The differential diagnosis of lung precursor glandular lesions, micro-invasive adenocarcinoma, and invasive adenocarcinoma using low dose spectral computed tomography perfusion imaging
Introduction
Lung cancer is the most prevalent malignant tumor with the highest morbidity and mortality rates in adults worldwide (1-3). Lung adenocarcinoma (LUAD) is the most common histological type of lung cancer, which is divided into precursor glandular lesions, minimally invasive adenocarcinoma (MIA), and invasive adenocarcinoma (IAC), according to the 2011 International Association for the Study of Lung Cancer (IASLC), American Thoracic Society (ATS) and European Respiratory Society (ERS), and 2021 World Health Organization (WHO) new classification of LUAD (4,5). Precursor glandular lesions are further divided into atypical adenomatous hyperplasia (AAH) and adenocarcinoma in situ (AIS). The invasiveness, clinical treatment, and prognosis differ among different pathological types. The overall survival rate has been reported at almost 100% for both precursor glandular lesions and MIA with complete resection; however, the prognosis of IAC is poor, with a 5-year survival rate of 49–84% (6,7). Traditional chest computed tomography (CT) can be used to evaluate the invasiveness of LUAD or the prognosis based on the morphology of the lesion edge (such as burr, lobulation), ground-glass density ratio, solid component size, and so on (8). The hemodynamic alterations of pulmonary lesions can be evaluated with non-invasive functional CT perfusion imaging techniques (9,10). The perfusion parameters include blood flow (BF), blood volume (BV), impulse residue function time of arrival (IRF TO), maximum slope of increase (MSI), mean transit time (MTT), permeability surface area product (PS), positive enhancement integral (PEI), time to peak (TTP), and maximum enhancement time (Tmax). To evaluate the hemodynamic characteristics of pulmonary nodules objectively, a new CT mode was established through quantitative multi-parameter analysis, which provides an alternative to evaluate invasive LUAD (4). Previous studies have mainly focused on the differentiation of benign and malignant pulmonary nodules, the differentiation of different pathological types of lung cancer, and the efficacy evaluation of LUAD. There is no available study on the relationship between CT perfusion parameters and different subtypes of LUAD (11-13).
The purpose of this study was to investigate the value of low dose CT perfusion imaging parameters (BF, BV, IRF TO, MSI, MTT, PS, PEI, TTP, and Tmax) to differential diagnosis of different pathological subtypes of LUAD. We present this article in accordance with the STROBE reporting checklist (available at https://qims.amegroups.com/article/view/10.21037/qims-23-487/rc).
Methods
Subjects
The study was conducted in accordance with the Declaration of Helsinki (as revised in 2013). The study was approved by the Medical Ethics Committee of Peking University Cancer Hospital & Institute (No. 2021KT04), and written informed consent was provided by all patients.
This was a cross-sectional study based on historical data. In this study, a total of 62 cases were enrolled in Peking University Cancer Hospital & Institute from January 2018 to May 2019. The inclusion criteria were as follows: (I) aged over 18 years, males or female; (II) diameter of the lesion greater than 6 mm; and (III) the diagnosis was confirmed with surgical pathology and biopsy. The exclusion criteria were as follows: (I) history of contrast agent allergy; (II) severe liver and renal insufficiency, thyroid toxicity; and (III) pregnant and lactating women. The detailed inclusion and exclusion criteria for patient selection are shown in Figure 1.
Preparation before examination
Before spectral CT perfusion scanning, the cases were informed of the specific examination process, precautions, and potential complications. After removing all foreign bodies that may interfere the chest scan, a blue indwelling needle was placed in the right anterior elbow vein of the patient. The chest and abdomen were fixed with a belt, and the subject was instructed to breathe calmly to reduce respiratory motion artifacts on the image.
Scan technique
Revolution Xtream CT (GE Healthcare, Waukesha, WI, USA) was used. Cases were scanned from the apex of the lung to the diaphragm at the bottom of the lung in direction from head to foot. A routine chest scan was performed first before CT perfusion imaging scanning was taken. The technical parameters of the CT scans were as follows: tube voltage 120 kVp, automatic milliampere technology, pitch 0.984, noise index 9.00, rotation time of X-ray tube ball 0.6 s per circle, scan field of view (SFOV) =500 mm, collimator width 40 mm, layer thickness 5 mm, and matrix 512×512. The group injection method was used in the right elbow median vein. At 5 seconds after injection of contrast medium, a continuous dynamic scanning of the local target lesion was performed, with a range of 16 mm and a duration of 40 seconds, and the dynamic perfusion basic image was obtained. Non-ionic iodine contrast agent iohexol Injection (300 mgI/mL) was used as contrast agent, and the injection flow rate and injection dosage were determined based on previous studies (12,14), with adjustment for the Chinese population. For cases with a body weight equal to or less than 70 kg, a dosage of 40 mL and an injection rate of 5 mL/s were used; for cases with a body weight more than 70 kg, a dosage of 50 mL and an injection rate of 6 mL/s were used. This was followed by a 30 mL physiological saline injection at the same rate, which is conducive to reflecting the blood perfusion state of lesions.
Image post-processing
The original basic image of dynamic perfusion imaging was transmitted to GE AW4.7 workstation for image post-processing. Perfusion images of pulmonary nodules were analyzed by two senior radiologists with Perfusion 4 analysis software (GE Healthcare). The aorta or main branch at the same level was selected as the feeding artery, and the region of interest (ROI) of pulmonary nodules and aorta were drawn respectively. The ROI was placed on the maximum cross section of the lesion, with an area of 1/3–2/3 of the lesion, avoiding liquefaction, necrosis, bleeding, calcification, and pulmonary vessels and bronchus. All tumors were assessed twice with an interval of 1 week, and the average value was calculated. The following CT perfusion imaging parameters of pulmonary nodule were obtained: BF, BV, IRF TO, MSI, MTT, PS, PEI, TTP, and Tmax.
Pathology evaluation
Biopsied lesion specimens were fixed with 10% neutral formalin, routinely embedded in paraffin, continuously sliced with 4 µm thickness, and stained with hematoxylin and eosin (HE). After HE staining, two senior pathologists (Wei Sun, MD and Xin Yang, MD, both with 12 years of experience in pathological diagnosis of lung cancer) confirmed the diagnosis without any information regarding the patients. The histological morphology was evaluated according to the 2011 IASLC/ATS/ERS LUAD standard and the 2021 WHO histological classification of lung tumors, and a comprehensive histological classification was performed. The lesions with diameter ≤3 cm and tumor cells growing on alveolar wall without interstitial, vascular, and pleural infiltration were defined as AIS. The lesions with diameter ≤3 cm, tumor cells adherent to the alveolar wall, interstitial infiltration range ≤5 mm, and no vascular and pleural infiltration were defined as MIA. The range of interstitial infiltration >5 mm was classified as IAC (5). Concurrently, immunohistochemical envision two-step method was used to detect antibodies P40, TTF-1, Napsin A, and Ki67.
Statistical analysis
The measurement data were expressed as mean ± standard deviation. Intraclass correlation coefficients (ICCs) were used to assess the reliability of perfusion parameters. ICCs less than 0.5, between 0.5 and 0.75, between 0.75 and 0.9, and greater than 0.90 are indicative of poor, moderate, good, and excellent reliability, respectively (15). Parameters with ICC ≥0.75 were included for further analysis. The quantitative parameters BF, BV, IRF TO, MSI, MTT, PS, PEI, TTP, and Tmax of spectral CT perfusion imaging of LUAD nodules in three groups (AIS, MIA, IAC) were compared by one-way analysis of variance (ANOVA) or Kruskal-Wallis test. Comparison was made between groups using Bonferroni method. The P value was corrected by Bonferroni method for multiple comparisons between groups. Mann-Whitney test was used to compare the parameters of CT perfusion imaging between the two groups of LUAD: precursor glandular lesions (AAH and AIS) and invasive lung cancer (MIA and IAC). All P values were two-sided. A P value <0.05 was considered statistically significant. All data analyses were performed using the software SPSS 18.0 (IBM Corp., Chicago, IL, USA).
Results
Clinical results
Thus, a total of 62 patients including 26 men and 36 women [median age 60 years, interquartile range (IQR) 42–81 years] were enrolled, including 23 cases of solid density and 39 cases of ground-glass density (Table 1). Pathological results showed AAH in 2 cases (3.2%), AIS in 3 cases (4.8%), MIA in 4 cases (6.5%), and IAC in 53 cases (85.5%). All lung cancers were confirmed by biopsy or surgical pathology.
Table 1
Clinical features | Number |
---|---|
Age (median, years) | 60 |
Sex, n (%) | |
Female | 36 (58.1) |
Male | 26 (41.9) |
Smoking, n (%) | |
Yes | 20 (32.3) |
No | 42 (67.7) |
Density, n (%) | |
Pure GGN | 5 (8.1) |
Mixed GGN | 34 (54.8) |
Solid | 23 (37.1) |
Pathological classification, n (%) | |
AAH | 2 (3.2) |
AIS | 3 (4.8) |
MIA | 4 (6.5) |
IAC | 53 (85.5) |
GGN, ground-glass nodule; AAH, atypical adenomatous hyperplasia; AIS, adenocarcinoma in situ; MIA, micro-invasive adenocarcinoma; IAC, invasive adenocarcinoma.
Comparison of quantitative parameters of AIS, MIA, and IAC of lung cancer in spectral CT perfusion imaging
All the perfusion parameters were highly reliable (ICCs ≥0.75). The comparison results of spectral CT perfusion imaging parameters of AIS, MIA, and IAC are shown in Tables 2-4 and Figures 2-4. For the quantitative parameters of spectral CT perfusion imaging among AIS, MIA, and IAC, there were significant differences in IRF TO value among the three groups (0.56±0.74 vs. 0.54±1.08 vs. 4.39±2.19 s, P=0.004). It was gradually increased in AISs or MIAs, compared with IACs (Table 2, Figures 2-4). There was no missing data for each variable of interest.
Table 2
Parameters | AAH (n=2) | AIS (n=3) | MIA (n=4) | IAC (n=53) | P value |
---|---|---|---|---|---|
BF (mL/min/100 g) | 11.92 | 91.63±80.16 | 74.55±90.06 | 55.27±42.42 | 0.703 |
BV (min/100 g) | 0.15 | 2.98±2.64 | 2.38±2.88 | 2.49±2.18 | 0.629 |
IRF TO (s) | 2.79 | 0.56±0.74 | 0.54±1.08 | 4.39±2.19 | 0.004 |
MSI (%) | 6.63 | 6.23±2.13 | 17.98±14.81 | 12.98±7.95 | 0.440 |
MTT (s) | – | 2.41±0.22 | 2.63±0.90 | 5.43±3.46 | 0.437 |
PS (mL/min/100 g) | 1.05 | 14.92±5.01 | 16.86±19.64 | 14.13±18.45 | 0.563 |
PEI (HU) | 25.95 | 0.07±0.08 | 0.10±0.13 | 0.34±0.43 | 0.642 |
Tmax (s) | 0.12 | 1.83±1.88 | 3.68±6.37 | 6.26±3.28 | 0.225 |
TTP (s) | 9.54 | 12.77±11.36 | 17.35±9.96 | 17.75±7.96 | 0.905 |
The data are presented by mean ± SD. CT, computed tomography; AIS, adenocarcinoma in situ; MIA, micro-invasive adenocarcinoma; IAC, invasive adenocarcinoma; AAH, atypical adenomatous hyperplasia; BF, blood flow; BV, blood volume; IRF TO, impulse residue function time of arrival; MSI, maximum slope of increase; MTT, mean transit time; PS, permeability surface area product; PEI, positive enhancement integral; HU, Hounsfield units; Tmax, maximum enhancement time; TTP, time to peak; SD, standard deviation.
Table 3
Parameters | MIA + IACs (n=57) | AAH + AISs (n=5) | P value |
---|---|---|---|
BF (mL/min/100 g) | 62.94±63.15 | 71.71±76.63 | 0.985 |
BV (min/100 g) | 2.68±2.53 | 2.27±2.58 | 0.699 |
IRF TO (s) | 3.75±2.79 | 1.12±1.27 | 0.031 |
MSI (%) | 11.83±8.99 | 6.33±1.75 | 0.183 |
MTT (s) | 4.16±3.07 | 1.95±0.80 | 0.201 |
PS (mL/min/100 g) | 13.25±16.16 | 10.24±12.60 | 0.602 |
PEI (HU) | 0.36±0.96 | 0.09±0.07 | 0.900 |
Tmax (s) | 5.16±4.06 | 4.40±4.64 | 0.787 |
TTP (s) | 17.64±10.41 | 13.63±9.66 | 0.826 |
The data are presented by mean ± SD. CT, computed tomography; AAH, atypical adenomatous hyperplasia; AIS, adenocarcinoma in situ; MIA, micro-invasive adenocarcinoma; IAC, invasive adenocarcinoma; BF, blood flow; BV, blood volume; IRF TO, impulse residue function time of arrival; MSI, maximum slope of increase; MTT, mean transit time; PS, permeability surface area product; PEI, positive enhancement integral; HU, Hounsfield units; Tmax, maximum enhancement time; TTP, time to peak; SD, standard deviation.
Table 4
Parameters | IACs (n=53) | AIS + AAH + MIAs (n=9) | P value |
---|---|---|---|
BF (mL/min/100 g) | 61.75±61.25 | 73.13±77.42 | 0.813 |
BV (min/100 g) | 2.71±2.53 | 2.33±2.53 | 0.461 |
IRF TO (s) | 4.12±2.69 | 0.83±1.13 | <0.001 |
MSI (%) | 11.22±8.23 | 12.11±1155 | 0.857 |
MTT (s) | 4.24±3.12 | 2.23±0.81 | 0.138 |
PS (mL/min/100 g) | 12.89±16.03 | 13.55±15.68 | 0.860 |
PEI (HU) | 0.38±1.00 | 0.09±0.10 | 0.968 |
Tmax (s) | 5.27±3.93 | 4.04±5.00 | 0.361 |
TTP (s) | 17.67±10.96 | 15.49±9.30 | 0.374 |
The data are presented by mean ± SD. CT, computed tomography; AAH, atypical adenomatous hyperplasia; AIS, adenocarcinoma in situ; MIA, micro-invasive adenocarcinoma; IAC, invasive adenocarcinoma; BF, blood flow; BV, blood volume; IRF TO, impulse residue function time of arrival; MSI, maximum slope of increase; MTT, mean transit time; PS, permeability surface area product; PEI, positive enhancement integral; HU, Hounsfield units; Tmax, maximum enhancement time; TTP, time to peak; SD, standard deviation.
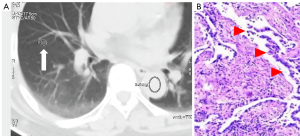
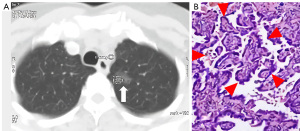
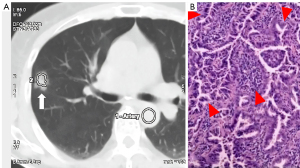
In the precursor glandular lesions (AAH + AIS) and lung invasive carcinomas (MIA + IAC) cohort, 5 precursor glandular lesions and 57 lung invasive carcinomas were assessed. Raw variables for differentiating AAH + AISs from MIA + IACs are shown in Table 3.
The quantitative parameters of IRF TO between AAH + AISs and MIA + IACs were (1.12±1.27 vs. 3.75±2.79 s, P=0.031), IRF TO of AAH + AISs was faster than that of MIA + IACs.
In the 100% disease-specific survival (DSS) lesions (AAH + AIS + MIAs) and IACs cohort, 9 DSS 100% lesions and 53 IACs were assessed (Table 4). These raw variables for differentiating AAH + AIS + MIAs from IACs are shown in Table 4. The IRF TO value of group AAH + AIS + MIAs was also significantly shorter than that of IACs (0.83±1.13 vs. 4.12±2.69 s, P<0.001).
There was no significant difference in BF, BV, MSI, MTT, PS, PEI, TTP, and Tmax among different types of LUAD (P>0.05).
Discussion
According to the 2011 IASLC/ATS/ERS classification of LUAD, the prognosis of LUAD is mainly affected by its pathological invasiveness. At present, it is difficult to obtain the preoperative pathological diagnosis of ground-glass nodules. High-resolution chest CT is often used to estimate the invasiveness of ground-glass nodules, and corresponding treatment measures are formulated. Previous traditional chest CT studies have suggested that the density of nodules, solid components, CT value, ground-glass density ratio, edge burr, lobulation, bronchial gas phase, and pleural traction, among others, can be used to estimate the invasiveness of LUAD (7,16-18). A previous study used texture analysis of high-resolution CT images to distinguish AIS, MIA, and IAC (19). It was possible to differentiate AIS-MIA from IAC using the 90th percentile CT numbers and entropy. Currently, although a few trials have studied the pathological invasion of LUAD using spectral CT imaging, there are no studies that have investigated CT perfusion imaging in the evaluation of pathological invasiveness of LUAD (20,21). It was postulated that the net enhancement value of CT or iodine-based map at a single energy level can be used to evaluate the invasiveness of LUAD (20,21). The net enhancement value of CT or iodine-based image is directly related to the distribution of intravascular and extracellular space in the tumor, which reflect the changes of tumor hemodynamics. Due to the vasculature differences between benign and malignant tumors, the CT parameters related to enhancement of these tumors also reflect the tumor invasiveness. Therefore, enhanced CT value was used to differentiate pulmonary nodules traditionally (22).
Studies of CT perfusion imaging showed that the spectral CT perfusion imaging was superior to that of the traditional CT enhancement value in evaluating the blood perfusion status and the invasiveness of pulmonary nodules. The sensitivity, specificity, and accuracy were 79–91%, 81–96%, and 80–96% respectively, for the differentiation of malignant from benign nodules (9,10). In this study, we discovered that IRF TO value of CT perfusion imaging was significantly different in AIS, MIA, and IAC (P=0.004). Histopathological study of LUAD also found that the microvessel density of different pathological subtypes of LUAD was different, and the microvessel density of solid LUAD was higher than that of non-solid LUAD (23). With the aggravation of the invasion of LUAD, the solid components gradually increased from AIS or MIA to IAC, which may be accompanied by the increase of microvessel density in the tumor. Our results show that there were no significant differences in BF, BV, MSI, MTT, PS, PEI, TTP, and Tmax among AIS, MIA, and IAC. The CT perfusion parameter IRF TO reflects the different changes of invasiveness exactly. The results of this study revealed that IRF TO increases with the increment of the invasiveness of LUAD. This may be attributed to the distinctive vascular distribution among different subtypes of LUAD, and quantitative study of microvessel density is warranted to investigate this issue.
For AIS, MIA, and IAC, IRF TO may indirectly reflect the potential tumor angiogenesis and vasculature distribution, and further indicates the differences of invasiveness of LUAD. In addition, the results of our study suggest that IRF TO contributes to differentiating precursor glandular lesions (AAH and AIS) from invasive LUAD (MIA and AIS) (Tables 2-4), the difference between which was statistically significant. Furthermore, a study had shown that the prognosis of solid LUAD was poor (24). Furthermore, MIA was separated from IAC and according to different prognosis, and combined AAH, and AIS as one group. The IRF TO of this group was significantly lower than that of IAC (P<0.001). These results may indicate that the quantitative parameter IRF TO of spectral CT perfusion imaging may be used to evaluate the prognosis of LUAD, which should be investigated in future studies.
There were some limitations in this study. The sample size, especially the number of AIS cases was too small, which could be attributed to the disease detection rate and the medical treatment rate. The correlation between the parameters of spectral CT perfusion imaging and the ratio of ground-glass component of LUAD was not analyzed in this study. The growth pattern of LUAD, such as adherent type, acinar type, papillary type, or solid growth, may also affect the outcomes, which was not examined in this study. Therefore, further studies should incorporate a larger sample size and more comprehensive pathological subtypes.
Conclusions
Spectral CT perfusion imaging has a potential application in the evaluation of the invasiveness of LUAD. The IRF TO value of spectral CT perfusion imaging increased with the increment of pathological invasiveness of adenocarcinoma, which may help to distinguish between AIS, MIA, and IAC.
Acknowledgments
Funding: This work was supported by
Footnote
Reporting Checklist: The authors have completed the STROBE reporting checklist. Available at https://qims.amegroups.com/article/view/10.21037/qims-23-487/rc
Conflicts of Interest: All authors have completed the ICMJE uniform disclosure form (available at https://qims.amegroups.com/article/view/10.21037/qims-23-487/coif). The authors have no conflicts of interest to declare.
Ethical Statement: The authors are accountable for all aspects of the work in ensuring that questions related to the accuracy or integrity of any part of the work are appropriately investigated and resolved. The study was conducted in accordance with the Declaration of Helsinki (as revised in 2013). The study was approved by the Medical Ethics Committee of Peking University Cancer Hospital & Institute (No. 2021KT04), and written informed consent was provided by all patients.
Open Access Statement: This is an Open Access article distributed in accordance with the Creative Commons Attribution-NonCommercial-NoDerivs 4.0 International License (CC BY-NC-ND 4.0), which permits the non-commercial replication and distribution of the article with the strict proviso that no changes or edits are made and the original work is properly cited (including links to both the formal publication through the relevant DOI and the license). See: https://creativecommons.org/licenses/by-nc-nd/4.0/.
References
- Torre LA, Bray F, Siegel RL, Ferlay J, Lortet-Tieulent J, Jemal A. Global cancer statistics, 2012. CA Cancer J Clin 2015;65:87-108. [Crossref] [PubMed]
- Chen W, Zheng R, Baade PD, Zhang S, Zeng H, Bray F, Jemal A, Yu XQ, He J. Cancer statistics in China, 2015. CA Cancer J Clin 2016;66:115-32. [Crossref] [PubMed]
- Wang Y, Hou K, Jin Y, Bao B, Tang S, Qi J, Yang Y, Che X, Liu Y, Hu X, Zheng C. Lung adenocarcinoma-specific three-integrin signature contributes to poor outcomes by metastasis and immune escape pathways. J Transl Int Med 2021;9:249-63. [Crossref] [PubMed]
- Travis WD, Brambilla E, Noguchi M, Nicholson AG, Geisinger KR, Yatabe Y, et al. International Association for the Study of Lung Cancer/American Thoracic Society/European Respiratory Society international multidisciplinary classification of lung adenocarcinoma. J Thorac Oncol 2011;6:244-85. [Crossref] [PubMed]
- Nicholson AG, Tsao MS, Beasley MB, Borczuk AC, Brambilla E, Cooper WA, Dacic S, Jain D, Kerr KM, Lantuejoul S, Noguchi M, Papotti M, Rekhtman N, Scagliotti G, van Schil P, Sholl L, Yatabe Y, Yoshida A, Travis WD. The 2021 WHO Classification of Lung Tumors: Impact of Advances Since 2015. J Thorac Oncol 2022;17:362-87. [Crossref] [PubMed]
- Van Schil PE, Asamura H, Rusch VW, Mitsudomi T, Tsuboi M, Brambilla E, Travis WD. Surgical implications of the new IASLC/ATS/ERS adenocarcinoma classification. Eur Respir J 2012;39:478-86. [Crossref] [PubMed]
- Behera M, Owonikoko TK, Gal AA, Steuer CE, Kim S, Pillai RN, Khuri FR, Ramalingam SS, Sica GL. Lung Adenocarcinoma Staging Using the 2011 IASLC/ATS/ERS Classification: A Pooled Analysis of Adenocarcinoma In Situ and Minimally Invasive Adenocarcinoma. Clin Lung Cancer 2016;17:e57-64. [Crossref] [PubMed]
- Takahashi M, Shigematsu Y, Ohta M, Tokumasu H, Matsukura T, Hirai T. Tumor invasiveness as defined by the newly proposed IASLC/ATS/ERS classification has prognostic significance for pathologic stage IA lung adenocarcinoma and can be predicted by radiologic parameters. J Thorac Cardiovasc Surg 2014;147:54-9. [Crossref] [PubMed]
- Mirsadraee S, van Beek EJ. Functional imaging: computed tomography and MRI. Clin Chest Med 2015;36:349-63. x. [Crossref] [PubMed]
- Chen ML, Sun YS. CT perfusion imaging: a valuable and feasible resolution of pulmonary nodules. Advanced Ultrasound in Diagnosis and Therapy 2019;27-34.
- Chen ML, Wei YY, Li XT, Qi LP, Sun YS. Low-dose spectral CT perfusion imaging of lung cancer quantitative analysis in different pathological subtypes. Transl Cancer Res 2021;10:2841-8. [Crossref] [PubMed]
- Ohno Y, Koyama H, Fujisawa Y, Yoshikawa T, Seki S, Sugihara N, Sugimura K. Dynamic contrast-enhanced perfusion area detector CT for non-small cell lung cancer patients: Influence of mathematical models on early prediction capabilities for treatment response and recurrence after chemoradiotherapy. Eur J Radiol 2016;85:176-86. [Crossref] [PubMed]
- Ohno Y, Koyama H, Matsumoto K, Onishi Y, Takenaka D, Fujisawa Y, Yoshikawa T, Konishi M, Maniwa Y, Nishimura Y, Ito T, Sugimura K. Differentiation of malignant and benign pulmonary nodules with quantitative first-pass 320-detector row perfusion CT versus FDG PET/CT. Radiology 2011;258:599-609. [Crossref] [PubMed]
- Bohlsen D, Talakic E, Fritz GA, Quehenberger F, Tillich M, Schoellnast H. First pass dual input volume CT-perfusion of lung lesions: The influence of the CT- value range settings on the perfusion values of benign and malignant entities. Eur J Radiol 2016;85:1109-14. [Crossref] [PubMed]
- Jiang C, Luo Y, Yuan J, You S, Chen Z, Wu M, Wang G, Gong J. CT-based radiomics and machine learning to predict spread through air space in lung adenocarcinoma. Eur Radiol 2020;30:4050-7. [Crossref] [PubMed]
- Zhang Y, Shen Y, Qiang JW, Ye JD, Zhang J, Zhao RY. HRCT features distinguishing pre-invasive from invasive pulmonary adenocarcinomas appearing as ground-glass nodules. Eur Radiol 2016;26:2921-8. [Crossref] [PubMed]
- Yanagawa M, Johkoh T, Noguchi M, Morii E, Shintani Y, Okumura M, Hata A, Fujiwara M, Honda O, Tomiyama N. Radiological prediction of tumor invasiveness of lung adenocarcinoma on thin-section CT. Medicine (Baltimore) 2017;96:e6331. [Crossref] [PubMed]
- Son JY, Lee HY, Lee KS, Kim JH, Han J, Jeong JY, Kwon OJ, Shim YM. Quantitative CT analysis of pulmonary ground-glass opacity nodules for the distinction of invasive adenocarcinoma from pre-invasive or minimally invasive adenocarcinoma. PLoS One 2014;9:e104066. [Crossref] [PubMed]
- Yagi T, Yamazaki M, Ohashi R, Ogawa R, Ishikawa H, Yoshimura N, Tsuchida M, Ajioka Y, Aoyama H. HRCT texture analysis for pure or part-solid ground-glass nodules: distinguishability of adenocarcinoma in situ or minimally invasive adenocarcinoma from invasive adenocarcinoma. Jpn J Radiol 2018;36:113-21. [Crossref] [PubMed]
- Zhang Y, Tang J, Xu J, Cheng J, Wu H. Analysis of pulmonary pure ground-glass nodule in enhanced dual energy CT imaging for predicting invasive adenocarcinoma: comparing with conventional thin-section CT imaging. J Thorac Dis 2017;9:4967-78. [Crossref] [PubMed]
- Son JY, Lee HY, Kim JH, Han J, Jeong JY, Lee KS, Kwon OJ, Shim YM. Quantitative CT analysis of pulmonary ground-glass opacity nodules for distinguishing invasive adenocarcinoma from non-invasive or minimally invasive adenocarcinoma: the added value of using iodine mapping. Eur Radiol 2016;26:43-54. [Crossref] [PubMed]
- Ohno Y, Nishio M, Koyama H, Miura S, Yoshikawa T, Matsumoto S, Sugimura K. Dynamic contrast-enhanced CT and MRI for pulmonary nodule assessment. AJR Am J Roentgenol 2014;202:515-29. [Crossref] [PubMed]
- Maeda R, Ishii G, Ito M, Hishida T, Yoshida J, Nishimura M, Haga H, Nagai K, Ochiai A. Number of circulating endothelial progenitor cells and intratumoral microvessel density in non-small cell lung cancer patients: differences in angiogenic status between adenocarcinoma histologic subtypes. J Thorac Oncol 2012;7:503-11. [Crossref] [PubMed]
- Barletta JA, Yeap BY, Chirieac LR. Prognostic significance of grading in lung adenocarcinoma. Cancer 2010;116:659-69. [Crossref] [PubMed]