Pericoronary adipose tissue attenuation in patients with acute aortic dissection based on coronary computed tomography angiography
Introduction
Acute aortic dissection (AAD) is a catastrophic event and a disease with high morbidity and mortality (1). Over the last 20 years, the disease incidence rate of aortic dissection has remained stable and has been estimated to be 7.7 per 100,000 person-years (2). The exact etiology of this disorder is unknown; however, some factors are thought to be possible causes, including high blood pressure, arteriosclerosis, smoking, aging, and genetics. Regardless of the etiological factor, patients may suffer from angiogenesis and inflammatory reactions (3).
Perivascular adipose tissue (PVAT) encompasses the adipose tissue surrounding the blood vessels, specifically the tissue in close proximity to the vascular wall. This adipose tissue envelops a wide array of systemic blood vessels, encompassing major arteries and veins, organ-specific vasculature, as well as microvessels within skeletal muscle (4). The distinct inflammatory attributes resulting from PVAT dysfunction have been documented across various vascular pathologies, such as atherosclerosis, hypertension, diabetes, aging, and obesity (5-7). Thus, it is important that methodologies be developed to assess the attributes of PVAT and evaluate its inflammatory state. Such endeavors would extend understandings of the initial phases of vascular pathologies and facilitate the formulation of novel therapeutic approaches centered on PVAT.
The density of pericoronary adipose tissue (PCAT) on coronary computed tomography angiography (CCTA) is an imaging biomarker for evaluating coronary arterial inflammation. Empirical evidence indicates that the inflammatory signaling of proinflammatory cytokines from the human arterial wall and their effects on lipolysis and adipogenesis will lead to the phenotypic alterations of PVAT (8). Consequently, these processes lead to modifications in adipocyte size and volume (9). The fat attenuation index (FAI) of CCTA can be used to detect changes in adipocyte dimensions and lipid and water composition within the tissue surrounding the coronary vasculature.
Given the potential presence of PVAT inflammation in patients with AAD, and the ability of PVAT to play both paracrine and endocrine roles in cardiovascular disease through the secretion of various adipocytokines (10), it was hypothesized that pericoronary adipose tissue attenuation (PCATa) would be increased in AAD patients. However, it was uncertain whether PCATa could serve as a sensitive imaging biomarker. Thus, this study sought to compare the features of PCATa in AAD patients and non-AAD patients. We present this article in accordance with the STROBE reporting checklist (available at https://qims.amegroups.com/article/view/10.21037/qims-23-253/rc).
Methods
Patients
Between May 2020 and September 2022, 136 consecutive patients with chest pain were included in the study. In total, 70 AAD patients were diagnosed by aorta computed tomography angiography (CTA) or triple-rule-out computed tomography angiography (TRO-CTA). In total, 66 non-AAD patients underwent TRO-CTA. The AAD patients confirmed by CTA scanning were scanned by CCTA the day after the CTA scan was finished.
To be eligible for inclusion in this study, the patients had to meet the following inclusion criteria: (I) be aged over 30 years; (II) present with acute chest pain; and (III) have a clinical suspicion of AAD. Patients were excluded from the study if they met any of the following exclusion criteria: (I) had contraindications to contrast-enhanced computed tomography (CT); (II) were below the age of 30 years; and/or (III) had acute chest pain that was attributed to alternative causes, such as pulmonary embolism, acute myocardial infarction, or chest infection (Figure 1).
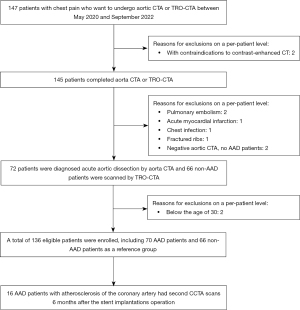
Of the 70 AAD patients, there were 12 Stanford A and 58 Stanford B patients. All the patients had successful stent implantations or ascending aorta replacements. Among the AAD patients with atherosclerosis of the coronary artery, 16 underwent second CCTA scans six months after the stent implantation operation.
This was a prospective, cross-sectional study. The study was conducted in accordance with the Declaration of Helsinki (as revised in 2013) and was approved by the Medical Ethics Committee of Chongqing General Hospital. Informed consent was obtained from all patients.
CTA acquisition
All the patients were scanned on a dual-layer spectral detector CT scanner (IQon, Philips Healthcare, Best, The Netherlands). The automatic bolus tracking method was used in the scans. The region of interest (ROI) was located at the center of the thoracic aorta at the bronchial bifurcation level. The ROI size was 8 mm2. When the predetermined signal attenuation threshold reached 100 Hounsfield units (HUs), the scans were initiated automatically. Contrast media (Visipaque Iodixanol 370; GE Healthcare, Ireland) was injected intravenously through the antecubital vein using an 18-gauge catheter dual-tube high pressure syringe (Ulrich REF XD 2051) with a fixed 4 mL/s flow rate. Next, 30 mL of saline was injected at the same injection rate. Contrast media was used on an individual basis according to body weight. The total amount of contrast media was calculated as follows: total amount of contrast media = patient weight × 0.8 mL/kg.
The scan parameters were as follows: tube voltage: 120 kVp; tube current automatic exposure control: dose right index =13; field of view: 250 mm; tube rotation time: 0.27 s; detector collimation: 64 mm × 0.625 mm; slice thickness: 0.9 mm; increment: 0.45 mm; and matrix: 512×512.
The patients diagnosed with AAD by a cardiac surgeon had CTA scans performed from the arcus aortae to the external iliac artery. The scanning range of the CCTA was from the lower edge of the heart to the tracheal eminence. The scanning range of the TRO-CTA was from the lung apices to the diaphragm. In CCTA and TRO-CTA, electrocardiogram-gated acquisitions were used. Before the CCTA and TRO-CTA examinations, a 25–50 mg of a β-receptor blocker (metoprolol succinate sustained-release tablets, AstraZeneca, Sweden) was orally administered to reduce and stabilize the heart rate of the patients with a heart rate >75 bpm.
PCATa analysis
Cardiovascular radiologists with more than 10 years of experience performed the measurements. The CT measurement of the PCAT was fully automated. In all the patients, PCATa was measured using a dedicated workstation (Coronary Artery Analysis 3.0.0.1, DeepWise version, China). An area of PCAT was defined as adipose tissue located within radial distances equal to the diameter of the coronary artery (8,11). An attenuation measurement of the adipose tissue was taken on all voxels with attenuation values between −190 and −30 HU, and the PCATa value was automatically calculated as the average CT for PCATa. As the PCAT was sampled with multiplanar reconstructions, a fully automated manner was used to track the lumen and the inner and outer vessel wall border in the pre-identified segment of interest. In relation to overall length, the mean CT PCATa values of the left anterior descending (LAD), the left circumflex artery (LCx), and the right coronary angiography (RCA) were recorded in an automated manner.
Statistical analysis
SPSS 26.0 statistical software was used. The measurement data are expressed as the mean ± standard deviation. The categorical variables are expressed as frequencies and percentages. The Shapiro-Wilk test was used to test the normal distribution the continuous data. Levene’s test was used to assess variance homogeneity. If the data were homogeneous and normally distributed, an independent sample t-test was used to compare the groups. The Chi-squared test was used to assess the categorical variables. A P value <0.05 was considered statistically significant. To ascertain the association between the CCTA-derived imaging parameters and AAD, multivariable logistic regression analysis was conducted, with adjustment variables, including age, sex, dysarteriotony, dyslipidaemia, and current smoking status.
Results
Clinical characteristics
From May 2020 to September 2022, 136 patients (42 female, 94 male; mean age: 63.3±11.9 years) suffering from acute chest pain were enrolled in the study and divided into two groups according to the presence of AAD on CCTA. Of these patients 70 patients (20 women, 50 men; mean age, 61.3±12.3 years; range, 34–93 years) had AAD, and 66 patients (22 women, 44 men; mean age, 65.4±11.2 years; range, 42–89 years) did not have AAD. The AAD patients were predominantly male, were smokers and had hyperlipidaemia. The RCAPCATa, LADPCATa and LCxPCATa values were significantly higher in the AAD patients than the non-AAD subjects (−85.1±9.3 vs. −92.9±10.0 HU; −83.2±7.4 vs. −89.9±9.1 HU; −77.5±8.4 vs. −85.6±7.9 HU, all P<0.001) (Table 1).
Table 1
Baseline characteristics | Total (n=136) | Patients with AAD (n=70) | Patients without AAD (n=66) | P value |
---|---|---|---|---|
Age (years) | 63.3±11.9 | 61.3±12.3 | 65.4±11.2 | 0.46 |
Male | 94 (69.1) | 50 (71.4) | 44 (66.7) | 0.55 |
Other risk factors | ||||
Dysarteriotony | 94 (69.1) | 43 (61.4) | 51 (77.3) | 0.04 |
Dyslipidemia | 73 (53.7) | 40 (57.1) | 33 (50.0) | 0.13 |
Current smoker | 40 (29.4) | 15 (21.4) | 25 (37.9) | 0.02 |
CACS | 68 (50.0) | 36 (51.4) | 32 (48.5) | 0.73 |
RCAPCATa (HU) | −88.9±10.4 | −85.1±9.3 | −92.9±10.0 | <0.001 |
LADPCATa (HU) | −86.4±8.9 | −83.2±7.4 | −89.9±9.1 | <0.001 |
LCxPCATa (HU) | −81.5±9.1 | −77.5±8.4 | −85.6±7.9 | <0.001 |
Data are presented as the mean ± standard deviation or number (percentage). AAD, acute aortic dissection; CACS, coronary artery calcium scoring; RCA, right coronary angiography; PCATa, pericoronary adipose tissue attenuation; LAD, left anterior descending; LCx, left circumflex.
Comparison of CT parameters stratified by AAD and non-AAD
Regardless of whether the coronary arteries were normal with or without obstructive stenosis, the RCAPCATa, LADPCATa and LCxPCATa values were significantly higher in the AAD group than the non-ADD group (normal coronary artery: −87.1±8.8 vs. −94.7±9.9 HU, P=0.002; −85.5±7.6 vs. −92.1±8.6 HU, P=0.002; −80.8±8.1 vs. −89.6±7.0 HU, P<0.001, respectively; without obstructive stenosis: −84.5±11.4 vs. −93.3±12.1 HU, P=0.03; −83.4±6.8 vs. −89.6±9.1 HU, P=0.04; −76.9±8.2 vs. −82.7±7.6 HU, P=0.04, respectively, Figure 2; with obstructive stenosis: −83.0±7.8 vs. −89.1±7.3 HU, P=0.01; −80.2±6.8 vs. −86.1±9.3 HU, P=0.03; −73.9±7.6 vs. −80.8±6.2 HU, P=0.004, Figure 3).
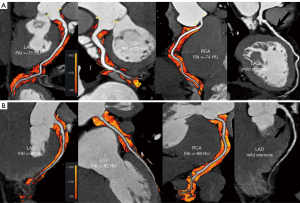
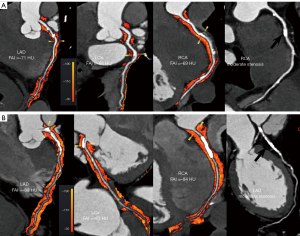
For the obstructive stenosis group, non-calcified plaque, calcified plaque, and mixed plaque were more commonly present in the AAD patients than the non-AAD patients (82.6% vs. 72.2%, P=0.43; 78.3% vs. 66.7%, P=0.41; 82.6% vs. 72.2%, P=0.43), but there were no significant differences between the AAD patients and non-AAD patients in terms of non-calcified plaque, calcified plaque, and mixed plaque. In the non-obstructive stenosis group, the AAD patients showed a significantly higher prevalence of mixed plaque than the non-AAD patients (94.4% vs. 60.0%, P=0.01). However, the incidence of non- and calcified plaque did not differ between the two groups (Table 2).
Table 2
Baseline characteristics | With obstructive stenosis (n=41) | Without obstructive stenosis (n=33) | Normal coronary artery (n=62) | ||||||||
---|---|---|---|---|---|---|---|---|---|---|---|
Patient with AAD (n=23) | Patient without AAD (n=18) | P value | Patient with AAD (n=18) | Patient without AAD (n=15) | P value | Patient with AAD (n=29) | Patient without AAD (n=33) | P value | |||
Age (years) | 71.7±10.7 | 70.8±8.9 | 0.92 | 57.0±10.0 | 64.9±11.1 | 0.06 | 55.9±10.0 | 62.8±11.6 | 0.01 | ||
Male | 15 (65.2) | 11 (61.1) | 0.79 | 12 (66.7) | 13 (86.7) | 0.31 | 23 (79.3) | 20 (60.6) | 0.11 | ||
Dysarteriotony | 14 (60.8) | 15 (93.3) | 0.12 | 13 (72.2) | 11 (73.3) | 0.94 | 16 (55.2) | 25 (75.8) | 0.09 | ||
Dyslipidemia | 14 (60.8) | 10 (55.6) | 0.74 | 11 (61.1) | 9 (60.0) | 0.95 | 18 (62.1) | 14 (42.4) | 0.07 | ||
Current smoking | 6 (26.1) | 6 (33.3) | 0.62 | 3 (16.7) | 7 (46.7) | 0.06 | 6 (20.7) | 12 (36.4) | 0.11 | ||
Non-calcified plaque | 19 (82.6) | 13 (72.2) | 0.43 | 8 (44.4) | 9 (60.0) | 0.38 | |||||
Mixed plaque | 19 (82.6) | 13 (72.2) | 0.43 | 17 (94.4) | 9 (60.0) | 0.01 | |||||
Calcified plaque | 18 (78.3) | 12 (66.7) | 0.41 | 9 (50.0) | 5 (33.3) | 0.35 | |||||
RCAPCATa (HU) | −83.0±7.8 | −89.1±7.3 | 0.01 | −84.5±11.4 | −93.3±12.1 | 0.03 | −87.1±8.8 | −94.7±9.9 | 0.002 | ||
LADPCATa (HU) | −80.2±6.8 | −86.1±9.3 | 0.03 | −83.4±6.8 | −89.6±9.1 | 0.04 | −85.5±7.6 | −92.1±8.6 | 0.002 | ||
LCxPCATa (HU) | −73.9±7.6 | −80.8±6.2 | 0.004 | −76.9±8.2 | −82.7±7.6 | 0.04 | −80.8±8.1 | −89.6±7.0 | <0.001 |
Data are presented as the mean ± standard deviation or number (percentage). CT, computed tomography; RCA, right coronary angiography; LAD, left anterior descending; LCx, left circumflex; PCATa, pericoronary adipose tissue attenuation; AAD, acute aortic dissection.
Comparison of CT parameters stratified by AAD pre- and post-operation
For the AAD patients with atherosclerosis of the coronary artery, the RCAPCATa, LADPCATa and LCxPCATa values were significantly higher preoperatively than postoperatively (−82.9±8.7 vs. −97.6±8.8 HU; −79.8±7.6 vs. −92.8±6.8 HU; −74.6±7.1 vs. −87.7±6.9 HU, all P<0.001) (Table 3, Figure 4).
Table 3
Indicators | Pre-operation (n=16) | Post-operation (n=16) | P value |
---|---|---|---|
RCAPCATa (HU) | −82.9±8.7 | −97.6±8.8 | <0.001 |
LADPCATa (HU) | −79.8±7.6 | −92.8±6.8 | <0.001 |
LCxPCATa (HU) | −74.6±7.1 | −87.7±6.9 | <0.001 |
Data are presented as the mean ± standard deviation. CT, computed tomography; RCA, right coronary angiography; LAD, left anterior descending; LCx, left circumflex; PCATa, pericoronary adipose tissue attenuation; AAD, acute aortic dissection.
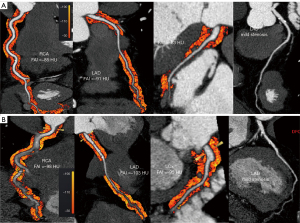
Multivariable analysis of the relationship between CCTA-based imaging parameters and AAD
After adjusting for all the other imaging parameters using multivariate logistic regression, high RCAPCATa and LADPCATa values were associated with AAD regardless of the degree of stenosis [odds ratio (OR) =0.014; 95% confidence interval (CI): 0.001–0.177; P=0.001 and OR =0.010; 95% CI: 0.001–0.189; P=0.002], while the LCxPCATa value was not found to be associated with AAD (OR =0.159; 95% CI: 0.012–2.127; P=0.16) (Table 4).
Table 4
Indicators | Univariate analysis | Multivariate analysis | |||||
---|---|---|---|---|---|---|---|
OR | 95% CI | P value | OR | 95% CI | P value | ||
Age (years) | 0.800 | 0.386–1.658 | 0.54 | 0.574 | 0.153–2.827 | 0.65 | |
Male | 2.579 | 1.275–5.215 | 0.008 | 0.114 | 0.029–0.445 | 0.002 | |
Dysarteriotony | 2.135 | 1.008–4.521 | 0.04 | 4.902 | 1.403–17.130 | 0.01 | |
Dyslipidemia | 0.591 | 0.298–1.171 | 0.13 | 2.176 | 0.754–6.282 | 0.15 | |
Current smoking | 2.383 | 1.120–5.070 | 0.02 | 0.320 | 0.177–0.877 | 0.02 | |
RCAPCATa (HU) | 7.250 | 3.022–17.395 | <0.001 | 0.014 | 0.001–0.177 | 0.001 | |
LADPCATa (HU) | 2.319 | 1.165–4.614 | 0.01 | 0.010 | 0.001–0.189 | 0.002 | |
LCxPCATa (HU) | 4.950 | 2.287–10.717 | <0.001 | 0.159 | 0.012–2.127 | 0.16 |
CCTA, coronary computed tomography angiography; OR, odds ratio; CI, confidence interval; RCA, right coronary angiography; PCATa, pericoronary adipose tissue attenuation; LAD, left anterior descending; LCx, left circumflex.
Discussion
The primary finding of this study was that the RCAPCATa and LADPCATa values were significantly more elevated in AAD patients than non-AAD patients, irrespective of the presence of coronary artery stenosis. Thus, an increase in PCATa could potentially serve as an initial imaging biomarker for identifying AAD patients, regardless of the existence of obstructive stenosis.
PVAT encompasses the adipose tissue surrounding the blood vessels positioned in close proximity to the vascular wall. It envelops a vast majority of the systemic blood vessels, comprising major arteries and veins, as well as vasculature specific to organs and microvessels within skeletal muscle. PVAT is adjacent to the adventitial layer of the vascular wall separating structure or layer in large vessels, as in small vessels. It is considered an additional layer of the vessel wall itself, as it can integrate into the vascular wall. Recent studies have reported that PVAT has some similarity to the endothelium in the modulation of vascular function (12-14). In a healthy state, PVAT secretes vasoprotective adipokines (e.g., adiponectin and omentin-1), which promote vasodilatation, and exert anti-inflammatory, antifibrotic, and antioxidant effects. PVAT directly induces inflammation of the adjacent arteries when it is dysfunctional or excessive (6). Vascular inflammation is a key feature in atherogenesis (15). PVAT inflammation may induce atherosclerosis as per the “out-side-to-inside” theory of vascular inflammation (16). PVAT and atherosclerotic lesions augment each other’s proinflammatory state by paracrine signaling, which results in a vicious cycle.
The PVAT around coronary arteries is part of the epicardial adipose tissue (EAT) compartment. EAT is adipose tissue that is situated entirely within the pericardial sac (17). The adipose tissue located in the mediastinum, outside the pericardial sac, is commonly known as intrathoracic adipose tissue. This includes the adipose tissue surrounding both the descending and ascending aorta. Previous research has shown that the association between EAT volume and coronary artery disease (CAD) is weaker than the association between intrathoracic adipose tissue volume and CAD in patients with cardiovascular disease (18). The Framingham study reported a consistent effect size for both total mediastinal adipose tissue (both intrathoracic and epicardial) and EAT in CAD (19). Additionally, an examination of postmortem samples revealed a correlation between the expression of adipokines and cytokines in PVAT surrounding the aorta and coronary arteries, and the presence of atherosclerosis (20). Consequently, measurements of adipose tissue surrounding the coronary arteries could be used as predictive indicators of atherosclerosis (21,22).
Previous research examined whether the FAI of PVAT method could be used to measure changes in adipocyte lipid content around human coronary arteries (23). The FAI of PVAT not only detects coronary inflammation early but also identifies changes in coronary inflammation. It is important that inflamed coronary arteries be detected in treatment. Patients with high PCATa values but without CAD have an increased cardiovascular risk and require early primary preventative measures. PCATa may also identify inflamed vessels, and patients with inflamed vessels require more intense medical therapy. Previous research has shown that PCATa may be able to trace the reaction of the coronaries to systemic anti-inflammatory therapies, which should be investigated further in future randomized studies (22). However, there are relatively few studies about PCATa of the coronaries to systemic anti-inflammatory effects.
In this study, the PCATa values were higher in atherosclerosis patients with AAD than atherosclerosis patients without AAD. This finding suggests that the mutual promotion of AAD and atherosclerosis leads to an increased state of inflammation in thoracic PVAT and EAT, which may contribute to inflammation in the vessel wall through paracrine signaling (11). Our study also showed that PCATa values are low in patients with AAD concomitant with atherosclerosis after systemic anti-inflammatory therapies. This may be the cause by which PCATa can trace the reaction of the coronaries to systemic anti-inflammatory therapies (23).
High vessel pressure, low shear stress, and a turbulent flow pattern have been shown to be related to acute type A and B aortic dissection (24). In this study, we found that the prevalence of abnormal blood pressure was high, reaching 61.4%. Pathologically, hypertension is related to the activation of the renin-angiotensin-aldosterone system and increased vascular oxidative stress. The PVAT and PVAT/adventitial border are the primary sites of the initial inflammation in hypertension (25-27). PVAT may play a key role in modulating perivascular inflammation in hypertension, and researchers have shown that almost all components of the renin-angiotensin-aldosterone system, except renin, are expressed in PVAT (7,28). During the course of hypertension, there is a notable accumulation of immune cells primarily in the PVAT surrounding major blood vessels, including the aorta and renal arteries (29). There is growing evidence that the involvement of EAT may contribute to the development of hypertension (24,30). Thus, we speculate that the two above-mentioned elements may be able to generate the high PCATa of AAD.
In terms of the etiological factors of AAD, multiple studies have shown that it is likely that a constellation of factors (e.g., athero-arteriolosclerosis, hypertension, bicuspid aortic valve, idiopathic aortic root dilation, and senescence) actively participate in the weakening of the aortic wall (7,31-36). Degenerative lesions of the aortic wall affect muscles and elasticity, which can weaken the aorta; generally speaking, they affect elastic tissue in the young, and smooth muscle tissue in the elderly (3). A clinicopathologic study of 513 patients revealed that the most common pathologic histologic finding of the ascending aorta is medial degeneration in older patients (37). A slight to marked inflammatory reaction has been observed in both degenerative and inflammatory diseases of the thoracic aorta (3). Our study showed that PCATa is also higher in AAD patients with normal coronary arteries. As mentioned above, we know that multiple factors that cause the weakening of the aortic wall are associated with inflammation. Based on our study, PCAT can be considered a marker of systemic perivascular inflammation. All of these factors may account for the high PCATa of AAD patients with normal coronary arteries.
The findings of the present study have a number of clinical implications. First, PCATa is higher in AAD patients, independent of existing atherosclerosis of the coronary artery. RCAPCATa and LADPCATa values were found to be sensitive parameters for capturing perivascular inflammation in AAD patients. It may serve as an imaging biomarker to identify underlying inflammation of the vasculature in AAD patients. In addition, RCAPCATa and LADPCATa values were sensitive parameters for the reaction to systemic anti-inflammatory therapies in AAD patients. It may serve as an imaging biomarker to identify the condition of inflammation reduction after systemic anti-inflammatory therapies in AAD concomitant atherosclerosis patients. Third, RCA and LAD are the preferred arteries for PCATa analysis.
Despite its promising results, the current study had several limitations. First, in this prospective study, the data were collected cross-sectionally, which means that only assumptions about possible etiological relationships can be made. Second, due to the sample size, multicenter and large-sample studies need to be conducted to verify our conclusions. Finally, the study data were obtained in routine medical care. However, laboratory inspection data on markers of dysfunction of fat surrounding coronary arteries, such as plasminogen activator inhibitor-1, were not recorded. The periaortic fat tissue is in contact with the aortic wall that can correspond to the level of periaortic inflammation. Thus, there might be a correlation between PCATa and periaortic fat tissue attenuation. More studies need to be conducted to explain it.
Conclusions
PCATa on CT was increased in patients with AAD regardless of the presence or absence of CAD. This suggests that vascular inflammation is present in AAD independent of CAD. Further research should be conducted to investigate the potential of this imaging biomarker to predict AAD and monitor patient responses to therapies for AAD.
Acknowledgments
We express our gratitude to our technical colleagues for their invaluable assistance in providing data support, as well as to our esteemed cardiovascular surgery colleagues for their invaluable support in conducting the study on patients with AAD.
Funding: This study was supported by
Footnote
Reporting Checklist: The authors have completed the STROBE reporting checklist. Available at https://qims.amegroups.com/article/view/10.21037/qims-23-253/rc
Conflicts of Interest: All authors have completed the ICMJE uniform disclosure form (available at https://qims.amegroups.com/article/view/10.21037/qims-23-253/coif). The authors have no conflicts of interest to declare.
Ethical Statement: The authors are accountable for all aspects of the work in ensuring that questions related to the accuracy or integrity of any part of the work are appropriately investigated and resolved. The study was conducted in accordance with the Declaration of Helsinki (as revised in 2013) and approved by the Medical Ethics Committee of Chongqing General Hospital. Informed consent was obtained from all patients.
Open Access Statement: This is an Open Access article distributed in accordance with the Creative Commons Attribution-NonCommercial-NoDerivs 4.0 International License (CC BY-NC-ND 4.0), which permits the non-commercial replication and distribution of the article with the strict proviso that no changes or edits are made and the original work is properly cited (including links to both the formal publication through the relevant DOI and the license). See: https://creativecommons.org/licenses/by-nc-nd/4.0/.
References
- Miniño AM, Murphy SL, Xu J, Kochanek KD. Deaths: final data for 2008. Natl Vital Stat Rep 2011;59:1-126.
- Nesi G, Anichini C, Tozzini S, Boddi V, Calamai G, Gori F. Pathology of the thoracic aorta: a morphologic review of 338 surgical specimens over a 7-year period. Cardiovasc Pathol 2009;18:134-9. [Crossref] [PubMed]
- Wada H, Sakata N, Tashiro T. Clinicopathological study on penetrating atherosclerotic ulcers and aortic dissection: distinct pattern of development of initial event. Heart Vessels 2016;31:1855-61. [Crossref] [PubMed]
- Wall C, Huang Y, Le EPV, Ćorović A, Uy CP, Gopalan D, et al. Pericoronary and periaortic adipose tissue density are associated with inflammatory disease activity in Takayasu arteritis and atherosclerosis. Eur Heart J Open 2021;1:oeab019. [Crossref] [PubMed]
- Guzik TJ, Mangalat D, Korbut R. Adipocytokines - novel link between inflammation and vascular function? J Physiol Pharmacol 2006;57:505-28.
- Ignacak A, Kasztelnik M, Sliwa T, Korbut RA, Rajda K, Guzik TJ. Prolactin--not only lactotrophin. A "new" view of the "old" hormone. J Physiol Pharmacol 2012;63:435-43.
- Mikolajczyk TP, Nosalski R, Szczepaniak P, Budzyn K, Osmenda G, Skiba D, Sagan A, Wu J, Vinh A, Marvar PJ, Guzik B, Podolec J, Drummond G, Lob HE, Harrison DG, Guzik TJ. Role of chemokine RANTES in the regulation of perivascular inflammation, T-cell accumulation, and vascular dysfunction in hypertension. FASEB J 2016;30:1987-99. [Crossref] [PubMed]
- Antonopoulos AS, Sanna F, Sabharwal N, Thomas S, Oikonomou EK, Herdman L, et al. Detecting human coronary inflammation by imaging perivascular fat. Sci Transl Med 2017;9:eaal2658. [Crossref] [PubMed]
- Antoniades C, Antonopoulos AS, Deanfield J. Imaging residual inflammatory cardiovascular risk. Eur Heart J 2020;41:748-58. [Crossref] [PubMed]
- Akoumianakis I, Tarun A, Antoniades C. Perivascular adipose tissue as a regulator of vascular disease pathogenesis: identifying novel therapeutic targets. Br J Pharmacol 2017;174:3411-24. [Crossref] [PubMed]
- Oikonomou EK, Marwan M, Desai MY, Mancio J, Alashi A, Hutt Centeno E, et al. Non-invasive detection of coronary inflammation using computed tomography and prediction of residual cardiovascular risk (the CRISP CT study): a post-hoc analysis of prospective outcome data. Lancet 2018;392:929-39. [Crossref] [PubMed]
- DeMartino RR, Sen I, Huang Y, Bower TC, Oderich GS, Pochettino A, Greason K, Kalra M, Johnstone J, Shuja F, Harmsen WS, Macedo T, Mandrekar J, Chamberlain AM, Weiss S, Goodney PP, Roger V. Population-Based Assessment of the Incidence of Aortic Dissection, Intramural Hematoma, and Penetrating Ulcer, and Its Associated Mortality From 1995 to 2015. Circ Cardiovasc Qual Outcomes 2018;11:e004689. [Crossref] [PubMed]
- Chen X, Dang Y, Hu H, Ma S, Ma Y, Wang K, Liu T, Lu X, Hou Y. Pericoronary adipose tissue attenuation assessed by dual-layer spectral detector computed tomography is a sensitive imaging marker of high-risk plaques. Quant Imaging Med Surg 2021;11:2093-103. [Crossref] [PubMed]
- Gollasch M. Vasodilator signals from perivascular adipose tissue. Br J Pharmacol 2012;165:633-42. [Crossref] [PubMed]
- Lee RM, Lu C, Su LY, Werstuck G, Gao YJ. Effects of hyperglycemia on the modulation of vascular function by perivascular adipose tissue. J Hypertens 2009;27:118-31. [Crossref] [PubMed]
- Ouwens DM, Sell H, Greulich S, Eckel J. The role of epicardial and perivascular adipose tissue in the pathophysiology of cardiovascular disease. J Cell Mol Med 2010;14:2223-34. [Crossref] [PubMed]
- Lu MT, Park J, Ghemigian K, Mayrhofer T, Puchner SB, Liu T, Fleg JL, Udelson JE, Truong QA, Ferencik M, Hoffmann U. Epicardial and paracardial adipose tissue volume and attenuation - Association with high-risk coronary plaque on computed tomographic angiography in the ROMICAT II trial. Atherosclerosis 2016;251:47-54. [Crossref] [PubMed]
- Britton KA, Fox CS. Perivascular adipose tissue and vascular disease. Clin Lipidol 2011;6:79-91. [Crossref] [PubMed]
- Dey D, Wong ND, Tamarappoo B, Nakazato R, Gransar H, Cheng VY, Ramesh A, Kakadiaris I, Germano G, Slomka PJ, Berman DS. Computer-aided non-contrast CT-based quantification of pericardial and thoracic fat and their associations with coronary calcium and Metabolic Syndrome. Atherosclerosis 2010;209:136-41. [Crossref] [PubMed]
- Rosito GA, Massaro JM, Hoffmann U, Ruberg FL, Mahabadi AA, Vasan RS, O'Donnell CJ, Fox CS. Pericardial fat, visceral abdominal fat, cardiovascular disease risk factors, and vascular calcification in a community-based sample: the Framingham Heart Study. Circulation 2008;117:605-13. [Crossref] [PubMed]
- Spiroglou SG, Kostopoulos CG, Varakis JN, Papadaki HH. Adipokines in periaortic and epicardial adipose tissue: differential expression and relation to atherosclerosis. J Atheroscler Thromb 2010;17:115-30. [Crossref] [PubMed]
- Gorter PM, van Lindert AS, de Vos AM, Meijs MF, van der Graaf Y, Doevendans PA, Prokop M, Visseren FL. Quantification of epicardial and peri-coronary fat using cardiac computed tomography; reproducibility and relation with obesity and metabolic syndrome in patients suspected of coronary artery disease. Atherosclerosis 2008;197:896-903. [Crossref] [PubMed]
- Mahabadi AA, Reinsch N, Lehmann N, Altenbernd J, Kälsch H, Seibel RM, Erbel R, Möhlenkamp S. Association of pericoronary fat volume with atherosclerotic plaque burden in the underlying coronary artery: a segment analysis. Atherosclerosis 2010;211:195-9. [Crossref] [PubMed]
- Nosalski R, Guzik TJ. Perivascular adipose tissue inflammation in vascular disease. Br J Pharmacol 2017;174:3496-513. [Crossref] [PubMed]
- Berdajs D, Mosbahi S, Ferrari E, Charbonnier D, von Segesser LK. Aortic Valve Pathology as a Predictive Factor for Acute Aortic Dissection. Ann Thorac Surg 2017;104:1340-8. [Crossref] [PubMed]
- Hansson GK, Hermansson A. The immune system in atherosclerosis. Nat Immunol 2011;12:204-12. [Crossref] [PubMed]
- Kirabo A, Fontana V, de Faria AP, Loperena R, Galindo CL, Wu J, et al. DC isoketal-modified proteins activate T cells and promote hypertension. J Clin Invest 2014;124:4642-56. [Crossref] [PubMed]
- Gálvez-Prieto B, Bolbrinker J, Stucchi P, de Las Heras AI, Merino B, Arribas S, Ruiz-Gayo M, Huber M, Wehland M, Kreutz R, Fernandez-Alfonso MS. Comparative expression analysis of the renin-angiotensin system components between white and brown perivascular adipose tissue. J Endocrinol 2008;197:55-64. [Crossref] [PubMed]
- Nguyen Dinh Cat A, Touyz RM. A new look at the renin-angiotensin system--focusing on the vascular system. Peptides 2011;32:2141-50. [Crossref] [PubMed]
- Guzik TJ, Hoch NE, Brown KA, McCann LA, Rahman A, Dikalov S, Goronzy J, Weyand C, Harrison DG. Role of the T cell in the genesis of angiotensin II induced hypertension and vascular dysfunction. J Exp Med 2007;204:2449-60. [Crossref] [PubMed]
- Roubíček T, Dolinková M, Bláha J, Haluzíková D, Bošanská L, Mráz M, Kremen J, Haluzík M. Increased angiotensinogen production in epicardial adipose tissue during cardiac surgery: possible role in a postoperative insulin resistance. Physiol Res 2008;57:911-7. [Crossref] [PubMed]
- Teijeira-Fernandez E, Eiras S, Grigorian-Shamagian L, Fernandez A, Adrio B, Gonzalez-Juanatey JR. Epicardial adipose tissue expression of adiponectin is lower in patients with hypertension. J Hum Hypertens 2008;22:856-63. [Crossref] [PubMed]
- Januzzi JL, Isselbacher EM, Fattori R, Cooper JV, Smith DE, Fang J, Eagle KA, Mehta RH, Nienaber CA, Pape LA. Characterizing the young patient with aortic dissection: results from the International Registry of Aortic Dissection (IRAD). J Am Coll Cardiol 2004;43:665-9. [Crossref] [PubMed]
- Homme JL, Aubry MC, Edwards WD, Bagniewsski SM, Pankratz VS, Kral CA, Tazelaar HD. Surgical pathology of the ascending aorta: a clinicopathologic study of 513 cases. Am J Surg Pathol 2006;30:1159-1168. [Crossref] [PubMed]
- Trachet B, Aslanidou L, Piersigilli A, Fraga-Silva RA, Sordet-Dessimoz J, Villanueva-Perez P, Stampanoni MFM, Stergiopulos N, Segers P. Angiotensin II infusion into ApoE-/- mice: a model for aortic dissection rather than abdominal aortic aneurysm? Cardiovasc Res 2017;113:1230-42. [Crossref] [PubMed]
- Zhang Y, Luo F, Fan P, Meng X, Yang K, Zhou X. Is primary aldosteronism a potential risk factor for aortic dissection? A case report and literature review. BMC Endocr Disord 2020;20:115. [Crossref] [PubMed]
- Virmani R, Avolio AP, Mergner WJ, Robinowitz M, Herderick EE, Cornhill JF, Guo SY, Liu TH, Ou DY, O'Rourke M. Effect of aging on aortic morphology in populations with high and low prevalence of hypertension and atherosclerosis. Comparison between occidental and Chinese communities. Am J Pathol 1991;139:1119-29.