Hippocampal volume and resting-state functional connectivity on magnetic resonance imaging in patients with Parkinson and depression
Introduction
Depression is the most common nonmotor psychiatric symptom in patients with Parkinson disease (PD) (1). Depressive symptoms are seen in about 35% of patients with PD (2). PD-related depression mainly manifests as a depressed mood and anhedonia. Depressive symptoms in patients with PD precede motor symptoms and are associated with PD duration, severity of motor symptoms, or dose of dopaminergic medication used (3). In recent years, neuroimaging research has reported multiple neurobiological models in explaining the pathogenesis of depression in PD (DPD). Numerous investigations have revealed that the limbic system is crucial to the development of DPD (4-6). As an important component of limbic system, the hippocampus is involved in the regulation of cognition and emotion.
The structure and function of the hippocampus have received considerable attention as a potential neurological basis for cognitive dysfunction and other nonmotor symptoms, such as depression, impulse control disorder, and hypoxemia (7,8). The hippocampus is a crucial subcortical structure associated with depression (9,10). Magnetic resonance imaging (MRI) studies of cognitively healthy persons with depression revealed subtle structural brain changes in the hippocampus (11-13). Meanwhile, other studies suggest that abnormal hippocampal formation in patients with PD may be associated with depression (14,15).
Resting-state functional MRI (rs-fMRI) is a noninvasive method that focuses on examining blood oxygen level-dependent (BOLD) spontaneous activity at low frequency in the brain at rest (16-18). Over the past 10 years, rs-fMRI has been extensively adopted in a variety of domains, including the investigation of brain dysfunction in PD (19,20), with research into resting-state functional connectivity (rs-FC) demonstrating particularly rapid progress. Different methodologies have already been applied to assess changes of BOLD signals in the brain, with the seed point-based method being one of the most important approaches for assessing changes of BOLD signals in the brain (21). Seed point-based analysis is essentially a model-based approach that selects seed points or regions of interest (ROIs) and finds the linear correlation of that seed-point region with all other voxels throughout the brain, producing a seed-based FC map (21).
Recent structural and functional imaging studies of PD-related depression have failed to reveal the mechanism of DPD, and relatively few studies have been conducted to examine the limbic systems such as the hippocampus. Some studies have recently reported there to be changes in hippocampal volume in patients with DPD, mainly in the form of smaller volumes compared to those of controls. However, the main areas of hippocampal volume reduction are not entirely consistent across these studies due to differences in study methods and techniques (22,23). In this study, we further investigated the abnormalities of hippocampal structure and resting-state function in patients with DPD through a combined high-resolution MRI-based volumetric and FC analysis. We also compared the DPD and nondepressed PD (NDPD) to distinguish the influence on hippocampus between depression and PD. This study may provide new insights into the pathogenesis of DPD and serve as a basis for the diagnosis and treatment of DPD. We present this article in accordance with the MDAR reporting checklist (available at https://qims.amegroups.com/article/view/10.21037/qims-23-919/rc).
Methods
Participants
In this study, 30 patients with DPD (DPD group; 17 females, 13 males), 30 patients with NDPD (NDPD group; 14 females, 16 males), and 30 normal controls (NCs; NC group; 15 females, 15 males) with no significant age or gender differences with the DPD group were included. The patients with DPD and NDPD were recruited from January 2017 to September 2020. The inclusion criteria of all patients were as follows: (I) patients diagnosed with PD according to the UK Parkinson’s Disease Society (PDS) Brain Bank Criteria; (II) patients with Hoehn and Yahr (H&Y) staging <3; (III) patients who had not received antidepressant drugs prior to this study; (IV) patients with Mini-Mental State Examination (MMSE) score >24; (V) right-handed patients; and (VI) in the DPD group, patients with PD diagnosed as DPD following the Diagnostic and Statistical Manual of Mental Disorders (DSM) and Hamilton Depression Rating Scale 17 item (HAMD-17) score >7. The exclusion criteria were as follows: (I) patients with a history of severe cerebrovascular disease, epilepsy, genetic diseases, or head trauma; (II) patients with manifestations of intracranial diseases on MRI; (III) patients with metal objects in their body that could not be scanned with MRI; and (IV) patients with claustrophobia who cannot complete MRI scans.
The inclusion criteria for the NC group included volunteers with the same age and gender as the patients in the DPD group. The exclusion criteria for the NC group were as follows: (I) patients with a history of psychosis, genetic diseases, or head trauma; (II) patients with a history of heavy alcohol consumption; (III) patients with positive results on neuropsychiatric examination; and (IV) patients with the manifestations of intracranial diseases on MRI images.
The study was conducted in accordance with the Declaration of Helsinki (as revised in 2013) and was approved by the Ethics Committee of Nantong First People’s Hospital. Informed consent was taken from all the patients. In addition, measurement of the relevant scales (H&Y staging, MMSE, and HAMD-17) were conducted 12 hours after the patients stopped taking parkinsonism-related drugs.
Clinical methods
Data collection
A GE Discovery 750-W 3.0-T MRI scanner (GE HealthCare, Chicago, IL, USA) was adopted for brain scanning. A standard head coil was used to fix the brain, and the entire brain was scanned. Special pads were used to hold the participant’s head in place to prevent it from moving during the scan, and special headphones were used to reduce noise interference. All participants were instructed to keep their heads still during the scan, close their eyes, relax as much as possible, and avoid any thinking. We obtained three-dimensional T1-weighted imaging (3D-T1WI) images and BOLD images from the included participants.
The scanning parameters of 3D-T1WI imaging were as follows: field of view (FOV) =256 mm × 256 mm; slice thickness =1 mm; repetition time (TR) =8.6 ms; echo time (TE) =3.2 ms; matrix =256×256; voxel size =1 mm × 1 mm × 1 mm; number of slices =176; and interslice space =0.
The scanning parameters of BOLD imaging were as follows: FOV =220 mm × 220 mm; slice thickness =3 mm; TR/TE =2,000 ms/30 ms; interslice space =1 mm; matrix =64×64; number of slices =36; flip angle (FA) =90°; scanning time =6 min 10 s; acquisition time points =185; and images =6,660.
Image and data processing
Measurements of hippocampal volume
In this study, 3D-T1WI images were obtained from all participants. The bilateral hippocampi of each case were manually segmented with the aid of ITK-SNAP 3.6.0 software (http://www.itksnap.org/pmwiki/pmwiki.php; Figure 1), and the volumes were measured using MATLAB software (MathWorks, Natick, MA, USA). The resulting hippocampi were used for morphological analysis. To reduce errors, each hippocampus was averaged in each case by three trained physicians after the volume was outlined and measured.
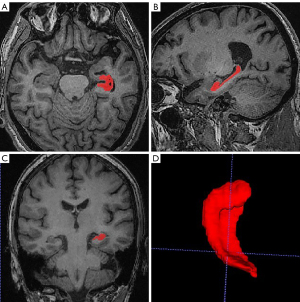
Preprocessing of rs-fMRI data
In this study, the rs-fMRI data collected with BOLD sequence were preprocessed by the Data Processing Assistant for Resting-State (DPARSF) toolbox on the MATLAB2014a platform, a MATLAB toolbox for processing brain MRI. Briefly, the pretreatment process consisted of the following steps: (I) format conversion was conducted, in which dcm2niigui in MRIcroN software was used to convert the Digital Imaging and Communications in Medicine (DICOM) format of the original image to the Neuroimaging Informatics Technology Initiative (NIfTI) format; (II) considering the instability of the participants and the machine at the beginning, the images of the first 10 time points were removed for each participant; (III) slice timing was performed, in which the remaining images were temporally aligned so that all layers of the brain were acquired at the same point in time; (IV) realignment was performed since the image acquisition process could have affected the signal due to noise generated by head movement, with the head movement rotation of the participants selected for this study being less than 1.5° or less than 1.5 mm flat movement; (V) space standardization was completed, in which all corrected image spaces were normalized to the Montreal Neurological Institute (MNI) template, with the data resampling for each participant being 3×3×3 mm3; (VI) the images were subjected to smoothing, which could reduce experimental errors, improve the signal-to-noise ratio of the data, and make the data more normally distributed, ultimately facilitating statistical analysis; Gaussian smoothing of image data was completed at a full width at half maximum (FWHM) of 8 mm × 8 mm × 8 mm; (VII) detrending was conducted because some manipulation was required (so that the resulting signal would accumulate a linear trend over time) to remove the linear drift due the scanning machine heating up during operation or due to the participant becoming fatigued from undergoing scanning for a long period of time; (VIII) filtering was used to remove low-frequency drift and high-frequency noise, such as the human breathing and heartbeat, and the physiological signal of low-frequency oscillation from 0.01 to 0.1 Hz was extracted; and (IX) regression was conducted to further reduce the effects of confounding factors, and the preprocessed data were subjected to removal of head movement parameters, with regression being completed for the covariates of head movement, cerebral white matter, and cerebrospinal fluid signals.
rs-FC
The bilateral hippocampi were used as the ROIs and as the seed points for FC. DPARSF software (http://rfmri.org/DPARSF) and Statistical Parametric Mapping 8 (SPM8) software (https://www.fil.ion.ucl.ac.uk/spm/software/spm8/) were used to complete the FC analysis of the preprocessed functional data of whole brain and the two seed points, respectively.
Statistical analysis
Statistical analysis of demographic and basic clinical data
The SPSS 22.0 (IBM Corp., Armonk, NY, USA) software was used for the statistical analysis of demographic and basic clinical data. After the normal distribution and homogeneity of variance for the collected quantitative data were verified, one-way analysis of variance (ANOVA) was performed for age among the three groups, and the chi-squared test was used to analyze gender. The duration of disease, levodopa-equivalent doses daily (LEDD), and HAMD scores between the DPD group and the NDPD group were analyzed using independent samples t-test. The significance level was set as α=0.05 for the results of all analyses, with P<0.05 being the threshold of a statistically significant difference.
Analysis of hippocampal volume
After the normal distribution and homogeneity of variance of the data were tested with SPSS 22.0 software, the differences in hippocampal volume among the DPD, NDPD, and NC groups were compared with ANOVA. Then post hoc pairwise comparisons were conducted to determine whether there were differences in hippocampal volume between any two groups (Bonferroni correction). Pearson correlation analysis was also performed between hippocampal volume and clinical index (HAMD scores) in the DPD group. Again, α=0.05 was considered set as the significance level for the results of all analyses, with P<0.05 being the threshold of a statistically significant difference.
Analysis of rs-FC data
The preprocessed data of the DPD group, NDPD group, and NC group were statistically analyzed with SPM8 software. Age, gender, and hippocampal volumes were taken as the covariates. ANOVA and a revised post hoc test conducted through AlphaSim testing were applied to determine the differences in FC of the whole brain based on left and right hippocampal seed points in the three groups. The results were corrected using AlphaSim, and after correction, the minimum cluster size was determined to be 46 voxels, corresponding to a corrected P value of 0.05. To investigate the relationship between neuroimaging abnormalities and depression severity, we extracted FC values from regions with significant differences in connectivity. Subsequently, a partial correlation analysis was performed between FC strength and HAMD scores in patients with DPD, with age and gender taken as covariates.
Results
Comparison of the demographic and basic clinical data among the three groups
In the DPD group, there were 30 patients, including 13 males and 17 females, and the age range was 43–77 years [mean ± standard deviation (SD), 65.5±7.8 years]. In the NDPD group, there were 30 patients, including 16 males and 14 females, and the age range was 43–75 years (mean ± SD, 64.1±8.0 years). In the NC group, there were 30 patients, including 15 males and 15 females, and the age range was 55–76 years (mean ± SD, 65.1±5.8 years). The comparisons of the demographic and basic clinical data in the DPD, NDPD, and NC groups are shown in Table 1. Disease duration was not statistically different between the DPD group and the NDPD group. Moreover, there was no statistical difference in age or gender between the DPD, NDPD, and NC groups. The HAMD score of the DPD group was higher than that of the NDPD group.
Table 1
Clinical data | DPD group | NDPD group | NC group | P value |
---|---|---|---|---|
Cases | 30 | 30 | 30 | – |
Gender (male/female) | 13/17 | 16/14 | 15/15 | 0.73 |
Age (years) | 65.5±7.8 | 64.1±8.0 | 65.1±5.8 | 0.75 |
Duration of PD (years) | 6.6±3.1 | 5.8±3.1 | NA | 0.32 |
HAMD score | 24.9±8.5 | 3.7±1.8 | NA | <0.001* |
LEDD (mg/d) | 401.7±150.6 | 366.7±162.1 | NA | 0.39 |
Data are presented as n or mean ± SD. *, the difference is significantly different. DPD, depression in Parkinson disease; NDPD, nondepressed Parkinson disease; NC, normal control; PD, Parkinson disease; NA, not available; HAMD, Hamilton Depression Rating Scale; LEDD, levodopa-equivalent doses daily; SD, standard deviation.
Comparison of hippocampal volume
The total right hippocampal volume was significantly larger than the total left hippocampal volume in the DPD, NDPD, and NC groups (see Table 2). Compared with those of the NC and NDPD groups, the bilateral hippocampal volumes of the DPD group were significantly reduced, and the difference was statistically significant (P<0.05) (Tables 3,4). However, no statistically significant difference in bilateral hippocampal volume existed between the NDPD group and the NC group (P>0.05) (Tables 3,4). Correlation analysis found a negative correlation between left and right hippocampal volumes and HAMD score in the DPD group (P<0.05) (Table 5). Overall, the bilateral hippocampal volumes of patients with DPD was decreased, and the hippocampal volumes were negatively correlated with the severity of depressive disorders.
Table 2
Groups | Hippocampal volume (mm3) | t value | P value | |
---|---|---|---|---|
Left | Right | |||
DPD | 2,564.40±298.21 | 2,637.37±300.02 | −4.292 | <0.001* |
NDPD | 2,753.30±239.71 | 2,868.70±299.20 | −3.258 | 0.003* |
NC | 2,877.27±278.84 | 2,995.00±330.36 | −3.695 | 0.001* |
Data are presented as mean ± SD. *, the difference is significantly different. DPD, depression in Parkinson disease; NDPD, nondepressed Parkinson disease; NC, normal control; SD, standard deviation.
Table 3
Groups | Mean deviation (mm3) | Standard error (mm3) | P value |
---|---|---|---|
DPD-NDPD | −188.900 | 70.575 | 0.027* |
DPD-NC | −312.867 | 70.575 | <0.001* |
NDPD-NC | −123.967 | 70.575 | 0.248 |
*, a statistically significant difference after correction by Bonferroni test. The Bonferroni correction probability P value output in this table is the obtained probability P multiplied by the comparison frequency k (k=3), with subsequent comparison with 0.05. DPD, depression in Parkinson disease; NDPD, nondepressed Parkinson disease; NC, normal control.
Table 4
Groups | Mean deviation (mm3) | Standard error (mm3) | P value |
---|---|---|---|
DPD-NDPD | −231.333 | 80.093 | 0.015* |
DPD-NC | −357.633 | 80.093 | <0.001* |
NDPD-NC | −126.300 | 80.093 | 0.355 |
*, a statistically significant difference after correction by Bonferroni test; a statistically significant difference after correction by Bonferroni test. The Bonferroni correction probability P value output in this table is the obtained probability P multiplied by the comparison frequency k (k=3), with subsequent comparison with 0.05. DPD, depression in Parkinson disease; NDPD, nondepressed Parkinson disease; NC, normal control.
Table 5
Clinical scale score | Left hippocampal volume | Right hippocampal volume | |||
---|---|---|---|---|---|
Correlation coefficient | P value | Correlation coefficient | P value | ||
HAMD score | −0.56 | 0.001* | −0.67 | <0.001* |
*, the difference is significantly different. HAMD, Hamilton Depression Rating Scale; DPD, depression in Parkinson disease.
Comparison of FC
Bilateral hippocampi with significant volume differences were considered ROIs for FC analysis with the whole brain. Figure 2 shows the ANOVA results of brain regions with differences in FC based on right hippocampus seed points among the three groups. According to post hoc pairwise comparisons (adjusted P value <0.05 using AlphaSim; cluster size >46 voxels, with one-way ANOVA results as mask) relative to the NC group, the right hippocampus in the DPD group showed reduced FC with the bilateral temporal lobes, right occipital lobes, bilateral frontal lobes, bilateral parietal lobes, and left precuneus. The NDPD group appeared to have reduced FC of the right hippocampus with the bilateral temporal lobes, bilateral frontal lobes, and bilateral parietal lobes when compared with the NC group. However, the FC of the right hippocampus with the right occipital lobe and left precuneus was lower in the DPD group than in the NDPD group (Figure 3, Table 6). No obvious abnormal FC of the left hippocampus was identified in the whole brain. Moreover, correlation analyses indicated that the FC values of the right hippocampus with the right occipital lobe and the left precuneus were negatively correlated with HAMD scores in the DPD group (see Figure 4).
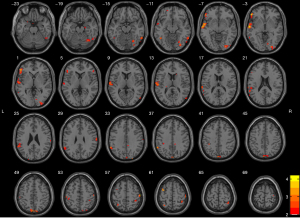
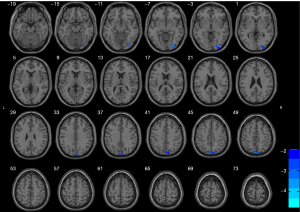
Table 6
Variate | Brain region | Voxel size | Peak MNI coordinates | t | P value | ||
---|---|---|---|---|---|---|---|
x | y | z | |||||
FC | Right occipital lobe | 51 | 27 | −87 | −6 | −4.56 | <0.001* |
Left precuneus | 13 | −3 | −84 | 45 | −4.57 | <0.001* |
*, the difference is significantly different. FC, functional connectivity; DPD, depression in Parkinson disease; NDPD, nondepressed Parkinson disease; MNI, Montreal Neurological Institute.
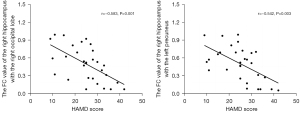
Discussion
Hippocampal volume changes in patients with DPD
Although hippocampal formation has been extensively reported to be related to depression, the majority of relevant studies mainly describe global volumetric changes in the hippocampus based on voxel-based morphometry (VBM) (24-26). The VBM method has some limitations, for example, a susceptibility to artifacts being produced in the segmentation process due to the large difference in the number of voxels at the junction between the brain parenchyma and the cerebrospinal fluid (27). In this study, 3D-T1WI images were obtained using a 3.0-T MRI scanner, and manual segmentation of the hippocampus was performed. Manual segmentation provides advantages over the automated segmentation, which has low accuracy (28).
In our study, the bilateral hippocampal volumes in the DPD group were significantly lower than those of the NDPD and NC groups, but the bilateral hippocampal volumes did not differ considerably between the NC and NDPD groups. In Roddy et al.’s study, patients with DPD showed smaller bilateral hippocampal volumes than did controls (22). It has also been reported that patients with a history of major depressive disorder exhibit smaller bilateral hippocampal volumes than do controls (29,30). Our findings are consistent with these studies. Using VBM, Goto et al. found that depressive symptoms in PD were associated with reduced volume in the left hippocampus and right parahippocampal gyrus (23), which is partially consistent with our findings, with any differences likely being attributable to the limitations of the VBM method. Additionally, our findings indicated that compared to individuals without depression, patients with DPD showed a substantial difference in hippocampal volume. Furthermore, we found that the total hippocampal volume of the right side was significantly larger than that of the left side in the DPD, NDPD, and NC groups, which we suspect might be related to the interhemispheric asymmetry of the brain (31). This suggests that the right hippocampal volume still maintains dominant hemispheric function both in healthy individuals and patients with PD.
Our study also found there to be a negative correlation between the left and right hippocampal volumes and the HAMD scores of patients with DPD. The severity of depressive symptoms of patients was negatively correlated with the left and right hippocampal volumes. In the findings of van Mierlo et al., depression scores were negatively correlated with bilateral hippocampal volumes (32), which is in line with our findings. Another study reported there to be relationship between hippocampal volume and depressive symptoms in other types of depression. In a large meta-analysis of VBM studies, smaller hippocampal volumes were found to be associated with major depressive disorder (33). However, it is unclear if hippocampal atrophy and depression symptoms are causally related. One explanation proposes that chronic stress increases cerebral glucocorticoids by activating the hypothalamic-pituitary-adrenal axis in major depression (34). Due to the high concentration of glucocorticoid receptors in the hippocampus, overexposure to glucocorticoids leads to the impairment of synaptic plasticity in the hippocampus, such as the decrease of neurogenesis, axonal branching, and dendrogenesis (35). Some pathophysiological changes, such as decreased production of neurotrophic factors, decreased neurogenesis, abnormal synaptic plasticity, and neuroinflammation in the hippocampus result in atrophy of the hippocampus in patients with depression (36). It may be that in any depressive disorder (including DPD), the changes in hippocampal volume are related to variations in either the intrinsic neurotrophic factors or extrinsic environmental variables.
In summary, depressive symptoms reduce the bilateral hippocampal volume in patients with PD, and hippocampal volumes are negatively correlated with the severity of depressive disorders. The changes in hippocampus structure may be a critical aspect in the pathophysiology of DPD. MRI can provide a more detailed imaging quantitative basis for depressive symptoms in patients with PD and aid in the clinical diagnosis and treatment of DPD.
rs-FC changes in DPD patients
After volumetric examination, the bilateral hippocampi that showed appreciable variations in gray matter volume were taken as seed points for FC in this study. The FC between the whole brain and the two seed points was then analyzed. Relative to that in the NC group, the right hippocampus in the DPD group showed reduced FC with the bilateral temporal lobes, right occipital lobes, bilateral frontal lobes, bilateral parietal lobes, and left precuneus. The NDPD group appeared to have reduced FC of the right hippocampus with the bilateral temporal lobes, bilateral frontal lobes, and bilateral parietal lobes when compared with the NC group; meanwhile, the DPD group demonstrated a lower FC of the right hippocampus with the right occipital lobe and left precuneus as compared with the NDPD group, and there were no differences of connectivity within the left hippocampus. Differences in FC were confined to the right hippocampus rather, and we suspect that the reason for this result may be related to the asymmetrical character of the pathological lesions in DPD (31), but this remains to be demonstrated. Moreover, correlation analyses showed that the values of FC of the right hippocampus with the right occipital lobe and the left precuneus were negatively correlated with HAMD scores in the DPD group. This suggests that more severe depressive disorders are associated with lower FC and thus that weakened right hippocampal connectivity with the right occipital lobe and left precuneus may play an important role in the emergence of DPD.
As a key structure of limbic circuits, the hippocampus has a crucial role in memory formation, emotional learning, and emotional regulation (7,37). Emotional abnormalities are considered core features of major depression (38). A task-based functional MRI study demonstrated that emotional processing involves the activation of multiple brain regions, including not only limbic structures, such as the hippocampus and amygdala, but also visual areas in the parietal and occipital lobes (39).
The occipital lobe encompasses most of the anatomical localization of the visual cortex that is engaged in external stimulus perception and information transmission to brain regions involved in emotional processing and response. The occipital cortex is part of the frontal-subcortical circuit responsible for emotion regulation (40,41). The structure and function of the occipital lobe are altered in patients with depression. Jaworska et al. observed abnormal visual cortex function in patients with major depression (42). Right occipital lobe dysfunction has also been revealed to be associated with depression (43). Guo et al. reported the decreased amplitude of low-frequency fluctuation (ALFF) in the occipital cortex of patients with depression and suggested that this was associated with major depression (44). Using diffusion tensor imaging (DTI) techniques, Jia et al. found that fractional anisotropy (FA) was much lower in the bilateral frontal and parietal lobes of patients with major depression than in healthy controls, pointing to changes in white matter microarchitecture in these regions (45). These alterations may affect the FC associated with this structure, which could account for the lower FC between the right hippocampus and the right occipital lobe in patients with DPD.
The precuneus of the brain, the medial part of the parietal cortex, is the central component of the default mode network. When the brain is at rest, the precuneus is active and primarily responsible for episodic memory, self-awareness, and self-reflection (46). Halari et al. found there to be abnormal precuneus metabolism in patients with depression (47), and Liang et al. reported decreased precuneus regional homogeneity (ReHO) in the brains of patients with bipolar and unipolar depression (48). It has also been demonstrated that this region experiences less spontaneous neural activity and that the function of the corresponding brain regions is altered (49). There is structural connection between the occipital lobe, parietal lobes, and the hippocampus, as revealed by other anatomical studies in monkeys and DTI studies in humans (50,51). However, the FC between the right hippocampus and the left precuneus is reduced in patients with DPD, which possibly indicates the dysfunctional processing of the right hippocampal FC.
Our study has some limitations. First, the relatively small number of participants included in this study may limit our ability to extrapolate our data to all patients with PD. However, the strict inclusion criteria minimized diagnostic bias. Future large-scale collaborative studies are needed to validate our results. Second, we employed a cross-sectional design. Future studies should include longitudinal follow-up analyses of patients. Third, although the patients with PD in this study had discontinued the relevant medications for at least 12 hours before undergoing magnetic resonance scanning and neuropsychological testing, the potential confounding effect of chronic medications on the experimental results cannot be excluded. Fourth, this study was based on seed point analysis to study changes in FC in DPD, which has the disadvantage of relying on seed selection and is susceptible to bias. We also preprocessed the data before the FC analysis and used the seed point size as a covariate to reduce the error during the FC analysis; however, this still could not completely prevent error. At a later stage, we will consider whether to apply hand-drawn ROIs for whole-brain FC analysis.
Conclusions
In this study, decreased FC of the right hippocampus with the right occipital lobe and the left precuneus was discovered in patients with DPD. Taken together, our results suggest that abnormal FC of the right hippocampus with the right occipital lobe and the left precuneus may play an important role in the neurological emergence of DPD. More importantly, this study opens new directions for further investigations into the neuropathological mechanisms of DPD.
Acknowledgments
Funding: None.
Footnote
Reporting Checklist: The authors have completed the MDAR reporting checklist. Available at https://qims.amegroups.com/article/view/10.21037/qims-23-919/rc
Conflicts of Interest: All authors have completed the ICMJE uniform disclosure form (available at https://qims.amegroups.com/article/view/10.21037/qims-23-919/coif). The authors have no conflicts of interest to declare.
Ethical Statement: The authors are accountable for all aspects of the work in ensuring that questions related to the accuracy or integrity of any part of the work are appropriately investigated and resolved. The study was conducted in accordance with the Declaration of Helsinki (as revised in 2013) and was approved by the Ethics Committee of Nantong First People’s Hospital. Informed consent was taken from all the patients.
Open Access Statement: This is an Open Access article distributed in accordance with the Creative Commons Attribution-NonCommercial-NoDerivs 4.0 International License (CC BY-NC-ND 4.0), which permits the non-commercial replication and distribution of the article with the strict proviso that no changes or edits are made and the original work is properly cited (including links to both the formal publication through the relevant DOI and the license). See: https://creativecommons.org/licenses/by-nc-nd/4.0/.
References
- Wang D, Wu JH, Ren YY. Clinical efficacy of high-frequency repetitive transcranial magnetic stimulation in patients with Parkinson's disease complicated with depression. Journal of International Psychiatry 2021;48:65-9.
- Hu X, Song X, Li E, Liu J, Yuan Y, Liu W, Liu Y. Altered Resting-State Brain Activity and Connectivity in Depressed Parkinson's Disease. PLoS One 2015;10:e0131133. [Crossref] [PubMed]
- Schapira AHV, Chaudhuri KR, Jenner P. Non-motor features of Parkinson disease. Nat Rev Neurosci 2017;18:509. [Crossref] [PubMed]
- Pizzagalli DA. Frontocingulate dysfunction in depression: toward biomarkers of treatment response. Neuropsychopharmacology 2011;36:183-206. [Crossref] [PubMed]
- Liao H, Yi J, Cai S, Shen Q, Liu Q, Zhang L, Li J, Mao Z, Wang T, Zi Y, Wang M, Liu S, Liu J, Wang C, Zhu X, Tan C. Changes in Degree Centrality of Network Nodes in Different Frequency Bands in Parkinson's Disease With Depression and Without Depression. Front Neurosci 2021;15:638554. [Crossref] [PubMed]
- Bhome R, Zarkali A, Thomas GEC, Iglesias JE, Cole JH, Weil RS. Thalamic white matter macrostructure and subnuclei volumes in Parkinson's disease depression. NPJ Parkinsons Dis 2022;8:2. [Crossref] [PubMed]
- Calabresi P, Castrioto A, Di Filippo M, Picconi B. New experimental and clinical links between the hippocampus and the dopaminergic system in Parkinson's disease. Lancet Neurol 2013;12:811-21. [Crossref] [PubMed]
- Converti I, Palermo A, Mancini A, Maggiore ME, Tartaglia GM, Ferrara E, et al. Chewing and cognitive performance: what we know. J Biol Reg Homeos Ag 2022;36:193-204.
- Kronenberg G, Tebartz van Elst L, Regen F, Deuschle M, Heuser I, Colla M. Reduced amygdala volume in newly admitted psychiatric in-patients with unipolar major depression. J Psychiatr Res 2009;43:1112-7. [Crossref] [PubMed]
- van Eijndhoven P, van Wingen G, van Oijen K, Rijpkema M, Goraj B, Jan Verkes R, Oude Voshaar R, Fernández G, Buitelaar J, Tendolkar I. Amygdala volume marks the acute state in the early course of depression. Biol Psychiatry 2009;65:812-8. [Crossref] [PubMed]
- Schwert C, Aschenbrenner S, Weisbrod M, Schröder A. Cognitive Impairments in Unipolar Depression: The Impact of Rumination. Psychopathology 2017;50:347-54. [Crossref] [PubMed]
- Pink A, Przybelski SA, Krell-Roesch J, Stokin GB, Roberts RO, Mielke MM, Knopman DS, Jack CR, Petersen RC, Geda YE. Cortical Thickness and Depressive Symptoms in Cognitively Normal Individuals: The Mayo Clinic Study of Aging. J Alzheimers Dis 2017;58:1273-81. [Crossref] [PubMed]
- Ballmaier M, Kumar A, Thompson PM, Narr KL, Lavretsky H, Estanol L, Deluca H, Toga AW. Localizing gray matter deficits in late-onset depression using computational cortical pattern matching methods. Am J Psychiatry 2004;161:2091-9. [Crossref] [PubMed]
- Chen F, Bertelsen AB, Holm IE, Nyengaard JR, Rosenberg R, Dorph-Petersen KA. Hippocampal volume and cell number in depression, schizophrenia, and suicide subjects. Brain Res 2020;1727:146546. [Crossref] [PubMed]
- Borgonovo J, Allende-Castro C, Laliena A, Guerrero N, Silva H, Concha ML. Changes in neural circuitry associated with depression at pre-clinical, pre-motor and early motor phases of Parkinson's disease. Parkinsonism Relat Disord 2017;35:17-24. [Crossref] [PubMed]
- Maller JJ, Réglade-Meslin C, Anstey KJ, Sachdev P. Sex and symmetry differences in hippocampal volumetrics: before and beyond the opening of the crus of the fornix. Hippocampus 2006;16:80-90. [Crossref] [PubMed]
- Zou Y, Tang W, Qiao X, Li J. Aberrant modulations of static functional connectivity and dynamic functional network connectivity in chronic migraine. Quant Imaging Med Surg 2021;11:2253-64. [Crossref] [PubMed]
- Wang F, Zhang M, Li Y, Li Y, Gong H, Li J, Zhang Y, Zhang C, Yan F, Sun B, He N, Wei H. Alterations in brain iron deposition with progression of late-life depression measured by magnetic resonance imaging (MRI)-based quantitative susceptibility mapping. Quant Imaging Med Surg 2022;12:3873-88. [Crossref] [PubMed]
- Prodoehl J, Burciu RG, Vaillancourt DE. Resting state functional magnetic resonance imaging in Parkinson's disease. Curr Neurol Neurosci Rep 2014;14:448. [Crossref] [PubMed]
- Weingarten CP, Sundman MH, Hickey P, Chen NK. Neuroimaging of Parkinson's disease: Expanding views. Neurosci Biobehav Rev 2015;59:16-52. [Crossref] [PubMed]
- Tahmasian M, Bettray LM, van Eimeren T, Drzezga A, Timmermann L, Eickhoff CR, Eickhoff SB, Eggers C. A systematic review on the applications of resting-state fMRI in Parkinson's disease: Does dopamine replacement therapy play a role? Cortex 2015;73:80-105. [Crossref] [PubMed]
- Roddy DW, Farrell C, Doolin K, Roman E, Tozzi L, Frodl T, O'Keane V, O'Hanlon E. The Hippocampus in Depression: More Than the Sum of Its Parts? Advanced Hippocampal Substructure Segmentation in Depression. Biol Psychiatry 2019;85:487-97. [Crossref] [PubMed]
- Goto M, Kamagata K, Hatano T, Hattori N, Abe O, Aoki S, Hori M, Gomi T. Depressive symptoms in Parkinson's disease are related to decreased left hippocampal volume: correlation with the 15-item shortened version of the Geriatric Depression Scale. Acta Radiol 2018;59:341-5. [Crossref] [PubMed]
- Zacková L, Jáni M, Brázdil M, Nikolova YS, Marečková K. Cognitive impairment and depression: Meta-analysis of structural magnetic resonance imaging studies. Neuroimage Clin 2021;32:102830. [Crossref] [PubMed]
- Gray JP, Müller VI, Eickhoff SB, Fox PT. Multimodal Abnormalities of Brain Structure and Function in Major Depressive Disorder: A Meta-Analysis of Neuroimaging Studies. Am J Psychiatry. 2020;177:422-34. Erratum in: Am J Psychiatry 2022;179:691. [Crossref] [PubMed]
- Chen L, Wang Y, Niu C, Zhong S, Hu H, Chen P, Zhang S, Chen G, Deng F, Lai S, Wang J, Huang L, Huang R. Common and distinct abnormal frontal-limbic system structural and functional patterns in patients with major depression and bipolar disorder. Neuroimage Clin 2018;20:42-50. [Crossref] [PubMed]
- Bergouignan L, Chupin M, Czechowska Y, Kinkingnéhun S, Lemogne C, Le Bastard G, Lepage M, Garnero L, Colliot O, Fossati P. Can voxel based morphometry, manual segmentation and automated segmentation equally detect hippocampal volume differences in acute depression? Neuroimage 2009;45:29-37. [Crossref] [PubMed]
- Malone IB, Leung KK, Clegg S, Barnes J, Whitwell JL, Ashburner J, Fox NC, Ridgway GR. Accurate automatic estimation of total intracranial volume: a nuisance variable with less nuisance. Neuroimage 2015;104:366-72. [Crossref] [PubMed]
- Sheline YI, Wang PW, Gado MH, Csernansky JG, Vannier MW. Hippocampal atrophy in recurrent major depression. Proc Natl Acad Sci U S A 1996;93:3908-13. [Crossref] [PubMed]
- Zaremba D, Enneking V, Meinert S, Förster K, Bürger C, Dohm K, Grotegerd D, Redlich R, Dietsche B, Krug A, Kircher T, Kugel H, Heindel W, Baune BT, Arolt V, Dannlowski U. Effects of cumulative illness severity on hippocampal gray matter volume in major depression: a voxel-based morphometry study. Psychol Med 2018;48:2391-8. [Crossref] [PubMed]
- Minkova L, Habich A, Peter J, Kaller CP, Eickhoff SB, Klöppel S. Gray matter asymmetries in aging and neurodegeneration: A review and meta-analysis. Hum Brain Mapp 2017;38:5890-904. [Crossref] [PubMed]
- van Mierlo TJ, Chung C, Foncke EM, Berendse HW, van den Heuvel OA. Depressive symptoms in Parkinson's disease are related to decreased hippocampus and amygdala volume. Mov Disord 2015;30:245-52. [Crossref] [PubMed]
- Kempton MJ, Salvador Z, Munafò MR, Geddes JR, Simmons A, Frangou S, Williams SC. Structural neuroimaging studies in major depressive disorder. Meta-analysis and comparison with bipolar disorder. Arch Gen Psychiatry 2011;68:675-90. [Crossref] [PubMed]
- Pace TW, Miller AH. Cytokines and glucocorticoid receptor signaling. Relevance to major depression. Ann N Y Acad Sci 2009;1179:86-105. [Crossref] [PubMed]
- Aarsland D, Påhlhagen S, Ballard CG, Ehrt U, Svenningsson P. Depression in Parkinson disease--epidemiology, mechanisms and management. Nat Rev Neurol 2011;8:35-47. [Crossref] [PubMed]
- Kim HK, Nunes PV, Oliveira KC, Young LT, Lafer B. Neuropathological relationship between major depression and dementia: A hypothetical model and review. Prog Neuropsychopharmacol Biol Psychiatry 2016;67:51-7. [Crossref] [PubMed]
- Gou H, Zhu Y, He J, Chen Y, Sun J, Zhu Y. Celecoxib Attenuates Brain Hippocampal Damage in PTZ-Induced Epilepsy Rats by Inhibiting NF-κB. J Biol Reg Homeos Ag 2022;36:1947-56.
- Burke J, McQuoid DR, Payne ME, Steffens DC, Krishnan RR, Taylor WD. Amygdala volume in late-life depression: relationship with age of onset. Am J Geriatr Psychiatry 2011;19:771-6. [Crossref] [PubMed]
- Colich NL, Ho TC, Foland-Ross LC, Eggleston C, Ordaz SJ, Singh MK, Gotlib IH. Hyperactivation in Cognitive Control and Visual Attention Brain Regions During Emotional Interference in Adolescent Depression. Biol Psychiatry Cogn Neurosci Neuroimaging 2017;2:388-95. [Crossref] [PubMed]
- Qiu L, Lui S, Kuang W, Huang X, Li J, Li J, Zhang J, Chen H, Sweeney JA, Gong Q. Regional increases of cortical thickness in untreated, first-episode major depressive disorder. Transl Psychiatry 2014;4:e378. [Crossref] [PubMed]
- Hakamata Y, Sato E, Komi S, Moriguchi Y, Izawa S, Murayama N, Hanakawa T, Inoue Y, Tagaya H. The functional activity and effective connectivity of pulvinar are modulated by individual differences in threat-related attentional bias. Sci Rep 2016;6:34777. [Crossref] [PubMed]
- Jaworska N, Yang XR, Knott V, MacQueen G. A review of fMRI studies during visual emotive processing in major depressive disorder. World J Biol Psychiatry 2015;16:448-71. [Crossref] [PubMed]
- Kraus C, Klöbl M, Tik M, Auer B, Vanicek T, Geissberger N, Pfabigan DM, Hahn A, Woletz M, Paul K, Komorowski A, Kasper S, Windischberger C, Lamm C, Lanzenberger R. The pulvinar nucleus and antidepressant treatment: dynamic modeling of antidepressant response and remission with ultra-high field functional MRI. Mol Psychiatry 2019;24:746-56. [Crossref] [PubMed]
- Guo WB, Liu F, Xue ZM, Xu XJ, Wu RR, Ma CQ, Wooderson SC, Tan CL, Sun XL, Chen JD, Liu ZN, Xiao CQ, Chen HF, Zhao JP. Alterations of the amplitude of low-frequency fluctuations in treatment-resistant and treatment-response depression: a resting-state fMRI study. Prog Neuropsychopharmacol Biol Psychiatry 2012;37:153-60. [Crossref] [PubMed]
- Jia Z, Huang X, Wu Q, Zhang T, Lui S, Zhang J, Amatya N, Kuang W, Chan RC, Kemp GJ, Mechelli A, Gong Q. High-field magnetic resonance imaging of suicidality in patients with major depressive disorder. Am J Psychiatry 2010;167:1381-90. [Crossref] [PubMed]
- Grimm S, Boesiger P, Beck J, Schuepbach D, Bermpohl F, Walter M, Ernst J, Hell D, Boeker H, Northoff G. Altered negative BOLD responses in the default-mode network during emotion processing in depressed subjects. Neuropsychopharmacology 2009;34:932-43. [Crossref] [PubMed]
- Halari R, Simic M, Pariante CM, Papadopoulos A, Cleare A, Brammer M, Fombonne E, Rubia K. Reduced activation in lateral prefrontal cortex and anterior cingulate during attention and cognitive control functions in medication-naïve adolescents with depression compared to controls. J Child Psychol Psychiatry 2009;50:307-16. [Crossref] [PubMed]
- Liang MJ, Zhou Q, Yang KR, Yang XL, Fang J, Chen WL, Huang Z. Identify changes of brain regional homogeneity in bipolar disorder and unipolar depression using resting-state FMRI. PLoS One 2013;8:e79999. [Crossref] [PubMed]
- Peng DH, Jiang KD, Fang YR, Xu YF, Shen T, Long XY, Liu J, Zang YF. Decreased regional homogeneity in major depression as revealed by resting-state functional magnetic resonance imaging. Chin Med J (Engl) 2011;124:369-73.
- Renier LA, Anurova I, De Volder AG, Carlson S, VanMeter J, Rauschecker JP. Preserved functional specialization for spatial processing in the middle occipital gyrus of the early blind. Neuron 2010;68:138-48. [Crossref] [PubMed]
- McIntyre CK, Marriott LK, Gold PE. Cooperation between memory systems: acetylcholine release in the amygdala correlates positively with performance on a hippocampus-dependent task. Behav Neurosci 2003;117:320-6. [Crossref] [PubMed]