Experimental study on the effects of different exposure conditions and contrast agent concentrations on spectral computed tomography virtual non-contrast images
Introduction
In recent years, with the aging of the population and changes in human lifestyles, human diseases caused by thrombosis and fat embolism, such as acute myocardial infarction, pulmonary embolism, and fat embolism syndrome, which seriously jeopardize human life and health, have become more common (1,2). Prompt diagnosis and early treatment are key to improving prognosis. Computed tomography (CT) is a well-established tissue imaging technique that uses an X-ray beam to scan the human body and is often used in medical examinations. CT scanning is one of the most common means of diagnosing blood clots and fat emboli. True non-contrast (TNC) imaging is inexpensive and fast. It can quickly diagnose fat emboli; however, it is limited in the diagnosis of blood clots.
Enhanced scans have a high spatial resolution, can accurately show normal organs and abnormal lesions, and can clearly show filling defects in blood vessels, so enhanced scans can be used to diagnose blood clots. To avoid the interference of contrast agents, conventional enhancement scans are performed by TNC and three-phase scanning, which results in superimposed scans. The radiation doses of superimposed scans cannot be ignored, and ionizing radiation may have important effects on vital biochemical structures and functions of the human body. According to the “as low as reasonably achievable” principle of optimization of radiation protection, the radiation dose should be minimized without compromising the image quality and clinical diagnosis (3).
With the continuous development of CT technology, new detectors with higher spatial resolution have become popular. Spectral CT is an energy CT imaging device based on a dual-layer detector, which can simultaneously receive high-energy and low-energy X-ray photons, and accurately separate mixed energies in X-ray scans (4). Spectral CT can simultaneously generate conventional images and spectral images, and meets the three core technical requirements of “simultaneous”, “homologous”, and “homogeneous” energy imaging (5-8). Spectral CT can also reconstruct multiple spectral images, provide more parametric information than conventional CT, improve the sensitivity of diagnosing disease, and perform lesion component identification and quantification (9-11).
Spectral CT is also able to distinguish between different components of a substance (e.g., iodine), and the effects of the iodine value can be removed by “iodine-water separation” (12). Thus, spectral CT-enhanced imaging can virtually remove the element of iodine to obtain an image similar to the TNC image without iodine. This type of imaging is called virtual non-contrast (VNC) imaging. VNC images can show the original Hounsfield unit (HU) values of all tissues except iodized tissues. VNC imaging is an important research direction in spectral CT scanning technology, as it can reduce the radiation dose by minimizing the need for repeated scans, and is widely used in clinical practice, especially for thoracic, and abdominal organs (13). Previous studies have shown that the CT values of VNC images are consistent with those of TNC images, so the VNC can be used as an alternative to TNC images (4). However, the quality of VNC images varies. Very few studies have examined the best contrast concentration and exposure conditions for quality VNC images (4). Thus, this study sought to investigate the effects of different exposure conditions and contrast concentrations on the quality of VNC images of blood clots and fat.
Methods
Materials
Fresh pig large intestine was purchased from the supermarket. It was divided into four sections, and the inner wall grease was removed. Duck blood clots (3.0 cm × 3.0 cm × 1.5 cm) and fat grease (2.0 cm × 2.0 cm × 1.0 cm) from the same source and of the same size were put into each of the four sections of the pig large intestine. Next, 50 mL of the non-ionic contrast agent (Ioversol injection, Aisuxian, Jiangsu Hengrui Pharmaceutical Corp., China, 100 mL, 350 mgI/mL) was diluted with normal saline at three concentrations of 15, 10 and 5 mgI/mL. The three concentrations were injected into three sections of the pig large intestine, which were labeled as group A (15 mgI/mL), group B (10 mgI/mL), and group C (5 mgI/mL), and saline was injected into the fourth section of the pig large intestine and labeled as group D (the control group) (Figure 1). The four groups of the pig large intestine were uniformly placed and fixed in a foam box filled with water, and the enhancement scans and TNC scans were simulated with different contrast concentrations.
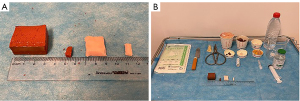
Scanning method
Scanning was performed using a Philips dual-layer spectral detector CT (IQon spectral CT, Philips Medical System, the Netherlands). The scanning range of the foam box was consistent under different exposure conditions. The scanning parameters were as follows: fixed tube voltage: 120 kVp; exposure: 200, 100, 50, and 20 mAs; pitch factor: 0.985, rotation speed: 0.5 s/r; scan length: 260 mm; matrix: 512 × 512; field of view: 320 mm2; reconstructed images: standard mode; thickness and spacing of the reconstructed layers: 1 mm.
Image post-processing
The obtained conventional images were imported into the Philips IntelliSpace Portal workstation (Version 10.1, Philips Medical System) for post-processing. The four sections of the pig large intestine were scanned under four different exposure conditions, and the obtained TNC images were labeled as the TNC200 mAs, TNC100 mAs, TNC50 mAs, and TNC20 mAs groups. The VNC images (VNCA, VNCB, and VNCC) were reconstructed based on the spectral CT images scanned by the three groups of models A, B, and C.
A CT imaging physician with five years of clinical experience outlined the region of interest (ROI) of lesion, and three adjacent levels of the lesion were outlined using copy-and-paste ROIs to ensure that the ROIs at the outlined levels were consistent in terms of the location, shape, and size. The ROI areas of the duck blood, fat, and water were approximately 200, 50, and 100 mm2, respectively. The CT imaging physician measured their CT and standard deviation (SD) values, and calculated the contrast noise ratios (CNRs) using the following formula (14):
Subjective evaluation
Two diagnostic imaging doctors with more than 10 years of work experience independently analyzed the TNC and VNC images using the double-blind method and scored the image quality. If any disagreement arose, a third senior physician with more than 20 years of work experience analyzed these images, and an agreement was reached. The subjective scoring was performed using a three-point method, under which 3 points indicated clear lesion display and anatomical details, and no obvious artifacts or noise, 2 points indicated fair lesion display and anatomical details, and artifacts and noise, and 1 point indicated poor lesion display and anatomical details, and severe artifacts and noise (15).
Statistical analysis
All the statistical analyses were conducted by using the Statistical Package for the Social Sciences software package (SPSS version 21.0, IBM Corp., USA), setting the priori significance level at P<0.05. The Kolmogorov-Smirnov and Levene methods were used to test the normality and variance homogeneity of the measurement data; the measurement data were expressed as the mean ± SD; the count data were expressed as the frequency. The measurement data and count data between multiple groups that did not satisfy variance homogeneity were analyzed by the Wilcoxon signed rank-sum test. Bland-Altman scatter plots were used to analyze the agreement between the tissue CT values in the TNC and VNC images, with the TNC measurements as the X-axis, the difference between the TNC and VNC images as the Y-axis, and the limits of agreement (LOA) as the median of the difference (mean) ±1.96 SD; an out-of-bounds percentage of less than 5% indicated good agreement between the TNC and VNC images (13). The Kappa test was used to examine the consistency of the subjective scores; a K value <0.4 indicated poor consistency between the TNC and VNC images; a K value of 0.4–0.75 indicated fair consistency; and a K value ≥0.75 indicated good consistency. A P value <0.05 was considered statistically significant.
Results
Comparison of the quantitative parameters between the VNC and TNC images at different concentrations
The CT value of the duck blood in the images of the VNCC group was higher than that of the TNCD group, and the difference was statistically significant (P<0.05). The CT values of the fat in the images of the VNCA, VNCB, and VNCC groups were higher than that of the TNCD group, and the differences were all statistically significant (P<0.05). The SD values of the duck blood and fat in the images of the VNCA, VNCB, and VNCC groups were lower than those of the TNCD group, and the CNR values were higher than those of the TNCD group, and the differences were all statistically significant (P<0.05). The CT values of the duck blood in the images of the VNCA and VNCB groups did not differ significantly compared with those in the images of the TNCD group (P>0.05) (Table 1, Figure 2).
Table 1
Parameters | Blood clot | Fat | |||||
---|---|---|---|---|---|---|---|
CT | SD | CNR | CT | SD | CNR | ||
TNCD | 2.64±1.43 | 6.97±2.67 | 2.16±0.38 | −105.86±2.29 | 7.04±3.14 | 17.55±8.17 | |
VNCA | 2.41±3.82 | 5.82±1.77 | 3.64±2.09 | −95.64±1.90 | 5.58±1.66 | 22.81±10.45 | |
VNCB | 2.58±1.43 | 5.81±2.82 | 2.98±1.37 | −90.53±1.44 | 5.83±2.31 | 21.55±10.35 | |
VNCC | 4.64±2.90 | 4.70±1.61 | 4.21±2.14 | −90.01±3.22 | 5.50±2.55 | 21.30±9.88 | |
Z | |||||||
TNCD-VNCA | −0.31 | −2.20 | −2.20 | −3.06 | −1.04 | −3.06 | |
TNCD-VNCB | −1.47 | −2.59 | −2.35 | −3.06 | −2.13 | −2.90 | |
TNCD-VNCC | −2.51 | −3.06 | −2.93 | −3.06 | −2.82 | −3.06 | |
P | |||||||
TNCD-VNCA | 0.75 | 0.03 | 0.03 | 0.01 | 0.04 | 0.01 | |
TNCD-VNCB | 0.14 | 0.01 | 0.02 | 0.01 | 0.03 | 0.01 | |
TNCD-VNCC | 0.01 | 0.01 | 0.01 | 0.01 | 0.01 | 0.01 |
A, B, C, and D indicate 15 mgI/mL, 10 mgI/mL, 5 mgI/mL and the control group. Data are expressed as mean ± SD. VNC, virtual non-contrast; TNC, true non-contrast; CT, computed tomography; SD, standard deviation; CNR, contrast noise ratio.
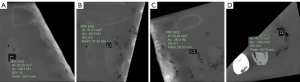
Comparison of the quantitative parameters between the VNC and TNC images under different exposure amounts
Under different exposure conditions, the CT values of the fat in the VNC images were higher than those of the TNC images; the SD values of the duck blood and fat in the VNC images were lower than those in the TNC images, and the differences were all statistically significant (P<0.05). The CNR values of the fat in the VNC images were higher than those in the TNC images under different exposures; the CNR values of the duck blood in the VNC200 mAs, VNC100 mAs, and VNC50 mAs images were higher than those in the TNC images; the CNR value of the duck blood in the VNC20 mAs images was lower than those in the TNC images, and the differences were all statistically significant (P<0.05) (Table 2).
Table 2
Parameters | Blood clot | Fat | |||||
---|---|---|---|---|---|---|---|
CT | SD | CNR | CT | SD | CNR | ||
TNC200 mAs | 2.53±0.35 | 4.23±0.13 | 2.24±0.11 | −105.13±0.26 | 3.20±0.30 | 30.68±0.08 | |
VNC200 mAs | 3.76±2.46 | 3.46±0.79 | 4.29±1.06 | −92.18±2.61 | 2.53±0.60 | 36.89±1.12 | |
TNC100 mAs | 4.67±0.59 | 5.53±0.28 | 2.55±0.09 | −106.17±1.63 | 5.53±0.63 | 14.51±0.25 | |
VNC100 mAs | 5.63±3.03 | 4.23±0.65 | 6.08±0.09 | −93.98±2.93 | 4.79±0.71 | 23.48±0.87 | |
TNC50 mAs | 1.73±0.87 | 7.17±0.96 | 2.28±0.14 | −104.67±1.77 | 8.50±0.92 | 14.92±0.29 | |
VNC50 mAs | 1.74±0.75 | 5.66±0.93 | 2.69±0.16 | −91.09±4.87 | 6.16±0.99 | 16.64±1.01 | |
TNC20 mAs | 1.63±0.74 | 10.93±0.5 | 1.59±0.08 | −107.47±3.24 | 10.93±0.85 | 10.06±0.35 | |
VNC20 mAs | −0.81±2.03 | 8.42±1.40 | 1.38±0.27 | −91.00±2.36 | 8.47±0.69 | 10.54±0.31 | |
Z | |||||||
Za | −1.13 | −2.08 | −2.67 | −2.67 | −2.25 | −2.67 | |
Zb | −0.77 | −2.55 | −2.67 | −2.67 | −2.08 | −2.67 | |
Zc | −0.06 | −2.19 | −2.67 | −2.67 | −2.67 | −2.67 | |
Zd | −2.43 | −2.55 | −2.10 | −2.67 | −2.67 | −2.43 | |
P | |||||||
Pa | 0.26 | 0.04 | 0.01 | 0.01 | 0.02 | 0.01 | |
Pb | 0.44 | 0.01 | 0.01 | 0.01 | 0.04 | 0.01 | |
Pc | 0.95 | 0.03 | 0.01 | 0.01 | 0.01 | 0.01 | |
Pd | 0.02 | 0.01 | 0.04 | 0.01 | 0.01 | 0.02 |
a, b, c, d represent TNC200 mAs-VNC200 mAs, TNC100 mAs-VNC100 mAs, TNC50 mAs-VNC50 mAs, TNC20 mAs-VNC20 mAs, respectively. Data are expressed as mean ± SD. VNC, virtual non-contrast; TNC, true non-contrast; CT, computed tomography; SD, standard deviation; CNR, contrast noise ratio.
Subjective ratings of image quality
Under the four exposure conditions, the two physicians had good agreement on the subjective evaluations of the duck blood and fat in the TNC and VNC images (Kappa value =0.80). The subjective scores of the two physicians for the VNC and TNC images were 2.31±0.70 and 2.19±0.75, respectively. The subjective scores (the average of the subjective scores of the two physicians) for the TNC and VNC images were 2.00±0.74 and 2.25±0.75, respectively, and the difference was not statistically significant (z=−1.34, P=0.18).
CT value consistency analysis
Compared with the TNC images, the average difference in the CT values of the blood clots and fat in the VNC images were 0.1 and −13.8 HU, respectively. The proportion of data points with VNC-TNC differences in the duck blood and fat tissues, which were outside the LOA boundary, was ≤5%, which indicated that there was a better consistency in the CT values of the tissues between the two images (Figure 3).
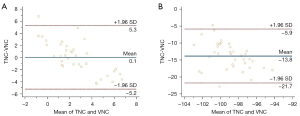
Discussion
Previous study (13) has shown that spectral CT can be used to reconstruct multiple spectral images, such as virtual single-energy level images, VNC images, iodine-density images, and effective atomic number images. VNC can reduce a TNC scan, VNC can reduce the radiation dose, shorten the examination time, reduce image noise, and improve the signal-to-noise ratio (16). In addition, the CT values in the VNC images did not differ greatly from those in the TNC images, so VNC images can effectively replace TNC images. To date, few studies have explored the effects of different contrast concentrations and different exposures on the quality of VNC images. Since very low-density gas emboli and high-density emboli are easily recognized by intracavitary blood contrast concentration in CT scanning, this study explored the effects of different exposure conditions and contrast concentrations on the image quality of spectral CT virtual scans of blood clots and fat using duck blood and pork fat (17,18).
The premise of replacing TNC scanning with VNC technology of double-layer detector spectral CT is whether the VNC image truly reflects the CT value and lesion condition of TNC image, and whether it can meet the requirements of imaging diagnosis. Among these parameters, the SD and CNR values are important for evaluating image quality, with lower SD values and higher CNR values representing higher image quality. Thus, this study investigated which exposure and concentration conditions produced the best quality reconstructions of the VNC images. Our findings will help us to choose the appropriate concentrations (e.g., in the arterial, venous, and delayed phases) and exposures to reconstruct VNC images in our clinical work.
The results of the study showed that the CNR value of the duck blood in the VNC20 mAs group was lower than that of the TNC group, and the CNR values of the duck blood and fat in the VNC group were higher than those of the TNC group in the remaining different exposure and concentration groups. The SD values of the duck blood and fat in the VNC group were lower than those of the TNC group in the different concentration and exposure groups, which may be related to the spectral reconstruction algorithm of the spectral CT and the noise suppression technique (19). The CT values of the duck blood in the VNC20 mAs and VNCC groups were lower than those of the TNC group, and the difference was statistically significant. There were no statistically significant differences between the CT values of the duck blood in the VNC and TNC images under the residual exposure (200, 100, and 50 mAs) and concentration (15 and 10 mgI/mL) conditions. This may be because low exposure and concentration result in reduced image resolution and large errors in the measurements. The CT values of the fat in the VNC images with different concentrations and different exposures were higher than those in the TNC images, and the differences were statistically significant, which is consistent with the results of Yang et al. (4). It may be that the existence of the partial volume effect and sclerosis effect in the enhanced scan makes the absolute CT value of the final measurement of the fat lower and the CT value of the fat itself negative. Thus, fat components or fat infiltration will increase the CT values of VNC images.
The Bland-Altman analysis showed that the difference in the CT number between the TNC and VNC images for fat and clots was ≤5% at points outside the LOA boundaries; thus, the CT consistency was good. Sauter et al. (20) concluded that the difference in CT values between VNC and TNC images was negligible at 10 HU or less, and that differences of 10 to 15 HU were acceptable. In the measurement of blood clots, the differences in the CT values between the VNC and TNC images were 97% <5 HU and 100% <10 HU, respectively. In the measurement of fat, the differences in CT values between the VNC and TNC images were 64% <15 HU. In this study, the differences between the subjective scores of the VNC and TNC images were not statistically significant, and the agreement between the CT values of low-density material in the VNC and TNC images was good. This indicates that spectral CT has a better deiodination effect, which reduces the density of the lesion and shows the lesion clearly.
This study had several limitations. First, the sample size was relatively small, and the data were only analyzed for lesions under three different concentration conditions and four different exposure conditions, which limits the generalizability of the results. A study with a larger sample size will be conducted in the future to further validate the accuracy and reliability of the method. Second, the VNC image quality is highly dependent on the post-processing software and its algorithms. The post-processing of spectral CT can be performed either before or after high-/low-energy reconstruction; the former reduces beam-hardening artifacts but requires higher computational power; the latter is easier to process the reconstructed images but requires correction of beam-hardening artifacts. In future studies, larger sample sizes will be used to provide strong support for VNC images replacing TNC images.
Conclusions
In summary, the study showed that the double-layer detector spectral CT can effectively restore the CT value of the clot under the different exposure conditions and contrast concentrations, except for 20 mAs exposure and 5 mgI/mL contrast concentration. Spectral CT can effectively reduce the noise and improve the signal-to-noise ratio of VNC images under the different exposure conditions and contrast concentrations, except for 20 mAs exposure. In addition, the CT values of the fat and duck blood had good agreement between the TNC and VNC images. Thus, when we reconstruct VNC images using spectral CT, we should choose time phases with higher contrast concentrations (e.g., the arterial and venous phases) and the right amount of exposures (e.g., 200, 100, and 50 mAs). As the radiation dose increases as exposure increases, a relatively low exposure of 50 mAs should be selected for clinical scanning, which should effectively reduce the radiation dose to the patient and reduce the scanning time.
Acknowledgments
Funding: None.
Footnote
Conflicts of Interest: All authors have completed the ICMJE uniform disclosure form (available at https://qims.amegroups.com/article/view/10.21037/qims-23-1092/coif). The authors have no conflicts of interest to declare.
Ethical Statement: The authors are accountable for all aspects of the work in ensuring that questions related to the accuracy or integrity of any part of the work are appropriately investigated and resolved.
Open Access Statement: This is an Open Access article distributed in accordance with the Creative Commons Attribution-NonCommercial-NoDerivs 4.0 International License (CC BY-NC-ND 4.0), which permits the non-commercial replication and distribution of the article with the strict proviso that no changes or edits are made and the original work is properly cited (including links to both the formal publication through the relevant DOI and the license). See: https://creativecommons.org/licenses/by-nc-nd/4.0/.
References
- Campello E, Spiezia L, Adamo A, Simioni P. Thrombophilia, risk factors and prevention. Expert Rev Hematol 2019;12:147-58. [Crossref] [PubMed]
- China Embolism-thrombosis after Surgery of Thorax Study Group, Hui L, Gening J. Guideline for the prevention and management of perioperative venous thromboembolism in thoracic malignancies in China(2022 version). Chinese Journal of Surgery 2022;60:721-31. [Crossref] [PubMed]
- Chen W, Yin K, Li M, Kang L, Yu H, Liang J, Wu M, Kashif D, Chen X, Sheng Z, Mu D. Feasibility study of reducing the radiation dose on virtual non-contrast scanning in dual-layer spectral coronary CT angiography. Chinese Journal of Radiological Medicine and Protection 2022;42:61-6.
- Yang Y, Yan F, Han Q, Chen X, Dong H. Feasibility study on dual-layer spectral detector CT-derived virtual non-contrast images substitute for true non-contrast images. Chinese Journal of Radiology 2019;53:33-9.
- Lee HA, Lee YH, Yoon KH, Bang DH, Park DE. Comparison of Virtual Unenhanced Images Derived From Dual-Energy CT With True Unenhanced Images in Evaluation of Gallstone Disease. AJR Am J Roentgenol 2016;206:74-80. [Crossref] [PubMed]
- Zhong J, Pan Z, Chen Y, Wang L, Xia Y, Wang L, Li J, Lu W, Shi X, Feng J, Yan F, Zhang H, Yao W. Robustness of radiomics features of virtual unenhanced and virtual monoenergetic images in dual-energy CT among different imaging platforms and potential role of CT number variability. Insights Imaging 2023;14:79. [Crossref] [PubMed]
- Langenbach IL, Wienemann H, Klein K, Scholtz JE, Pennig L, Langzam E, Pahn G, Holz JA, Maintz D, Naehle CP, Langenbach MC. Coronary calcium scoring using virtual non-contrast reconstructions on a dual-layer spectral CT system: Feasibility in the clinical practice. Eur J Radiol 2023;159:110681. [Crossref] [PubMed]
- Hur J, Lee ES, Park HJ, Choi W, Park SB. Diagnostic performance of dual-energy computed tomography for HCC after transarterial chemoembolization: Utility of virtual unenhanced and low keV virtual monochromatic images. Medicine (Baltimore) 2022;101:e31171. [Crossref] [PubMed]
- Chinese Journal of Radiology Cooperative Group of Clinical Application of Dual-layer Spectral Detector CT. China expert consensus on clinical application of dual-layer spectral detector CT (first edition). Chinese Journal of Radiology 2020;54:635-43.
- Zhu X, Chen C, Wei X, Zhang H, Lv P, Yu H, Liu S, Li M, Hu A, Zhu B, Zhang B. Study on CT Attenuation Consistency of Liver and Adrenal Glands by Spectral CT Virtual Non-Contrast Images. Journal of Clinical Radiology 2021;40:354-8.
- Fu L, Pan X, Liu S, Li Q, Yang Y. Feasibility analysis of double-layer spectral detector CT virtual non-contrast images instead of true non-contrast images in evaluating thyroid nodules. Radiologic Practice 2022;37:302-6.
- Deng XQ, Duan SW, Xiao DL, Ou YF, Luo WQ. The value of virtual non-contrast CT images for follow-up of patients with surgically clipped intracranial aneurysms. J Pract Radiol 2022;38:1229-32,1251.
- Jamali S, Michoux N, Coche E, Dragean CA. Virtual unenhanced phase with spectral dual-energy CT: Is it an alternative to conventional true unenhanced phase for abdominal tissues? Diagn Interv Imaging 2019;100:503-11. [Crossref] [PubMed]
- Yang Z, Chen X, Chen X. The application of spectral virtual non-contrast CT in thyroid diseases. Journal of Practical Radiology 2019;35:455-8.
- Li DX, Fu ZH, Du C, Xu CJ, Tian YT. Application of CT virtual non-contrast scanning of double-layer spectral detector in the detection of hepatocellular carcinoma. Shandong Medical Journal 2020;60:70-2.
- Rajiah P, Parakh A, Kay F, Baruah D, Kambadakone AR, Leng S. Update on Multienergy CT: Physics, Principles, and Applications. Radiographics 2020;40:1284-308. [Crossref] [PubMed]
- Chappard C, Abascal J, Olivier C, Si-Mohamed S, Boussel L, Piala JB, Douek P, Peyrin F. Virtual monoenergetic images from photon-counting spectral computed tomography to assess knee osteoarthritis. Eur Radiol Exp 2022;6:10. [Crossref] [PubMed]
- Michael AE, Boriesosdick J, Schoenbeck D, Woeltjen MM, Saeed S, Kroeger JR, Horstmeier S, Lennartz S, Borggrefe J, Niehoff JH. Image-Quality Assessment of Polyenergetic and Virtual Monoenergetic Reconstructions of Unenhanced CT Scans of the Head: Initial Experiences with the First Photon-Counting CT Approved for Clinical Use. Diagnostics (Basel) 2022;12:265. [Crossref] [PubMed]
- Lin Y, Zhang XX, Zhang YB, Wang JA, Cao DR, Chen XB. Application of dual-layer spectral detector CT virtual non-contrast images in hepatic triple-phase enhanced scan. Chin J Med Imaging Technol 2020;36:29-33.
- Sauter AP, Muenzel D, Dangelmaier J, Braren R, Pfeiffer F, Rummeny EJ, Noël PB, Fingerle AA. Dual-layer spectral computed tomography: Virtual non-contrast in comparison to true non-contrast images. Eur J Radiol 2018;104:108-14. [Crossref] [PubMed]