Aberrant brain functional hubs and causal effective connectivity in menstrually-related and non-menstrually-related migraine without aura
Introduction
Migraine is a primary neurologic disorder featured with complex manifestations beyond just headache, and is often accompanied by vomiting, nausea, tiredness, photophobia, and phonophobia (1). As a particular subtype of migraine, menstrual migraine without aura (MRM) is accompanied by additional migraine attack corresponding to the menstrual cycle (2). Compared with non-menstrual migraine without aura (NMM), MRM has typically been treated with higher doses of acute medication, is less responsive to acute therapy, and is associated with greater disability (3,4). In light of these differences, there are likely neuromechanism differences present in MRM patients compared to NMM patients.
Menstruation is arguably the most common migraine trigger of all, having been noted in up to 70% of female migraineurs (5). Hormonal factors exert a tremendous impact on migraine. As characteristic of migraine in women, neuroendocrine vulnerability may facilitate initiation of migraine attack (6). Abnormal physiological fluctuations of sex hormones (i.e., estradiol and progesterone) in female migraineurs can influence the central nervous system (CNS) on multiple levels, which may be the physiological basis of MRM (7). A drop in estrogen may lead to increased susceptibility to prostaglandins (PGs). Neurogenic inflammation caused by increased sensitivity to PGs can lead to increased release of calcitonin gene-related peptide in the trigeminovascular system, which may increase their susceptibility to migraine during menstruation (8). Migraine is a multifaceted CNS disorder. An increasing number of neuroimaging studies indicate that MRM is not only related to abnormality in pain processing networks but also other regions of the CNS (9,10). A better understanding of the disorder of the brain functional network may provide novel insights into the neural mechanism of MRM.
As a core node, the insula is closely related to a wide range of cortical and subcortical regions and participates in coordination between various brain functions such as sensorimotor and pain perception processing, orchestrating interceptions, autonomic control, emotional awareness, attention, or salience. Besides, the insula has the capability to choose the most critical information from multiple internal and external stimuli (11). It is also known to be involved in specific behaviors related to disease conditions such as migraine. As a node of the salience network, the insula acts as a switcher between salience, executive control, and default mode network (DMN), which may respond to fluctuating levels of estrogen (12,13). In a longitudinal menstrual cycle study, higher hormone levels during the mid-luteal phase resulted in increased within-salience network connectivity, which reflected enhanced inter-hemispheric connectivity between the insulae (14). In girls with central precocious puberty, decreased gray matter volume of the left insula was associated with increased follicle-stimulating hormone levels. Besides, increased resting state functional connectivity within bilateral insular cortices was also related to basal and peak estrogen levels (15). Similar to previous functional magnetic resonance imaging (fMRI) findings in healthy young females (16), these findings suggest that brain regions involved in cognition and emotion processing may be partly modulated by hormone levels. However, how the insula and other cerebral regions in the whole brain interact with each other in MRM patients needs further exploration.
Voxel-wise degree centrality (DC) may provide an objective and comprehensive assessment of brain functional connectivity patterns in certain diseases. On the basis of graph theory, DC calculates the time series correlation between each node and other voxels in the whole brain without selecting nodes or networks of interest in advance (17). Abnormal DC values reflect that relevant cerebral regions are located in the hub of the whole brain information communication network. Granger causality analysis (GCA) can be used to explore the top-down effective connectivity between the selected node and other brain regions, which reflects causality between cerebral regions (18). Our previous study also demonstrated disruption of directional functional connectivity in the amygdala in migraine patients without aura (19). However, few studies have explored the aberrant brain functional hubs between MRM and NMM patients combining DC and GCA.
Disrupted whole brain networks and directional functional connectivity might be the neuromechanism of MRM patients, and the insula may play an important role. To address this issue, we aimed to use DC to explore the hub of the whole brain information communication network between MRM and NMM patients, and then apply GCA to explore the top-down effective connectivity. We hypothesized that alterations of directional functional connectivity in the hub node explored by DC analysis can reflect the physiopathologic mechanism of MRM. We present this article in accordance with the STROBE reporting checklist (available at https://qims.amegroups.com/article/view/10.21037/qims-23-838/rc).
Methods
Participants
A total of 46 MRM patients and 43 age-, gender-, and years of education-matched NMM patients were consecutively recruited in the study between May 2018 and June 2022; all participants were female and right-handed. A case in the MRM group was excluded from further analysis because of pregnancy, and three cases in the NMM group was excluded because of drug abuse and cerebral vascular disease. Thus, 45 MRM patients and 40 age-, gender-, and years of education-matched NMM patients were enrolled in our study (Figure 1). In this retrospective and cross-sectional study, all participants were recruited from the Neurology and Pain Outpatient Department of Nanjing First Hospital. As recommended by the International Classification of Headache Disorders (ICHD), in the third version of ICHD (ICHD-3beta) (2), MRM was defined an occurrence of migraine without aura (MwoA) in the perimenstrual period (2 days before menstruation to 3 days after the menstrual period) in at least 2 out of 3 menstrual cycles, with additional migraines occurring outside the perimenstrual period was considered the MRM group. Female patients with MwoA that occurred only outside of the menstrual cycle were included in the NMM group. All MwoA female patients had had a regular menstrual cycle for 6 months or more and were 18–50 years old. The exclusion criteria were pregnant, breast-feeding, or probable medication overuse-related headache. All participants completed the Self-rating Anxiety Scale (SAS), Self-rating Depression Scale (SDS), Montreal Cognitive Assessment (MoCA), Headache Impact Test-6 (HIT-6), and Migraine Disability Assessment (MIDAS). To prevent any possible influence from migraine attack, alcohol, smoke, cerebrovascular disease, and medication on fMRI signal fluctuation, patients were migraine free, had not taken medicine, alcohol, or smoked for at least 3 days before MRI scan and were followed up 3 days after scanning to ensure that they did not have any migraine attacks during this period. MRI was performed outside the menstrual period, approximately during days 12–16 of the menstrual cycle. This study was conducted in accordance with the Declaration of Helsinki (as revised in 2013). The study was approved by the Institutional Review Board of Nanjing Medical University and informed consent was provided by all individual participants before MRI scan.
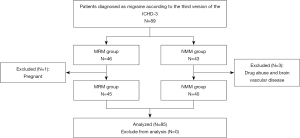
Imaging methods
MR examinations were conducted at 3.0 Tesla MRI scanner (Ingenia, Philips Medical Systems, Best, Netherlands). All cases were scanned with almost the same protocol including high-resolution 3-dimensional fast-echo T1-weighted MR images [repetition time (TR) =8.1 ms; echo time (TE) =3.7 ms, resolution 1×1×1, scan time about 4:20 min], functional images (echo-planar imaging, resolution 2.75×2.75×4 mm3, TR/TE =2,000 ms/30 ms, 230 volumes, awake and eyes closed, scan time about 8:08 min), and fluid-attenuated inversion recovery imaging (FLAIR; TR =7,000 ms; TE =120 ms; slices =18; slice thickness =6 mm). Based on visual inspections on structural MR images, patients with brain tumor, brain vascular disease, hydrocephalus, or obvious white matter hyperintensity were excluded from this study.
Image preprocessing
The preprocessing of the functional data was executed by the GRETNA package using MATLAB (MathWorks, Natick, MA, USA). The steps of preprocessing included removing the first 10 time points, slice timing correction, head motion correction, normalization of the native space to Montreal Neurological Institute (MNI) space with a final size of 3×3×3 mm3, regression of signals from white matter, cerebrospinal fluid, and 24 head movement parameters, linear trend removal in time series from each voxel, and bandpass filtering (0.01–0.1 Hz).
Voxel-wise DC analysis
We used the REST package to perform voxel-wise DC calculations by taking each voxel as a node. The specific steps for obtaining DC maps had been detailed in the previous study (20). In short, it includes the following steps: the time course of each voxel was extracted first. Subsequently, we calculated each voxel’s Pearson correlation coefficient (r) of the time course between each voxel and all the other voxels. Finally, the threshold of the obtained Pearson correlation coefficient matrix was set to r>0.25. In the present research, we used binary DC values. To improve the normalization of the data, we transformed the correlation coefficients to z-scores using Fisher’s r-to-z transformation.
Seed-based GCA analysis
This step used REST package to perform GCA analysis. Firstly, the brain regions where there were clusters with increased or decreased DC values displayed in MRM compared to NMM were defined as regions of interest. Then, GCA was used to describe directional connections: between the reference time series of the seed region and the time series of each voxel within the whole brain. We performed two analyses for the selected seed: seed-to-other brain voxel analysis (X to Y effect) and other brain voxel-to-seed analysis (Y to X effect). In our study, the time series of the selected seed were defined as seed time series x, and time series y denoted the time series of all voxels in the brain. A positive coefficient from X to Y (Fx→y) indicated the linear direct influence of x on y. Similarly, a negative coefficient from X to Y (Fy→x) suggested the linear direct influence of y on x. A bivariate coefficient GCA was performed to assess the causal effect between the selected seed and other voxels of the whole brain so that two Granger causality maps were generated based on the influence measures for each case. The residual-based F was normalized (F’) and standardized to Z score for each voxel (Zx→y and Zy→x, subtracting the global mean F’values, divided by standard deviation). For the group analysis on the effective connectivity of the selected seed, mean values of Zx→y and Zy→x maps were computed for each group. All four Granger causality maps were acquired, with two for each direction and two for each group (the right insula with Zx→y and Zy→x for both the MRM and NMM groups).
DC and GCA
Between-group comparisons of DC and GCA maps were performed in the SPM12 software (Wellcome Centre for Human Neuroimaging, London, UK) using general linear model (GLM) analysis. The 2-samples t-test was conducted to analyze the differences of DC and GCA values between MRM and NMM groups with age, gender, and years of education as covariates. For multiple comparison correction, Gaussian random field (GRF) correction with voxel level P<0.01 (Z>2.58) and cluster level P<0.05 was used. The threshold of cluster size was set at 100 voxels. For DC analysis, a mask was created by combining the significant clusters in both groups, which were obtained from 1-sample t-test results (P<0.05, GRF corrected). For GCA analysis, positive clusters based on REST-plus were generated as binary mask, and the connective strengths of the significant regions were extracted based on the z-maps.
Statistical analysis
Demographic data and clinical assessment data between MRM and NMM groups were analyzed using 2-sample t-test for means, and the chi-square test was adopted to examine differences between genders (P<0.05 was considered to be significant). To investigate the relationship between fMRI data and clinical characteristic of MRM groups, regions showing significant differences between groups were extracted. Then, the mean z-values of aberrant DC and GCA region masks were calculated within every case. The software SPSS software 17.0 (IBM Corp., Chicago, IL, USA) were performed to calculate Pearson’s correlation coefficients. P<0.05 was considered statistically significant, corrected for age, and years of education. Bonferroni correction was used for multiple comparisons in the correlation analyses.
Results
Demographic and clinical characteristics
As summarized in Table 1, neither group showed any significant differences for age (P=0.325), education duration (P=0.173), MoCA score (P=0.072), SDS score (P=0.242), HIT-6 score (P=0.081), MIDAS score (P=0.074), frequency (P=0.071), or duration (P=0.069). Nevertheless, the average SAS score of the MRM group was significantly higher than that of the NMM group [P=0.032, 95% confidence interval (CI): 0.363–7.761] (Table 1).
Table 1
Characteristics | MRM (n=45) | NMM (n=40) | P value |
---|---|---|---|
Age (years) | 34.46±9.98 | 36.63±7.22 | 0.325 |
Education (years) | 14.09±2.7 | 15±2.62 | 0.173 |
SAS | 39.83±8.34 | 35.77±6.2 | 0.032* |
SDS | 41.9±8.02 | 39.51±8.18 | 0.242 |
MoCA | 26.6±1.68 | 27.4±1.81 | 0.072 |
HIT-6 | 61.17±6.84 | 62.35±5.93 | 0.081 |
MIDAS | 12±6.562 | 11.28±6.15 | 0.074 |
Frequency (days per month) | 4.8±3.1 | 4.2±2.9 | 0.071 |
Duration (years) | 11.5±8.2 | 12.1±7.5 | 0.069 |
Data are represented as the mean ± standard deviation. Unless otherwise indicated, P-values were calculated with 2-tailed t-tests. *, P<0.05 by the 2-sample t-test. MRM, menstrual migraine without aura; NMM, non-menstrual migraine without aura; SAS, Self-rating Anxiety Scale; SDS, Self-rating Depression Scale; MoCA, Montreal Cognitive Assessment; HIT-6, Headache Impact Test-6; MIDAS, Migraine Disability Assessment.
DC and GCA
Compared with the NMM group, significantly decreased DC of the right insula (T=−4.253) was found in the MRM group (Table 2 and Figure 2). The MRM group demonstrated strongly enhanced effective connectivity from the right insula to the ipsilateral middle temporal gyrus (T=4.138) and contralateral superior temporal gyrus (T=3.523). Furthermore, the MRM group showed obviously decreased effective connectivity from several brain regions to the right insula, which included the right inferior occipital gyrus (T=−4.498), left middle frontal gyrus (T=−4.879), right precuneus (T=−4.644), and left inferior parietal gyrus (T=−4.113) (Table 2, Figures 3-5). All the resultant T-maps were corrected using GRF with voxel P<0.01 and cluster P<0.05.
Table 2
Brain region | Peak MNI coordinates | Voxel size | Peak T score | ||
---|---|---|---|---|---|
X | Y | Z | |||
Lower DC in MRM vs. NMM | |||||
Right insula | 42 | 9 | −12 | 108 | −4.253 |
Directional FC from right insula in MRM vs. NMM | |||||
L_Sup_Temporal | −60 | −66 | 15 | 200 | 3.523 |
R_Mid_Temporal | 60 | 0 | −24 | 180 | 4.138 |
Directional FC to right insula in MRM versus NMM | |||||
R_Inf_Occipital | 48 | −81 | −12 | 170 | −4.498 |
L_Mid_Frontal | −27 | 60 | 6 | 195 | −4.879 |
R_Precuneus | 9 | −66 | 21 | 200 | −4.644 |
L_Inf_Parietal | −54 | −63 | 39 | 125 | −4.113 |
Voxel P<0.01 and cluster P<0.05, Gaussian random field corrected). DC, degree centrality; FC, functional connectivity; MRM, menstrual migraine without aura; NMM, non-menstrual migraine without aura; MNI, Montreal Neurological Institute; L_Sup_Temporal, left superior temporal gyrus; R_Mid_Temporal, right middle temporal gyrus; R_Inf_Occipital, right inferior occipital gyrus; L_Mid_Frontal, left middle frontal gyrus; R_Precuneus, right precuneus; L_Inf_Parietal, left inferior parietal gyrus.
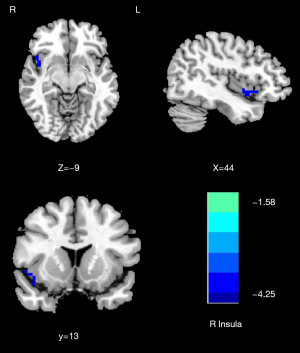
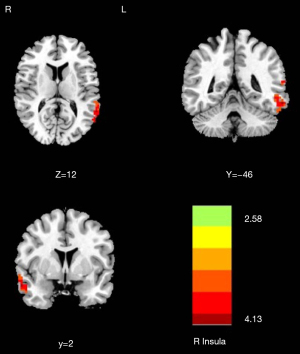
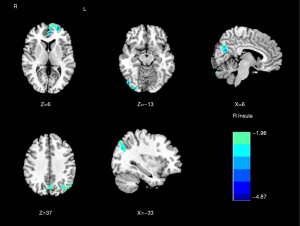
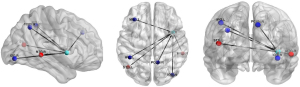
Correlation analysis
As exhibited in Figure 6, disease duration was negatively correlated with the mean value of DC in the right insula in patients with MRM (r=−0.428, P=0.01). No other significant linear correlations were observed.
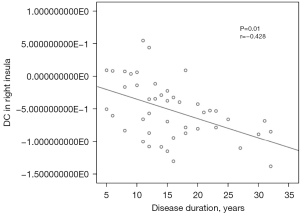
Discussion
This study was conducted to explore effective functional connectivity differences between MRM and NMM patients. The specificity of the study was combining DC and GCA approaches. Our main finding showed a reduction in the network DC of the right insula in MRM patients, which was significantly negatively associated with disease duration of migraine. Furthermore, our findings demonstrated that patients with MRM showed disrupted causal effective connectivity of the right insula in several cerebral regions including diverse functional areas.
Our study indicated that DC of the right insula was significantly decreased in MRM patients compared with the NMM group. The insula is located within the lateral sulcus and acts as a “cortical hub” in integrating multiple brain functions including sensorimotor and pain perception processing, autonomic control, and emotional awareness. It is also believed to play a crucial role in migraine (21). Previous studies have demonstrated that migraineurs have abnormal functional connectivity between the insula and other cerebral regions involved in pain processing such as the thalamus, periaqueductal gray, somatosensory cortex, or cognitive function regions such as the DMN (22-25). In addition to functional abnormalities, structural imaging research also demonstrated subtle gray matter changes of the right insula in MRM patients compared to healthy controls (26). As a particular subtype of MwoA, MRM tends to be more painful, of longer duration, less responsive to acute therapy, and more prone to recurrence than NMM. Our result of higher SAS score of the MRM group than that of the NMM group also reflects that the MRM patients exhibit more anxiety than the NMM patients. During menstruation, fluctuations in ovarian hormone levels have a significant impact on emotions and are the main cause of anxiety. Previous findings have indicated that the insula plays a crucial role in subjective evaluation of visceral consciousness and interoceptive stimulus, perception of internal bodily states, and affective experiences (27-29). Patients with MRM are prone to social and emotional adjustment problems. The fluctuations in ovarian hormone levels, abnormality of emotional perception and migraine attack experienced by MRM patients might partially be associated with decreased DC of the right insula in our study. The frequency of migraine attacks in MRM patients is higher than that in NMM patients, which can occur both inside and outside of the perimenstrual period. The altered DC of the insula in our study may explain this difference. Zhang et al. also found that the placebo effect was achieved through the insula’s regulation of the brain’s sensitivity to pain in MRM patients (30). Although other studies may show different outcomes due to diversity of analytical methods, the aberrant functional activity of insula has always been found in migraineurs, which suggested that the insula acts as “hub of activity” in migraineurs and is worthy for further research to explore the neuromechanism of MRM.
Our research detected enhanced effective connectivity from the right insula to the ipsilateral middle temporal gyrus and contralateral superior temporal gyrus in the MRM group. The temporal lobe is an important component for auditory perception and emotion regulation in the human brain (31,32). Additionally, the temporal lobe is involved in the descending pain modulation pathway (33), which may play a critical role in the occurrence and progression of migraine. In support of this view, research has demonstrated the significance of the superior temporal gyrus in pain anticipation and encoding (34). Altered gray matter volumes in the temporal lobe have also been reported in vestibular migraine patients, indicating that this brain region is majorly involved in pain regulation (35). Li et al. discovered that regional efficiency in the bilateral superior temporal gyrus was decreased in MRM patients compared to healthy controls, which was attributed to the decreased network integration (36). Anatomically, the insula and the mesial temporal lobe are directly connected through amygdaloinsular fasciculus in the extreme capsule, forming the temporoinsular projection system (37). Cerebral network reorganization of MRM is more complex than that of NMM as migraine attacks inside and outside the perimenstrual period would also contribute to the subtle changes in the cortical organization of brain. Our result of abnormal connectivity between the insula and temporal lobe indicates that reorganization of the temporoinsular projection system may be consequence of repeated pain stimulation. The temporal lobe may be an important component of the neural regulation for high-frequency attacks in MRM patients.
The middle frontal gyrus, as one of components in the executive control network, is responsible for higher cognitive control functions and modulation of pain (38,39). A recent study observed decreased effective connectivity from the left middle frontal gyrus to the right insula in the MRM group. Connection between the insula and the frontal lobe is implicated in cognitive and pain processing (40). In a previous study, MRM patients exhibited lower amplitude of low‑frequency fluctuations values in dorsolateral and medial prefrontal cortex than pure menstrual migraine (10). The frontal cortex is one of the important regulatory centers in the brain of migraineurs. It is also a predisposing element in the pathological mechanisms of migraine patients (41). Abnormal activity in the middle frontal gyrus also demonstrated a negative correlation with the pain intensity in migraineurs (42). Our finding of a disrupted pathway from the frontal lobe to the insula might be associated with emotional management and inhibitory control of pain perception disorder in MRM patients.
The present study also detected decreased effective connectivity from the right precuneus, right inferior occipital gyrus, and left inferior parietal gyrus to the right insula in the MRM group. Precuneus and inferior parietal gyrus are core components of the DMN (43). The DMN acts as an important affective component in pain regulation, especially in the encoding of painful processes (44). Additionally, Zou et al. (45) discovered that after acupuncture treatment for chronic migraine, alteration of DMN functional activity was associated with relief of pain intensity and migraine frequency. A previous study investigated whether gender differences affect the thickness of cerebral cortex in migraineurs. They found that cerebral cortical thickness in the precuneus was increased in female migraineurs compared to male migraineurs and healthy controls (46). These results revealed sex-specific morphology changes in migraineurs. The inferior occipital gyrus participates in visual perception and processing. The MRM group showed an overall increase in occipital activation with photic activation, particularly during the mid-follicular phase of the menstrual cycle (47). Combining our findings, we speculate that the abnormal regional functional activity of the occipital lobe may be related to photophobia in MRM patients. The abnormal functional connectivity between these regions and insula might be associated with self-adaptation of pain sensation to the recurrent migraine attack in the MRM group.
There are several potential limitations in the present study. First, due to the relatively small sample size, our results are not comprehensive. Second, given the important role of hormones in MRM, the relationship between hormonal levels and neuroimaging needs further exploration in future research. Lastly, the lack of a healthy control group prevents us from providing a more in-depth discussion of the differences observed.
Conclusions
To summarize, our study discovered that the disruption in the brain functional network, especially the insula, plays a crucial role in executive dysfunction of MRM. In addition, the disrupted directional functional connectivity of the right insula might be associated with functional impairments in pain processing and emotion regulation, which may play an important role in the neuromechanism of MRM.
Acknowledgments
The authors would like to thank all study participants.
Funding: This research was supported by
Footnote
Reporting Checklist: The authors have completed the STROBE reporting checklist. Available at https://qims.amegroups.com/article/view/10.21037/qims-23-838/rc
Conflicts of Interest: All authors have completed the ICMJE uniform disclosure form (available at https://qims.amegroups.com/article/view/10.21037/qims-23-838/coif). The authors have no conflicts of interest to declare.
Ethical Statement: The authors are accountable for all aspects of the work in ensuring that questions related to the accuracy or integrity of any part of the work are appropriately investigated and resolved. This study was conducted in accordance with the Declaration of Helsinki (as revised in 2013). The study was approved by the Institutional Review Board of Nanjing Medical University and informed consent was provided by all individual participants before undergoing MRI.
Open Access Statement: This is an Open Access article distributed in accordance with the Creative Commons Attribution-NonCommercial-NoDerivs 4.0 International License (CC BY-NC-ND 4.0), which permits the non-commercial replication and distribution of the article with the strict proviso that no changes or edits are made and the original work is properly cited (including links to both the formal publication through the relevant DOI and the license). See: https://creativecommons.org/licenses/by-nc-nd/4.0/.
References
- Migraine Ashina M. N Engl J Med 2020;383:1866-76. [Crossref] [PubMed]
- Olesen J, Dodick DW, Ducros A, Evers S, Terwindt GM. The International Classification of Headache Disorders, 3rd edition (ICHD-3). Cephalalgia 2018;38. doi:
10.1177/0333102417738202 . - Calhoun AH. Understanding Menstrual Migraine. Headache 2018;58:626-30. [Crossref] [PubMed]
- Silberstein S, Patel S. Menstrual migraine: an updated review on hormonal causes, prophylaxis and treatment. Expert Opin Pharmacother 2014;15:2063-70. [Crossref] [PubMed]
- MacGregor A. Migraine associated with menstruation. Funct Neurol 2000;15:143-53.
- Pavlović JM, Allshouse AA, Santoro NF, Crawford SL, Thurston RC, Neal-Perry GS, Lipton RB, Derby CA. Sex hormones in women with and without migraine: Evidence of migraine-specific hormone profiles. Neurology 2016;87:49-56. [Crossref] [PubMed]
- McEwen BS, Milner TA. Understanding the broad influence of sex hormones and sex differences in the brain. J Neurosci Res 2017;95:24-39. [Crossref] [PubMed]
- Cupini LM, Corbelli I, Sarchelli P. Menstrual migraine: what it is and does it matter? J Neurol 2021;268:2355-63. [Crossref] [PubMed]
- Zhang Y, Xu T, Wang Z, Li D, Du J, Wen Y, Zhao Y, Liao H, Liang F, Zhao L. Differences in topological properties of functional brain networks between menstrually-related and non-menstrual migraine without aura. Brain Imaging Behav 2021;15:1450-9. [Crossref] [PubMed]
- Xu T, Zhang Y, Wang C, Liao H, Zhou S, Li D, Huang S, Shi Y, Wang Z, Chen J, Liang FR, Zhao L. Brain structural and functional differences between pure menstrual migraine and menstrually-related migraine. Sci Rep 2020;10:16454. [Crossref] [PubMed]
- Uddin LQ, Nomi JS, Hébert-Seropian B, Ghaziri J, Boucher O. Structure and Function of the Human Insula. J Clin Neurophysiol 2017;34:300-6. [Crossref] [PubMed]
- Zhou Y, Friston KJ, Zeidman P, Chen J, Li S, Razi A. The Hierarchical Organization of the Default, Dorsal Attention and Salience Networks in Adolescents and Young Adults. Cereb Cortex 2018;28:726-37. [Crossref] [PubMed]
- Sridharan D, Levitin DJ, Menon V. A critical role for the right fronto-insular cortex in switching between central-executive and default-mode networks. Proc Natl Acad Sci U S A 2008;105:12569-74. [Crossref] [PubMed]
- Hidalgo-Lopez E, Zeidman P, Harris T, Razi A, Pletzer B. Spectral dynamic causal modelling in healthy women reveals brain connectivity changes along the menstrual cycle. Commun Biol 2021;4:954. [Crossref] [PubMed]
- Chen T, Lu Y, Wang Y, Guo A, Xie X, Fu Y, Shen B, Lin W, Yang D, Zhou L, Liu X, Liu P, Yan Z. Altered Brain Structure and Functional Connectivity Associated with Pubertal Hormones in Girls with Precocious Puberty. Neural Plast 2019;2019:1465632. [Crossref] [PubMed]
- Zuo XN, Xing XX. Test-retest reliabilities of resting-state FMRI measurements in human brain functional connectomics: a systems neuroscience perspective. Neurosci Biobehav Rev 2014;45:100-18. [Crossref] [PubMed]
- Syan SK, Minuzzi L, Costescu D, Smith M, Allega OR, Coote M, Hall GBC, Frey BN. Influence of endogenous estradiol, progesterone, allopregnanolone, and dehydroepiandrosterone sulfate on brain resting state functional connectivity across the menstrual cycle. Fertil Steril 2017;107:1246-55.
- Friston K, Moran R, Seth AK. Analysing connectivity with Granger causality and dynamic causal modelling. Curr Opin Neurobiol 2013;23:172-8. [Crossref] [PubMed]
- Huang X, Zhang D, Wang P, Mao C, Miao Z, Liu C, Xu C, Yin X, Wu X. Altered amygdala effective connectivity in migraine without aura: evidence from resting-state fMRI with Granger causality analysis. J Headache Pain 2021;22:25. [Crossref] [PubMed]
- Xing C, Chen YC, Tong Z, Xu W, Xu JJ, Yin X, Wu Y, Cai Y. Aberrant brain functional hubs and causal connectivity in presbycusis. Brain Imaging Behav 2021;15:453-63. [Crossref] [PubMed]
- Borsook D, Veggeberg R, Erpelding N, Borra R, Linnman C, Burstein R, Becerra L. The Insula: A "Hub of Activity" in Migraine. Neuroscientist 2016;22:632-52. [Crossref] [PubMed]
- Amin FM, Hougaard A, Magon S, Sprenger T, Wolfram F, Rostrup E, Ashina M. Altered thalamic connectivity during spontaneous attacks of migraine without aura: A resting-state fMRI study. Cephalalgia 2018;38:1237-44. [Crossref] [PubMed]
- Schwedt TJ, Larson-Prior L, Coalson RS, Nolan T, Mar S, Ances BM, Benzinger T, Schlaggar BL. Allodynia and descending pain modulation in migraine: a resting state functional connectivity analysis. Pain Med 2014;15:154-65. [Crossref] [PubMed]
- Silvestro M, Tessitore A, Di Nardo F, Scotto di Clemente F, Trojsi F, Cirillo M, Esposito F, Tedeschi G, Russo A. Functional connectivity changes in complex migraine aura: beyond the visual network. Eur J Neurol 2022;29:295-304. [Crossref] [PubMed]
- Yu D, Yuan K, Luo L, Zhai J, Bi Y, Xue T, Ren X, Zhang M, Ren G, Lu X. Abnormal functional integration across core brain networks in migraine without aura. Mol Pain 2017;13:1744806917737461. [Crossref] [PubMed]
- Schmidt-Wilcke T, Gänssbauer S, Neuner T, Bogdahn U, May A. Subtle grey matter changes between migraine patients and healthy controls. Cephalalgia 2008;28:1-4. [Crossref] [PubMed]
- Baumgartner T, Fischbacher U, Feierabend A, Lutz K, Fehr E. The neural circuitry of a broken promise. Neuron 2009;64:756-70. [Crossref] [PubMed]
- Levens SM, Phelps EA. Insula and orbital frontal cortex activity underlying emotion interference resolution in working memory. J Cogn Neurosci 2010;22:2790-803. [Crossref] [PubMed]
- Menon V, Uddin LQ. Saliency, switching, attention and control: a network model of insula function. Brain Struct Funct 2010;214:655-67. [Crossref] [PubMed]
- Zhang Y, Wang Z, Du J, Liu J, Xu T, Wang X, Sun M, Wen Y, Li D, Liao H, Zhao Y, Zhao L. Regulatory Effects of Acupuncture on Emotional Disorders in Patients With Menstrual Migraine Without Aura: A Resting-State fMRI Study. Front Neurosci 2021;15:726505. [Crossref] [PubMed]
- Moerel M, De Martino F, Formisano E. An anatomical and functional topography of human auditory cortical areas. Front Neurosci 2014;8:225. [Crossref] [PubMed]
- Yi HG, Leonard MK, Chang EF. The Encoding of Speech Sounds in the Superior Temporal Gyrus. Neuron 2019;102:1096-110. [Crossref] [PubMed]
- Wei HL, Li J, Guo X, Zhou GP, Wang JJ, Chen YC, Yu YS, Yin X, Li J, Zhang H. Functional connectivity of the visual cortex differentiates anxiety comorbidity from episodic migraineurs without aura. J Headache Pain 2021;22:40. [Crossref] [PubMed]
- Houde F, Martel M, Coulombe-Lévêque A, Harvey MP, Auclair V, Mathieu D, Whittingstall K, Goffaux P, Léonard G. Perturbing the activity of the superior temporal gyrus during pain encoding prevents the exaggeration of pain memories: A virtual lesion study using single-pulse transcranial magnetic stimulation. Neurobiol Learn Mem 2020;169:107174. [Crossref] [PubMed]
- Messina R, Rocca MA, Colombo B, Teggi R, Falini A, Comi G, Filippi M. Structural brain abnormalities in patients with vestibular migraine. J Neurol 2017;264:295-303. [Crossref] [PubMed]
- Li X, Hao H, Li Y, Au LW, Du G, Gao X, Yan J, Tong RK, Lou W. Menstrually-related migraine shapes the structural similarity network integration of brain. Cereb Cortex 2023;33:9867-76. [Crossref] [PubMed]
- Nachtergaele P, Radwan A, Swinnen S, Decramer T, Uytterhoeven M, Sunaert S, van Loon J, Theys T. The temporoinsular projection system: an anatomical study. J Neurosurg 2019;132:615-23. [Crossref] [PubMed]
- Seeley WW, Menon V, Schatzberg AF, Keller J, Glover GH, Kenna H, Reiss AL, Greicius MD. Dissociable intrinsic connectivity networks for salience processing and executive control. J Neurosci 2007;27:2349-56. [Crossref] [PubMed]
- Chen Z, Chen X, Chen Z, Liu M, He H, Ma L, Yu S. Alteration of gray matter texture features over the whole brain in medication-overuse headache using a 3-dimentional texture analysis. J Headache Pain 2017;18:112. [Crossref] [PubMed]
- Ramnani N, Owen AM. Anterior prefrontal cortex: insights into function from anatomy and neuroimaging. Nat Rev Neurosci 2004;5:184-94. [Crossref] [PubMed]
- Schmitz N, Arkink EB, Mulder M, Rubia K, Admiraal-Behloul F, Schoonman GG, Kruit MC, Ferrari MD, van Buchem MA. Frontal lobe structure and executive function in migraine patients. Neurosci Lett 2008;440:92-6. [Crossref] [PubMed]
- Russo A, Tessitore A, Giordano A, Corbo D, Marcuccio L, De Stefano M, Salemi F, Conforti R, Esposito F, Tedeschi G. Executive resting-state network connectivity in migraine without aura. Cephalalgia 2012;32:1041-8. [Crossref] [PubMed]
- Zhao Q, Swati ZNK, Metmer H, Sang X, Lu J. Investigating executive control network and default mode network dysfunction in major depressive disorder. Neurosci Lett 2019;701:154-61. [Crossref] [PubMed]
- Tseng MT, Kong Y, Eippert F, Tracey I. Determining the Neural Substrate for Encoding a Memory of Human Pain and the Influence of Anxiety. J Neurosci 2017;37:11806-17. [Crossref] [PubMed]
- Zou Y, Tang W, Li X, Xu M, Li J. Acupuncture Reversible Effects on Altered Default Mode Network of Chronic Migraine Accompanied with Clinical Symptom Relief. Neural Plast 2019;2019:5047463. [Crossref] [PubMed]
- Maleki N, Linnman C, Brawn J, Burstein R, Becerra L, Borsook D. Her versus his migraine: multiple sex differences in brain function and structure. Brain 2012;135:2546-59. [Crossref] [PubMed]
- Ances BM, Detre JA. Perfusion changes with photic stimulation during two phases of the menstrual cycle: a pilot study comparing controls and true menstrual migraine patients. Cephalalgia 2003;23:907-13. [Crossref] [PubMed]