterations along the primary visual system pathway.
In two studies of remote ON, the affected optic nerve displayed a decreased FA compared to the unaffected optic nerve (109,110), while one study found this same phenomenon between remote ON and healthy controls (103) (see Figure 3F,3H, and Table S6), and this correlates with residual contrast sensitivity, visual acuity, optical coherence tomography, and visually evoked potential (25,110). These results suggest that the correlation of FA with ophthalmological measures stabilizes over time and is most robust for remote ON.
Regarding FA in the optic radiations, three out of five findings were reported for remote forms of ON when compared to healthy controls (see Table S6), though it should be emphasized that more of these contrasts were performed in the first place (see Figure 3E-3H). Additionally, for remote ON, Kolbe and colleagues [2012] (102) found that a decrease in FA was linked to decreased AD, and especially increased RD, throughout the bilateral optic radiations. In one study, FA within the optic radiation was the only diffusion metric to show a significant annualized change (of −2.6% per annum); this was present along with an increase in RD and MD, all of which correlate with V1 thinning (105). This suggests that neurodegeneration within the optic radiation is persistently associated with ON, even when determined to be independent of optic radiation lesions (105).
Overall, ON did not yield as many significant results for reduced FA within the primary visual pathway as compared to POAG. The ROI associated with the highest ratio of significance was the optic radiations, where most results were affiliated with remote ON (see Figure 3B). Interestingly, remote ON shows more consistent reductions of FA within the optic radiations compared to the optic nerves (no realistic comparison can be made to the optic tracts since only one study assessed this region at all), whilst acute ON seems to impact FA values more readily within the optic nerves. This may be associated with a directional degeneration, as is discussed further in section Potential cellular mechanisms.
RD
RD seems to have enhanced discriminatory value compared to FA for detecting the effects of ON within the visual system (Figure 3B). Within the optic nerve, a significant increase of RD was found for eleven out of sixteen performed comparisons, with five resulting from acute ON (27,28,103,104) and six from remote ON (25,26,103,109), though the ratio of significance was higher for acute ON. When assessing their ON patient group with a wide range of time from onset (median of 4.0 years since the first instance of ON), Naismith and colleagues [2010] (26) reported that they were able to distinguish the affected optic nerve from the unaffected optic nerve based on RD, while also differentiating between severity of visual outcomes (mild to severe). This is interesting and suggests that DTI could ultimately aid in prognosis.
For remote ON, RD within the optic nerve also correlates with RNFLT, contrast sensitivity, visual acuity, and the visually evoked potential latency and amplitude (25,26,109). Not all results showed a simple increase of RD within the optic nerve, with Goodyear and colleagues [2015] (28) finding that RD within the bilateral optic nerve decreased immediately after ON and increased back to a normal level after 6 months. Interestingly, Nguyen and colleagues [2022] (111) discovered a non-linear trend where RD within the affected optic nerve was less than the unaffected nerve at baseline, whilst it yielded higher RD values 3 months after ON onset, a relationship which still persisted 12 months after onset. Goodyear and colleagues [2015] (28) also showed that the affected optic nerve showed reduced RD (along with reduced AD and MD) when compared to the unaffected nerve at onset, yet did not differ after 6-months had passed (109). These findings suggest a potential nonlinear nature of diffusion with ON progression and emphasize that the time of analysis is increasingly important when assessing ON.
The optic radiations also exhibit increased RD (102,103,105-107), primarily associated with remote ON, with Kolbe and colleagues [2016] (105) finding that RD changes in the optic radiations were observable immediately after ON. Overall, RD seems to be one of the most robust DTI related biomarkers of ON damage, yet the longitudinal nature of RD changes within the visual pathway is uncertain, and more studies will be needed to clarify its progression.
MD
MD increases have been found in the optic nerve (25,28,102-104,108,109) and optic radiations (105), as well as within the occipital lobe (112). These findings, however, are slightly sparser than for FA and RD, especially in the optic radiations (Figure 3B). The seemingly diminished utility of MD in discriminating effects of ON compared to FA and RD could be because of a simultaneous decrease of AD and an increase of RD (102). It is notable that this co-occurrent finding is not common in POAG. This might conceivably indicate differences in the pathogenesis of the two diseases. In sum, MD seems to be an unlikely candidate for diagnosing ON but could prove to be an effective metric in differentiating ON from POAG and from TON.
AD
AD provides the most complex relationship with respect to ON progression, with a non-linear progression of decreased followed by increased AD, specifically in the optic nerve, being shown repeatedly (25,27,28). Because of this non-linear relationship, we have decided to consider both decreased and increased AD as potentially meaningful (see Table S2). Li and colleagues [2011] have shown that acute ON led to a reduced AD, yet subacute and remote stages of ON showed signs of increased AD within the ON. Consistently, two studies found that the initial reduction of AD within the optic nerve was no longer present 6 months after ON onset (28,111), and it was shown that AD further increased to be significantly higher than the unaffected nerve (111). AD within the optic nerve has once been shown to be the first diffusion parameter to show a significant alteration after ON onset (25), and was found to be the DTI metric which, at baseline, best predicted clinical measures at baseline and 6-months after ON onset (113). Additionally, AD is the only metric to yield a significant result in the optic tracts and correlated with RNFLT in the affected eye (107). In this same study, the optic
Overall, although AD seems to be the least consistent diffusion metric in terms of disentangling ON’s effect on the white matter of the primary visual pathway, it is clear that it has a more complex relationship with respect to ON progression over time. This complex relationship is worthy of disentangling as it may be increasingly relevant to clinical measures and ON recovery.
ON results—conclusion
The ON literature shows that DTI is capable of detecting abnormal white matter tracts in the brain, although the results are only modestly replicable thus far. The diffusion metrics that seem to be most robustly impacted are FA and RD, while MD also shows some consistency. Some direct observations of non-linear AD are reported which leads to more questions than answers as to its relationship with ON over time. These data do not support any simple model whereby ON strictly results in axonal damage; it is more likely that there is a combination of axonal damage and myelin degradation. Also, DTI metrics do appear to change over time and reflect at least some aspects of recovered function. In theory, the time in which the DTI parameters return to relatively normal levels could also prove to be functionally meaningful (27,28). In addition, a general trend is suggested in which acute forms of ON yield the most significant results when compared to healthy controls, while remote forms of ON seem to yield the most consistent results when comparing the affected to the contralateral side (Figure 3E-3H).
TON
Very few studies have been conducted in which DTI has been utilized to measure the impact of TON, most likely because of the criticality of timing with respect to trauma and obtaining a subject within a short enough time after injury. The results to date yield little consensus, emphasizing the need for more direct TON research in order to delineate its neural impact on the visual network (see Table S7).
The impact that TON has on the visual pathway is difficult to condense because of the current lack of agreement amongst studies. In the optic nerve, Yang and colleagues [2011] (114) find a lower FA and higher MD in the affected compared to the unaffected optic nerve. Although not in the same section of the optic nerve, Bodanapally and colleagues [2013] (115) found that TON patients have a lower MD in the posterior section of the orbital optic nerve compared to both controls and TBI patients, with no FA difference. Also, RD decreased, and interestingly AD decreased, being the most affected metric (115). Bodanapally et al. [2013] (115) did notice that their results were in disagreement with Yang et al. [2011] (114), leading them to suggest an alternative putative cellular pathology [see also (47,116)]. Li and colleagues [2014] (117) further investigated this issue and looked more closely at the time-since-onset. The pattern of results is complex but provides more support to the findings of Yang et al. [2011] (114). In addition, Li et al. [2014] (117) found that RNFL and optic nerve thickness correlated with each other as well as with the increased RD and MD; decreased FA only with RNFL thickness (117). Ultimately, Li and colleagues [2014] (117) speculate that the sequelae of events may be axonal injury without gliosis or demyelination, followed by demyelination, and then finally axonal loss (after 30 days). In conclusion, the current data is too limited and inconsistent to support any confident pattern of results. However, the findings with the most support, either via replication or via correlation with ophthalmological status, are at least in the expected direction based on our knowledge of POAG and ON: decreased FA and AD and increased MD and RD.
Discussion
By reviewing the DTI literature on POAG, ON, and TON it has become evident that there are some common trends both within and between these distinct neuropathies with respect to their impact on diffusion within the visual system (see Figure 4). By comparing them to one another we can better understand the reliability and consistency within the results to date, develop more robust hypotheses regarding the cellular mechanisms involved with each, and uncover future directions of research.
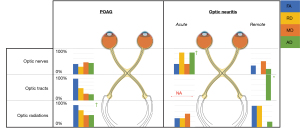
POAG
POAG, a chronic and slowly progressing neurodegenerative disease characterized by loss of RGCs (119-121), consistently shows DTI results akin to structural degradation within the visual system, primarily decreased FA, and increased RD, increased MD; meanwhile, AD appears to be less consistently impacted. It is notable that the majority of findings within the visual system are from the optic radiations, with FA and RD yielding the most consistent findings (Figure 3, Table S5). Surprisingly, a relatively low ratio of studies found significant changes within the optic nerve, although those studies did find correlates of DTI changes within the optic nerve to several ophthalmological measurements, primarily: POAG severity (73,87,88), cup-to-disk ratio (87,89), RNFLT (86,87,90). The most obvious explanation may be methodological: it may be easier to make robust measurements in the large expanse of optic radiations than in the thin optic nerve that is hampered by potential susceptibility effects and motion artifacts (122). This may be why substantially more studies investigated the optic radiations while omitting the optic nerves and is thus a meaningful issue for future studies to consider.
Interestingly, a recent investigation that tested 5 distinct animal models of glaucoma found that decreased FA and increased RD were the most consistent findings, both showing an enhanced utility over AD (62), and another study assessing dogs with glaucoma found a trend for reduced FA throughout all regions of the visual system assessed (123). Meanwhile, a meta-analysis specific to DTI of glaucoma which was performed in 2014 showed that the most consistent and statistically relevant measures were that of decreased FA in the optic nerve and optic radiations, along with increased MD in the optic tracts (7). This same meta-analysis found a strong correlation between decreased FA and glaucoma severity (7). Consistent with our own conclusions, it is FA which is most strongly associated with glaucoma pathology, then followed by RD, MD, and lastly AD. Finally, it should be acknowledged that multiple studies have found global DTI impacts specific to POAG (80,124,125), indicating that POAG may be associated with a whole-brain structural reorganization.
ON
ON, an acute and inflammatory demyelinating disorder of the optic nerve that is associated with a unilateral impact on the visual system (19-22), like POAG, also shows highest ratio of significant findings within the optic radiations. It is FA, RD, and then MD that show the most consistency of the DTI measures, though the inconsistent and seemingly complex nature of ON on AD is more than worthy of future investigation. When assessing acute ON it is evident that the majority of significant findings were obtained when comparing ON patients to healthy subjects. This seems partly due to the existence of more between-group studies than within-subject studies. For patients with remote ON, the majority of significant findings occurred when comparing the affected to the contralateral side, likely showing direct evidence of a long-term impact. Another stimulating result that we suggest is a prime area for further analysis is the non-linear trend that has been observed with diffusion measures when subjects transition from acute to remote ON (25). This has been hypothesized to be a result of either compensatory mechanisms or secondary effects (51). ON prognosis might benefit from direct investigation into this phenomenon through longitudinal study designs.
TON
Lastly, through reviewing the current DTI literature on TON, it became clear that data is very limited, and additionally, the results are inconsistent. However, FA, MD, and RD correlations to ophthalmological measures were observed, including RNFLT (117) and ganglion cell complex thickness (GCCT) (117). The pattern is indeed what might be expected, based upon the patterns seen for POAG and ON, and based on the working model of cellular mechanism presented in the introduction and further discussed below. More research should be conducted on the entire visual system since the current investigations have limited their scope almost entirely to the optic nerves and radiations. Better characterization of TON through DTI analysis, and ideally in conjunction with other MRI modalities, may lead to advances in diagnosis and prognosis, where there is a real clinical need. This is particularly due to the fact that the main tools utilized for TON diagnosis (primarily the relative afferent pupil defect), especially in cases with bilateral damage and lack of responsiveness from the patient, are prone to fail. Ultimately, with the identification of reliable biomarkers, it may be possible to predict which patients are the best candidates for experimental treatments in clinical trials.
Potential cellular mechanisms
Despite the magnitude of observed FA, MD, and RD alterations in both POAG and ON, understanding the underlying cellular causes is still challenging. There are many suggested mechanisms for both POAG and ON (12,126), but anterograde transsynaptic neurodegeneration is the most common (6,67,127,128). Anterograde transsynaptic neurodegeneration can be defined as axonal degeneration which travels away from the cell body towards its own terminal (i.e., from the retina to the visual cortex) and is characterized by neuronal atrophy which eventually leads to apoptosis (105). Retrograde transsynaptic neurodegeneration proceeds towards the cell body (i.e., from the visual cortex to the retina) (129-132) (see Figure 5). Wallerian degeneration, also known as orthograde degeneration, refers to the process in which axonal transection is followed by retrograde axonal degeneration and ultimately neuronal degeneration (105).
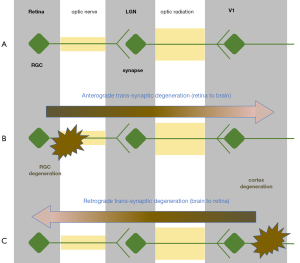
A common suggestion has been that transsynaptic neurodegeneration can be an instigator of POAG (66,67,133) and ON (102,106,134,135). For POAG, authors have particularly emphasized anterograde transsynaptic neurodegeneration (6,67,127,128,133). This distinction can be justified by repeated evidence of increased DTI related damage in pre-geniculate as opposed to post-geniculate regions (128), the link between optic radiation damage and damage to the retinal neurons (66,67,133), and a relationship between cortical thinning and altered RD in the optic radiations (67). In accordance with this, it was found that the extent of optic radiation rarefaction correlates with the extent of optic nerve atrophy and visual field defects (66).
Encouragingly, it has also been suggested that AD as a marker of axonal damage and RD as a marker for myelin damage could be applicable (see Figure 2). Indeed, You and colleagues [2019] (67) have suggested “the fact that radial diffusivity changes extended more posteriorly compared to axial diffusivity suggests myelin pathology might start earlier in the process of anterograde trans-neuronal degeneration”, which agrees with the more prevalent RD findings (primarily in the optic radiations) compared to the scarce AD findings within the POAG studies reviewed. The idea of AD as a potentially transient but leading indicator is worth testing in future studies.
For ON, a similar mechanism of anterograde neurodegeneration may be responsible for the similar trends in DTI results (67,106). Again, several studies show abnormalities within the optic radiations (102,106,134,135), and correlations with retinal thinning, RNFL thickness, and visual field assessments (102,134,135). It may be that FA, RD, and MD changes in the optic radiations—the most consistent findings for acute ON—may represent anterograde transsynaptic degeneration (106). However, because some but not all ON patients exhibit lesions in the optic radiations, this mechanism cannot account for all cases. Kolbe and colleagues [2016] (105) showed that patients with optic radiation lesions have changes of FA, MD, and RD within the radiations that correlate with V1 thinning, while patients without these lesions have reduced AD which correlates with RNFL thinning. They then suggested that reduced AD within the optic radiation is actually a biomarker of anterograde transsynaptic degeneration.
Additionally, AD alterations have repeatedly shown a non-linear relationship with respect to time-from-onset, all of which were in the optic nerve (25,27,28). The mechanisms of this change in AD, going from an initial decrease towards a gradual and sustained increase, are not known, and more research geared towards disentangling these phenomena may lead to insights with high promising clinical utility.
Moreover, the possibility that AD decreases while RD simultaneously increases, should be considered when FA and MD alterations are interpreted. If RD and AD exhibit similar changes of magnitude (i.e., both increase or both decrease) then it is possible that FA remains unchanged whilst MD is impacted, meanwhile if RD and AD have opposite changes in magnitude then it could be that MD remains constant whilst FA shows a significant alteration.
Standardization and quantification
DTI research specific to optic neuropathies could likely benefit from better standardization of methods. Using a shared DTI parameter strategy could aid in elevating the comparability between studies (see Tables S1-S4 for a summary of these parameters). Another notable inconsistency between studies was that of group classification. Particularly, the classification of glaucomatous patients into severity groups was not consistently standardized between studies. The most common method, though, was the Hodapp-Anderson-Parrish (HAP) 6-stage classification scale (136). Also, the criteria used to separate subjects into the acute and remote ON categories were not always reported. There was also varying terminology to describe acute or remote ON, with the most consistent means of acute description being within 30 days of onset (25,103,106,137), while some simply refer to it as a baseline (108) or recent (28) time of ON. Remote forms of ON also illustrated ranges of timing from one to four years, depending on the study. Importantly, multi-group ON analyses have been performed where all groups have distinct lengths of time between the MRI scan and ON onset (103). This allows for an increased understanding of longitudinal ON effects. Several studies also included DTI measurements obtained during a subacute or relapsing-remitting categorization of ON subjects (i.e., 1 month < time-of-onset < 1 year (28,103,106,111,113), which is useful for getting an increased resolution into the temporal trajectory of ON’s impact on diffusion within the visual system. Overall, longitudinal based studies in general are helpful for documenting the longitudinal relationship between DTI and clinical metrics, something which has shown much promise in animal-based studies (138,139) and has been performed in a small cohort of the reviewed studies. More studies of this nature are encouraged.
Finally, it is appropriate to note that while DTI is a relatively novel method of MRI, there are even newer methods for analysis of dMRI which should be considered in the future. Two already implemented methods are diffusion kurtosis imaging (DKI) (140-144) and fixel-based analysis (FBA) (133,145); both of which are geared towards addressing the shortcomings of DTI when it comes to measuring microstructural organization. It is noted that the information gained from these distinct modes of dMRI can be complementary.
Utility of DTI with other imaging modalities
Optical coherence technology (OCT) has been one of the most beneficial advancements in the field of ophthalmology which allows for in-vivo, real time, non-invasive scanning of the macula and optic nerve head (146,147). Capable of assessing the retinal nerve fiber layer and ganglion cell complex (146), OCT is capable of providing objective measures of optic nerve atrophy or swelling and often can predict visual outcome (147) or even detect the presence of current or remote optic neuropathies (148,149). The synergistic relationship between DTI and OCT is evident within the repeatably published correlational results, found for both POAG and ON (see Tables S5,S6). Both modalities have their own strengths and weaknesses, but we suggest that it is likely the combined use of both that will allow for maximal diagnostic potential, particularly because of OCT’s high affinity for measuring highly subtle structural changes to the earliest stages of the visual system, whilst DTI is capable of measuring structural changes found beyond the limits of OCT.
In addition to OCTs utility, other MRI modalities other than DTI have shown utility for detecting distinct optic neuropathies. Both resting state and visually driven functional MRI (fMRI) have shown neuropathy specific alterations, with ON and glaucoma displaying distinguished patterns of activity, both spatially and temporally (150,151). Whilst DTI is particularly geared towards the structural integrity of the white matter, fMRI is able to indirectly capture electrical activity within the grey matter through measuring alterations in blood oxygenation levels.
This is very promising as the methodologies that are currently available for measuring the visual system, whether it be specific to the structures surrounding the retina (i.e., OCT), the white matter (i.e., DTI), or the activity within the grey matter (i.e., fMRI) of the primary visual system, have repeatably provided significant results for deciphering optic neuropathy specific deviations to the visual system.
Conclusions
Through reviewing the DTI-related literature specific to POAG, ON, and TON it is evident that DTI can measure distinct differences both between neuropathic and healthy visual systems as well as between affected and unaffected sides of the visual system within optic neuropathies (primarily ON). We show that for both POAG and ON it is FA, RD, and MD that show the most consistent findings. Past POAG studies have put an emphasis on investigating FA and MD, especially in the optic radiations, though the studies showed a far more even spread of analyses with respect to region and DTI metric compared to ON. Indeed 94 out of 98 performed contrasts within ON studies were specific to the optic nerve and radiations, highlighting a lack of data on intermediary regions, primarily the optic tracts. This hinders our ability to perform a balanced comparison of how diffusion is impacted between regions as well as to decipher any sort of longitudinal trends. TON, however, has received by far the least attention with 3 studies total, likely affiliated with the complexity associated with performing research on this neuropathy compared to the others. Currently all published DTI studies of TON focused only on the optic nerves, preventing any comparison between regions. We suggest that MRI, particularly DTI, could potentially fill a clinical need for better prognosis in the case of ON, and for better diagnosis and prognosis for TON. We aimed here to document the real progress made to date for POAG and ON, and by extension to encourage more such studies of TON.
Acknowledgments
The authors thank Dr. Leonard Levin (McGill Vision Research and Department of Ophthalmology; Department of Neurology and Neurosurgery, McGill University) for his advice and suggested improvements to the manuscript.
Funding: This work was supported by
Footnote
Reporting Checklist: The authors have completed the Narrative Review reporting checklist. Available at https://qims.amegroups.com/article/view/10.21037/qims-23-779/rc
Conflicts of Interest: All authors have completed the ICMJE uniform disclosure form (available at https://qims.amegroups.com/article/view/10.21037/qims-23-779/coif). J.D.M. and A.S. report that this study was supported by a grant from the U.S. Department of Defense CDMRP Vision Research Program (Award No. W81XWH1910853). The other authors have no conflicts of interest to declare.
Ethical Statement: The authors are accountable for all aspects of the work in ensuring that questions related to the accuracy or integrity of any part of the work are appropriately investigated and resolved.
Open Access Statement: This is an Open Access article distributed in accordance with the Creative Commons Attribution-NonCommercial-NoDerivs 4.0 International License (CC BY-NC-ND 4.0), which permits the non-commercial replication and distribution of the article with the strict proviso that no changes or edits are made and the original work is properly cited (including links to both the formal publication through the relevant DOI and the license). See: https://creativecommons.org/licenses/by-nc-nd/4.0/.
References
- Behbehani R. Clinical approach to optic neuropathies. Clin Ophthalmol 2007;1:233-46.
- Kim JD, Hashemi N, Gelman R, Lee AG. Neuroimaging in ophthalmology. Saudi J Ophthalmol 2012;26:401-7. [Crossref] [PubMed]
- Spalton DJ, Tonge KA. The role of MRI scanning in neuro-ophthalmology. Eye (Lond) 1989;3:651-62. [Crossref] [PubMed]
- Simha A, Irodi A, David S. Magnetic resonance imaging for the ophthalmologist: a primer. Indian J Ophthalmol 2012;60:301-10. [Crossref] [PubMed]
- Townsend KA, Wollstein G, Schuman JS. Clinical application of MRI in ophthalmology. NMR Biomed 2008;21:997-1002. [Crossref] [PubMed]
- Mendoza M, Shotbolt M, Faiq MA, Parra C, Chan KC. Advanced Diffusion MRI of the Visual System in Glaucoma: From Experimental Animal Models to Humans. Biology (Basel) 2022;11:454. [Crossref] [PubMed]
- Li K, Lu C, Huang Y, Yuan L, Zeng D, Wu K. Alteration of fractional anisotropy and mean diffusivity in glaucoma: novel results of a meta-analysis of diffusion tensor imaging studies. PLoS One 2014;9:e97445. [Crossref] [PubMed]
- Shi WQ, Zhu PW, Shao Y. The Application of Functional Magnetic Resonance Imaging in Ophthalmology. Am J Transl Med 2020;4:19-33.
- Weinreb RN, Aung T, Medeiros FA. The pathophysiology and treatment of glaucoma: a review. JAMA 2014;311:1901-11. [Crossref] [PubMed]
- Tuck MW, Crick RP. The age distribution of primary open angle glaucoma. Ophthalmic Epidemiol 1998;5:173-83. [Crossref] [PubMed]
- Sharma P, Sample PA, Zangwill LM, Schuman JS. Diagnostic tools for glaucoma detection and management. Surv Ophthalmol 2008;53:S17-32. [Crossref] [PubMed]
- Band LR, Hall CL, Richardson G, Jensen OE, Siggers JH, Foss AJ. Intracellular flow in optic nerve axons: a mechanism for cell death in glaucoma. Invest Ophthalmol Vis Sci 2009;50:3750-8. [Crossref] [PubMed]
- Khaw PT, Elkington AR. ABC of Eyes: Glaucoma—1: Diagnosis. BMJ 2004;328:97-9. [Crossref] [PubMed]
- Duncan RO, Sample PA, Weinreb RN, Bowd C, Zangwill LM. Retinotopic organization of primary visual cortex in glaucoma: Comparing fMRI measurements of cortical function with visual field loss. Prog Retin Eye Res 2007;26:38-56. [Crossref] [PubMed]
- Duncan RO, Sample PA, Weinreb RN, Bowd C, Zangwill LM. Retinotopic organization of primary visual cortex in glaucoma: a method for comparing cortical function with damage to the optic disk. Invest Ophthalmol Vis Sci 2007;48:733-44. [Crossref] [PubMed]
- Hu CX, Zangalli C, Hsieh M, Gupta L, Williams AL, Richman J, Spaeth GL. What do patients with glaucoma see? Visual symptoms reported by patients with glaucoma. Am J Med Sci 2014;348:403-9. [Crossref] [PubMed]
- McKean-Cowdin R, Wang Y, Wu J, Azen SP, Varma R. Impact of visual field loss on health-related quality of life in glaucoma: the Los Angeles Latino Eye Study. Ophthalmology 2008;115:941-948.e1. [Crossref] [PubMed]
- Engelhorn T, A, Schmidt M, Dörfler A, Michelson G. Diffusion tensor imaging of the visual pathway in glaucomatous optic nerve atrophy. Ophthalmologe 2017;114:906-21. [Crossref] [PubMed]
- Kale N. Optic neuritis as an early sign of multiple sclerosis. Eye Brain 2016;8:195-202. [Crossref] [PubMed]
- Fraser C, Plant GT. Optic Neuritis: Pathophysiology, Clinical Features, and Management. Neuroinflammation 2011;253-76.
- Wilhelm H, Schabet M. The Diagnosis and Treatment of Optic Neuritis. Dtsch Arztebl Int 2015;112:616-25; quiz 626. [Crossref] [PubMed]
- Hoorbakht H, Bagherkashi F. Optic neuritis, its differential diagnosis and management. Open Ophthalmol J 2012;6:65-72. [Crossref] [PubMed]
- Abel A, McClelland C, Lee MS. Critical review: Typical and atypical optic neuritis. Surv Ophthalmol 2019;64:770-9. [Crossref] [PubMed]
- Morrow MJ, Wingerchuk D. Neuromyelitis optica. J Neuroophthalmol 2012;32:154-66. [Crossref] [PubMed]
- Naismith RT, Xu J, Tutlam NT, Snyder A, Benzinger T, Shimony J, Shepherd J, Trinkaus K, Cross AH, Song SK. Disability in optic neuritis correlates with diffusion tensor-derived directional diffusivities. Neurology 2009;72:589-94. [Crossref] [PubMed]
- Naismith RT, Xu J, Tutlam NT, Trinkaus K, Cross AH, Song SK. Radial diffusivity in remote optic neuritis discriminates visual outcomes. Neurology 2010;74:1702-10. [Crossref] [PubMed]
- van der Walt A, Kolbe SC, Wang YE, Klistorner A, Shuey N, Ahmadi G, Paine M, Marriott M, Mitchell P, Egan GF, Butzkueven H, Kilpatrick TJ. Optic nerve diffusion tensor imaging after acute optic neuritis predicts axonal and visual outcomes. PLoS One 2013;8:e83825. [Crossref] [PubMed]
- Goodyear BG, Zayed NM, Cortese F, Trufyn J, Costello F. Skewness of Fractional Anisotropy Detects Decreased White Matter Integrity Resulting From Acute Optic Neuritis. Invest Ophthalmol Vis Sci 2015;56:7597-603. [Crossref] [PubMed]
- Yu-Wai-Man P. Traumatic optic neuropathy-Clinical features and management issues. Taiwan J Ophthalmol 2015;5:3-8. [Crossref] [PubMed]
- Jang SY. Traumatic Optic Neuropathy. Korean J Neurotrauma 2018;14:1-5. [Crossref] [PubMed]
- Kumaran AM, Sundar G, Chye LT. Traumatic optic neuropathy: a review. Craniomaxillofac Trauma Reconstr 2015;8:31-41. [Crossref] [PubMed]
- Kyncl M, Lestak J, Tintera J, Haninec P. Traumatic optic neuropathy-a contralateral finding: A case report. Exp Ther Med 2019;17:4244-8. [Crossref] [PubMed]
- Zhao Y, Li Q, Du J, He H, Liang P, Lu J, Li K. Exploring the Relationship between Gray and White Matter in Healthy Adults: A Hybrid Research of Cortical Reconstruction and Tractography. Biomed Res Int 2021;2021:6628506. [Crossref] [PubMed]
- Ulivi L, Kanber B, Prados F, Davagnanam I, Merwick A, Chan E, Williams F, Hughes D, Murphy E, Lachmann RH, Wheeler-Kingshott CAMG, Cipolotti L, Werring DJ. White matter integrity correlates with cognition and disease severity in Fabry disease. Brain 2020;143:3331-42. [Crossref] [PubMed]
- Longstreth WT Jr, Manolio TA, Arnold A, Burke GL, Bryan N, Jungreis CA, Enright PL, O'Leary D, Fried L. Clinical correlates of white matter findings on cranial magnetic resonance imaging of 3301 elderly people. The Cardiovascular Health Study. Stroke 1996;27:1274-82. [Crossref] [PubMed]
- Schmithorst VJ, Wilke M, Dardzinski BJ, Holland SK. Cognitive functions correlate with white matter architecture in a normal pediatric population: a diffusion tensor MRI study. Hum Brain Mapp 2005;26:139-47. [Crossref] [PubMed]
- Sasson E, Doniger GM, Pasternak O, Tarrasch R, Assaf Y. White matter correlates of cognitive domains in normal aging with diffusion tensor imaging. Front Neurosci 2013;7:32. [Crossref] [PubMed]
- Chabran E, Noblet V, Loureiro de Sousa P, Demuynck C, Philippi N, Mutter C, Anthony P, Martin-Hunyadi C, Cretin B, Blanc F. Changes in gray matter volume and functional connectivity in dementia with Lewy bodies compared to Alzheimer's disease and normal aging: implications for fluctuations. Alzheimers Res Ther 2020;12:9. [Crossref] [PubMed]
- Takeuchi H, Taki Y, Nouchi R, Yokoyama R, Kotozaki Y, Nakagawa S, Sekiguchi A, Iizuka K, Yamamoto Y, Hanawa S, Araki T, Miyauchi CM, Shinada T, Sakaki K, Sassa Y, Nozawa T, Ikeda S, Yokota S, Daniele M, Kawashima R. Global associations between regional gray matter volume and diverse complex cognitive functions: evidence from a large sample study. Sci Rep 2017;7:10014. [Crossref] [PubMed]
- Ramanoël S, Hoyau E, Kauffmann L, Renard F, Pichat C, Boudiaf N, Krainik A, Jaillard A, Baciu M. Gray Matter Volume and Cognitive Performance During Normal Aging. A Voxel-Based Morphometry Study. Front Aging Neurosci 2018;10:235. [Crossref] [PubMed]
- Taki Y, Thyreau B, Kinomura S, Sato K, Goto R, Kawashima R, Fukuda H. Correlations among brain gray matter volumes, age, gender, and hemisphere in healthy individuals. PLoS One 2011;6:e22734. [Crossref] [PubMed]
- Jiang L, Cao X, Li T, Tang Y, Li W, Wang J, Chan RC, Li C. Cortical Thickness Changes Correlate with Cognition Changes after Cognitive Training: Evidence from a Chinese Community Study. Front Aging Neurosci 2016;8:118. [Crossref] [PubMed]
- O'Donnell LJ, Westin CF. An introduction to diffusion tensor image analysis. Neurosurg Clin N Am 2011;22:185-96. viii. [Crossref] [PubMed]
- Soares JM, Marques P, Alves V, Sousa N. A hitchhiker's guide to diffusion tensor imaging. Front Neurosci 2013;7:31. [Crossref] [PubMed]
- Mukherjee P, Chung SW, Berman JI, Hess CP, Henry RG. Diffusion tensor MR imaging and fiber tractography: technical considerations. AJNR Am J Neuroradiol 2008;29:843-52. [Crossref] [PubMed]
- Assaf Y, Pasternak O. Diffusion tensor imaging (DTI)-based white matter mapping in brain research: a review. J Mol Neurosci 2008;34:51-61. [Crossref] [PubMed]
- Alexander AL, Lee JE, Lazar M, Field AS. Diffusion tensor imaging of the brain. Neurotherapeutics 2007;4:316-29. [Crossref] [PubMed]
- Sbardella E, Tona F, Petsas N, Pantano P. DTI Measurements in Multiple Sclerosis: Evaluation of Brain Damage and Clinical Implications. Mult Scler Int 2013;2013:671730. [Crossref] [PubMed]
- Aung WY, Mar S, Benzinger TL. Diffusion tensor MRI as a biomarker in axonal and myelin damage. Imaging Med 2013;5:427-40. [Crossref] [PubMed]
- Song SK, Sun SW, Ramsbottom MJ, Chang C, Russell J, Cross AH. Dysmyelination revealed through MRI as increased radial (but unchanged axial) diffusion of water. Neuroimage 2002;17:1429-36. [Crossref] [PubMed]
- Fink F, Klein J, Lanz M, Mitrovics T, Lentschig M, Hahn HK, Hildebrandt H. Comparison of diffusion tensor-based tractography and quantified brain atrophy for analyzing demyelination and axonal loss in MS. J Neuroimaging 2010;20:334-44. [Crossref] [PubMed]
- Colby JB, O’Hare ED, Bramen JE, Sowell ER. Structural Brain Development: Birth Through Adolescence. In: Neural Circuit Development and Function in the Heathy and Diseased Brain. Elsevier; 2013;207-30.
- Suzuki Y, Matsuzawa H, Kwee IL, Nakada T. Absolute eigenvalue diffusion tensor analysis for human brain maturation. NMR Biomed 2003;16:257-60. [Crossref] [PubMed]
- Winklewski PJ, Sabisz A, Naumczyk P, Jodzio K, Szurowska E, Szarmach A. Understanding the Physiopathology Behind Axial and Radial Diffusivity Changes-What Do We Know? Front Neurol 2018;9:92. [Crossref] [PubMed]
- Budde MD, Xie M, Cross AH, Song SK. Axial diffusivity is the primary correlate of axonal injury in the experimental autoimmune encephalomyelitis spinal cord: a quantitative pixelwise analysis. J Neurosci 2009;29:2805-13. [Crossref] [PubMed]
- DeBoy CA, Zhang J, Dike S, Shats I, Jones M, Reich DS, Mori S, Nguyen T, Rothstein B, Miller RH, Griffin JT, Kerr DA, Calabresi PA. High resolution diffusion tensor imaging of axonal damage in focal inflammatory and demyelinating lesions in rat spinal cord. Brain 2007;130:2199-210. [Crossref] [PubMed]
- Song SK, Sun SW, Ju WK, Lin SJ, Cross AH, Neufeld AH. Diffusion tensor imaging detects and differentiates axon and myelin degeneration in mouse optic nerve after retinal ischemia. Neuroimage 2003;20:1714-22. [Crossref] [PubMed]
- Wu Q, Butzkueven H, Gresle M, Kirchhoff F, Friedhuber A, Yang Q, Wang H, Fang K, Lei H, Egan GF, Kilpatrick TJ. MR diffusion changes correlate with ultra-structurally defined axonal degeneration in murine optic nerve. Neuroimage 2007;37:1138-47. [Crossref] [PubMed]
- Mac Donald CL, Dikranian K, Song SK, Bayly PV, Holtzman DM, Brody DL. Detection of traumatic axonal injury with diffusion tensor imaging in a mouse model of traumatic brain injury. Exp Neurol 2007;205:116-31. [Crossref] [PubMed]
- Mac Donald CL, Dikranian K, Bayly P, Holtzman D, Brody D. Diffusion tensor imaging reliably detects experimental traumatic axonal injury and indicates approximate time of injury. J Neurosci 2007;27:11869-76. [Crossref] [PubMed]
- Concha L, Gross DW, Wheatley BM, Beaulieu C. Diffusion tensor imaging of time-dependent axonal and myelin degradation after corpus callosotomy in epilepsy patients. Neuroimage 2006;32:1090-9. [Crossref] [PubMed]
- Colbert MK, Ho LC, van der Merwe Y, Yang X, McLellan GJ, Hurley SA, Field AS, Yun H, Du Y, Conner IP, Parra C, Faiq MA, Fingert JH, Wollstein G, Schuman JS, Chan KC. Diffusion Tensor Imaging of Visual Pathway Abnormalities in Five Glaucoma Animal Models. Invest Ophthalmol Vis Sci 2021;62:21. [Crossref] [PubMed]
- Alba-Ferrara LM, de Erausquin GA. What does anisotropy measure? Insights from increased and decreased anisotropy in selective fiber tracts in schizophrenia. Front Integr Neurosci 2013;7:9. [Crossref] [PubMed]
- Pierpaoli C, Basser PJ. Toward a quantitative assessment of diffusion anisotropy. Magn Reson Med 1996;36:893-906. [Crossref] [PubMed]
- Basser PJ, Pierpaoli C. Microstructural and physiological features of tissues elucidated by quantitative-diffusion-tensor MRI. J Magn Reson B 1996;111:209-19. [Crossref] [PubMed]
- Engelhorn T, Michelson G, Waerntges S, Struffert T, Haider S, Doerfler A. Diffusion tensor imaging detects rarefaction of optic radiation in glaucoma patients. Acad Radiol 2011;18:764-9. [Crossref] [PubMed]
- You Y, Joseph C, Wang C, Gupta V, Liu S, Yiannikas C, Chua BE, Chitranshi N, Shen T, Dheer Y, Invernizzi A, Borotkanics R, Barnett M, Graham SL, Klistorner A. Demyelination precedes axonal loss in the transneuronal spread of human neurodegenerative disease. Brain 2019;142:426-42. [Crossref] [PubMed]
- Tahmasian M, Shao J, Meng C, Grimmer T, Diehl-Schmid J, Yousefi BH, Förster S, Riedl V, Drzezga A, Sorg C. Based on the Network Degeneration Hypothesis: Separating Individual Patients with Different Neurodegenerative Syndromes in a Preliminary Hybrid PET/MR Study. J Nucl Med 2016;57:410-5. [Crossref] [PubMed]
- Greicius MD, Kimmel DL. Neuroimaging insights into network-based neurodegeneration. Curr Opin Neurol 2012;25:727-34. [Crossref] [PubMed]
- Hanekamp S, Ćurčić-Blake B, Caron B, McPherson B, Timmer A, Prins D, Boucard CC, Yoshida M, Ida M, Hunt D, Jansonius NM, Pestilli F, Cornelissen FW. White matter alterations in glaucoma and monocular blindness differ outside the visual system. Sci Rep 2021;11:6866. [Crossref] [PubMed]
- Bolacchi F, Garaci FG, Martucci A, Meschini A, Fornari M, Marziali S, Mancino R, Squillaci E, Floris R, Cerulli L, Simonetti G, Nucci C. Differences between proximal versus distal intraorbital optic nerve diffusion tensor magnetic resonance imaging properties in glaucoma patients. Invest Ophthalmol Vis Sci 2012;53:4191-6. [Crossref] [PubMed]
- Dai H, Yin D, Hu C, Morelli JN, Hu S, Yan X, Xu D. Whole-brain voxel-based analysis of diffusion tensor MRI parameters in patients with primary open angle glaucoma and correlation with clinical glaucoma stage. Neuroradiology 2013;55:233-43. [Crossref] [PubMed]
- Garaci FG, Bolacchi F, Cerulli A, Melis M, Spanò A, Cedrone C, Floris R, Simonetti G, Nucci C. Optic nerve and optic radiation neurodegeneration in patients with glaucoma: in vivo analysis with 3-T diffusion-tensor MR imaging. Radiology 2009;252:496-501. [Crossref] [PubMed]
- Chen Z, Lin F, Wang J, Li Z, Dai H, Mu K, Ge J, Zhang H. Diffusion tensor magnetic resonance imaging reveals visual pathway damage that correlates with clinical severity in glaucoma. Clin Exp Ophthalmol 2013;41:43-9. [Crossref] [PubMed]
- Lu P, Shi L, Du H, Xie B, Li C, Li S, Liu T, Feng H, Wang J. Reduced white matter integrity in primary open-angle glaucoma: a DTI study using tract-based spatial statistics. J Neuroradiol 2013;40:89-93. [Crossref] [PubMed]
- Tellouck L, Durieux M, Coupé P, Cougnard-Grégoire A, Tellouck J, Tourdias T, Munsch F, Garrigues A, Helmer C, Malet F, Dartigues JF, Dousset V, Delcourt C, Schweitzer C. Optic Radiations Microstructural Changes in Glaucoma and Association With Severity: A Study Using 3Tesla-Magnetic Resonance Diffusion Tensor Imaging. Invest Ophthalmol Vis Sci 2016;57:6539-47. [Crossref] [PubMed]
- Li M, Ke M, Song Y, Mu K, Zhang H, Chen Z. Diagnostic utility of central damage determination in glaucoma by magnetic resonance imaging: An observational study. Exp Ther Med 2019;17:1891-5. [Crossref] [PubMed]
- Song XY, Puyang Z, Chen AH, Zhao J, Li XJ, Chen YY, Tang WJ, Zhang YY. Diffusion Tensor Imaging Detects Microstructural Differences of Visual Pathway in Patients With Primary Open-Angle Glaucoma and Ocular Hypertension. Front Hum Neurosci 2018;12:426. [Crossref] [PubMed]
- Wang R, Tang Z, Sun X, Wu L, Wang J, Zhong Y, Xiao Z. White Matter Abnormalities and Correlation With Severity in Normal Tension Glaucoma: A Whole Brain Atlas-Based Diffusion Tensor Study. Invest Ophthalmol Vis Sci 2018;59:1313-22. [Crossref] [PubMed]
- Qu X, Wang Q, Chen W, Li T, Guo J, Wang H, Zhang X, Wang Y, Wang N, Xian J. Combined machine learning and diffusion tensor imaging reveals altered anatomic fiber connectivity of the brain in primary open-angle glaucoma. Brain Res 2019;1718:83-90. [Crossref] [PubMed]
- Zikou AK, Kitsos G, Tzarouchi LC, Astrakas L, Alexiou GA, Argyropoulou MI. Voxel-based morphometry and diffusion tensor imaging of the optic pathway in primary open-angle glaucoma: a preliminary study. AJNR Am J Neuroradiol 2012;33:128-34. [Crossref] [PubMed]
- Kaushik M, Graham SL, Wang C, Klistorner A. A topographical relationship between visual field defects and optic radiation changes in glaucoma. Invest Ophthalmol Vis Sci 2014;55:5770-5. [Crossref] [PubMed]
- Michelson G, Engelhorn T, Wärntges S, El Rafei A, Hornegger J, Doerfler A. DTI parameters of axonal integrity and demyelination of the optic radiation correlate with glaucoma indices. Graefes Arch Clin Exp Ophthalmol 2013;251:243-53. [Crossref] [PubMed]
- Schoemann J, Engelhorn T, Waerntges S, Doerfler A, El-Rafei A, Michelson G. Cerebral microinfarcts in primary open-angle glaucoma correlated with DTI-derived integrity of optic radiation. Invest Ophthalmol Vis Sci 2014;55:7241-7. [Crossref] [PubMed]
- Frezzotti P, Giorgio A, Toto F, De Leucio A, De Stefano N. Early changes of brain connectivity in primary open angle glaucoma. Hum Brain Mapp 2016;37:4581-96. [Crossref] [PubMed]
- Sidek S, Ramli N, Rahmat K, Ramli NM, Abdulrahman F, Tan LK. Glaucoma severity affects diffusion tensor imaging (DTI) parameters of the optic nerve and optic radiation. Eur J Radiol 2014;83:1437-41. [Crossref] [PubMed]
- Miller N, Liu Y, Krivochenitser R, Rokers B. Linking neural and clinical measures of glaucoma with diffusion magnetic resonance imaging (dMRI). PLoS One 2019;14:e0217011. [Crossref] [PubMed]
- Chang ST, Xu J, Trinkaus K, Pekmezci M, Arthur SN, Song SK, Barnett EM. Optic nerve diffusion tensor imaging parameters and their correlation with optic disc topography and disease severity in adult glaucoma patients and controls. J Glaucoma 2014;23:513-20. [Crossref] [PubMed]
- Nucci C, Mancino R, Martucci A, Bolacchi F, Manenti G, Cedrone C, Culasso F, Floris R, Cerulli L, Garaci FG. 3-T Diffusion tensor imaging of the optic nerve in subjects with glaucoma: correlation with GDx-VCC, HRT-III and Stratus optical coherence tomography findings. Br J Ophthalmol 2012;96:976-80. [Crossref] [PubMed]
- Omodaka K, Murata T, Sato S, Takahashi M, Tatewaki Y, Nagasaka T, Doi H, Araie M, Takahashi S, Nakazawa T. Correlation of magnetic resonance imaging optic nerve parameters to optical coherence tomography and the visual field in glaucoma. Clin Exp Ophthalmol 2014;42:360-8. [Crossref] [PubMed]
- Erichev VP, Panyushkina LA, Novikov IA, Bryukhov VV. Diffusion tensor magnetic resonance imaging in the diagnosis of visual pathway neurodegeneration in glaucoma. Vestn Oftalmol 2015;131:59-63. [Crossref] [PubMed]
- Li T, Miao W, He HG, Xian JF. Study of optic radiations in patients with primary open-angle glaucoma with diffusion tensor imaging. Zhonghua Yi Xue Za Zhi 2017;97:347-52. [Crossref] [PubMed]
- Boucard CC, Hanekamp S, Ćurčić-Blake B, Ida M, Yoshida M, Cornelissen FW. Neurodegeneration beyond the primary visual pathways in a population with a high incidence of normal-pressure glaucoma. Ophthalmic Physiol Opt 2016;36:344-53. [Crossref] [PubMed]
- Engelhorn T, Michelson G, Waerntges S, Hempel S, El-Rafei A, Struffert T, Doerfler A. A new approach to assess intracranial white matter abnormalities in glaucoma patients: changes of fractional anisotropy detected by 3T diffusion tensor imaging. Acad Radiol 2012;19:485-8. [Crossref] [PubMed]
- Song SK, Yoshino J, Le TQ, Lin SJ, Sun SW, Cross AH, Armstrong RC. Demyelination increases radial diffusivity in corpus callosum of mouse brain. Neuroimage 2005;26:132-40. [Crossref] [PubMed]
- Zhang YQ, Li J, Xu L, Zhang L, Wang ZC, Yang H, Chen CX, Wu XS, Jonas JB. Anterior visual pathway assessment by magnetic resonance imaging in normal-pressure glaucoma. Acta Ophthalmol 2012;90:e295-302. [Crossref] [PubMed]
- El-Rafei A, Engelhorn T, Wärntges S, Dörfler A, Hornegger J, Michelson G. Glaucoma classification based on visual pathway analysis using diffusion tensor imaging. Magn Reson Imaging 2013;31:1081-91. [Crossref] [PubMed]
- Xu ZF, Sun JS, Zhang XH, Feng YY, Pan AZ, Gao MY, Zhao H. Microstructural visual pathway abnormalities in patients with primary glaucoma: 3 T diffusion kurtosis imaging study. Clin Radiol 2018;73:591.e9-591.e15. [Crossref] [PubMed]
- Zhou W, Muir ER, Chalfin S, Nagi KS, Duong TQ. MRI Study of the Posterior Visual Pathways in Primary Open Angle Glaucoma. J Glaucoma 2017;26:173-81. [Crossref] [PubMed]
- Frezzotti P, Giorgio A, Motolese I, De Leucio A, Iester M, Motolese E, Federico A, De Stefano N. Structural and functional brain changes beyond visual system in patients with advanced glaucoma. PLoS One 2014;9:e105931. [Crossref] [PubMed]
- Giorgio A, Zhang J, Costantino F, De Stefano N, Frezzotti P. Diffuse brain damage in normal tension glaucoma. Hum Brain Mapp 2018;39:532-41. [Crossref] [PubMed]
- Kolbe S, Bajraszewski C, Chapman C, Nguyen T, Mitchell P, Paine M, Butzkueven H, Johnston L, Kilpatrick T, Egan G. Diffusion tensor imaging of the optic radiations after optic neuritis. Hum Brain Mapp 2012;33:2047-61. [Crossref] [PubMed]
- Li M, Li J, He H, Wang Z, Lv B, Li W, Hailla N, Yan F, Xian J, Ai L. Directional diffusivity changes in the optic nerve and optic radiation in optic neuritis. Br J Radiol 2011;84:304-14. [Crossref] [PubMed]
- Zhang Y, Wan SH, Wu GJ, Zhang XL. High sensitivity of diffusion tensor imaging in discriminating idiopathic demyelinating optic neuritis. Int J Ophthalmol 2016;9:1082-5. [Crossref] [PubMed]
- Kolbe SC, van der Walt A, Butzkueven H, Klistorner A, Egan GF, Kilpatrick TJ. Serial Diffusion Tensor Imaging of the Optic Radiations after Acute Optic Neuritis. J Ophthalmol 2016;2016:2764538. [Crossref] [PubMed]
- Tur C, Goodkin O, Altmann DR, Jenkins TM, Miszkiel K, Mirigliani A, Fini C, Gandini Wheeler-Kingshott CA, Thompson AJ, Ciccarelli O, Toosy AT. Longitudinal evidence for anterograde trans-synaptic degeneration after optic neuritis. Brain 2016;139:816-28. [Crossref] [PubMed]
- Raz N, Bick AS, Ben-Hur T, Levin N. Focal demyelinative damage and neighboring white matter integrity: an optic neuritis study. Mult Scler 2015;21:562-71. [Crossref] [PubMed]
- Hickman SJ, Wheeler-Kingshott CA, Jones SJ, Miszkiel KA, Barker GJ, Plant GT, Miller DH. Optic nerve diffusion measurement from diffusion-weighted imaging in optic neuritis. AJNR Am J Neuroradiol 2005;26:951-6.
- Trip SA, Wheeler-Kingshott C, Jones SJ, Li WY, Barker GJ, Thompson AJ, Plant GT, Miller DH. Optic nerve diffusion tensor imaging in optic neuritis. Neuroimage 2006;30:498-505. [Crossref] [PubMed]
- Kolbe S, Chapman C, Nguyen T, Bajraszewski C, Johnston L, Kean M, Mitchell P, Paine M, Butzkueven H, Kilpatrick T, Egan G. Optic nerve diffusion changes and atrophy jointly predict visual dysfunction after optic neuritis. Neuroimage 2009;45:679-86. [Crossref] [PubMed]
- Nguyen MNL, Zhu C, Kolbe SC, Butzkueven H, White OB, Fielding J, Kilpatrick TJ, Egan GF, Klistorner A, van der Walt A. Early predictors of visual and axonal outcomes after acute optic neuritis. Front Neurol 2022;13:945034. [Crossref] [PubMed]
- Bester M, Heesen C, Schippling S, Martin R, Ding XQ, Holst B, Fiehler J. Early anisotropy changes in the corpus callosum of patients with optic neuritis. Neuroradiology 2008;50:549-57. [Crossref] [PubMed]
- Naismith RT, Xu J, Tutlam NT, Lancia S, Trinkaus K, Song SK, Cross AH. Diffusion tensor imaging in acute optic neuropathies: predictor of clinical outcomes. Arch Neurol 2012;69:65-71. [Crossref] [PubMed]
- Yang QT, Fan YP, Zou Y, Kang Z, Hu B, Liu X, Zhang GH, Li Y. Evaluation of traumatic optic neuropathy in patients with optic canal fracture using diffusion tensor magnetic resonance imaging: a preliminary report. ORL J Otorhinolaryngol Relat Spec 2011;73:301-7.
- Bodanapally UK, Kathirkamanathan S, Geraymovych E, Mirvis SE, Choi AY, McMillan AB, Zhuo J, Shin RK. Diagnosis of traumatic optic neuropathy: application of diffusion tensor magnetic resonance imaging. J Neuroophthalmol 2013;33:128-33.
- Sorensen AG, Wu O, Copen WA, Davis TL, Gonzalez RG, Koroshetz WJ, Reese TG, Rosen BR, Wedeen VJ, Weisskoff RM. Human acute cerebral ischemia: detection of changes in water diffusion anisotropy by using MR imaging. Radiology 1999;212:785-92.
- Li J, Shi W, Li M, Wang Z, He H, Xian J, Lv B, Yan F. Time-dependent diffusion tensor changes of optic nerve in patients with indirect traumatic optic neuropathy. Acta Radiol 2014;55:855-63. [Crossref] [PubMed]
- Perkel JM. The software that powers scientific illustration. Nature 2020;582:137-8.
- Kuehn MH, Fingert JH, Kwon YH. Retinal ganglion cell death in glaucoma: mechanisms and neuroprotective strategies. Ophthalmol Clin North Am 2005;18:383-95. vi. [Crossref] [PubMed]
- Harwerth RS, Quigley HA. Visual field defects and retinal ganglion cell losses in patients with glaucoma. Arch Ophthalmol 2006;124:853-9. [Crossref] [PubMed]
- Munemasa Y, Kitaoka Y. Molecular mechanisms of retinal ganglion cell degeneration in glaucoma and future prospects for cell body and axonal protection. Front Cell Neurosci 2012;6:60. [Crossref] [PubMed]
- Deng F, Reinshagen KL, Li MD, Juliano AF. Motion degradation in optic nerve MRI: A randomized intraindividual comparison study of eye states. Eur J Radiol 2021;142:109865.
- Graham KL, Johnson PJ, Barry EF, Pérez Orrico M, Soligo DJ, Lawlor M, White A. Diffusion tensor imaging of the visual pathway in dogs with primary angle-closure glaucoma. Vet Ophthalmol 2021;24:63-74. [Crossref] [PubMed]
- Cio FD, Garaci F, Minosse S, Passamonti L, Martucci A, Lanzafame S, Giuliano FD, Picchi E, Mancino R, Guerrisi M, Nucci C, Floris R, Toschi N. Disruption of structural brain networks in Primary Open Angle Glaucoma. Annu Int Conf IEEE Eng Med Biol Soc 2020;2020:1705-8. [Crossref] [PubMed]
- Di Ciò F, Garaci F, Minosse S, Passamonti L, Martucci A, Lanzafame S, Di Giuliano F, Picchi E, Cesareo M, Guerrisi MG, Floris R, Nucci C, Toschi N. Reorganization of the structural connectome in primary open angle Glaucoma. Neuroimage Clin 2020;28:102419.
- Zhang HJ, Mi XS, So KF. Normal tension glaucoma: from the brain to the eye or the inverse? Neural Regen Res 2019;14:1845-50. [Crossref] [PubMed]
- Nucci C, Martucci A, Cesareo M, Mancino R, Russo R, Bagetta G, Cerulli L, Garaci FG. Brain involvement in glaucoma: advanced neuroimaging for understanding and monitoring a new target for therapy. Curr Opin Pharmacol 2013;13:128-33. [Crossref] [PubMed]
- Haykal S, Jansonius NM, Cornelissen FW. Progression of Visual Pathway Degeneration in Primary Open-Angle Glaucoma: A Longitudinal Study. Front Hum Neurosci 2021;15:630898. [Crossref] [PubMed]
- Coleman M. Axon degeneration mechanisms: commonality amid diversity. Nat Rev Neurosci 2005;6:889-98. [Crossref] [PubMed]
- Saxena S, Caroni P. Mechanisms of axon degeneration: from development to disease. Prog Neurobiol 2007;83:174-91. [Crossref] [PubMed]
- Davis BM, Crawley L, Pahlitzsch M, Javaid F, Cordeiro MF. Glaucoma: the retina and beyond. Acta Neuropathol 2016;132:807-26. [Crossref] [PubMed]
- Al-Louzi O, Button J, Newsome SD, Calabresi PA, Saidha S. Retrograde trans-synaptic visual pathway degeneration in multiple sclerosis: A case series. Mult Scler 2017;23:1035-9. [Crossref] [PubMed]
- Haykal S, Curcic-Blake B, Jansonius NM, Cornelissen FW. Fixel-Based Analysis of Visual Pathway White Matter in Primary Open-Angle Glaucoma. Invest Ophthalmol Vis Sci 2019;60:3803-12. [Crossref] [PubMed]
- Ngamsombat C, Tian Q, Fan Q, Russo A, Machado N, Polackal M, George IC, Witzel T, Klawiter EC, Huang SY. Axonal damage in the optic radiation assessed by white matter tract integrity metrics is associated with retinal thinning in multiple sclerosis. Neuroimage Clin 2020;27:102293. [Crossref] [PubMed]
- Rocca MA, Mesaros S, Preziosa P, Pagani E, Stosic-Opincal T, Dujmovic-Basuroski I, Drulovic J, Filippi M. Wallerian and trans-synaptic degeneration contribute to optic radiation damage in multiple sclerosis: a diffusion tensor MRI study. Mult Scler 2013;19:1610-7. [Crossref] [PubMed]
- Hodapp EA, Parrish RK, Anderson DR. Clinical decisions in glaucoma. Mosby-Year Book; 1993.
- Chen J, Zhu L, Li H, Lu Z, Chen X, Fang S. Diffusion tensor imaging of occult injury of optic radiation following optic neuritis in multiple sclerosis. Exp Ther Med 2016;12:2505-10. [Crossref] [PubMed]
- van der Merwe Y, Murphy MC, Sims JR, Faiq MA, Yang XL, Ho LC, Conner IP, Yu Y, Leung CK, Wollstein G, Schuman JS, Chan KC. Citicoline Modulates Glaucomatous Neurodegeneration Through Intraocular Pressure-Independent Control. Neurotherapeutics 2021;18:1339-59. [Crossref] [PubMed]
- Yang XL, van der Merwe Y, Sims J, Parra C, Ho LC, Schuman JS, Wollstein G, Lathrop KL, Chan KC. Age-related Changes in Eye, Brain and Visuomotor Behavior in the DBA/2J Mouse Model of Chronic Glaucoma. Sci Rep 2018;8:4643. [Crossref] [PubMed]
- Nucci C, Garaci F, Altobelli S, Di Ciò F, Martucci A, Aiello F, Lanzafame S, Di Giuliano F, Picchi E, Minosse S, Cesareo M, Guerrisi MG, Floris R, Passamonti L, Toschi N. Diffusional Kurtosis Imaging of White Matter Degeneration in Glaucoma. J Clin Med 2020;9:3122. [Crossref] [PubMed]
- Henriques RN, Correia MM, Marrale M, Huber E, Kruper J, Koudoro S, Yeatman JD, Garyfallidis E, Rokem A. Diffusional Kurtosis Imaging in the Diffusion Imaging in Python Project. Front Hum Neurosci 2021;15:675433. [Crossref] [PubMed]
- Arab A, Wojna-Pelczar A, Khairnar A, Szabó N, Ruda-Kucerova J. Principles of diffusion kurtosis imaging and its role in early diagnosis of neurodegenerative disorders. Brain Res Bull 2018;139:91-8. [Crossref] [PubMed]
- Sun Z, Parra C, Bang JW, Fieremans E, Wollstein G, Schuman JS, Chan KC. Diffusion Kurtosis Imaging Reveals Optic Tract Damage That Correlates with Clinical Severity in Glaucoma. Annu Int Conf IEEE Eng Med Biol Soc 2020;2020:1746-9. [Crossref] [PubMed]
- Lu P, Yuan T, Liu X, Tian G, Zhang J, Sha Y. Role of Diffusional Kurtosis Imaging in Differentiating Neuromyelitis Optica-Related and Multiple Sclerosis-Related Acute Optic Neuritis: Comparison With Diffusion-Weighted Imaging. J Comput Assist Tomogr 2020;44:47-52. [Crossref] [PubMed]
- Haykal S, Jansonius NM, Cornelissen FW. Investigating changes in axonal density and morphology of glaucomatous optic nerves using fixel-based analysis. Eur J Radiol 2020;133:109356. [Crossref] [PubMed]
- Chan NCY, Chan CKM. The use of optical coherence tomography in neuro-ophthalmology. Curr Opin Ophthalmol 2017;28:552-7. [Crossref] [PubMed]
- Iorga RE, Moraru A, Ozturk MR, Costin D. The role of Optical Coherence Tomography in optic neuropathies. Rom J Ophthalmol 2018;62:3-14.
- Sihota R, Sony P, Gupta V, Dada T, Singh R. Comparing glaucomatous optic neuropathy in primary open angle and chronic primary angle closure glaucoma eyes by optical coherence tomography. Ophthalmic Physiol Opt 2005;25:408-15. [Crossref] [PubMed]
- Ratchford JN, Quigg ME, Conger A, Frohman T, Frohman E, Balcer LJ, Calabresi PA, Kerr DA. Optical coherence tomography helps differentiate neuromyelitis optica and MS optic neuropathies. Neurology 2009;73:302-8. [Crossref] [PubMed]
- Sujanthan S, Shmuel A, Mendola JD. Resting-state functional MRI of the visual system for characterization of optic neuropathy. Front Hum Neurosci 2022;16:943618. [Crossref] [PubMed]
- Sujanthan S, Shmuel A, Mendola JD. Visually driven functional MRI techniques for characterization of optic neuropathy. Front Hum Neurosci 2022;16:943603. [Crossref] [PubMed]