Differences in pulmonary nodular consolidation and pulmonary cavity among drug-sensitive, rifampicin-resistant and multi-drug resistant tuberculosis patients: the Guangzhou computerized tomography study
Introduction
The emergence of drug-resistant (DR) tuberculosis (TB) increases the burden of TB control. Multidrug-resistant tuberculosis (MDR-TB) refers to TB infection resistant to at least two first-line anti-TB drugs, isoniazid and rifampicin. About 0.5 million people developed DR-TB in 2018, of these 78% were MDR-TB, while only 1/3 of the confirmed MDR-TB cases were adequately treated (1). Extensively drug-resistant TB (XDR-TB) is defined as TB that has evolved resistance to rifampin and isoniazid, as well as to any member of the quinolone family and at least one of the second-line injectable drugs: kanamycin, amikacin, and capreomycin. Of MDR-TBs, XDR-TB accounts for 4–20% of these infections (2,3). Recently, a new category of DR Mycobacterium tuberculosis (M.tb) strain named totally drug-resistant TB (TDR-TB) has been identified, which is resistant to all first- and second-line drugs used to treat TB (4). Moreover, it is estimated that there are 1.9 million latent MDR-TB infected individuals around the world (5). When resistant mutants arise during treatment with anti-TB drugs, it is considered as acquired resistance (previously treated MDR-TB). Patients infected with an already drug-resistant strain develop primary resistance (new MDR-TB), which is observed in newly diagnosed TB patients. It has been estimated that globally 3.5% (which can be much higher in some regions) of newly diagnosed TB patients, and 20.5% of previously treated patients, are MDR-TB (1,6).
Despite that a wide range of geno- and phenotypic tests are available to detect DR M.tb strains and their susceptibility to drugs used, delay of appropriate MDR-TB treatment is common. Specimens used to detect MDR-pulmonary tuberculosis (PTB) are mostly sputum and bronchoalveolar lavage fluid, but when effective samples are not available, the utility of existing detection methods is limited. There have been interests to use chest imaging as a supporting tool to suggest the diagnosis of MDR-PTB (7). The suspicion of MDR/XDR-PTB by chest imaging can further guide and even intensify the diagnostic process for MDR-TB. A number of published articles described the potential imaging features difference between drug-sensitive (DS) and MDR-PTB (7-24). It had been suggested that MDR-TB cases tended to have more extensive disease, more likely to be bilateral, to have pleural involvement, to have bronchiectasis, and to have lung volume loss (7). XDR-TB overall appears even more aggressive than MDR-TB, with a greater number of cavities, larger cavities, and cavities of thicker wall (7,17). However, these signs alone are considered not sufficient for the differential diagnosis of MDR/XDR-PTB from DS-PTB (7). On the other hand, there may be no biological rationale that MDR-PTB shall demonstrates higher lung lesion extent or more lung destructions than DS-PTB. Some studies did not show imaging feature differences between DS-PTB vs. MDR-PTB or did not show imaging feature differences between MDR-PTB vs. XDR-PTB (25-27). In fact, that there is no imaging feature difference between DS-PTB and MDR-PTB is also the perception of many practicing radiologists (personal communications). There have been concerns that reported radiological feature differences between DS-PTB and MDR-PTB were confounded by that MDR cases tend to have a longer history prior to being diagnosed as MDR, thus the radiological features shown in MDR-PTB may not be intrinsic to MDR-PTB pathology. The variation in imaging manifestations across the studies could be a consequence of differential time intervals between disease onset and chest imaging (7).
Based on earlier literature reviews (20,21,28) and our own analysis (9), we considered that pulmonary nodular (PN) and pulmonary cavity (PC) represent the two most promising imaging signs in differentiating MDR-PTB from DS-PTB. In a recent study [Dalian study (29)], using history length matched DS-PTB and MDR-PTB cases from a well-defined urban region in Dalian, China, we analysed the CT feature differences of these paired cases with a focus on PN and PC. There were 33 consecutive MDR-PTB cases [inclusive of rifampicin-resistant (RR) cases], with 19 cases had a history of <1 month, and 8 and 6 cases had a history of 1–6 and >6 months respectively. To pair the MDR-PTB cases according to the history length, matched 33 cases of DS-PTB patients were included. The first computed tomography (CT) exams prior to treatment were analysed. It was found that, compared with DS cases, MDR cases had a higher prevalence of PN and a higher number of PN per positive case for PN. For the cases >1 month history, MDR-PTB had a higher number of PC per positive case than that of DS-PTB cases. The lung field distribution of all lesions tended to be wider for MDR-PTB cases. Since the Dalian study only had a limited sample size (29), we conducted another study (the Guangzhou study) using patient data from another hospital in Guangzhou, China, with the goal to confirm these newly noted results.
Methods
Patient data
This study was approved by our institutional ethics committee and conducted in accordance with the Declaration of Helsinki (as revised in 2013). The patient consent was waived due to the retrospective nature of this study. All PTB cases were from the Guangzhou Chest Hospital, Guangzhou, China. Data was retrieved from the electronic case archiving system of the hospital. According to the 2016 World Health Organization (WHO) update, MDR-PTB management strategy is recommended for all patients with RR-PTB, regardless of confirmation of the isoniazid resistance (30); therefore, in this study RR-PTB was included as MDR-PTB cases. We retrieved data of consecutive MDR/RR cases treated during the period of July 2020 and December 2021, and according to the electronic case archiving system records, the main PTB-related symptoms/signs history was ≤3 months till the first CT scan in Guangzhou Chest Hospital was taken. We only included new cases who had never been treated for TB. In total we retrieved 46 MDR-PTB cases (34 males, 12 females, age: 38.5±16.85 years, range: 19–84 years, among them 22 cases were RR resistant cases, 47.8%). For the 24 (non-RR) MDR-PTB cases, 13 cases, 8 cases, 2 cases, and 1 case were resistant to 2, 3, 4, and 5 anti-TB drugs respectively (Table S1). The Drug sensitivity confirmation tests included a combination of sputum culture and Genexpert test results. There was no XDR-PTB among our cases. To pair the MDR-PTB cases with equal disease history length, from our hospital database we retrospectively collected 46 cases of PTB patients (31 males, 15 females, age: 46±18 years, range: 18–83 years) who were confirmed to be DS-TB. These DS cases were diagnosed and treated in our hospital also during July December 2020 and December 2021. All patients were HIV-negative, and none had immunocompromised status.
The first CT in Guangzhou Chest Hospital was analysed in this study. For all cases, lung CT was performed with a TOSHIBA Asteion 16-slice spiral CT scanner. The slice thickness was 5.0 mm. Only plain CT scans without contrast agent administration were analysed in this study. Since in many cases earlier chest X-ray or CT conducted in primary care clinics were available, some patients started anti-TB treatment before the CT examination in Guangzhou Chest Hospital (Figure 1).
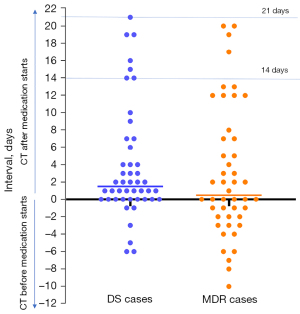
All PTB lesions were read for their extent. A longitudinal axis was taken from the upper apices of the lungs down to the diagram, and then this axis was divided into three segments of equal length with each segment correlated to a zone. In this way, two lungs were divided into six zones. Modifications were made to the Fleischner Society Glossary definitions for PN and PC (29,31). A PN was a rounded or oval (but not band-like) solid opacity with a relatively clear boundary measuring between >6 mm to 3 cm in diameter (Figure 2), and quantified for their number. Smaller nodules and aggregation of smaller nodules were not counted as PN in this study. A PC was a gas-filled space, seen as a lucency area within pulmonary consolidation or a nodule. PC was counted only for those with a lumen diameter >5 mm. Multiple cavities in a single consolidation is counted as one cavity. Worm-eroding like cavity (WELC) is more likely to be numerous, these usually small cavities in one consolidation were together counted as one cavity. A PC within a PN was counted as both one PC and one PN. PC was also differentiated from bulla and cyst with a thin wall. CT images were jointly read by a radiology trainee (SNT) and a specialist radiologist (YXJW), with consensus all achieved.
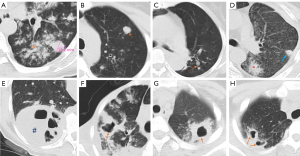
The percentage lung volume involvements by TB lesions were evaluated quantitatively, using PyCharm (JetBrains s.r.o., Prague, Czech Republic). Lung parenchyma regions were initially extracted by a combination of CT density thresholding and morphology. Then CT density thresholding was applied to segment diseased parts of the lung parenchyma (i.e., areas with increased CT density), sparing healthy lung areas. Graphical operation was applied to fill in the blood vessels in the lungs as well as to divide the boundaries of the bronchi. Finally, the volume of the diseased lung was calculated by counting the number of voxels in all slices.
During the course of CT assessment, it became clear to the authors that some of the patients had PTB history much longer than 3 months. Apparent radiological signs associated with chronicity, such as extensive fibrosis, apparent lesion calcifications, or contraction of the chest cage due to lung destruction, were noted in 10 cases in the DS group (10/46) and 9 cases in the MDR group (10/46). Further discussions were held with respiratory physicians, and it was informed that such a phenomenon is relatively common among Guangzhou Chest Hospital patients. A substantial portion of patients often ignore mild symptoms initially, and only report the history when the symptoms become more apparent. Additional checking showed that, 22 (47.8%, 22/46) of the DS patients and 30 (65.2%, 30/46, P=0.09) of the MDR patients were from rural communities.
Statistical analysis was processed using GraphPad Prism (San Diego, CA, USA). Comparisons between two groups were conducted with Chi-squared test for categorical variables and Mann-Whitney U test for continuous variable. Receiver operating characteristic (ROC) curve analysis was used to determine the diagnostic performance, reporting the area under the ROC (AUROC) and optimal cut-off values with sensitivity and specificity. A P value of less than 0.05 was considered statistically significant.
Results
With both DS and MDR cases, some patients had lesions that only involved a small part of the lungs, whereas some patients had very extensive lesions including thick-walled cavities (Figures 3,4). The lung volume involvement was 11.3%±8.3% for DS cases and 8.4%±6.6% for MDR cases (P=0.022, Figure 5). DS cases showed a slightly higher extent of lung volume involvement than MDR cases.
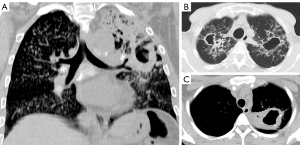
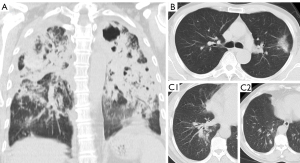
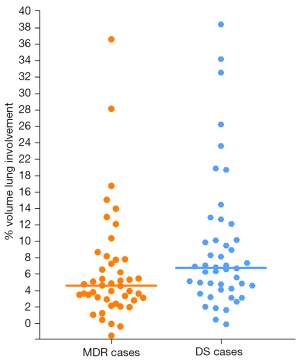
The DS-PTB vs. MDR-PTB differences of prevalence (positive rate) and lesion number for PN and PC are shown in Tables 1,2, and Figures 6,7. PC and any lesion distributions tended to be slightly wider for MDR-PTB cases. There was no statistical difference between DS cases and MDR cases both in PN prevalence and in PC prevalence. For positive cases, MDR-PTB had more PN number and PC number, and this was statistically significant for PC.
Table 1
Lesions | Prevalence (%) | Mean No. # | Mean size # |
---|---|---|---|
PN | |||
DS-PTB | 63.04 (29/46) | 2.28 | 12.75 |
MDR-PTB | 69.57 (32/46) | 2.63 | 11.90 |
P value | 0.508 | 0.381 | 0.681 |
PC | |||
DS-PTB | 63.04 (29/46) | 1.38 | 18.40 |
MDR-PTB | 60.87 (28/46) | 2.14 | 18.36 |
P value | 0.830 | 0.001 | 0.967 |
#, counting per positive case. DS, drug-sensitive; PTB, pulmonary tuberculosis; MDR, multidrug-resistant resistant (including rifampicin); PN, pulmonary nodular; PC, pulmonary cavity.
Table 2
Distribution | PN | PC | Any lesions | |||||
---|---|---|---|---|---|---|---|---|
DS (n=29) | MDR (n=32) | DS (n=29) | MDR (n=28) | DS (n=46) | MDR (n=46) | |||
Lung fields | 1.58±0.76† | 1.50±0.61† | 1.24±0.43‡ | 1.64±0.85‡ | 1.77±0.83§ | 2.07±1.06§ | ||
Bilateral lungs | 10 (34.48) | 5 (15.63) | 4 (13.79) | 7 (25.00) | 14 (18.75) | 14 (20.83) | ||
R upper field | 5 (17.24) | 9 (28.13) | 10 (34.48) | 14 (50.00) | ||||
L upper field | 12 (41.38) | 14 (43.75) | 13 (44.83) | 13 (46.43) | ||||
Upper fields (R + L) | 17 (58.62) | 23 (71.88) | 23 (79.31) | 27 (96.43) | ||||
R middle field | 8 (27.59) | 5 (15.63) | 1 (3.45) | 5 (17.86) | ||||
L middle field | 9 (31.03) | 7 (21.88) | 4 (13.79) | 10 (35.71) | ||||
Middle fields (R + L) | 17 (58.62) | 12 (37.50) | 5 (19.23) | 15 (31.25) | ||||
R lower field | 5 (17.24) | 6 (18.75) | 3 (17.24) | 5 (17.86) | ||||
L lower field | 7 (24.14) | 5 (15.63) | 2 (6.90) | 2 (7.14) | ||||
Lower fields (R + L) | 12 (41.38) | 11 (34.38) | 5 (17.24) | 7 (25.00) |
Data are presented as mean ± standard deviation or number (percentage). †, P=0.819; ‡, P=0.034; §, P=0.106. DS, drug-sensitive; MDR, multidrug-resistant; PN, pulmonary nodular; PC, pulmonary cavity; R, right; L, left.
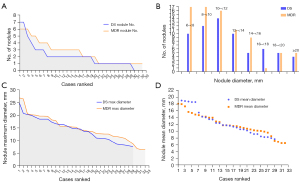
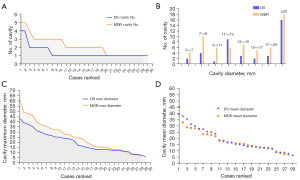
AUROC analysis results are selectively shown in Table 3. Together with Dalian results, data suggests PC/PN number ≥3 or 4 were associated with a high probability of the PTB patient to be MDR. Graphic analysis shows a combination of PC number and maximum diameter of PC allowed a separation of DS cases and MDR cases on probability term (Figure 8); and a combination of PC number, maximum diameter of PC, and PN number might even allow an even better statistical separation of DS cases and MDR cases (Figure 9).
Table 3
Data source | No. of lesions | Specificity, % | Sensitivity, % |
---|---|---|---|
PN | |||
Current study | ≥4 | 86 | 25 |
Song et al. (29) | ≥3 | 93.9 | 48.5 |
PC | |||
Current study | ≥3 | 93 | 36 |
Song et al. (29) | ≥4 | 84.9 | 39.4 |
Both this Guangzhou study and the Dalian study show PN/PC number of ≥3 or ≥4 suggests relatively high specificity for suggesting MDR, however the sensitivities are relatively low. PN, pulmonary nodular; PC, pulmonary cavity; MDR, multidrug-resistant.
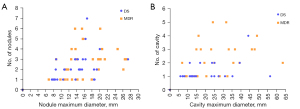
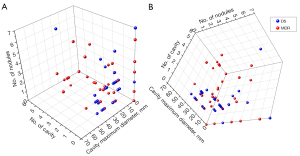
Discussion
Despite our initial intention to recruit patients with clearly defined short disease history length of <3 months, the results of recruitment were not as anticipated but these still reflect clinical practice in many scenarios. This experience was different from that of the Dalian study (29), and mostly likely related to the hospital setting and patient sources. Moreover, this Guangzhou study is a radiologist-initiated study, being less sensitive to the issues of history taking during the initial patient inclusion. It may be possible that DS cases could have had an overall slightly longer disease history, due to that 10 cases (21.7%) of DS cases had apparent chronicity CT sign while 9 cases (19.6%) of MDR cases had apparent chronicity CT sign. This may also help explain that lung involvement volume percentage was higher for DS patients than for MDR patients, being 11.3%±8.3% for DS cases and 8.4%±6.6% for MDR cases (P=0.022). However, a higher number of MDR cases (MDR: 65.2%; DS: 47.8%) were from rural communities, and rural community patients may seek medical care at a later stage of the disease. Overall, considering our sample strategy, we believe that the history length difference between DS patients and MDR patients in the current study might not be substantial.
The general perception of many practicing radiologists is that there is no imaging feature difference between DS-PTB and MDR-PTB, and it is impossible to differentiate MDR-PTB from DS-PTB subjectively on chest imaging. Indeed, our current quantitative analysis showed lesion volume extent was even higher among DS patients than among MDR patients. The distributions of PC and all lesions were only slightly wider for MDR-PTB cases than for DS-PTB cases (Table 1). This is consistent with our Dalian study, where the mean lung field involvement was 3.52 for DS cases and 3.88 for MDR cases, and bilateral lung involvement was 51.5% for DS cases and 66.7% for MDR cases (29).
Our recent Dalian study showed that MDR cases had a higher prevalence of PN and a higher number of PN per positive case for PN (29). The difference was apparent even for the cases with <1 month history. On the other hand, it takes time for PC lesions to develop in the infected lungs (29). In contrast to our Dalian study where for early-stage cases a bigger difference was noted for PN (rather than for PC) between DS-PTB and MDR-PTB, our current study showed for cases with a possibly longer disease history a bigger difference was noted for PC rather than for PN, which is on the other hand consistent with a number of earlier reports (7,10,17,21,22). Thus, this study further supports the notion that we should consider patient history length when analyzing the CT features of PTB patients. The current study and the Dalian study both suggest that PN/PC number ≥3 or 4 may be associated with a high probability of a PTB patient being MDR (Table 3). This is particularly interesting considering that in this study the lesion lung involvement percentage is higher in DS patients than in MDR patients. Our current study further supports that a combination of PC number, PC maximum diameter, and PN number might allow a separation of DS-PTB cases and MDR-PTB cases on statistical terms. Current difficulties include that qualification of PC/PN numbers and sizes are not only time consuming but also are associated with some subjectivities for smaller lesions or clustered lesions quantifications. It is noted that, for PC and PN positive cases, the Dalian results had a higher PC/PN number per case for the high-count cases than the current study. We will further investigate to understand if this was due to genuine patient differences, or it was due to PC/PN identification subjectivity. Further development of artificial intelligence technology may allow better consistency in lesion characterization with high time-efficiency (32-35). We also plan to publish a teaching atlas to standardize human reading.
According to the 2016 WHO update, MDR-PTB management strategy is recommended for all patients with RR-PTB, regardless of confirmation of the isoniazid resistance (30). Therefore, in clinical practice, the differentiation between RR cases and true MDR cases may be less important. However, we tentatively checked the PN/PC differences between RR cases and true MDR cases in this study, and the results are shown in Figure S1 and Table S2. The lung volume involvement was 9.37%±8.57% for RR cases, and 7.43%±4.04% for true MDR cases (P>0.05). Table S2 shows a slight trend of more PN and PC changes among true MDR cases than among RR cases, though there was no statistical significance. We additionally reviewed our Dalian results (29) (Figure S2). The data also tentatively suggest that MDR cases might have demonstrated higher PN/PC counts for true MDR cases than for RR cases. More studies are required to confirm this point.
There are many limitations to this study. The first limitation is that we failed to quantify disease history length which is a subjective measure by patients themselves. Due to the nature of the setup of Guangzhou Chest Hospital, a large proportion of the patients (56.5% in total) in this study were from rural areas, it is understandable that some of the patients only presented to the hospital till their discomforts reached a certain degree, or their discomforts had protracted for a long period of time. However, such a phenomenon may represent a real-world possibility. It should be noted that the patients were not all treatment naive when the first CT scan was taken in Guangzhou Chest Hospital. While how this would have affected the results of this study is unknown, however, we expect the impact on the analysed results would be small. In the study of Lee et al. (36), 1 month after anti-TB treatment, 59.2% (45/76) cases had chest X-ray improvement whereas 34.2% (26/76) did not show chest X-ray changes. Two months after anti-TB treatment, 71.9% (100/139) cases had chest X-ray improvement whereas 34.2% (35/139) did not show chest X-ray changes. In the study of How et al. (37), 8 weeks after anti-TB treatment, 61.7% (71/115) cases had chest X-ray improvement while 30.4% (35/115) did not show chest X-ray changes. In a more recent quantitative 18F-FDG PET-CT study, Malherbe et al. (38) described that 1 month into anti-TB treatment, most cases had slight improvement in metabolic lesion volume and mean lesion intensity. For the cases in the current study, when the CT was taken, most cases had drug treatment for less than 2 weeks, and none had been treated for more than 3 weeks (Figure 1). We can anticipate this duration of treatment may have only slightly improved some exudative lesions or some infiltrative lesions but change for the PN (which is more solid) and PC (which is known to be slower in drug treatment response) may be group-wise minimal statistically. Another limitation is our relatively small sample size, which also limits the further analysis for the differences between RR patients and true MDR-patients. Since all our cases were new MDR-PTB, whether these features can be generalized to previously treated PTB or child patients should be further investigated (7,39,40).
Conclusions
In conclusion, following the Dalian study (29), this study continues to suggest a combination of PC number, maximum PC diameter, and PN number may statistically suggest the probability of MDR-PTB. This study further supports the notion that we should consider patient disease history length when analyzing the CT features of MDR-PTB patients.
Acknowledgments
Funding: This study was supported by
Footnote
Conflicts of Interest: All authors have completed the ICMJE uniform disclosure form (available at https://qims.amegroups.com/article/view/10.21037/qims-23-694/coif). Y.X.J.W. serves as the Editor-in-Chief of Quantitative Imaging in Medicine and Surgery. The other authors have no conflicts of interest to declare.
Ethical Statement: The authors are accountable for all aspects of the work in ensuring that questions related to the accuracy or integrity of any part of the work are appropriately investigated and resolved. This study was approved by our institutional ethics committee and conducted in accordance with the Declaration of Helsinki (as revised in 2013). The patient consent was waived due to the retrospective nature of this study.
Open Access Statement: This is an Open Access article distributed in accordance with the Creative Commons Attribution-NonCommercial-NoDerivs 4.0 International License (CC BY-NC-ND 4.0), which permits the non-commercial replication and distribution of the article with the strict proviso that no changes or edits are made and the original work is properly cited (including links to both the formal publication through the relevant DOI and the license). See: https://creativecommons.org/licenses/by-nc-nd/4.0/.
References
- World Health Organization. Global Tuberculosis Report 2019. Available online: https://www.who.int/publications/i/item/9789241565714
- Raviglione MC, Smith IM. XDR tuberculosis--implications for global public health. N Engl J Med 2007;356:656-9. [Crossref] [PubMed]
- Aziz MA, Wright A, Laszlo A, De Muynck A, Portaels F, Van Deun A, Wells C, Nunn P, Blanc L, Raviglione MGlobal Project on Anti-Tuberculosis Drug Resistance Surveillance. Epidemiology of antituberculosis drug resistance (the Global Project on Anti-tuberculosis Drug Resistance Surveillance): an updated analysis. Lancet 2006;368:2142-54. [Crossref] [PubMed]
- Parida SK, Axelsson-Robertson R, Rao MV, Singh N, Master I, Lutckii A, Keshavjee S, Andersson J, Zumla A, Maeurer M. Totally drug-resistant tuberculosis and adjunct therapies. J Intern Med 2015;277:388-405. [Crossref] [PubMed]
- Knight GM, McQuaid CF, Dodd PJ, Houben RMGJ. Global burden of latent multidrug-resistant tuberculosis: trends and estimates based on mathematical modelling. Lancet Infect Dis 2019;19:903-12. [Crossref] [PubMed]
- World Health Organization. Global tuberculosis report. 2014. Available online: https://apps.who.int/iris/handle/10665/137094
- Wáng YXJ, Chung MJ, Skrahin A, Rosenthal A, Gabrielian A, Tartakovsky M. Radiological signs associated with pulmonary multi-drug resistant tuberculosis: an analysis of published evidences. Quant Imaging Med Surg 2018;8:161-73. [Crossref] [PubMed]
- Song Q, Guo X, Zhang L, Yang L, Lu X. New Approaches in the Classification and Prognosis of Sign Clusters on Pulmonary CT Images in Patients With Multidrug-Resistant Tuberculosis. Front Microbiol 2021;12:714617. [Crossref] [PubMed]
- Huang XL, Skrahin A, Lu PX, Alexandru S, Crudu V, Astrovko A, et al. Prediction of multiple drug resistant pulmonary tuberculosis against drug sensitive pulmonary tuberculosis by CT nodular consolidation sign. bioRxiv preprint 2019. Doi:
10.1101/833954 . - Chuchottaworn C, Thanachartwet V, Sangsayunh P, Than TZ, Sahassananda D, Surabotsophon M, Desakorn V. Risk Factors for Multidrug-Resistant Tuberculosis among Patients with Pulmonary Tuberculosis at the Central Chest Institute of Thailand. PLoS One 2015;10:e0139986. [Crossref] [PubMed]
- Chung MJ, Lee KS, Koh WJ, Kim TS, Kang EY, Kim SM, Kwon OJ, Kim S. Drug-sensitive tuberculosis, multidrug-resistant tuberculosis, and nontuberculous mycobacterial pulmonary disease in nonAIDS adults: comparisons of thin-section CT findings. Eur Radiol 2006;16:1934-41. [Crossref] [PubMed]
- Kim HC, Goo JM, Lee HJ, Park SH, Park CM, Kim TJ, Im JG. Multidrug-resistant tuberculosis versus drug-sensitive tuberculosis in human immunodeficiency virus-negative patients: computed tomography features. J Comput Assist Tomogr 2004;28:366-71. [Crossref] [PubMed]
- Kim SH, Min JH, Lee JY. Radiological Findings of Primary Multidrug-resistant Pulmonary Tuberculosis in HIV-seronegative Patients. Hong Kong J Radiol 2014;17:4-8.
- Yeom JA, Jeong YJ, Jeon D, Kim KI, Kim CW, Park HK, Kim YD. Imaging findings of primary multidrug-resistant tuberculosis: a comparison with findings of drug-sensitive tuberculosis. J Comput Assist Tomogr 2009;33:956-60. [Crossref] [PubMed]
- Kim W, Lee KS, Kim HS, Koh WJ, Jeong BH, Chung MJ, Jang HW. CT and microbiologic follow-up in primary multidrug-resistant pulmonary tuberculosis. Acta Radiol 2016;57:197-204. [Crossref] [PubMed]
- Cha J, Lee HY, Lee KS, Koh WJ, Kwon OJ, Yi CA, Kim TS, Chung MJ. Radiological findings of extensively drug-resistant pulmonary tuberculosis in non-AIDS adults: comparisons with findings of multidrug-resistant and drug-sensitive tuberculosis. Korean J Radiol 2009;10:207-16. [Crossref] [PubMed]
- Jung YB, Jung HJ, Siti HT, Lee YS, Lee HJ, Lee SH, Cheon HY. Comparison of anterior cruciate ligament reconstruction with preservation only versus remnant tensioning technique. Arthroscopy 2011;27:1252-8. [Crossref] [PubMed]
- Lee ES, Park CM, Goo JM, Yim JJ, Kim HR, Lee HJ, Lee IS, Im JG. Computed tomography features of extensively drug-resistant pulmonary tuberculosis in non-HIV-infected patients. J Comput Assist Tomogr 2010;34:559-63. [Crossref] [PubMed]
- Fishman JE, Sais GJ, Schwartz DS, Otten J. Radiographic findings and patterns in multidrug-resistant tuberculosis. J Thorac Imaging 1998;13:65-71. [Crossref] [PubMed]
- Sulistijawati RS, Icksan AG, Lolong DB, Nurwidya F. Thoracic Radiography Characteristics of Drug Sensitive Tuberculosis and Multi Drug Resistant Tuberculosis: A Study of Indonesian National Tuberculosis Prevalence Survey. Acta Medica (Hradec Kralove) 2019;62:24-9. [Crossref] [PubMed]
- Saifullah A, Mallhi TH, Khan YH, Iqbal MS, Alotaibi NH, Alzarea AI, Rasheed M. Evaluation of risk factors associated with the development of MDR- and XDR-TB in a tertiary care hospital: a retrospective cohort study. PeerJ 2021;9:e10826. [Crossref] [PubMed]
- Li CH, Fan X, Lv SX, Liu XY, Wang JN, Li YM, Li Q. Clinical and Computed Tomography Features Associated with Multidrug-Resistant Pulmonary Tuberculosis: A Retrospective Study in China. Infect Drug Resist 2023;16:651-9. [Crossref] [PubMed]
- Prasitpuriprecha C, Jantama SS, Preeprem T, Pitakaso R, Srichok T, Khonjun S, Weerayuth N, Gonwirat S, Enkvetchakul P, Kaewta C, Nanthasamroeng N. Drug-Resistant Tuberculosis Treatment Recommendation, and Multi-Class Tuberculosis Detection and Classification Using Ensemble Deep Learning-Based System. Pharmaceuticals (Basel) 2022;16:13. [Crossref] [PubMed]
- Shin HS, Choi DS, Na JB, Choi HY, Kim JE, Choi HC, Won JH, Lee SJ, Park MJ. Low pectoralis muscle index, cavitary nodule or mass and segmental to lobar consolidation as predictors of primary multidrug-resistant tuberculosis: A comparison with primary drug sensitive tuberculosis. PLoS One 2020;15:e0239431. [Crossref] [PubMed]
- Oriekot A, Sereke SG, Bongomin F, Bugeza S, Muyinda Z. Chest X-ray findings in drug-sensitive and drug-resistant pulmonary tuberculosis patients in Uganda. J Clin Tuberc Other Mycobact Dis 2022;27:100312. [Crossref] [PubMed]
- Xu L, Xu S. CT Imaging Characteristics of Nontuberculous Mycobacteria Lung Disease, Active Tuberculosis and Multi-Drug Resistant Tuberculosis. Sarcoidosis Vasc Diffuse Lung Dis 2022;39:e2022008. [Crossref] [PubMed]
- Mehrian P, Farnia P, Jalalvand D, Chamani MR, Bakhtiyari M. Computerised tomography scan in multi-drug-resistant versus extensively drug-resistant tuberculosis. Pol J Radiol 2020;85:e39-44. [Crossref] [PubMed]
- Flores-Treviño S, Rodríguez-Noriega E, Garza-González E, González-Díaz E, Esparza-Ahumada S, Escobedo-Sánchez R, Pérez-Gómez HR, León-Garnica G, Morfín-Otero R. Clinical predictors of drug-resistant tuberculosis in Mexico. PLoS One 2019;14:e0220946. [Crossref] [PubMed]
- Song QS, Zheng CJ, Wang KP, Huang XL, Tartakovsky M, Wáng YXJ. Differences in pulmonary nodular consolidation and pulmonary cavity among drug-sensitive, rifampicin-resistant and multi-drug resistant tuberculosis patients: a computerized tomography study with history length matched cases. J Thorac Dis 2022;14:2522-31. [Crossref] [PubMed]
- WHO treatment guidelines for drug resistant tuberculosis OCTOBER 2016 revision. Available online: https://apps.who.int/iris/bitstream/handle/10665/250125/9789241549639-eng.pdf
- Hansell DM, Bankier AA, MacMahon H, McLoud TC, Müller NL, Remy J. Fleischner Society: glossary of terms for thoracic imaging. Radiology 2008;246:697-722. [Crossref] [PubMed]
- Xu Z, Bagci U, Kubler A, Luna B, Jain S, Bishai WR, Mollura DJ. Computer-aided detection and quantification of cavitary tuberculosis from CT scans. Med Phys 2013;40:113701. [Crossref] [PubMed]
- Jaeger S, Juarez-Espinosa OH, Candemir S, Poostchi M, Yang F, Kim L, Ding M, Folio LR, Antani S, Gabrielian A, Hurt D, Rosenthal A, Thoma G. Detecting drug-resistant tuberculosis in chest radiographs. Int J Comput Assist Radiol Surg 2018;13:1915-25. [Crossref] [PubMed]
- Zhou W, Cheng G, Zhang Z, Zhu L, Jaeger S, Lure FYM, Guo L. Deep learning-based pulmonary tuberculosis automated detection on chest radiography: large-scale independent testing. Quant Imaging Med Surg 2022;12:2344-55. [Crossref] [PubMed]
- Yang F, Yu H, Kantipudi K, Karki M, Kassim YM, Rosenthal A, Hurt DE, Yaniv Z, Jaeger S. Differentiating between drug-sensitive and drug-resistant tuberculosis with machine learning for clinical and radiological features. Quant Imaging Med Surg 2022;12:675-87. [Crossref] [PubMed]
- Lee JH, Kim OH, Kim YJ, Shim TS, Jo KW. Changes in chest X-ray findings in 1- and 2-month group after treatment initiation for suspected pulmonary tuberculosis. Korean J Intern Med 2020;35:1145-53. [Crossref] [PubMed]
- How SH, Kuan YC, Ng TH, Razali MR, Fauzi AR. Monitoring treatment response in sputum smear positive pulmonary tuberculosis patients: comparison of weight gain, sputum conversion and chest radiograph. Malays J Pathol 2014;36:91-6.
- Malherbe ST, Chen RY, Dupont P, Kant I, Kriel M, Loxton AG, et al. Quantitative 18F-FDG PET-CT scan characteristics correlate with tuberculosis treatment response. EJNMMI Res 2020;10:8. [Crossref] [PubMed]
- Geng E, Kreiswirth B, Burzynski J, Schluger NW. Clinical and radiographic correlates of primary and reactivation tuberculosis: a molecular epidemiology study. JAMA 2005;293:2740-5. [Crossref] [PubMed]
- Manikkam S, Archary N, Bobat R. Chest X-ray patterns of pulmonary multidrug-resistant tuberculosis in children in a high HIV-prevalence setting. SA Journal of Radiology 2016;20:1-6.