Choline as a specific manifestation of delayed encephalopathy after acute carbon monoxide poisoning: a description of two cases
Introduction
Carbon monoxide, a colorless and odorless toxic gas, causes carbon monoxide poisoning when carbonaceous substances are burned incompletely (1). Some patients with acute carbon monoxide intoxication can develop a series of neurological and psychiatric disorders after a “pseudo-healing period” of 2 to 60 days after recovery of consciousness, which is referred to as delayed encephalopathy after acute carbon monoxide poisoning (DEACMP). DEACMP is a common complication of acute carbon monoxide poisoning, with a prevalence of 3% to 40%, and leads to permanent neurological deficits in approximately 25% of patients. However, the clinical symptoms of DEACMP are not specific (2), which causes some difficulties in the diagnosis of patients with an unclear medical history.
Proton magnetic resonance spectroscopy (1H MRS) is a detection method that uses the magnetic resonance chemical shift phenomenon to determine the molecular composition of constituent substances and is the only noninvasive method that can detect metabolites in living tissues. With developments in magnetic resonance field strength, detection sequences, and data analysis methods, advanced MRS techniques can reliably and efficiently detect metabolite concentrations, including glutamate, glutamine, gamma-amino butyric acid, glutamate-glutamine, N-acetyl aspartate (NAA), and creatine (Cr), in specific brain regions (3). Moreover, metabolite changes in carbon monoxide poisoning can be measured by 1H MRS (4,5). Herein, we report two cases of MRS in patients with DEACMP, with the aim of proposing a new approach to the diagnosis of this disease.
Case presentation
All procedures performed in this study were in accordance with the relevant ethical standards of the institutional and/or national research committee(s) and with the Helsinki Declaration (as revised in 2013). Written informed consent was obtained from the patients for publication of this case report and accompanying images. A copy of the written consent is available for review by the editorial office of this journal.
Patient 1
A 49-year-old woman was admitted to the hospital after a week of abnormal behavior and unresponsiveness. According to her family, she had been burning firewood in a partially confined space 2 months prior. The patient was subsequently admitted to the hospital due to drowsiness, and her arterial carboxyhemoglobin (COHb) level was 21.1% on admission. The patient was treated and discharged. Thereafter, the patient had no abnormalities until the onset of symptoms a week prior to presentation. The patient was admitted with a temperature of 36.5 ℃, pulse rate of 72 beats/min, respiration of 20 breaths/min, and blood pressure of 127/72 mmHg. The patient was previously healthy, with no history of hypertension or infectious diseases and no family history of genetic diseases. The results of her neurological examination are as follows: clear consciousness, unresponsiveness, memory loss, calculation decrease, disorientation, and clear speech. After admission, the patient’s condition progressively worsened, with symptoms of resting tremor, increased muscle tone, and hyperactive tendon reflexes. The relevant cognitive assessments were as follows: Mini-Mental State Examination, 1 point (reference value: literacy primary >20 points); Montreal Cognitive Assessment scale, 6 points (reference range: ≥26 points); and Anxiety and Depression Scale, patient unable to cooperate. Laboratory test results on admission were as follows: low-density lipoprotein (LDL), 4.03 mmol/L (reference range: <3.4 mmol/L); and vitamin B12, 78.13 pmol/L (reference range: 103 to 516 pmol/L). Blood sedimentation, infection immune quadruple, glycated hemoglobin, antinuclear antibody (ANA) and extractable nuclear antigens (ENAs) profile, homocysteine, and tumor markers were all normal.
Brain magnetic resonance imaging (MRI) showed large lamellar long T1 and long T2 signals symmetrically distributed in the white matter (WM) of the bilateral cerebral hemispheres, with symmetrical high signal on diffusion-weighted imaging (DWI) and equal and slightly low signal on the apparent diffusion coefficient (ADC). T2 fluid-attenuated inversion recovery (FLAIR) showed symmetrically distributed low signal foci in the bilateral pallidum, with high signal rings at the edges (Figure 1). MRS showed a decrease in the bilateral frontal NAA peak and an increase in the choline (Cho) peak (Figure 2). Based on the patient’s history of exposure and the characteristic imaging presentation, a diagnosis of DEACMP was made. Finally, the patient was transferred to the hospital because she needed hyperbaric oxygen therapy.
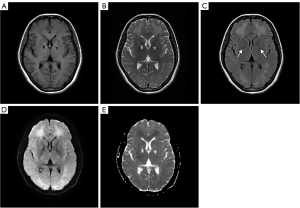
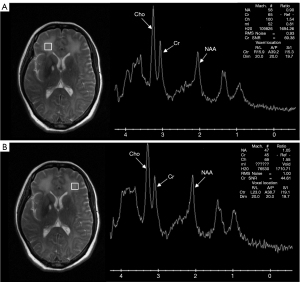
Patient 2
The husband of patient 1, a 55-year-old man, was admitted to the Department of Neurology of the Second Hospital of Xiamen Medical College with blunted reflexes for >2 months. Patient 2 had the same history as patient 1, developed drowsiness after burning wood in a partially confined space, and had a COHb level of 16.3% on admission. He was treated and discharged with recovery. However, 14 days after the CO poisoning, the patient developed slower speech, memory loss, and a feeling of walking lightly, and he became unresponsive. The patient was admitted with a temperature of 36.4 ℃, a pulse rate of 60 beats/min, respiration of 18 breaths/min, and blood pressure of 100/69 mmHg. The patient had a history of lumbar disc herniation for several years but no history of hypertension, coronary heart disease, diabetes mellitus, hepatitis, or tuberculosis. The results of the patient’s neurological examination were as follows: clear consciousness, unresponsiveness, memory loss, calculation loss, disorientation, and clear speech. Muscle strength and tone as well as nerve reflexes were normal, and pathological signs were negative. The relevant cognitive assessments were as follows: Mini-Mental State Examination, 19 points (reference range: junior high school education >24 points); Montreal Cognitive Assessment scale, 8 points (reference value: ≥26 points); and Anxiety and Depression Scale, patient unable to cooperate. The following laboratory tests were performed on admission: routine blood, C-reaction protein, coagulation tetramer, D-dimer, routine urine, blood sedimentation, infection immune tetramer, and tumor markers. The results of all tests were normal.
Brain MRI showed symmetrical lamellar long-T1 and long-T2 signal foci in the WM and a semioval center of the frontal-occipital lobe bilaterally, with high signals in T2 FLAIR and DWI (Figure 3). MRS showed a slight decrease in bilateral frontal NAA peak and a significant increase in Cho peak (Figure 4). Although his MRI findings were nonspecific, the combination of his carbon monoxide exposure history and his wife’s diagnosis led to the diagnosis of DEACMP. Subsequently, the patient was transferred to the hospital, also for hyperbaric oxygen therapy.
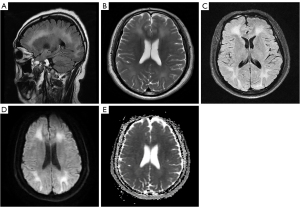

Discussion
DEACMP is a group of neuropsychiatric symptoms, mainly acute dementia, that reappears after a “pseudo-healing” period of several days or weeks of normal or near-normal performance after resuscitation and recovery from acute carbon monoxide poisoning (6). DEACMP is diagnosed on the basis of neurological symptoms presenting after a pseudo-healing period and confirmed by novel hyperintense WM lesions on T2-weighted imaging and FLAIR (7). In addition, a clear history of carbon monoxide exposure contributes to the diagnosis of DEACMP.
The couple in this report were brought to the clinic for unresponsiveness, and the wife also had abnormal behavior. The wife had bilateral pallid bulb and symmetrical abnormal signal lesions, which is the characteristic presentation of DEACMP. MRS showed a noticeable decrease in NAA/Cr in the wife and a slight decrease in the husband, but a significant increase in Cho/Cr in both (Figure 5). For more accurate measurement of individual metabolite levels, we used a linear combination of the model spectra (Section 6.3 of the LCModel manual). Using the absolute values of NAA and Cho measured by the LCModel, we obtained the same results as described above. In most previous studies (7-9), encephalopathy in carbon monoxide poisoning was accompanied by a decrease in NAA and an increase in Cho, which is consistent with our results. Similarly, studies on the period of appearance of metabolites report an early increase in the Cho:Cr ratio in metabolites and a decrease in the late chronic phase; in these studies, the earliest decrease in the NAA:Cr ratio started at 3 to 4 weeks (4,8,10,11). In DEACMP, Cho increases with increased membrane metabolism due to cell membrane disruption or demyelination (9,12,13), whereas NAA decreases due to neuronal damage or dysfunction (8). Myelin rupture after injury produces more nerve growth inhibitory factors and thus inhibits neuronal axon regeneration (6); this would explain why increased Cho appears before decreased NAA. Consequently, Cho may be a more sensitive indicator than NAA for the diagnosis of DEACMP.
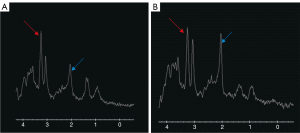
At present, the main examination method for the diagnosis of DEACMP is MRI, but it is not specific for patients with only WM lesions, and our report provides a new specific examination method for its differential diagnosis. In this case report, the husband had only nonspecific cerebral WM lesions, which posed some diagnostic obstacles. Fortunately, the two patients were a couple with the same history of carbon monoxide exposure, and the wife had specific MR manifestations of carbon monoxide poisoning encephalopathy, which aided in the husband’s diagnosis. Furthermore, we found that in both patients the Cho level was significantly increased on MRS. This suggests that changes in metabolite level may be more specific in the differential diagnosis of DEACMP compared to changes in MRI.
Some limitations to this study include the small number of cases and insufficient follow-up information; nevertheless, this report provides valuable information and suggestions for diagnosing DEACMP.
In conclusion, the main examination method for DEACMP is currently MRI, which has a degree of reference value for diagnosis but low specificity. Therefore, we propose a new method based on this to detect the changes in the patient’s brain by MRS at the metabolite level. Meanwhile, our results suggest that increased Cho level may be a specific manifestation of DEACMP, which may help to identify the nonspecific WM lesion phase of the disease.
Acknowledgments
Funding: This research was supported by
Footnote
Conflicts of Interest: All authors have completed the ICMJE uniform disclosure form (available at https://qims.amegroups.com/article/view/10.21037/qims-23-902/coif). The authors have no conflicts of interest to declare.
Ethical Statement: The authors are accountable for all aspects of the work in ensuring that questions related to the accuracy or integrity of any part of the work are appropriately investigated and resolved. All procedures performed in this study were in accordance with the ethical standards of the institutional and/or national research committee(s) and with the Helsinki Declaration (as revised in 2013). Written informed consent was obtained from the patients for publication of this case report and accompanying images. A copy of the written consent is available for review by the editorial office of this journal.
Open Access Statement: This is an Open Access article distributed in accordance with the Creative Commons Attribution-NonCommercial-NoDerivs 4.0 International License (CC BY-NC-ND 4.0), which permits the non-commercial replication and distribution of the article with the strict proviso that no changes or edits are made and the original work is properly cited (including links to both the formal publication through the relevant DOI and the license). See: https://creativecommons.org/licenses/by-nc-nd/4.0/.
References
- Lo CP, Chen SY, Chou MC, Wang CY, Lee KW, Hsueh CJ, Chen CY, Huang KL, Huang GS. Diffusion-tensor MR imaging for evaluation of the efficacy of hyperbaric oxygen therapy in patients with delayed neuropsychiatric syndrome caused by carbon monoxide inhalation. Eur J Neurol 2007;14:777-82. [Crossref] [PubMed]
- Ng PC, Long B, Koyfman A. Clinical chameleons: an emergency medicine focused review of carbon monoxide poisoning. Intern Emerg Med 2018;13:223-9. [Crossref] [PubMed]
- Puts NA, Edden RA. In vivo magnetic resonance spectroscopy of GABA: a methodological review. Prog Nucl Magn Reson Spectrosc 2012;60:29-41. [Crossref] [PubMed]
- Beppu T. The role of MR imaging in assessment of brain damage from carbon monoxide poisoning: a review of the literature. AJNR Am J Neuroradiol 2014;35:625-31. [Crossref] [PubMed]
- Hurley RA, Hopkins RO, Bigler ED, Taber KH. Applications of functional imaging to carbon monoxide poisoning. J Neuropsychiatry Clin Neurosci 2001;13:157-60. [Crossref] [PubMed]
- Huang YQ, Peng ZR, Huang FL, Yang AL. Mechanism of delayed encephalopathy after acute carbon monoxide poisoning. Neural Regen Res 2020;15:2286-95. [Crossref] [PubMed]
- Kuroda H, Fujihara K, Mugikura S, Takahashi S, Kushimoto S, Aoki M. Altered white matter metabolism in delayed neurologic sequelae after carbon monoxide poisoning: A proton magnetic resonance spectroscopic study. J Neurol Sci 2016;360:161-9. [Crossref] [PubMed]
- Kondziella D, Danielsen ER, Hansen K, Thomsen C, Jansen EC, Arlien-Soeborg P. 1H MR spectroscopy of gray and white matter in carbon monoxide poisoning. J Neurol 2009;256:970-9. [Crossref] [PubMed]
- Yang KC, Yang BH, Lirng JF, Liu MN, Hu LY, Liou YJ, Chan LA, Chou YH. Interaction of dopamine transporter and metabolite ratios underpinning the cognitive dysfunction in patients with carbon monoxide poisoning: A combined SPECT and MRS study. Neurotoxicology 2021;82:26-34. [Crossref] [PubMed]
- Terajima K, Igarashi H, Hirose M, Matsuzawa H, Nishizawa M, Nakada T. Serial assessments of delayed encephalopathy after carbon monoxide poisoning using magnetic resonance spectroscopy and diffusion tensor imaging on 3.0T system. Eur Neurol 2008;59:55-61. [Crossref] [PubMed]
- Prockop LD. Carbon monoxide brain toxicity: clinical, magnetic resonance imaging, magnetic resonance spectroscopy, and neuropsychological effects in 9 people. J Neuroimaging 2005;15:144-9. [Crossref] [PubMed]
- Govind V, Gold S, Kaliannan K, Saigal G, Falcone S, Arheart KL, Harris L, Jagid J, Maudsley AA. Whole-brain proton MR spectroscopic imaging of mild-to-moderate traumatic brain injury and correlation with neuropsychological deficits. J Neurotrauma 2010;27:483-96. [Crossref] [PubMed]
- Beppu T, Nishimoto H, Fujiwara S, Kudo K, Sanjo K, Narumi S, Oikawa H, Onodera M, Ogasawara K, Sasaki M. 1H-magnetic resonance spectroscopy indicates damage to cerebral white matter in the subacute phase after CO poisoning. J Neurol Neurosurg Psychiatry 2011;82:869-75. [Crossref] [PubMed]